DOI:
10.1039/D1RA09284G
(Paper)
RSC Adv., 2022,
12, 6459-6462
Structure and absolute configuration of liquid molecules based on adamantane derivative cocrystallization†
Received
23rd December 2021
, Accepted 31st January 2022
First published on 23rd February 2022
Abstract
Liquid molecules are difficult to crystallize, and their structures and absolute configurations cannot be directly determined by X-ray crystallography. We herein report the rapid cocrystallization of tetraaryladamantanes with liquid molecules. The structure of the liquid small molecules can be obtained by determining the crystal structure of the cocrystallized compound. The absolute configuration of chiral molecules can also be assigned, which cannot be accomplished by other methods such as nuclear magnetic resonance. In this paper, liquid compounds such as phenylethanol and phenylpropanol derivatives were selected. 1,3,5,7-Tetrakis(2,4-diethoxyphenyl)adamantane (TEO) powder was heated and dissolved in liquid molecules and allowed to stand overnight to undergo cocrystallization. The results show that the single-crystal structures and the absolute configurations of 16 liquid molecules were determined by cocrystallization, and the homochiral natures of chiral compounds were confirmed by solid circular dichroism spectral measurements.
Introduction
When a new compound is synthesized or isolated, it is usually characterized by nuclear magnetic resonance spectroscopy, mass spectrometry, and infrared spectroscopy, which provide essential information regarding its molecular formula, functional groups, and bond connectivities. However, the absolute stereochemistry of enantiomers was not obtained by the above methods. The most powerful method to determine molecular information is X-ray single-crystal diffraction. However, X-ray crystallography requires a crystal for analysis, and many small or highly flexible molecules, such as liquids or amorphous solids, do not crystallize readily. Chemists are searching for new methods to obtain accurate information on molecular structures. A particularly significant “crystalline sponge method” for the structural determination of noncrystalline compounds by X-ray single-crystal diffraction was reported by Fujita and coworkers.1–7 The crystal sponge most frequently employed in their studies was the metal–organic framework {[(ZnI2)3(tris(4-pyridyl)-1,3,5-triazene)2]x(solvent)}n. However, the crystal sponges reported thus far are based on metal–organic frameworks, and their limitations are as follows: first, when the size of the target molecule is larger than one of the pores, the target molecule does not enter the crystal sponge. Second, it is difficult for hydrophilic molecules to enter the hydrophobic pores, and the “crystal sponge method” has been mainly applied to the determination of hydrophobic molecular structures. Several methods are being pursued to address these limitations. Recently, another particularly significant method using “crystallization chaperones” was presented. These crystallization chaperones, including tetraaryladamantanes8–19 and trimesic acid,20–27 readily form crystalline inclusion complexes with a range of guest molecules and provide accurate structural information on difficult-to-crystallize small molecules. Meanwhile, the high-throughput nanoscale crystallization of organic-soluble small molecules (encapsulated nanodroplet crystallization) was reported.28 However, the cocrystallization of universal chaperones with various small molecules, such as porphyrins, calixarenes, or cyclodextrins, with a proven ability to obtain the structure of a broad range of different organic molecules is yet to be reported.12 Here, we report the rapid cocrystallization of 1,3,5,7-tetrakis(2,4-diethoxyphenyl)adamantane (TEO) with liquid small molecules (Scheme 1), such as phenylethanol and phenylpropanol derivatives, and determine the absolute configuration of chiral small molecules.
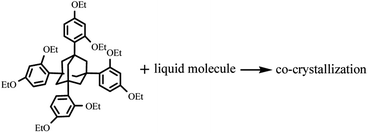 |
| Scheme 1 Cocrystallization of TEO with liquid small molecules. | |
Experimental section
Materials and physical measurements
1,3,5,7-Tetrakis(2,4-diethoxyphenyl)adamantane (TEO) was customized by WuXi AppTec (Tianjin, China). All of the other chemicals are commercially available and used without further purification. The solid circular dichroism (CD) spectra were recorded in the 200–350 nm region using a JASCO J-815 spectropolarimeter.
General methods for cocrystallization
Approximately 2 mg of solid TEO (0.0025 mmol) in a cylindrical sample bottle (1 mL) was added to approximately 40 mg of liquid analyte (2 drops). The resulting suspension was heated on a hot plate until a clear solution formed. The resulting solution was allowed to cool to room temperature with the sample bottle on the plate. After 12 h, colorless prism- or block-shaped crystals were obtained and then analysed by X-ray single-crystal diffraction.
X-ray crystallography
Single-crystal data were collected on a Bruker Apex II diffractometer at 296 K using Mo-Kα radiation (λ = 0.71073 Å) or on a SuperNova Dual AtlasS2 diffractometer at 150 K using Cu-Kα radiation (λ = 1.54184 Å). All empirical absorption corrections were applied by the SADABS program29 or CrysAlisPro (Rigaku, V1.171.39.46, 2018). The structures were solved by direct methods, which yielded the positions of all nonhydrogen atoms. These were refined first isotropically and then anisotropically. All nonhydrogen atoms were refined anisotropically. Hydrogen atom positions were calculated geometrically and refined using the riding model. All calculations were performed using the SHELXTL system of computer programs.30 Most of the guest molecules are disordered in cocrystallization. The crystallographic data are summarized in Table S1.† CCDC reference numbers 2126622 to 2126637 for 1–16, respectively. See http://www.rsc.org/suppdata/ for crystallographic data in CIF or other electronic format.
Results and discussion
We chose structurally similar liquid analytes, such as phenylethanol and phenylpropanol derivatives, for cocrystallization experiments with TEO. A mixture of the analytes and TEO without any solvent was heated for approximately 30 s on a laboratory hotplate until a clear solution formed. The resulting solution was left to cool to room temperature. Colourless crystals for X-ray diffraction were obtained after crystallization overnight, and the crystal structures of the liquid analytes were confirmed by X-ray crystallography. With most guest molecules, triclinic crystal systems and a host/guest ratio of 1
:
1 were found. However, with 1-(4-chlorophenyl)ethanol and 2-(4-methylphenyl)-1-propanol, TEO crystallized in a trigonal crystal system (Table S1†).
Successful examples can be found from the full list of 16 liquid small molecules tested in this study (Table 1), including a series of phenylethanol derivatives (1a–1e) (Fig. 1), phenylpropanol derivatives (2a–2d) (Fig. 2), ethylbenzylamine (3a–3c) (Fig. 3), isomers such as 2-chloropyridine (4a) and 3-chloropyridine (4b), styralyl acetate (5) used in fragrance and flavor, and methacrylic acid (6) (Fig. 4) applied to organic chemical raw materials and intermediates of polymers (Fig. 5). There are no covalent bonds or strong mutual interactions, such as hydrogen bonding between host and guest molecules in the asymmetric unit of the complex (Fig. 6). The liquid small molecules solidified and crystallized with the aid of TEO, and most of the liquid molecules were disordered due to the lack of mutual interactions with the host during cocrystallization. TEO can rapidly crystallize, perhaps due to its shape and symmetry, in which alkoxy groups can adopt different conformations to accommodate different guest molecules.14 However, a crystalline sponge needs to be preformed with a suitable crystal lattice for accommodating analytes. TEO solvated by liquid molecules can crystallize without any special interactions and encapsulate a broad range of molecules and would be a potential candidate for extended crystalline sponges.
Table 1 Cocrystallization experiments of TEO and analytes
Serial No. |
Analytes |
Crystal system |
R1 |
Flack values |
Sample No. |
1 |
Phenylethanol |
Triclinic |
0.064 |
|
1a |
2 |
1-(3-fluorophenyl)ethanol |
Triclinic |
0.060 |
|
1b |
3 |
1-(4-chlorophenyl)ethanol |
Trigonal |
0.081 |
|
1c |
4 |
2-Phenylethanol |
Triclinic |
0.074 |
|
1d |
5 |
(R)-(−)-2-chloro-1-phenylethanol |
Triclinic |
0.096 |
0.124(14) |
1e |
6 |
1-Phenyl-1-propanol |
Triclinic |
0.066 |
|
2a |
7 |
(S)-(−)-1-phenyl-1-propanol |
Triclinic |
0.045 |
−0.01(4) |
2b |
8 |
(R)-(+)-1-phenyl-1-propanol |
Triclinic |
0.041 |
0.04(3) |
2c |
9 |
2-(4-methylphenyl)-1-propanol |
Trigonal |
0.071 |
|
2d |
10 |
α-ethylbenzylamine |
Triclinic |
0.076 |
|
3a |
11 |
(R)-(+)-α-ethylbenzylamine |
Triclinic |
0.048 |
0.06(5) |
3b |
12 |
(S)-(−)-α-ethylbenzylamine |
Triclinic |
0.067 |
0.03(8) |
3c |
13 |
2-Chloropyridine |
Triclinic |
0.062 |
|
4a |
14 |
3-Chloropyridine |
Triclinic |
0.066 |
|
4b |
15 |
Styralyl acetate |
Triclinic |
0.065 |
|
5 |
16 |
Methacrylic acid |
Triclinic |
0.097 |
|
6 |
 |
| Fig. 1 X-ray crystal structures of liquid phenylethanol derivatives obtained by cocrystallization with TEO (ORTEP plots of the encapsulated molecules at the 50% probability level). | |
 |
| Fig. 2 X-ray crystal structures of liquid phenylpropanol derivatives obtained by cocrystallization with TEO (ORTEP plots of the encapsulated molecules at the 50% probability level). | |
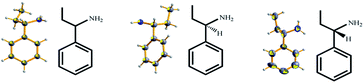 |
| Fig. 3 X-ray crystal structures of liquid α-ethylbenzylamine obtained by cocrystallization with TEO (ORTEP plots of the encapsulated molecules at the 50% probability level). | |
 |
| Fig. 4 X-ray crystal structures of other liquid analytes obtained by cocrystallization with TEO (ORTEP plots of the encapsulated molecules at the 50% probability level). | |
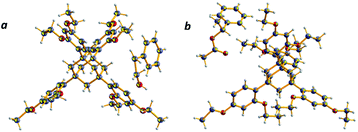 |
| Fig. 5 Structure of representative cocrystals and 50% probability displacement ellipsoids for non-H atoms: (a) 2-phenylethanol/TEO and (b) styralyl acetate/TEO. | |
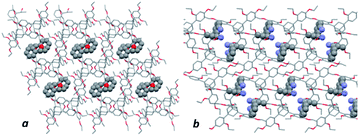 |
| Fig. 6 Packing arrangement in representative cocrystals: (a) 1-phenyl-1-propanol/TEO and (b) α-ethylbenzylamine/TEO. | |
Structurally similar liquid phenyl alcohols or amines were selected, including two pairs of enantiomers, namely, (R)- and (S)-1-phenyl-1-propanol (2b and 2c) and (R)- and (S)-α-ethylbenzylamine (3b and 3c). Flack parameters were in the range of −0.01(4) to 0.124(14) (Table 1), allowing for the unambiguous assignment of absolute configuration. The absolute structure parameters are critical for determining the correct absolute configuration of the chiral guest molecule. The initial structural model was solved using SHELXTL programs, and the intensity data appeared to be centrosymmetric due to the strong reflexes originating from host molecules, although the encapsulated guest molecule was chiral. To resolve this conflict, it is necessary to introduce particular treatments according to previous reports.18 Compared to the above reports, the homochiral natures of chiral compounds were further confirmed by solid CD spectrum.
Circular dichroism spectra
The crystal samples were filtered, dried, and determined after grinding and pressing on a JASCO J-815 spectropolarimeter. The results of the selected solid circular dichroism measurements are consistent with the results of the X-ray crystal structures. As shown in Fig. 7a, the bulk crystals of 3b showed negative and positive Cotton effects at 207 and 273 nm, respectively, and those of 3c also showed positive (208 and 231 nm) and negative Cotton effects (296 nm). The selected corresponding UV spectra of 3b in Fig. S1.†
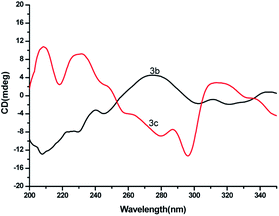 |
| Fig. 7 Solid CD spectra of TEO-S-α-ethylbenzylamine (3b) and TEO-R-α-ethylbenzylamine (3c). | |
Conclusions
In summary, we report a rapid method for the structure determination of liquid small molecules. These liquid small molecules can cocrystallize rapidly with 1,3,5,7-tetrakis(2,4-diethoxyphenyl)adamantane without covalent bonds or strong directional interactions and can be used to determine the absolute configuration of chiral liquid molecules. Unfortunately, most liquid molecules are disordered during cocrystallization, increasing the difficulty of structural analysis. 1,3,5,7-Tetrakis(2,4-diethoxyphenyl)adamantane can cocrystallize rapidly with liquid molecules and encapsulate a broad range of molecules, demonstrating its potential application in extended crystalline sponges.
Conflicts of interest
There are no conflicts to declare.
Acknowledgements
This work is supported by the National Natural Science Foundation of China (51772091), the Scientific Research Fund of Hunan Provincial Education Department (20A210), and the Construct Program of Applied Characteristic Discipline in Hunan University of Science and Engineering.
Notes and references
- Y. Inokuma, S. Yoshioka, J. Ariyoshi, T. Arai, Y. Hitora, K. Takada, S. Matsunaga, K. Rissanen and M. Fujita, Nature, 2013, 495, 461 CrossRef CAS PubMed.
- T. Kawamichi, T. Haneda, M. Kawano and M. Fujita, Nature, 2009, 461, 633 CrossRef CAS PubMed.
- Y. Inokuma, S. Yoshioka, J. Ariyoshi, T. Arai and M. Fujita, Nat. Protoc., 2014, 9, 246 CrossRef CAS PubMed.
- S. Yoshioka, Y. Inokuma, M. Hoshino, T. Sato and M. Fujita, Chem. Sci., 2015, 6, 3765 RSC.
- N. Zigon, M. Hoshino, S. Yoshioka, Y. Inokuma and M. Fujita, Angew. Chem., Int. Ed., 2015, 54, 9033 CrossRef CAS PubMed.
- Y. Inokuma, M. Kawano and M. Fujita, Nat. Chem., 2011, 3, 349 CrossRef CAS PubMed.
- G. A. O'Brien, A. Maruyama, Y. Inokuma, M. Fujita, P. S. Baran and D. G. Blackmond, Angew. Chem., Int. Ed., 2014, 53, 11868 CrossRef PubMed.
- J. L. Johnson, K. C. Entzminger, J. Hyun, S. Kalyoncu, D. P. Heaner Jr, A. Morales, A. Sheppard, J. C. Gumbart, J. A. Maynard and R. L. Lieberman, Acta Crystallogr., Sect. D: Biol. Crystallogr., 2015, 71, 896 CrossRef CAS PubMed.
- M. B. Bhatt and G. R. Desiraju, CrystEngComm, 2008, 10, 1747 RSC.
- M. P. Bryn, C. J. Curtis, Y. Hsiou, S. I. Khan, P. A. Sawin, S. K. Kendrick, A. Terzis and C. E. Strouse, J. Am. Chem. Soc., 1993, 115, 9480 CrossRef.
- T. Gruber, C. Fischer, W. Seichter, P. Bombicz and E. Weber, CrystEngComm, 2011, 13, 1422 RSC.
- M. Ceborska, Chem. Phys. Lett., 2016, 651, 192 CrossRef CAS.
- Y. Li, S. Tang, A. Yusov, J. Rose, A. N. Borrfors, C. T. Hu and M. D. Ward, Nat. Commun., 2019, 10, 4477 CrossRef PubMed.
- A. Schwenger, W. Frey and C. Richert, Chem.–Eur. J., 2015, 21, 8781 CrossRef CAS PubMed.
- P. E. Alexandre, A. Schwenger, W. Frey and C. Richert, Chem.–Eur. J., 2017, 23, 9018 CrossRef CAS PubMed.
- A. Schwenger, W. Frey and C. Richert, Angew. Chem., Int. Ed., 2016, 55, 13706 CrossRef CAS PubMed.
- F. Krupp, S. He, W. Frey and C. Richert, Synlett, 2018, 29, 1707 CrossRef CAS.
- F. Krupp, W. Frey and C. Richert, Angew. Chem., Int. Ed., 2020, 59, 15875 CrossRef CAS PubMed.
- F. Rami, J. Nowak, F. Krupp, W. Frey and C. Richert, Beilstein J. Org. Chem., 2021, 17, 1476–1480 CrossRef CAS PubMed.
- S. V. Kolotuchin, E. E. Fenlon, R. W. Wilson, C. J. Loweth and S. C. Zimmerman, Angew. Chem., Int. Ed. Engl., 1995, 34, 2654 CrossRef CAS.
- S. V. Kolotuchin, E. E. Fenlon, R. W. Wilson, C. J. Loweth and S. C. Zimmerman, Angew. Chem., Int. Ed. Engl., 1995, 34, 2654 CrossRef CAS.
- Y. C. Yan, B. M. Kariuki, C. E. Hughes, A. J. Logsdail and K. D. M. Harris, Cryst. Growth Des., 2020, 20, 5736 CrossRef CAS.
- A. Ibenskas, M. Simenas and E. E. Tornau, J. Phys. Chem. C, 2016, 120, 6669 CrossRef CAS.
- M. R. Goldyn, D. Larowska and E. Bartoszak-Adamska, Cryst. Growth Des., 2021, 21, 396 CrossRef CAS.
- L. Rajput, N. Jana and K. Biradha, Cryst. Growth Des., 2010, 10, 4565 CrossRef CAS.
- S. Bhattacharya and B. K. Saha, Cryst. Growth Des., 2011, 11, 2194 CrossRef CAS.
- G. C. Ou, Q. Wang, Q. Zhou and X. F. Wang, Crystals, 2021, 11, 409 CrossRef CAS.
- A. R. Tyler, R. Ragbirsingh, C. J. McMonagle, P. G. Waddell, S. E. Heaps, J. W. Steed, P. Thaw, M. J. Hall and M. R. Probert, Chem, 2020, 6, 1755 CAS.
- G. M. Sheldrick, SHELXL-2014/7: program for the solution of crystal structures, University of Göttingen, Göttingen, Germany, 2014 Search PubMed.
- G. M. Sheldrick, Acta Crystallogr., Sect. C: Struct. Chem., 2015, 71, 3 Search PubMed.
Footnote |
† Electronic supplementary information (ESI) available. CCDC 2126622–2126637 for 1–16. For ESI and crystallographic data in CIF or other electronic format see DOI: 10.1039/d1ra09284g |
|
This journal is © The Royal Society of Chemistry 2022 |