DOI:
10.1039/D2RA00213B
(Paper)
RSC Adv., 2022,
12, 5386-5394
Nano-[Fe3O4@SiO2/N-propyl-1-(thiophen-2-yl)ethanimine][ZnCl2] as a nano magnetite Schiff base complex and heterogeneous catalyst for the synthesis of pyrimido[4,5-b]quinolones†
Received
11th January 2022
, Accepted 4th February 2022
First published on 14th February 2022
Abstract
Nano-[Fe3O4@SiO2/N-propyl-1-(thiophen-2-yl)ethanimine][ZnCl2] as a nano magnetite Schiff base complex was designed and fully characterized by various analyses such as Fourier transform infrared spectroscopy (FT-IR), energy-dispersive X-ray spectroscopy (EDX), X-ray diffraction (XRD), thermal gravimetric analysis (TGA), differential thermal gravimetric analysis (DTA), vibrating sample magnetometry (VSM), scanning electron microscopy (SEM), and transmission electron micrographs (TEM). The presented nano magnetite Schiff base complex was used as a heterogeneous catalyst for the synthesis of pyrimido[4,5-b]quinolones by the reaction of aryl aldehyde, dimedone and 6-amino-1,3-dimethyluracil in EtOH
:
H2O (7
:
3) as a solvent at 60 °C.
1. Introduction
Heterocyclic compounds containing pyrimidine moieties in their structure show an important role in medicinal chemistry.1 Biological properties of pyrimido[4,5-b]quinolones give them special activities in medicinal chemistry such as antifungal,2 anticancer,3 antimalarial,4 antiviral,5 anticancer,6 anti-inflammatory,7 antihistaminic,8 and anti-inflammatory activities.9 A significant method for the preparation of pyrimido[4,5-b]quinolones is the multi-component reaction of aryl aldehyde, dimedone and 6-amino-1,3-dimethyluracil.1 Multi-component reactions are one of the most successful methods in organic chemistry to increase the structural diversity and molecular complexity using a simple process without the formation of side-products and save energy, time, solvent and chemical materials and reduce the chemical waste.10–16 Multi-component reaction of aryl aldehyde, dimedone and 6-amino-1,3-dimethyluracil is carried out by various catalysts including nano-[Fe3O4@-SiO2@R-NHMe2][H2PO4],1 SBA-15/PrN(CH2PO3H2)2,17 [TSSECM],18 Fe3O4@cellulose sulfuric acid,19 Agar-entrapped sulfonated DABCO,20 [C4(DABCO)2]·2OH,21 nanocrystalline MgO,22 [H2-DABCO][ClO4]2 (ref. 23) and [bmim]Br.24 Due to the importance of pyrimido[4,5-b]quinolones in medicinal chemistry, finding new methods for the preparation of them is still required.
Heterogeneous catalysts are a group of catalysts in which the starting materials and the catalyst are not in the same phase. Increasing the active surface of the catalyst to create a suitable substrate for the reaction and also the stability of this substrate, which causes its reusability of the catalyst, is one of their special advantages.25
Adsorption of starting materials on the surface of the catalyst, reaction and desorption of chemical products is done on the active surface of the heterogeneous catalyst. Faster production of large-scale products, selective products formation and recovery of this group of catalysts are features that have made them significant in the chemical industry.26
The placement of these catalysts on a magnetic iron oxide surface, due to its stability, ease of preparation and cheapness of it, gives them some capabilities, including the simple separation of the catalyst from the reaction mixture by an external magnet without filtration.27–33
The zinc(II) ions are important factors for many biological processes, their complexes being present in a wide range of biological processes. Also, Zn complexes containing thiophene moieties show antimycobacterial properties. These complexes show higher biological activities than free metal ion.34–36 Having above facts, in continues to previous works on the design and use heterogeneous catalyst based on Schiff base complex in organic synthesis,37–43 we have introduced a new nano magnetite Schiff base complex namely, nano-[Fe3O4@SiO2/N-propyl-1-(thiophen-2-yl)ethanimine][ZnCl2] and used it as an efficient heterogeneous catalyst for the preparation of pyrimido[4,5-b]quinolones by the reaction of aryl aldehyde, dimedone and 6-amino-1,3-dimethyluracil (Scheme 1).
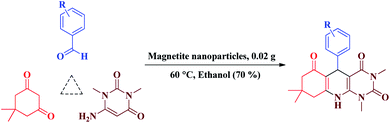 |
| Scheme 1 The preparation of pyrimido[4,5-b]quinolones. | |
2. Results and discussion
2.1. Synthesis and characterization of catalyst
Zinc(II) Schiff base complexes in tetrahedral form with coordination of a Schiff base ligand containing thiophene ring and salicylaldehyde derivatives are previously reported.36,44 With this issue in mind, we have decided to design a new nano magnetite Schiff base complex of zinc(II) which coordinated with a ligand containing thiophene moieties.
For this purpose, to design and preparation of the nano magnetite Schiff base complex, initially by the reaction of (3-aminopropyl)triethoxysilane with 2-acetyl thiophen the Schiff base ligand was prepared. ZnCl2 was added to prepared ligand and stirred in dry toluene to give (OEt)3Si/N-propyl-1-(thiophen-2-yl)ethanimine as a Schiff base complex. In the next step, Fe3O4@SiO2 nanoparticles were prepared according to previous literature30,31 and dispersed in toluene and then reacted with Schiff base complex to give nano-[Fe3O4@SiO2/N-propyl-1-(thiophen-2-yl)ethanimine][ZnCl2] as a nano magnetite Schiff base complex (Scheme 2). The presented nano particles were fully characterized by various analyses including FT-IR, EDX, SEM mapping, FESEM, TEM, TGA, DTG and VSM analyses.
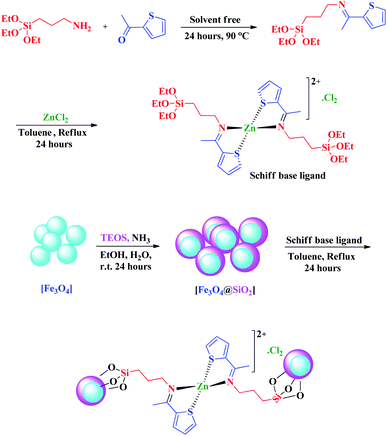 |
| Scheme 2 The preparation of nano-[Fe3O4@SiO2/N-propyl-1-(thiophen-2-yl)ethanimine][ZnCl2]. | |
FT-IR spectrum of nano-[Fe3O4@SiO2/N-propyl-1-(thiophen-2-yl)ethanimine][ZnCl2] was studied. According that, the peaks which observed at 1104 cm−1 and 790 cm−1 could be related to the un-symmetric and symmetric linear stretching vibrations of Si–O–Si bonding respectively.45 Also, another peak at 1604 cm−1 could be corresponded to stretching mode of C
N bond in nano-[Fe3O4@SiO2/N-propyl-1-(thiophen-2-yl)ethanimine][ZnCl2] which coordinated with zinc chloride (Fig. 1).
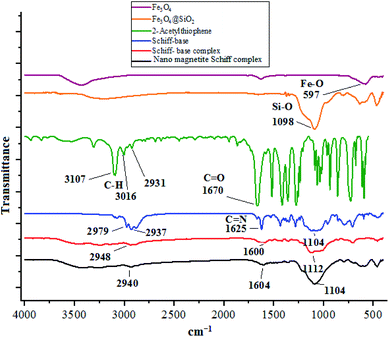 |
| Fig. 1 The FT-IR spectrum of nano magnetite Schiff base complex in comparison with other species in its structure. | |
To show the presence of the expected elements in nano magnetite Schiff base complex, energy-dispersive X-ray spectroscopy (EDX) from nano-[Fe3O4@SiO2/N-propyl-1-(thiophen-2-yl)ethanimine][ZnCl2] was studied and the desired elements including carbon, oxygen, nitrogen, sulfur, iron, silicon, zinc and chlorine were presented in the structure of nano magnetite Schiff base complex (Fig. 2).
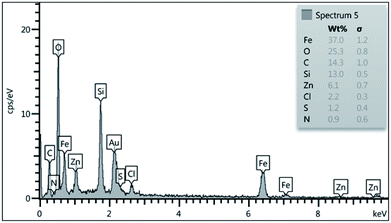 |
| Fig. 2 The EDX analysis of nano magnetite Schiff base complex. | |
In another study, to show the expected elements in the catalyst, the SEM analysis was coupled with EDX analysis (SEM mapping) to determine carbon, oxygen, nitrogen, sulfur, iron, silicon, zinc and chlorine in the structure of nano magnetite Schiff base complex (Fig. 3).
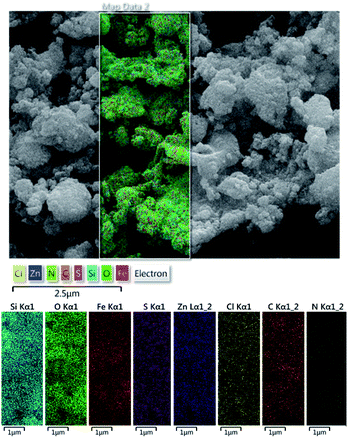 |
| Fig. 3 SEM coupled EDX (SEM mapping) of nano magnetite Schiff base complex. | |
The morphology size of the prepared nano magnetite Schiff base complex were studied by the scanning electron microscope (SEM) micrographs of nano-[Fe3O4@SiO2/N-propyl-1-(thiophen-2-yl)ethanimine][ZnCl2]. According this analysis, indicates that the particles were prepared in nano size, less than 100 nanometers (Fig. 4).
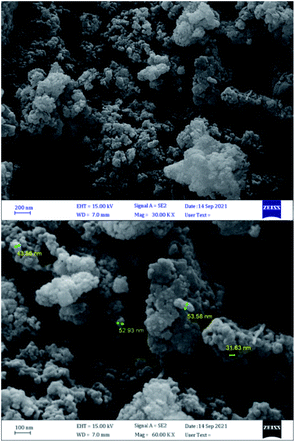 |
| Fig. 4 The FESEM analysis of nano magnetite Schiff base complex. | |
For further studies on the size of prepared nano particles of the catalyst, the presented particles of nano-[Fe3O4@SiO2/N-propyl-1-(thiophen-2-yl)ethanimine][ZnCl2], were studied by transmission electron microscopy (TEM). As it is shown in Fig. 5, indicates that, some particles of the catalyst were prepared in nano size (Fig. 5).
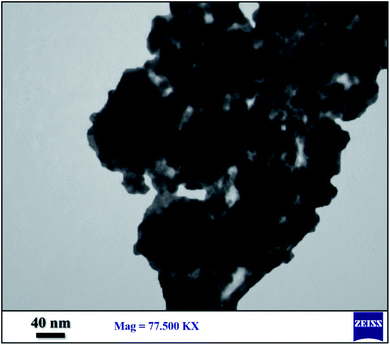 |
| Fig. 5 TEM image of nano magnetite Schiff base complex. | |
To show the thermal stability of nano magnetite catalyst in organic reactions, thermal gravimetric analysis (TGA) of nano-[Fe3O4@SiO2/N-propyl-1-(thiophen-2-yl)ethanimine][ZnCl2] was studied. According that, the prepared catalyst could be used until 220 °C without any special changes in its chemical structure (Fig. 6). By the increasing the temperature more than 220 °C, the organic part of the catalyst namely Schiff base ligand will be decomposed. Weight loss at this temperature may be due to the organic part leaving the catalyst structure.
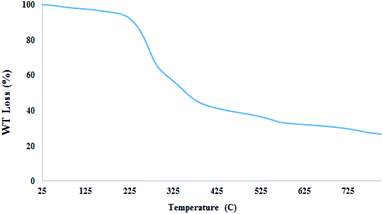 |
| Fig. 6 Thermal gravimetric analysis (TGA) of nano magnetite Schiff base complex. | |
The magnetic behavior of nano magnetite catalyst was measured using vibrating sample magnetometer (VSM) at room temperature. The saturation magnetizations for nano-[Fe3O4@SiO2/N-propyl-1-(thiophen-2-yl)ethanimine][ZnCl2] was found to be 22 emu g−1. The related hysteresis loop of the nano magnetite catalyst is depicted in Fig. 7.
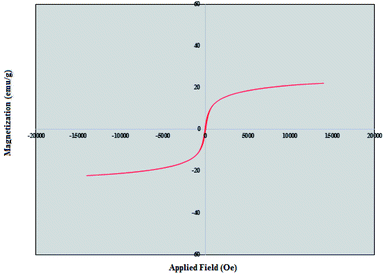 |
| Fig. 7 Vibrating sample magnetometer (VSM) analysis of nano magnetite Schiff base complex. | |
2.2. Catalytic properties of the catalyst
After the preparation and identification of nano-[Fe3O4@SiO2/N-propyl-1-(thiophen-2-yl)ethanimine][ZnCl2] as a nano magnetite Schiff base complex, the catalytic application of it was tested on the synthesis of pyrimido[4,5-b]quinolones. For this purpose, the reaction of benzaldehyde, 6-amino-1,3-dimethyluracil and dimedone was considered as a model reaction and the effect of the catalyst amounts, temperature and various solvents were examined on this reaction. The results were depicted in Table 1. According that, the best result was obtained using 20 mg of nano magnetite catalyst in EtOH
:
H2O (7
:
3) as a solvent at 60 °C. various solvent including ethyl acetate, n-hexane, CH2Cl2, CHCl3, CH3CN and H2O, which using in this reaction, did not improve the yields and reaction times of products. Also, the solvent-free and catalyst-free conditions were tested on the model reaction which there was no significant progress (Table 1).
Table 1 The effect of the catalyst amount, kinds of solvent and temperature on the reaction of benzaldehyde (1 mmol), 6-amino-1,3-dimethyluracil (1 mmol) and dimedone (1 mmol) in the presence of nano magnetite catalyst
Entry |
Solvent |
Catalyst amount (mg) |
Temp. (°C) |
Time (min) |
Yielda (%) |
Isolated yield. |
1 |
EtOH : H2O (7 : 3) |
10 |
60 |
20 |
86 |
2 |
EtOH : H2O (7 : 3) |
20 |
60 |
20 |
93 |
3 |
EtOH : H2O (7 : 3) |
30 |
60 |
20 |
92 |
4 |
EtOH : H2O (7 : 3) |
40 |
60 |
20 |
90 |
5 |
EtOH : H2O (7 : 3) |
20 |
r.t. |
270 |
65 |
6 |
EtOH : H2O (7 : 3) |
20 |
Reflux |
20 |
90 |
7 |
H2O |
20 |
Reflux |
30 |
80 |
8 |
Ethyl acetate |
20 |
Reflux |
30 |
40 |
9 |
CHCl3 |
20 |
Reflux |
30 |
24 |
10 |
CH2Cl2 |
20 |
Reflux |
30 |
Trace |
11 |
n-Hexane |
20 |
Reflux |
30 |
Trace |
12 |
CH3CN |
20 |
Reflux |
360 |
16 |
13 |
EtOH |
20 |
Reflux |
20 |
85 |
14 |
— |
20 |
60 |
60 |
Trace |
15 |
EtOH : H2O (7 : 3) |
— |
Reflux |
360 |
Trace |
2.3. Efficiency of the catalyst
After finding the best reaction condition, to show the efficiency of the catalyst, for the preparation of pyrimido[4,5-b]quinolones using nano magnetite catalyst, various aromatic aldehydes containing electron withdrawing substituents, electron-releasing substituents and halogens, were examined in this reaction to demonstrate the generality and capability of the catalyst (Table 2). As it is depicted in Table 2, indicates that the presented catalyst successfully carried out the reaction with high yields and suitable reaction times.
Table 2 Synthesis of pyrimido[4,5-b]quinolones using nano magnetite catalyst
Entry |
Product |
Time (min) |
Yielda (%) |
Mp °C (Lit.)ref |
Isolated yield. |
1 |
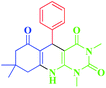 |
20 |
93 |
282–284 (280–285)17 |
2 |
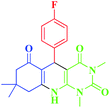 |
20 |
95 |
232–234 (232–236)19 |
3 |
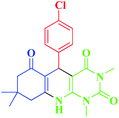 |
20 |
94 |
295–297 (296–297)1 |
4 |
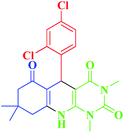 |
20 |
95 |
323–326 (>300)1 |
5 |
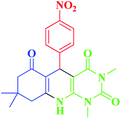 |
20 |
94 |
236–239 (234)19 |
6 |
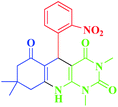 |
20 |
87 |
288–290 (279–280)1 |
7 |
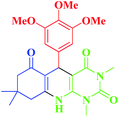 |
20 |
62 |
238–240 (—) |
8 |
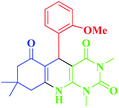 |
20 |
68 |
305 (>300)19 |
9 |
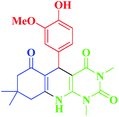 |
20 |
92 |
328–330 (—) |
10 |
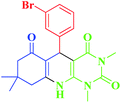 |
20 |
74 |
280–280 (285–287)1 |
11 |
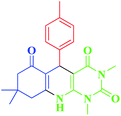 |
20 |
50 |
299–303 (>300)17 |
12 |
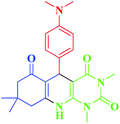 |
20 |
45 |
251–254 (253–255)1 |
In a proposed mechanism which is supported by the literature,17–24,44 in the first step, dimedone in enole form was reacted with activated aldehyde by the catalyst to give (I) and then by removing of one molecule of H2O, intermediate (II) as an Michael acceptor was prepared. In the next step, by the reaction of 6-amino-1,3-dimethyluracil with (II), which was activated by the catalyst, (III) was obtained. Finally, by the intramolecular nucleophilic attack in intermediate (III), the desired product was prepared after removing of one molecule of H2O and tautomerization (Scheme 3).
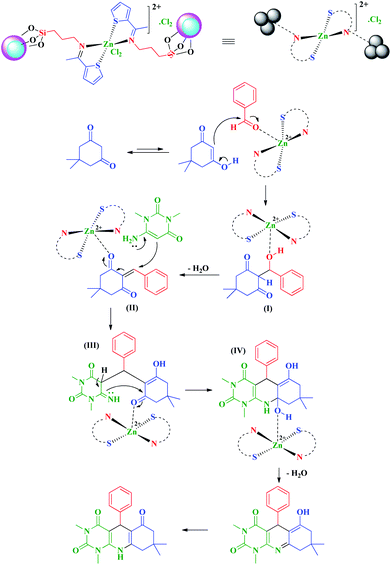 |
| Scheme 3 The proposed mechanism for the synthesis of pyrimido[4,5-b]quinolones. | |
2.4. The recovery of the catalyst
To examine the reusability of nano magnetite catalyst, the reaction of benzaldehyde, 6-amino-1,3-dimethyluracil and dimedone as a model reaction was tested in the presence of reused catalyst, which the time and yield of the reactions are acceptable for two runs (Fig. 8). The IR spectrum of fresh catalyst and reused catalyst was compared with each other to confirm the stability of catalyst after reusing it for two runs (Fig. 9).
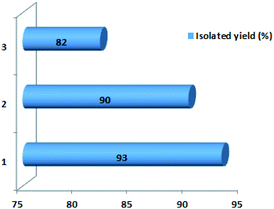 |
| Fig. 8 The reusability of the catalyst. The reactions were carried out for the synthesis of compound (1) in EtOH : H2O (7 : 3) using 0.02 g of catalyst within 20 minutes at 60 °C. | |
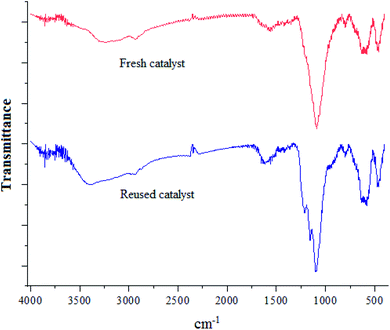 |
| Fig. 9 The IR spectrum of fresh catalyst in comparison with reused catalyst. | |
To show the applicability and the efficiency of nano magnetite catalyst with some previous reported catalysts for the preparation of pyrimido[4,5-b]quinolones, we have summarised the results of these catalysts for the reaction of 4-chlorobenzaldehyde, dimedone and 6-amino-1,3-dimethyluracil in Table 3. As it is shown in Table 3, indicates that the presented nano magnetite catalyst is superior from the some reported catalyst to catalyze the reaction.
Table 3 Comparison of the results of the reaction of 4-chlorobenzaldehyde, dimedone and 6-amino-1,3-dimethyluracil catalyzed by nano magnetite catalyst with some previously reported catalysts
Catalyst & temperature |
Catalyst amount |
Time (min) |
Yielda (%) |
Ref. |
Isolated yield. Our work. |
P-TSA, PEG : H2O (v/v = 1 : 1), 80 °C |
20 mol% |
240 |
78 |
46 |
Fe3O4@cellulose–SO3H, H2O, 80 °C |
25 mg |
20 |
90 |
19 |
[bmim]Br, H2O, 95 °C |
2 mL |
210 |
90 |
24 |
[TSSECM], 125 °C |
24 mg |
40 |
92 |
18 |
SBA-15/PrN(CH2PO3H2)2, 100 °C |
10 mg |
15 |
85 |
17 |
Nano magnetite catalyst, 60 °C |
20 mg |
20 |
94 |
—b |
3. Conclusions
Nano-[Fe3O4@SiO2/N-propyl-1-(thiophen-2-yl)ethanimine][ZnCl2] as a nano magnetite Schiff base complex was prepared and fully characterized by various analyses and successfully used as a heterogeneous catalyst for the synthesis of pyrimido[4,5-b]quinolones by the reaction of aryl aldehyde, dimedone and 6-amino-1,3-dimethyluracil in EtOH
:
H2O (7
:
3) as a solvent at 60 °C. The generality, high yields, short reaction times, clean reaction condition, simplicity and ease of separation of the catalyst from the reaction mixture are some important advantages of the presented methodology.
4. Experimental
4.1. Materials
All chemicals were purchased from the chemical companies Merck or Fluka. The known products were identified by comparison of their melting points and spectral data with those reported in the literature.
4.2. General procedure for synthesis of Fe3O4
To a mixture of distilled water (25 mL) and hydrochloric acid 37% (0.85 mL) was added FeCl3·6H2O (10 mmol) and FeCl2·4H2O (19.33 mmol) and stirred in an oil bath at 60 °C under nitrogen atmosphere for 30 minutes. This solution was added drop wise to a solution of NaOH (1.5 M, 250 mL) in an oil bath at 60 °C for 2 hours. The prepared mixture was vigorously stirred for 30 minutes. Lastly, the iron oxide nanoparticles were separated with an external magnet and washed twice with distilled water.30
4.3. General procedure for synthesis of Fe3O4@SiO2 core–shell
Fe3O4 nanoparticles (3 g) were dispersed in a mixture of ethanol and water (200
:
10, % v/v) using an ultrasonic bath for 10 minutes. Then 10 mL of ammonia solution (25%) and tetramethylorthosilicate (3 mL) were added dropwise to the mixture and stirred at room temperature for 24 hours. The obtained Fe3O4@SiO2 nanoparticles were separated by an external magnet, washed with methanol and finally dried in an oven.30,31
4.4. Synthesis of (OEt)3Si/N-propyl-1-(thiophen-2-yl)ethanimine
In a round-bottomed flask connected to a reflux condenser (3-aminopropyl)triethoxysilane (1 mmol) and 2-acetyl thiophen (1 mmol) were added and stirred at 90 °C for 24 hours under solvent free condition. Finally, the obtained product as a Schiff base ligand was purified by washing with dry CH2Cl2. The product was identified by FT-IR and 1H NMR.
4.5. Metalation of (OEt)3Si/N-propyl-1-(thiophen-2-yl)ethanimine
In a round-bottomed flask, the prepared ligand (2 mmol) and ZnCl2 (1 mmol) were added and stirred in dry toluene (10 mL) under reflux condition for 24 hours. After the complexation process was completed, the prepared Schiff base complex was filtered off and washed with ethanol.
4.6. Synthesis of nano-[Fe3O4@SiO2/N-propyl-1-(thiophen-2-yl)ethanimine][ZnCl2]
Fe3O4@SiO2 nanoparticles (2 g) were dispersed in toluene (10 mL) for 10 minutes. Then, the prepared Schiff base complex (1 mmol) was added to the mixture and stirred for 24 hours under reflux condition. Finally, nano magnetite Schiff base complex was obtained and separated by an external magnet, washed with ethanol (three times) and dried at 60 °C.
4.7. General procedure for synthesis of pyrimido[4,5-b]quinolones
In a round-bottomed flask which connected to a reflux condenser, a mixture of compounds containing of aromatic aldehydes (1 mmol), 6-amino-1,3-dimethyluracil (1 mmol, 0.155 g), dimedone (1 mmol, 0.140 g) and nano magnetite Schiff base complex (0.02 g) as a catalyst were stirred in ethanol: H2O (7
:
3) (3 mL) as a solvent at 60 °C. After the completion of the reaction as monitored by TLC (n-hexane/ethyl acetate: 1/2), the reaction mixture was extracted by ethanol and the magnetite catalyst was separated by an external magnet and washed with acetone for three times to reuse for the next run. The desired product was purified by the recrystallization in ethanol (70%).
4.8. Selected spectral data
1,3,8,8-Tetramethyl-5-phenyl-7,8,9,10-tetrahydropyrimido[4,5-b]quinoline-2,4,6(1H,3H,5H)-trione (1). IR (KBr, cm−1): 3363, 3203, 2955, 2932, 1700, 1655, 1614, 1507, 1363, 1257; 1H NMR (300 MHz, DMSO-d6): δ 1.06 (s, 3H), 1.15 (s, 3H), 2.31 (s, 2H), 2.41 (s, 2H), 3.12 (s, 3H), 3.38 (s, 3H), 5.5 (s, 1H), 7.12 (d, J = 6.00 Hz, 2H), 7.23 (d, J = 6.00 Hz, 2H), 7.32 (s, 1H), 12.84 (s, 1H); 13C-NMR (DMSO-d6, 300 MHz): δ 27.5, 28.4, 29.3, 30.8, 31.6, 33.4, 44.0, 49.9, 88.3, 114.2, 125.7, 126.8, 128.4, 140.2, 150.6, 155.1, 16.1, 176.5, 199.8.
5-(4-Fluorophenyl)-1,3,8,8-tetramethyl-5,8,9,10-tetrahydropyrimido[4,5-b]quinoline-2,4,6(1H,3H,7H)-trione (2). IR (KBr, cm−1): 3361, 3258, 3217, 2963, 2934, 3895, 1692, 1655, 1593, 1505, 1219, 1157, 761, 507; 1H NMR (300 MHz, DMSO-d6): δ 1.05 (s, 3H), 1.13 (s, 3H), 2.31 (s, 2H), 2.41 (s, 2H), 3.12 (s, 3H), 3.38 (s, 3H), 5.47 (s, 1H), 7.06–7.12 (m, 3H) (7.33 s, 1H), 12.85 (s, 1H) 13C-NMR (DMSO-d6, 300 MHz): δ 27.5, 28.5, 29.3, 30.8, 31.6, 32.9, 44.0, 49.9, 88.2, 114.2, 115.1, 128.5, 136.1, 150.6, 155.1, 159.1, 162.3, 164.1, 176.5, 199.8.
5-(4-Chlorophenyl)-1,3,8,8-tetramethyl-5,8,9,10-tetrahydropyrimido[4,5-b]quinoline-2,4,6(1H,3H,7H)-trione (3). IR (KBr, cm−1): 3405, 3363, 3226, 2961, 2941, 2885, 1696, 1655, 1595, 152, 1509, 1362, 1257, 1014, 925, 784, 500; 1H NMR (300 MHz, DMSO-d6): δ 1.05 (s, 3H), 1.13 (s, 3H), 2.29 (s, 2H), 2.39 (s, 2H), 3.12 (s, 3H), 3.37 (s, 3H), 5.39 (s, 1H), 6.63 (d, J = 6.00 Hz, 2H), 6.88 (d, J = 6.00 Hz, 2H), 7.29 (s, 1H), 12.84 (s, 1H); 13C-NMR (DMSO-d6, 300 MHz): δ 27.5, 28.4, 29.3, 30.7, 31.5, 32.6, 44.0, 50.0, 88.6, 114.5, 115.2, 127.7, 129.9, 150.6, 154.9, 155.3, 164.0, 176.4, 199.8.
5-(2,4-Dichlorophenyl)-1,3,8,8-tetramethyl-5,8,9,10-tetrahydropyrimido[4,5-b]quinoline-2,4,6(1H,3H,7H)-trione (4). IR (KBr, cm−1): 3280, 3221, 3093, 2957, 2931, 2875, 1704, 1661, 1643, 1494, 1379, 1211, 1149, 1046, 962, 845, 755; 1H NMR (300 MHz, DMSO-d6): δ 0.90 (s, 3H), 1.05 (s, 3H), 1.98 (d, J = 15.0 Hz, 1H), 2.21 (d, J = 15.0 Hz, 1H), 2.57 (s, 2H), 3.06 (s, 3H), 3.46 (s, 3H), 5.14 (s, 1H), 7.28–7.35 (m, 3H), 9.05 (s, 1H); 13C-NMR (DMSO-d6, 300 MHz): δ 26.7, 28.0, 29.6, 30.7, 32.4, 34.0, 50.4, 89.6, 110.8, 126.9, 128.7, 131.3, 133.6, 134.0, 143.0, 144.6, 150.5, 150.9, 160.9, 194.8.
5-(4-Nitorophenyl)-1,3,8,8-tetramethyl-5,8,9,10-tetrahydropyrimido[4,5-b]quinoline-2,4,6(1H,3H,7H)-trion (5). IR (KBr, cm−1): 3367, 3337, 3203, 2961, 2911, 1697, 1671, 1592, 1512, 1412, 1344, 1253, 1045, 748, 583; 1H NMR (300 MHz, DMSO-d6): δ 1.06 (s, 3H), 1.14 (s, 3H), 2.33 (s, 2H), 2.43 (2H), 3.12 (s, 3H), 3.38 (s, 3H), 5.60 (s, 1H), 7.35–7.40 (m, 2H), 8.12 (d, J = 9, 2H), 12.77 (s, 1H); 13C-NMR (DMSO-d6, 300 MHz): δ 27.7, 28.5, 29.2, 30.8, 31.6, 34.1, 43.9, 49.9, 87.6, 113.8, 123.6, 128.2, 145.9, 149.5, 150.6, 155.1, 164.1, 176.7, 199.7.
5-(3,4,5-Trimethoxyphenyl)-1,3,8,8-tetramethyl-5,8,9,10-tetrahydropyrimido[4,5-b]quinoline-2,4,6(1H,3H,7H)-trione (7). IR (KBr, cm−1): 3421, 3191, 2997, 2944, 2875, 2839, 1703, 1656, 1600, 15.6, 1467, 1415, 1358, 1257, 1125, 998, 853, 786, 518, 490; 1H NMR (300 MHz, DMSO-d6): δ 1.07 (s, 3H), 1.16 (s, 3H), 2.31 (d, J = 6.0 Hz, 2H), 2.41 (d, J = 15.0 Hz, 2H), 3.15 (s, 3H), 3.65 (s, 3H), 3.67 (s, 9H), 5.45 (s, 1H), 6.39 (s, 1H), 7.30(s, 1H), 12.91 (s, 1H); 13C-NMR (DMSO-d6, 300 MHz): δ 27.0, 28.5, 29.5, 30.8, 31.4, 33.5, 44.0, 49.9, 56.2, 60.4, 88.0, 104.4, 114.5, 135.7, 135.8, 150.6, 152.9, 155.0, 164.2, 176.7, 199.7.
5-(4-Hydroxy-3-methoxyphenyl)-1,3,8,8-tetramethyl-5,8,9,10-tetrahydropyrimido[4,5-b]quinoline-2,4,6(1H,3H,7H)-trione (9). IR (KBr, cm−1): 3426, 3302, 3225, 3080, 2961, 1703, 1661, 1644, 1615, 1379, 1359, 1208, 1126, 1034; 1H NMR (300 MHz, DMSO-d6): δ 0.94 (s, 3H), 1.06 (s, 3H), 2.06 (d, J = 15.0 Hz, 1H), 2.24 (d, J = 18.0 Hz, 1H), 2.58 (d, J = 9.0 Hz, 2H), 3.13 (s, 3H), 3.46 (s, 3H), 3.71 (s, 3H), 4.81 (s, 1H), 6.59–6.6 (s, 2H), 6.81 (s, 1H), 8.67 (s, 1H), 8.99 (s, 1H); 13C-NMR (DMSO-d6, 300 MHz): δ 26.8, 28.1, 29.7, 30.6, 32.5, 33.4, 39.9, 50.5, 55.9, 91.0, 112.4, 112.7, 115.3, 120.17, 138.1, 143.9, 145.2, 147.2, 149.7, 151.0, 161.2, 195.1.
5-(3-Bromophenyl)-1,3,8,8-tetramethyl-5,8,9,10-tetrahydropyrimido[4,5-b]quinoline-2,4,6(1H,3H,7H)-trione (10). IR (KBr, cm−1): 3410, 3163, 2961, 2933, 2872, 1697, 1593, 1507, 1355, 1303, 1244, 1204, 1145, 936, 778; 1H NMR (300 MHz, DMSO-d6): δ 1.06 (s, 3H), 1.13 (s, 3H), 2.31 (s, 2H), 2.42 (s, 2H), 3.13 (s, 3H), 3.37 (s, 3H), 5.51 (s, 1H), 7.13 (d, J = 6.0 Hz, 1H), 7.22 (t, J = 6 Hz, 1H), 7.27 (s, 1H), 7.32 (d, J = 6 Hz, 1H), 12.85 (s, 1H); 13C NMR (300 MHz, DMSO-d6): δ 27.4, 28.5, 29.3, 30.8, 31.6, 33.3, 43.9, 49.9, 87.7, 113.8, 122.0, 126.0, 128.7, 129.6, 130.5, 143.5, 150.6, 155.2, 164.1, 176.6, 199.7.
Conflicts of interest
There are no conflicts to declare.
Acknowledgements
We thank the Bu-Ali Sina University, Hamedan University of Technology for financial support to our research group.
Notes and references
- A. Zare, N. Lotfifar and M. Dianat, J. Mol. Struct., 2020, 1211, 128030 CrossRef CAS.
- A. Drabczynska, C. E. Muller, A. Schiedel, B. Schumacher, J. K. Wojciechowska, A. Fruzinski, W. Zobnina, O. Yuzlenko and K. Kiec-Kononowicz, Bioorg. Med. Chem., 2007, 15, 6956–6974 CrossRef CAS PubMed.
- J. A. Valderrama, P. Colonelli, D. Vasquez, M. F. Gonzalez, J. A. Rodríguez and C. Theoduloz, Bioorg. Med. Chem., 2008, 16, 10172–10181 CrossRef CAS PubMed.
- A. A. Joshi and C. L. Viswanathan, Agents Med. Chem., 2006, 5, 105–122 CAS.
- V. Nair, G. Chi, Q. Shu, J. Julander and D. F. Smee, Bioorg. Med. Chem. Lett., 2009, 19, 1425–1427 CrossRef CAS PubMed.
- S. I. Alqasoumi, A. M. Al-Taweel, A. M. Alafeefy, E. Noaman and M. M. Ghorab, Eur. J. Med. Chem., 2010, 45, 738–744 CrossRef CAS PubMed.
- A. B. A. El-Gazzar, H. N. Hafez and G. A. M. Nawwar, Eur. J. Med. Chem., 2009, 44, 1427–1436 CrossRef CAS PubMed.
- V. Alagarsamy, Pharmazie, 2004, 59, 753–755 CAS.
- V. Alagarsamy, R. Venkatesaperumal, S. Vijayakumar, T. Angayarkanni, P. Pounammal, S. Senthilganesh and S. Kandeeban, Pharmazie, 2002, 57, 306–307 CAS.
- A. R. Moosavi-Zare, Z. Asgari, A. Zare, M. A. Zolfigol and M. Shekouhy, RSC Adv., 2014, 4, 60636 RSC.
- A. R. Moosavi-Zare, M. A. Zolfigol, M. Zarei, A. Zare and V. Khakyzadeh, J. Mol. Liq., 2015, 211, 373 CrossRef CAS.
- Multicomponent Reactions, ed. J. Zhu and H. Bienayme, Wiley, Weinheim, 2005 Search PubMed.
- M. A. Zolfigol, A. Khazaei, A. R. Moosavi-Zare, A. Zare and V. Khakyzadeh, Appl. Catal., A, 2011, 400, 70 CrossRef CAS.
- A. R. Moosavi-Zare and H. Afshar-Hezarkhani, Org. Prep. Proced. Int., 2020, 52, 410–421 CrossRef CAS.
- A. R. Moosavi-Zare, M. A. Zolfigol, F. Derakhshan-Panah and S. Balalaie, Mol. Catal., 2018, 449, 142–151 CrossRef CAS.
- A. R. Moosavi-Zare, H. Goudarziafshar and Z. Jalilian, Appl. Organomet. Chem., 2019, 33, e4584 CrossRef.
- F. Jalili, M. Zarei, M. A. Zolfigol, S. Rostamnia and A. R. Moosavi-Zare, Microporous Mesoporous Mater., 2020, 294, 109865 CrossRef CAS.
- A. Zare, M. Dianat and M. M. Eskandari, New J. Chem., 2020, 44, 4736–4743 RSC.
- F. Osanlou, F. Nemati and S. Sabaqian, Res. Chem. Intermed., 2017, 43, 2159–2174 CrossRef CAS.
- I. M. Moghaddampour, F. Shirini and M. S. N. Langarudi, J. Mol. Struct., 2021, 1226, 129336 CrossRef CAS PubMed.
- O. G. Jolodar, F. Shirini and M. Seddighi, Chin. J. Catal., 2017, 38, 1245–1251 CrossRef CAS.
- A. M. Rad and M. Mokhtary, Int. Nano Lett., 2015, 5, 109–123 CrossRef CAS.
- F. Shirini, M. S. N. Langarudi, N. Daneshvar, N. Jamasbi and M. Irankhah-Khanghah, J. Mol. Struct., 2018, 1161, 366–382 CrossRef CAS.
- D.-Q. Shi, S.-N. Ni, F. Yang, J.-W. Shi, G.-L. Dou, X.-Y. Li, X.-S. Wang and S.-J. Ji, J. Heterocycl. Chem., 2008, 45, 693 CrossRef CAS.
- R. Schlögl, Angew. Chem. Int. Ed., 2015, 54, 3465–3520 CrossRef PubMed.
- J. M. Thomas and W. J. Thomas, Principles and practice of heterogeneous catalysis, 2nd edn, 2014, Weinheim, Germany Search PubMed.
- M. A. Zolfigol, V. Khakyzadeh, A. R. Moosavi-Zare, A. Rostami, A. Zare, N. Iranpoor, M. H. Beyzavi and R. Luque, Green Chem., 2013, 15, 2132–2140 RSC.
- A. Khazaei, A. R. Moosavi-Zare, H. Afshar-Hezarkhani and V. Khakyzadeh, RSC Adv., 2014, 4, 32142–32147 RSC.
- A. Khazaei, F. Gholami, V. Khakyzadeh, A. R. Moosavi-Zare and J. Afsar, RSC Adv., 2015, 5, 14305–14310 RSC.
- M. Bagherzadeh, M. M. Haghdoost and A. Shahbazirad, J. Coord. Chem., 2012, 65, 591–601 CrossRef CAS.
- S. Saedi and J.-W. Rhim, Food Packaging and Shelf Life, 2020, 24, 100473 CrossRef.
- A. R. Sardarian, M. Zangiabadi and I. D. Inaloo, RSC Adv., 2016, 6, 92057–92064 RSC.
- A. R. Sardarian, I. D. Inaloo and M. Zangiabadi, New J. Chem., 2019, 43, 8557–8565 RSC.
- M. T. H. Tarafder, K. B. Chew, K. A. Crouse, A. M. Ali, B. M. Yamin and H. K. Fun, Polyhedron, 2002, 21, 2683–2690 CrossRef CAS.
- H. A. Ali, M. D. Darawsheh and E. Rappocciolo, Polyhedron, 2013, 61, 235–241 CrossRef.
- S. A. da Silva, C. Q. F. Leite, F. R. Pavan, N. Masciocchi and A. Cuin, Polyhedron, 2014, 79, 170–177 CrossRef CAS.
- A. R. Moosavi-Zare, H. Goudarziafshar and L. Ghaffari, Appl. Organomet. Chem., 2017, 31, e3845 CrossRef.
- A. R. Moosavi-Zare, H. Goudarziafshar and Z. Jalilian, Appl. Organomet. Chem., 2019, 33, e4584 CrossRef.
- A. R. Moosavi-Zare, H. Goudarziafshar and K. Saki, Appl. Organomet. Chem., 2018, 32, e3968 CrossRef.
- H. Goudarziafshar, A. R. Moosavi-Zare and J. Hasani, Appl. Organomet. Chem., 2020, 34, e5372 CrossRef CAS.
- A. R. Moosavi-Zare, H. Goudarziafshar and F. Nooraei, Appl. Organomet. Chem., 2020, 34, e5252 CrossRef CAS.
- A. R. Moosavi-Zare, H. Goudarziafshar, M. A. Delkhosh and Z. Jalilian, Org. Prep. Proced. Int., 2021, 53, 402–412 CrossRef CAS.
- A. R. Moosavi-Zare, H. Goudarziafshar and P. Fashi, Res. Chem. Intermed., 2020, 46, 5567–5582 CrossRef CAS.
- M. Jayendran, P. M. S. Begum and M. R. P. Kurup, J. Mol. Struct., 2020, 1206, 127682 CrossRef CAS.
- J. Xu, C. Ju, J. Sheng, F. Wang, Q. Zhang, G. Sun and M. Sun, Bull. Korean Chem. Soc., 2013, 34, 2408–2412 CrossRef CAS.
- G.-p. Lu and C. Cai, J. Heterocycl. Chem., 2014, 51, 1595–1602 CrossRef CAS.
Footnote |
† Electronic supplementary information (ESI) available. See DOI: 10.1039/d2ra00213b |
|
This journal is © The Royal Society of Chemistry 2022 |