DOI:
10.1039/D2RA00078D
(Paper)
RSC Adv., 2022,
12, 5517-5521
Highly efficient synthesis of 3,4-diarylbutadiene sulfones using Heck–Matsuda reaction†
Received
6th January 2022
, Accepted 6th February 2022
First published on 15th February 2022
Abstract
For the first time we describe a general method for the synthesis of previously not synthesized unsymmetrical 3,4-diarylbutadiene sulfones which can be stable convenient precursors for 2,3-diaryl-1,3-butadienes. Our method for arylation of butadiene sulfones via Heck–Matsuda reaction allows to obtain unsymmetrical 3,4-diarylbutadiene sulfones with a variety of alkyl, alkoxy, nitro, ethoxycarbonyl, perfluoroalkyl and halogen substituents (30 examples) in very good yields using readily available reagents and catalysts.
Introduction
Conjugated dienes are one of the most important precursors in organic synthesis that allow to obtain much more complex compounds using fewer synthetic steps.1–7 Also they are well known to be significant scaffolds in a wide range of natural products (such as trichostatin A, fuzanin C, modiolin and azoxymycin C) (Fig. 1).8,9 2-Aryl- and 2,3-diaryl-1,3-butadienes have attracted the interest of organic chemists due to their ability to combine Diels–Alder10,11 and hetero-Diels–Alder cyclization.12,13 This type of conjugated unsaturated compound was also investigated in some other reactions, including hydrohydroxymethylation14 and hydroxymethylation15 catalyzed by iridium and ruthenium complexes respectively. Regarding all these facts the efficient and approachable preparation method for 2,3-diaryl-1,3-butadienes possessing certain regioselectivity is of particular interest both from academic and practical points of view.
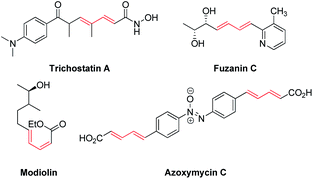 |
| Fig. 1 Bioactive molecules with 1,3-diene motif. | |
Among the possible ways to obtain 1,3-dienes, Wittig reactions,16 reduction of 1,3-diynes or 1,3-enyne,17 transition metal-catalyzed cross-coupling reactions18 and 1,2- or 1,4-elimination of allylic alcohols19 should be named in the first instance. Nevertheless, obtaining unsymmetrical (containing different aryl groups) 2,3-diaryl-1,3-butadienes is much less studied. The preparation of these important compounds can be proceeded using palladium-catalyzed cross-coupling of p-tolyl iodide with 3-silyl-3-phenylbuta-1,3-diene (40% yield)20 or styryl triflates with styryl boronates (31–76% yield).21 Palladium-catalyzed Stille cross-coupling was applied to obtain an unsymmetrical 2,3-bis-(2-nitroaryl)-1,3-butadiene derivative in 61% yield.22 The two different hydrazones were assayed in cross-coupling reaction to give unsymmetrical dienes as well as symmetrical ones causing modest yields of necessary products.23 Also the ene–yne cross-metathesis reaction was reported on one example to make 2,3-diphenyl-1,3-butadiene from ethylene as olefin substrate and internal alkyne.24 Then this method was successfully applied to the variety of alkynes, but it became inefficient in case of substituted unsymmetrical phenylacetylene derivatives since ortho-substitution in aryl ring might cause the low reactivity of alkynes in the reaction25 due to both steric hindrance and potential catalyst inhibition of by the coordination of the ortho-substitutes to the metal center. Obviously, a highly regioselective synthetic methodology for unsymmetrical 2,3-diaryl-1,3-butadienes from simple and readily available starting materials is significantly desirable.
The substituted 3-sulfolenes are well-known key precursors of not only different sulfolanes,26,27 polycyclic heterocycles28 but also conjugated unsaturated compounds.29,30 The thermal extrusion of SO2 (temperatures typically >100 °C) from these stable compounds provides corresponding 1,3-dienes.31
In our previous work we have developed simple and efficient method for preparation of 3-arylbutadiene sulfones via Heck–Matsuda reaction (Scheme 1).32
 |
| Scheme 1 Synthesis of 3-aryl-3-sulfolenes. | |
Concerning our interest in Pd-catalyzed chemistry,33–38 for the first time we disclose an efficient and facile Pd-catalyzed cross-coupling reaction of 3-arylbutadiene sulfones with aryldiazonium salts to construct 3,4-diarylbutadiene sulfones with high regioselectivity. Thus, our proposed method discovered the possibility to explore unsymmetrical diaryl sulfones and their transformations, especially substituted 1,3-dienes synthesis.
Results and discussion
3,4-Diarylbutadiene sulfones synthesis via Heck–Matsuda reaction
To investigate the possibility of 3-arylbutadiene sulfones arylation we have started using the conditions that had already shown the best results in the synthesis of 3-arylbutadiene sulfones32 (1 eq. of aryldiazonium tetrafluoroborate, 3 mol% Pd(OAc)2 in methanol). On the first stage compound 3′ (Scheme 2) is formed due to the arylpalladium syn addition to the C–C double bond from the less hindered face of the double bond anti to the Ph group followed by syn palladium hydride elimination resulting in the migration of the C–C bond39 in the reaction product. The base induced double-bond isomerization in case of 3,4-diarylbutadiene sulfones require much harsher conditions than in case of 3-arylbutadiene sulfones maybe due to the sterical hindrance provided by an aryl group on C-3.40 Refluxing with DBN (1,5-diazabicyclo(4.3.0)non-5-ene) in 1,4-dioxane turned out to be the most suitable system, which leads to formation the only thermodynamically stable 3,4-diarylbutadiene sulfones (Scheme 2).
 |
| Scheme 2 3,4-Diarylbutadiene sulfones synthesis using Heck–Matsuda reaction. | |
However the incomplete conversion and moderate product yields caused us to investigate the optimal aryldiazonium salt and Pd(OAc)2 amounts using the model reaction between 3-phenyl sulfolene and p-nitrophenyldiazonium tetrafluoroborate, that turned out to be 1.5 eq. and 5 mol% Pd(OAc)2 respectively.
After that the impact of aryldiazonium salt structure was studied on the 6 examples of Heck–Matsuda reaction using the 3-phenyl sulfolene as a substrate (Table 1). In all cases the full conversion of 3-arylbutadiene sulfones took place on the cross-coupling stage, including the example with the sterically hindered o-methoxyphenyldiazonium tetrafluoroborate. The migration of double-bond always showed quantitative yield, but required more time in case of methoxy substituted products 3e and 3f (2–4 hours for 3a–d vs. 24 hours for 3e and 3f).
Table 1 Arylation of 3-phenyl-3-sulfolene with different aryldyazonium saltsa
Reaction conditions: step 1: 2a (1 mmol), aryldiazonium salt (1.5 mmol), Pd(OAc)2 (5 mol%), MeOH 5 ml, 2 h; step 2: DBN 0.2 ml, 1.4-dioxane 5 ml, 100 °C, 2–24 h. |
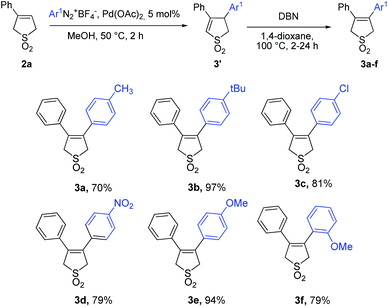 |
To investigate the scope and the limitations of the considered method the influence of the aryl substitution in the starting 3-arylbutadiene sulfones in the Pd-catalyzed Heck–Matsuda reaction was explored (Table 2).
Table 2 Scope of 3,4-diarylbutadiene sulfonesa
Reaction conditions: step 1: 2 (1 mmol), aryldiazonium salt (1.5 mmol), Pd(OAc)2 (5 mol%), MeOH 5 ml, 2 h; step 2: DBN 0.2 ml, 1.4-dioxane 5 ml, 100 °C, 2–24 h. |
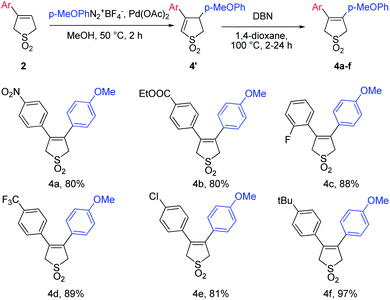 |
Series of 3-arylbutadiene sulfones bearing different electron-donating and electron-withdrawing groups were involved and corresponding 3,4-diarylbutadiene sulfones were obtained in good or excellent yields. To show the versatility of the conditions proposed else 18 examples (Table 3) of unsymmetrical 3,4-diarylbutadiene sulfones bearing different donor, acceptor or neutral substitutions on both aryl rings were obtained.
Table 3 Scope of 3,4-diarylbutadiene sulfonesa
Reaction conditions: step 1: 2 (1 mmol), aryldiazonium salt (1.5 mmol), Pd(OAc)2 (5 mol%), MeOH 5 ml, 2 h; step 2: DBN 0.2 ml, 1.4-dioxane 5 ml, 100 °C, 2–24 h. |
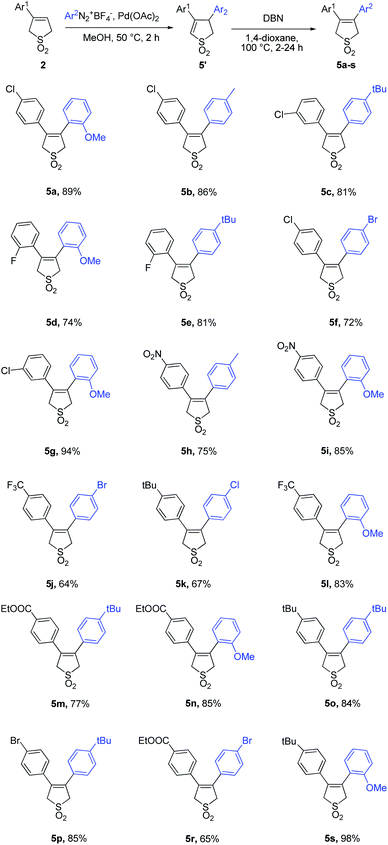 |
As it can be found from the Tables 2 and 3, introduction of the electron-withdrawing substitutions to the aryldiazonuim salt (Table 3, 5f, 5j, 5k and 5r) lead to the slight yield decrease compared with the arylation agents with the electron-donating groups, even in the ortho-position (Table 3, 5a, 5d, 5g, 5i, 5l, 5n). On the contrary in case of the starting 3-arylbutadiene sulfones bearing electron-donating groups (Tables 2 and 3, 5k, 5o, 5s) the moderate yields were observed, while introducing EWG substitutions to sulfones lead to the yield increase. Therefore it can be concluded that the aryldiazonium salt should contain electron-donating substitution whereas the 3-arylbutadiene sulfones should bear the electron-withdrawing one to provide the better product yields.
The 3,4-diaryl butadiene sulfones' reactivity investigation
Conversion of 3,4-diarylbutadiene sulfones to 2,3-diaryl-1,3-butadienes. As it has been already mentioned in the introduction, 3,4-diarylbutadiene sulfones can be easily converted to the corresponding 2,3-diaryl-1,3-butadienes via the SO2 thermal extrusion from the sulfone solution at 100–150 °C.41 Since no one had ever produced unsymmetrical 3,4-diarylbutadiene sulfones before us, we felt it necessary to try them in the classical sulfone chemistry transformations. Some of the unsymmetrical 3,4-diarylbutadiene sulfones obtained in the first part of this work underwent this transformation in the 1,2-dichlorobenzene solution at 140 °C resulting with the stable unsymmetrical 2,3-diaryl-1,3-butadienes with the high yields (Scheme 3, 8a–8c). These 3 examples of 2,3-diaryl-1,3-butadienes have the different electron-donating and electron-withdrawing substituents in the phenyl rings and were synthesized for the first time. It is also worth mentioning that the whole scope of obtained 3,4-diarylbutadiene sulfones (Tables 2 and 3) may be considered as the precursors for the corresponding 2,3-diaryl-1,3-butadienes, and in most cases (except for Table 3, 5o42,43) after SO2 thermal extrusion previously unavailable 2,3-diaryl-1,3-butadienes may be originally synthesized.
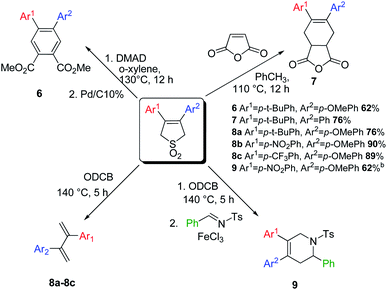 |
| Scheme 3 Transformation of 3,4-diaryl-3-sulfolenes to different products. | |
[4 + 2] cycloaddition reactions. Cyclization reactions are of significant value in the synthesis of complex structures.23 3,4-Diarylbutadiene sulfones are shown to be the convenient and stable substrates in place of 1,3-dienes for cycloaddition reactions, most significantly the [4 + 2] Diels–Alder reaction, in various synthetic routes such as the synthesis of natural products,44,45 porphyrins46,47 and functionalized fullerenes.48,49Having explored the scope of 3,4-diarylbutadiene sulfones we have carried out a number of them with some well-known dienophiles via Diels–Alder reaction. Thus the cycloadduct between maleic anhydride and 3-(p-tert-butylphenyl)-4-phenyl-butadiene sulfone (3b) was obtained in good yield (76%) (Scheme 3, 7).
The reaction of 3-(p-OMe-phenyl)-4-(p-tert-butylphenyl)-butadiene sulfones with dimethyl acetylenedicarboxylate results to the mixture of the cycloadduct and the product of its aromatization. The comprehensive aromatization of the mixture can be catalyzed by treatment with DDQ or palladium on activated charcoal (Scheme 3, 6).
We have also shown that unsymmetrical dienes can react regioselectively with asymmetric dienophiles via Diels–Alder reaction. N-Tosyl tetrahydropyridine 9 was obtained as the single regioisomer in good yield (Scheme 3).50
Thus, the unsymmetrical diaryl sulfones showed good reactivity under Diels–Alder reaction conditions, which significantly expands the potential scope of polyaromatic compounds which can be used in liquid crystals, microelectronics, etc.51–53
Conclusions
In summary, the facile, mild and highly regioselective synthesis of previously undescribed unsymmetrical 3,4-diarylbutadiene sulfones bearing donor, acceptor and sterical hindered substituents was developed. It was shown that this approach is applicable for obtaining different previously unavailable unsymmetrical 2,3-diaryl-1,3-butadienes in very good yield. For the first time the reactivity of 3,4-diarylbutadiene sulfones under Diels–Alder reactions conditions has been demonstrated, which makes it possible to explore new, more complicated polyaromatic compounds.
Conflicts of interest
There are no conflicts to declare.
Acknowledgements
The study was supported by the Russian Science Foundation (project number 19-73-10185). Part of this work was carried out by Andrey F. Asachenko and Sergey A. Rzhevskiy, as part of the A. V. Topchiev Institute of Petrochemical Synthesis (TIPS) Russian Academy of Sciences (RAS) State Program. This work was performed using the equipment of the Shared Research Center ‟Analytical center of deep oil processing and petrochemistry of TIPS RAS”.
Notes and references
- F. Erver, J. R. Kuttner and G. Hilt, J. Org. Chem., 2012, 77, 8375–8385 CrossRef CAS PubMed.
- J.-A. Funel and S. Abele, Angew. Chem., Int. Ed., 2013, 52, 3822–3863 CrossRef CAS PubMed.
- M. Hirano, T. Okamoto, N. Komine and S. Komiya, Organometallics, 2012, 31, 4639–4642 CrossRef CAS.
- S. Ogoshi, K.-i. Tonomori, M.-a. Oka and H. Kurosawa, J. Am. Chem. Soc., 2006, 128, 7077–7086 CrossRef CAS PubMed.
- Y. Wen, L. Huang and H. Jiang, J. Org. Chem., 2012, 77, 5418–5422 CrossRef CAS PubMed.
- M. A. Belaya, D. A. Knyazev, D. D. Borisov, R. A. Novikov and Y. V. Tomilov, J. Org. Chem., 2021, 86, 8089–8100 CrossRef CAS PubMed.
- M. A. Belaya, D. A. Knyazev, R. A. Novikov and Y. V. Tomilov, Tetrahedron Lett., 2020, 61, 151990 CrossRef CAS.
- K. Trisuwan, V. Rukachaisirikul, Y. Sukpondma, S. Preedanon, S. Phongpaichit, N. Rungjindamai and J. Sakayaroj, J. Nat. Prod., 2008, 71, 1323–1326 CrossRef CAS PubMed.
- M. DellaGreca, C. Di Marino, A. Zarrelli and B. D'Abrosca, J. Nat. Prod., 2004, 67, 1492–1495 CrossRef CAS PubMed.
- S. Kotha, M. Meshram and A. Tiwari, Chem. Soc. Rev., 2009, 38, 2065–2092 RSC.
- S. Kotha and V. Seema, Synlett, 2011, 2011, 2329–2334 CrossRef.
- R. B. Lewis and J. Read de Alaniz, Tetrahedron, 2017, 73, 4045–4051 CrossRef CAS.
- H. Srour, K. Abidi, Z. Sahli, S. Basker, N. Hamdi, M. Achard and C. Bruneau, ChemCatChem, 2011, 3, 1876–1879 CrossRef CAS.
- K. D. Nguyen, D. Herkommer and M. J. Krische, J. Am. Chem. Soc., 2016, 138, 14210–14213 CrossRef CAS PubMed.
- T. Smejkal, H. Han, B. Breit and M. J. Krische, J. Am. Chem. Soc., 2009, 131, 10366–10367 CrossRef CAS PubMed.
- B. E. Maryanoff and A. B. Reitz, Chem. Rev., 1989, 89, 863–927 CrossRef CAS.
- K. N. Campbell and L. T. Eby, J. Am. Chem. Soc., 1941, 63, 216–219 CrossRef CAS.
- K. C. Nicolaou, P. G. Bulger and D. Sarlah, Angew. Chem., Int. Ed. Engl., 2005, 44, 4442–4489 CrossRef CAS PubMed.
- T. Kitahara, T. Matsuoka, H. Kiyota, Y. Warita, H. Kurata, A. Horiguchi and K. Mori, Synthesis, 1994, 1994, 692–694 CrossRef.
- Z. Ikeda, K. Oshima and S. Matsubara, Org. Lett., 2005, 7, 4859–4861 CrossRef CAS PubMed.
- D. Eom, S. Park, Y. Park, T. Ryu and P. H. Lee, Org. Lett., 2012, 14, 5392–5395 CrossRef CAS PubMed.
- N. H. Ansari, C. A. Dacko, N. G. Akhmedov and B. C. G. Söderberg, J. Org. Chem., 2016, 81, 9337–9349 CrossRef CAS PubMed.
- H. Jiang, L. He, X. Li, H. Chen, W. Wu and W. Fu, Chem. Commun., 2013, 49, 9218–9220 RSC.
- G. Hilt and S. Roesner, Synthesis, 2011, 2011, 662–668 CrossRef.
- M. K. Abderrezak, Z. Kabouche, C. Bruneau and C. Fischmeister, Catalysts, 2017, 7, 365 CrossRef.
- K. Meng, J. Xia, Y. Wang, X. Zhang, G. Yang and W. Zhang, Org. Chem. Front., 2017, 4, 1601–1605 RSC.
- Y. Kita, S. Hida, K. Higashihara, H. S. Jena, K. Higashida and K. Mashima, Angew. Chem., Int. Ed., 2016, 55, 8299–8303 CrossRef CAS PubMed.
- K. Ando and H. Takayama, Heterocycles, 1994, 37, 1417–1439 CrossRef CAS.
- R. Bloch, C. Benecou and E. Guibé-Jampel, Tetrahedron Lett., 1985, 26, 1301–1304 CrossRef CAS.
- R. Bloch, D. Hassan and X. Mandard, Tetrahedron Lett., 1983, 24, 4691–4694 CrossRef CAS.
- M. G. Brant and J. E. Wulff, Synthesis, 2016, 48, 1–17 CAS.
- S. A. Rzhevskiy, V. N. Bogachev, L. I. Minaeva, G. K. Sterligov, M. S. Nechaev, M. A. Topchiy and A. F. Asachenko, Mendeleev Commun., 2021, 31, 548–549 CrossRef CAS.
- E. V. Bermesheva, A. I. Wozniak, F. A. Andreyanov, G. O. Karpov, M. S. Nechaev, A. F. Asachenko, M. A. Topchiy, E. K. Melnikova, Y. V. Nelyubina, P. S. Gribanov and M. V. Bermeshev, ACS Catal., 2020, 10, 1663–1678 CrossRef CAS.
- A. I. Wozniak, E. V. Bermesheva, N. N. Gavrilova, I. R. Ilyasov, M. S. Nechaev, A. F. Asachenko, M. A. Topchiy, P. S. Gribanov and M. V. Bermeshev, Macromol. Chem. Phys., 2018, 219, 1800323 CrossRef.
- A. A. Ageshina, G. K. Sterligov, S. A. Rzhevskiy, M. A. Topchiy, G. A. Chesnokov, P. S. Gribanov, E. K. Melnikova, M. S. Nechaev, A. F. Asachenko and M. V. Bermeshev, Dalton Trans., 2019, 48, 3447–3452 RSC.
- G. A. Chesnokov, A. A. Ageshina, A. V. Maryanova, S. A. Rzhevskiy, P. S. Gribanov, M. A. Topchiy, M. S. Nechaev and A. F. Asachenko, Russ. Chem. Bull., 2020, 69, 2370–2377 CrossRef CAS.
- S. A. Rzhevskiy, M. A. Topchiy, V. N. Bogachev, L. I. Minaeva, I. R. Cherkashchenko, K. V. Lavrov, G. K. Sterligov, M. S. Nechaev and A. F. Asachenko, Mendeleev Commun., 2021, 31, 409–411 CrossRef CAS.
- S. A. Rzhevskiy, M. A. Topchiy, V. N. Bogachev, A. A. Ageshina, L. I. Minaeva, G. K. Sterligov, M. S. Nechaev and A. F. Asachenko, Mendeleev Commun., 2021, 31, 478–480 CrossRef CAS.
- R. C. Larock, E. K. Yum and H. Yang, Tetrahedron, 1994, 50, 305–321 CrossRef CAS.
- T. S. Chou, S. C. Hung and H. H. Tso, J. Org. Chem., 1987, 52, 3394–3399 CrossRef CAS.
- C.-W. Ko and T.-s. Chou, J. Org. Chem., 1998, 63, 4645–4653 CrossRef CAS.
- Y. Zhang, J. Li, X. Li and J. He, Macromolecules, 2014, 47, 6260–6269 CrossRef CAS.
- X. Zhao, S. Zhu, F.-L. Qing and L. Chu, Chem. Commun., 2021, 57, 9414–9417 RSC.
- T.-s. Chou, S.-J. Lee and N.-K. Yao, Tetrahedron, 1989, 45, 4113–4124 CrossRef CAS.
- J. Leonard, S. P. Fearnley, M. R. Finlay, J. A. Knight and G. Wong, J. Chem. Soc., Perkin Trans. 1, 1994, 17, 2359–2361 RSC.
- O. Finikova, A. Cheprakov, I. Beletskaya and S. Vinogradov, Chem. Commun., 2001, 3, 261–262 RSC.
- M. G. H. Vicente, A. C. Tomé, A. Walter and J. S. Cavaleiro, Tetrahedron Lett., 1997, 38, 3639–3642 CrossRef CAS.
- H. Ishida, H. Asaji, T. Hida, K. Itoh and M. Ohno, Tetrahedron Lett., 2000, 41, 2153–2156 CrossRef CAS.
- H. Ishida, K. Itoh, S. Ito, N. Ono and M. Ohno, Synlett, 2001, 2001, 0296–0298 CrossRef.
- R. Tomifuji, K. Maeda, T. Takahashi, T. Kurahashi and S. Matsubara, Org. Lett., 2018, 20, 7474–7477 CrossRef CAS PubMed.
- H. Bock, M. Rajaoarivelo, S. Clavaguera and É. Grelet, Eur. J. Org. Chem., 2006, 2006, 2889–2893 CrossRef.
- T. Osawa, T. Kajitani, D. Hashizume, H. Ohsumi, S. Sasaki, M. Takata, Y. Koizumi, A. Saeki, S. Seki, T. Fukushima and T. Aida, Angew. Chem., Int. Ed., 2012, 51, 7990–7993 CrossRef CAS PubMed.
- J. Yin, H. Qu, K. Zhang, J. Luo, X. Zhang, C. Chi and J. Wu, Org. Lett., 2009, 11, 3028–3031 CrossRef CAS PubMed.
Footnote |
† Electronic supplementary information (ESI) available: Detailed experimental procedures, 1H and 13C NMR, IR, HRMS data. See DOI: 10.1039/d2ra00078d |
|
This journal is © The Royal Society of Chemistry 2022 |
Click here to see how this site uses Cookies. View our privacy policy here.