DOI:
10.1039/D1RA09136K
(Paper)
RSC Adv., 2022,
12, 9815-9820
Palladium-catalyzed hydroboration reaction of unactivated alkynes with bis (pinacolato) diboron in water†
Received
17th December 2021
, Accepted 14th March 2022
First published on 29th March 2022
Abstract
A highly efficient and mild palladium-catalyzed hydroboration of unactivated internal alkynes in water is described. Both aryl- and alkyl-substituted alkynes proceeded smoothly within the reaction time to afford the desired vinylboronates in moderate to high yields. Bis (pinacolato) diboron was used to afford α- and β-hydroborated products in the presence of HOAc. These reactions showed high reactivities and tolerance, thus providing a promising method for the synthesis of alkenyl boron compounds.
Introduction
In the past few decades, organoboron compounds have received extensive attention due to their diverse biological activities and important synthetic intermediates in the fields of organic synthesis, materials science and drug development, especially as important synthetic intermediates in organic synthesis, the versatility shown by the structure is very eye-catching.1,2 Organoboron compounds could be used as essential carbon nucleophiles for the introduction of functional groups and the C–C bond formation process such as Suzuki–Miyaura coupling and Petasis reaction. The current research provides numerous feasible and effective new methods for preparing organoboron compounds, such as asymmetric catalytic reaction to prepare a chiral borane. In this circumstance, vinyl boronates, which are versatile organic synthetic intermediates, are now gaining increasing attention and have been widely used in various carbon–carbon bond forming reactions.3 Compared with the previous methods that used organic halides (I, Br, Cl) as reagents, our method of using organic boron reagents is more effective for the formation of C–C bonds and direct hydroboration reactions. High-yield products can be obtained by the method. The method we developed is very compatible with various functional groups.4 The organoboron reagent can be used as a necessary carbon nucleophile in the reaction of introducing functional groups and the formation of C–C bonds (such as Suzuki–Miyaura coupling and Petasis reaction); it is an excellent borohydride reagent.5 It is very important to develop synthetic methods for organoboron compounds because these compounds can be directly used in various transformations, such as the construction of C–C bonds and C–B bonds.6
Considerable attention was focused on alkenylboron compounds, whose versatility has been demonstrated by the stereodefined construction of valuable multi-substituted alkenes including biologically active molecules, natural products, and functional materials.7 Cobalt,8 ruthenium,9 aluminum,10 copper,11 iron,12 manganese,13 and ytterbium14 were used in the hydroboration reaction of alkenes recently. For example, oxazolinyl phenyl picolinic acid amide as a ligand, styrene was subjected to a highly Markovnikov selective hydroboration reaction under iron catalysis to provide the branched borohydride product (Scheme 1, eqn (1)),15a and Jiang et al. have successfully developed the regioselective palladium(II)-catalyzed hydroboration of aryl alkenes with B2pin2.15b Alkenyl boron reagents are ubiquitous in synthesis and very important in stereospecific cross-coupling reactions to generate olefins. The hydroboration of alkynes is a useful and concise method for the preparation of alkenyl boranes, which are versatile organic building blocks in subsequent couplings.16 For example, Arase and Hoshi reported that at room temperature, in THF, Cy2BH and 9-BBN catalyzed the regioselective cis hydroboration reaction of alkynes, thereby preparing the E-alkenyl pinacol borate in high yields without being polluted by metal catalysts.17a In the past two decades, the Arase–Hoshi R2BH-catalyzed alkyne hydroboration reaction has been widely used (Scheme 1, eqn (2)–(4)).17b–d Recently, the groups of Yun Sawamura and Hoveyda have made great contributions to the borohydride reaction with Cu as a catalyst. In the case of adding MeOH, the organoboron reagent combined with α,β-unsaturated carbonyl compounds,18 olefins19 and alkyne20 underwent an addition reaction, respectively.
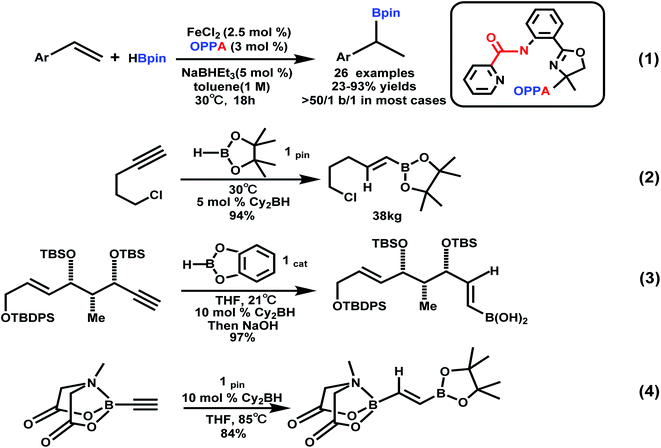 |
| Scheme 1 Hydroboration of styrenes and selected applications of Arase–Hoshi hydroboration.15,17b–d | |
In general, the type of alkyne insertion in the transition metal-catalyzed borohydride reaction determines the cis configuration of the final product. However, in the case of asymmetric internal alkynes as reactants, the problem of regioselectivity always exists.16b,d For example, Yun et al. have attempted to develop a new catalytic system for the regioselective synthesis of alkenyl boron compounds with diboron reagents.21 Ge et al. reported a cobalt-catalyzed Z-selective hydrosilylation of alkynes relying on catalysts Co(OAC)2 and pyridine-2,6-diimine ligands.22 Recently, transition metals have been used as catalysts for the hydroboration of alkynes, and the hydroboration of HBpin with internal asymmetric alkynes has been developed. The reaction had high enantioselectivity.23 Furthermore, the hydroboration of alkynes has made great progress, and numerous papers on hydroboration of alkynes have been published. Most reports indicated that the active alkyne and organic solvent (such as methanol or tetrahydrofuran) participation is essential for the reaction to proceed. In continuation of our efforts to develop organic transformations in aqueous media with numerous inherent advantages than reactions in conventional organic solvents,24 such as the environmental protection and harmlessness of the reaction process. Herein, we, for the first time, report the palladium-catalyzed hydroboration of unactivated alkynes in water. We explored the new method under green and mild conditions, and its catalytic system reacted well and had a wide range of substrates.
Results and discussion
In our initial experiments, we investigated the hydroboration of 4-octynes by employing a catalytic amount of Pd(PPh3)4 in the presence of B2(pin)2. The results are summarized in Table 1. When using THF as a solvent, moderate overall yield (45%) of series of isomers was obtained (Table 1, entry 1), which was confirmed by NMR and GC-MS.25 Fortunately, the isomerization problem was successfully avoided when the solvent THF was simply changed into pure water, which afforded a moderate yield (58%) with only one isomer (Table 1, entry 1). When 4 eq. HOAc was mixed with H2O, a high yield was obtained (Table 1, entry 1). Under the same conditions, when other acids were used, the yield decreased. For example, when CF3CO2H, PTSA·H2O and EtCO2H were used, only 12%, 5% and 39% yields were obtained, respectively (Table 1, entries 5, 6 and 7). It turned out that these acids were not better than HOAc. When solvents were replaced with MeOH, EA or DMF, all yields decreased (Table 1, entry 3, 4 and 8). Among the different solvents investigated, water was the best solvent for the hydroboronation reaction of 4-octynes (Table 1, entry 2). It is important to note that the employment of Pd(0) is indispensable for the hydroboration reaction, and without it, no desired product was obtained (Table 1, entry 13). It is also noteworthy to mention that only use H2O or HOAc as a solvent, and the reaction using Pd(PPh3)4 proceeded sluggishly to afford the desired product in a lower yield (Table 1, entries 9 and 10). Various palladium catalysts were also investigated in the hydroboration reaction, and when using Pd(OAc)2 or Pd2(dba)3 as catalysts, just trace products were obtained (Table 1, entries 11 and 12). The reaction was performed at different temperatures, as shown in Table 1, entries 14 and 15, within 60 and 70 °C, just 37% and 51% yields were obtained, respectively. However, the desired product was obtained with equivalent yields at a higher temperature, such as 90 °C (Table 1, entry 16). The reaction was also performed at different reaction times, as shown in Table 1, entries 17 and 18. When the time was 3 h and 6 h, the yields were only 32% and 41%, respectively. However, when reaction time was extended, almost same yields of the desired product were obtained with longer reaction time periods of 18 h and 24 h (Table 1, entry 19 and 20). With an optimal reaction protocol in hand, numerous unactivated alkynes were examined.
Table 1 Optimization of the reaction conditions using 4-octyne 1 and bis(pinacolato)-diboron 2a

|
Entry |
Catalysts |
Solvents |
Temperature |
Reaction time |
Yieldb (%) |
Reaction conditions: Pd catalyst (5%), solvent (1.5 mL), 1a (1 eq.), B2(pin)2 (2 eq.), HOAc (4 eq.). Isolated yield. |
1 |
Pd(PPh3)4 |
THF/HOAc |
80 °C |
12 h |
45 |
2 |
Pd(PPh3)4 |
H2O/HOAc |
80 °C |
12 h |
58 |
3 |
Pd(PPh3)4 |
MeOH/HOAc |
80 °C |
12 h |
52 |
4 |
Pd(PPh3)4 |
EA/HOAc |
80 °C |
12 h |
50 |
5 |
Pd(PPh3)4 |
H2O/CF3CO2H |
80 °C |
12 h |
12 |
6 |
Pd(PPh3)4 |
H2O/PTSA·H2O |
80 °C |
12 h |
5 |
7 |
Pd(PPh3)4 |
H2O/EtCO2H |
80 °C |
12 h |
39 |
8 |
Pd(PPh3)4 |
DMF/HOAc |
80 °C |
12 h |
48 |
9 |
Pd(PPh3)4 |
HOAc |
80 °C |
12 h |
23 |
10 |
Pd(PPh3)4 |
H2O |
80 °C |
12 h |
12 |
11 |
Pd(OAc)2 |
H2O/HOAc |
80 °C |
12 h |
Trace |
12 |
Pd2(dba)3 |
H2O/HOAc |
80 °C |
12 h |
Trace |
13 |
— |
H2O/HOAc |
80 °C |
12 h |
0 |
14 |
Pd(PPh3)4 |
H2O/HOAc |
60 °C |
12 h |
37 |
15 |
Pd(PPh3)4 |
H2O/HOAc |
70 °C |
12 h |
51 |
16 |
Pd(PPh3)4 |
H2O/HOAc |
90 °C |
12 h |
57 |
17 |
Pd(PPh3)4 |
H2O/HOAc |
80 °C |
3 h |
32 |
18 |
Pd(PPh3)4 |
H2O/HOAc |
80 °C |
6 h |
41 |
19 |
Pd(PPh3)4 |
H2O/HOAc |
80 °C |
18 h |
57 |
20 |
Pd(PPh3)4 |
H2O/HOAc |
80 °C |
24 h |
59 |
As shown in Table 2, Pd(PPh3)4 efficiently catalyzed the hydroboration reactions of various alkynes and B2(pin)2 in H2O at 80 °C to afford the corresponding products in moderate to good yields. Both aryl- and alkyl-substituted alkynes proceeded smoothly within the reaction time to provide the desired products in good to high yields (Table 2, entries 1 and 2). With 1-phenyl-1-butyne and 1-phenyl-1-pentyne substrates, under the optimal catalytic conditions, α-vinyl boronate could be exclusively furnished in excellent yields of 85% and 70%, respectively (Table 2, entries 5 and 6). For the activated substrates (Table 2, entries 7 and 8), the reaction proceeded smoothly with 68% and 60% yields to afford the desired products. When 1-phenyl-1-heptyne and 1-phenyl-1-propyne were used as substrates, under the optimal catalytic conditions, the desired products were provided with yields of 62% and 60%, respectively (Table 2, entries 11 and 13). For 1j, the corresponding product 2j was produced under the structure of the 1,4-bisarylation group. The reaction proceeded smoothly with a yield of 74%. It further demonstrated that this reaction had a wide range of substrates. It was gratifying to find that for the substrate 1l, the reaction delivered only one regioisomer 2l (Table 2, entries 12), which may be due to the complexation effect of the ortho olefin substituent with the palladium catalyst.
Table 2 Screening the reactivity of various alkynes in the boron addition catalyzed by Pd(PPh3)4a
It is exciting to find that with the substrate 1l, the reaction delivered only one regioisomer 2l (Table 2, entry 12) and 2l was in the Z configuration. Therefore, this result can inspire us to use this type of substrate to produce the product we need, without producing other by-products, and to conduct a strongly targeted synthesis. Such a high yield product can be applied in industrial synthesis. Furthermore, functional groups such as hydroxyl, ester and halide were all tolerated in this reaction, which further proved the high compatibility of this transformation (Table 2, entries 3 and 7–9).
On the basis of our experiments as well as literature precedents,15b we proposed the mechanism, which is shown in Scheme 2. The palladium(0) complex initially delivers the palladium hydride complex (intermediate 1) after the reaction with acetic acid, and the hydrogen atom transfers from AcOH to palladium, providing the [PdH] species 1. Next, intermediate 1 subsequently produces intermediate 2 after complexation and migratory insertions with alkyne I. Then, intermediate 2 undergoes a transmetallation with B2pin2 and furnishes intermediate 3. Lastly, intermediate 3, after a reductive elimination would generate the desired product II and regenerate the palladium(0) complex to close the catalytic cycle.
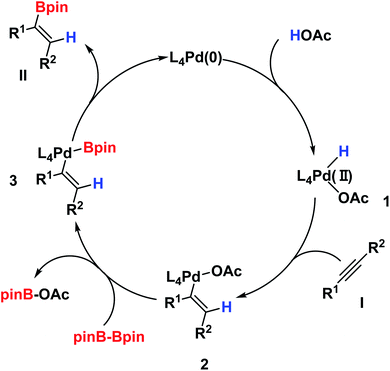 |
| Scheme 2 Proposed reaction mechanism. | |
Conclusion
In summary, we have developed an efficient procedure for the hydroboration reactions with unactivated alkynes based on a palladium catalyst, which provides a route for the synthesis of α-borylated-α,β-alkenes in water. These studies suggested that H2O is a suitable solvent for the palladium-catalyzed hydroboration reaction. Studies are underway to extend the application of the boron derivatives in organic syntheses.
Author contributions
MY, YY, WM and GZ executed the synthesis of the compounds and analysis of spectral data. MY, YY, YY and DL conceptualized the research work, wrote the main text. MY, YF, YW and FZ were involved in troubleshooting the synthesis and writing the ESI.† YY and DL provided the funding acquisition. All authors reviewed the manuscript and the ESI.†
Conflicts of interest
The authors declare no conflicts of interest.
Acknowledgements
This work was supported by the Wuhan Textile University (017/202127, 017/202161J), the Nanyang Technological University, Ministry of Education (No. M45110000), and A*STAR S. E. R. C. (No. 0721010024) and the Hubei Superior and Distinctive Discipline Group of “Mechatronics and Automobiles” (No. XKQ2019009).
References
- For reviews, see:
(a) Boronic Acids-Preparation and Applications in Organic Synthesis and Medicine, ed. D. G. Hall, Wiley-VCH Publishers, Weinheim, Germany, 2005 Search PubMed;
(b) I. Beletskaya and C. Moberg, Chem. Rev., 2006, 106, 2320–2354 CrossRef CAS PubMed;
(c) I. Beletskaya and C. Moberg, Chem. Rev., 1999, 99, 3435–3461 CrossRef CAS PubMed;
(d) G. J. Irvine, M. J. G. Lesley, T. B. Marder, N. C. Norman, C. R. Rice, E. G. Robins, W. R. Roper, G. R. Whittell and L. J. Wright, Chem. Rev., 1998, 98, 2685–2722 CrossRef CAS PubMed;
(e) N. Miyaura and A. Suzuki, Chem. Rev., 1995, 95, 2457–2483 CrossRef CAS.
-
(a) A. M. Irving, C. M. Vogels, L. G. Nikolcheva, J. P. Edwards, X.-F. He, M. G. Hamilton, M. O. Baerlocher, F. J. Baerlocher, A. Decken and S. A. Westcott, New J. Chem., 2003, 27, 1419–1424 RSC;
(b) H. Ito, C. Kawakami and M. Sawamura, J. Am. Chem. Soc., 2005, 127, 16034–16035 CrossRef CAS PubMed;
(c) S. Krautwald, M. J. Bezdek and P. J. Chirik, J. Am. Chem. Soc., 2017, 139, 3868–3875 CrossRef CAS PubMed.
- For reviews, see:
(a) A. Suzuki in Organoboranes in syntheses, ACS symposium series 783, ed. P. V. Ramachandran and H. C. Brown, American Chemical Society, Washington, 2001, pp. 80–93, ch. 6 Search PubMed;
(b) N. Miyaura in Organoboranes in syntheses, ACS symposium series 783, ed. P. V. Ramachandran and H. C. Brown, American Chemical Society, Washington, 2001, pp. 94–107, ch. 7 Search PubMed;
(c) Palladium reagents and catalysts, ed. J. Tusiji, John Wiley & Sons Publishers, Chichester, England, 2014, pp. 218–227 Search PubMed.
- M.-Y. Wang and Z.-Z. Shi, Chem. Rev., 2020, 120, 7348–7398 CrossRef CAS PubMed.
-
(a) Boronic Acids, ed. D. G. Hall, Wiley-VCH Publishers, Weinheim, 2011 Search PubMed;
(b) N. Miyaura and A. Suzuki, Chem. Rev., 1995, 95, 2457 CrossRef CAS;
(c) S. Kotha, K. Lahiri and D. Kashinath, Tetrahedron, 2002, 58, 9633 CrossRef CAS;
(d) G. A. Molander and N. Ellis, Acc. Chem. Res., 2007, 40, 275 CrossRef CAS PubMed.
- K. Nakajima, T. Kato and Y. Nishibayashi, Org. Lett., 2017, 19, 4323–4326 CrossRef CAS PubMed.
-
(a) A. Howell, J. E. R. Robertson, J. Quaresma Albano, A. Aschermannova, L. Mauriac, U. R. Kleeberg, I. Vergote, B. Erikstein, A. Webster and C. Moriis, J. Clin. Incol., 2002, 20, 3396–3403 CrossRef CAS PubMed;
(b) V. C. Jordan, J. Med. Chem., 2003, 46, 1081–1111 CrossRef CAS PubMed;
(c) M. Kusama, K. Miyauchi, H. Aoyama, M. Sano, M. Kimura, M. Mitsuyama, K. Komaki and H. Doihara, Breast Cancer Res. Treat., 2004, 8, 1–8 CrossRef PubMed.
-
(a) W. N. Palmer, T. Diao, I. Pappas and P. J. Chirik, ACS Catal., 2015, 5, 622–626 CrossRef CAS;
(b) A. D. Ibrahim, S. W. Entsminger and A. R. Fout, ACS Catal., 2017, 7, 3730–3734 CrossRef CAS;
(c) L. Zhang, Z. Zuo, X. Wan and Z. Huang, J. Am. Chem. Soc., 2014, 136, 15501–15504 CrossRef CAS PubMed.
-
(a) S. Kisan, V. Krishnakumar and C. Gunanathan, ACS Catal., 2017, 7, 5950–5954 CrossRef CAS;
(b) A. Kaithal, B. Chatterjee and C. Gunanathan, Org. Lett., 2015, 17, 4790–4793 CrossRef CAS PubMed.
-
(a) A. Bismuto, M. J. Cowley and S. P. Thomas, ACS Catal., 2018, 8, 2001–2005 CrossRef CAS;
(b) V. K. Jakhar, M. K. Barman and S. Nembenna, Org. Lett., 2016, 18, 4710–4713 CrossRef CAS PubMed.
-
(a) W. J. Jang, S. M. Song, J. H. Moon, J. Y. Lee and J. Yun, J. Am. Chem. Soc., 2017, 139, 13660–13663 CrossRef CAS PubMed;
(b) D. Gao, Y. Xiao, M. Liu, Z. Liu, M. K. Karunananda, J. S. Chen and K. M. Engle, ACS Catal., 2018, 8, 3650–3654 CrossRef CAS PubMed.
-
(a) K. Tseng, J. W. Kampf and N. K. Szymczak, ACS Catal., 2015, 5, 411–415 CrossRef CAS;
(b) A. J. MacNair, C. R. P. Millet, G. S. Nichol, A. Ironmonger and S. P. Thomas, ACS Catal., 2016, 6, 7217–7221 CrossRef CAS;
(c) F. Zhang, H. Song, X. Zhuang, C. Tung and W. Wang, J. Am. Chem. Soc., 2017, 139, 17775–17778 CrossRef CAS PubMed;
(d) S. R. Tamang and M. Findlater, J. Org. Chem., 2017, 82, 12857–12862 CrossRef CAS PubMed.
- G. Zhang, H. Zeng, J. Wu, Z. Yin, S. Zheng and J. C. Fettinger, Angew. Chem., Int. Ed., 2016, 55, 14369–14372 CrossRef CAS PubMed.
- W. Wang, X. Shen, F. Zhao, H. Jiang, W. Yao, S. Pullarkat, L. Xu and M. Ma, J. Org. Chem., 2018, 83, 69–74 CrossRef CAS PubMed.
-
(a) X. Chen, Z.-Y. Cheng and Z. Lu, Org. Lett., 2017, 19, 969–971 CrossRef CAS PubMed;
(b) J. Huang, W. Yan, C. Tan, W. Wu and H. Jiang, Chem. Commun., 2018, 54, 1770–1773 RSC.
-
(a) J. E. A. Luithle, J. Pietruszka and A. Witt, Chem. Commun., 1998, 2651–2652 RSC;
(b) X. He and J. F. Hartwig, J. Am. Chem. Soc., 1996, 118, 1696–1702 CrossRef CAS;
(c) N. Iwadate and M. Suginome, Org. Lett., 2009, 11, 1899–1902 CrossRef CAS PubMed;
(d) C. E. Tucker, J. Davidson and P. Knochel, J. Org. Chem., 1992, 57, 3482–3485 CrossRef CAS.
-
(a) A. Arase, M. Hoshi, A. Mijin and K. Nishi, Synth. Commun., 1995, 25, 1957–1962 CrossRef CAS;
(b) D. A. Evans and J. T. Starr, J. Am. Chem. Soc., 2003, 125, 13531–13540 CrossRef CAS PubMed;
(c) J. R. Struble, S. J. Lee and M. D. Burke, Tetrahedron, 2010, 66, 4710–4718 CrossRef CAS;
(d) E. M. Bassan, C. A. Baxter, G. L. Beutner, K. M. Emerson, F. J. Fleitz, S. Johnson, S. Keen, M. M. Kim, J. T. Kuethe, W. R. Leonard, P. R. Mullens, D. J. Muzzio, C. Roberge and N. Yasuda, Org. Process Res. Dev., 2012, 16, 87–95 CrossRef CAS.
-
(a) S. Mun, J. E. Lee and J. Yun, Org. Lett., 2006, 8, 4887–4889 CrossRef CAS PubMed;
(b) J. E. Lee and J. Yun, Angew. Chem., Int. Ed., 2008, 47, 145–147 CrossRef CAS PubMed;
(c) D. Noh, H. Chea, J. Ju and J. Yun, Angew. Chem., Int. Ed., 2009, 48, 6062–6064 CrossRef CAS PubMed;
(d) H. Chea, H. S. Sim and J. Yun, Adv. Synth. Catal., 2009, 351, 855–858 CrossRef CAS;
(e) H. S. Sim, X. Feng and J. Yun, Chem. - Eur. J., 2009, 15, 1939–1943 CrossRef CAS PubMed;
(f) J. M. O'Brrien, K. S. Lee and A. H. Hoveyda, J. Am. Chem. Soc., 2010, 132, 10630–10633 CrossRef PubMed;
(g) K. S. Lee, A. R. Zhugralin and A. M. Hoveyda, J. Am. Chem. Soc., 2009, 131, 7253–7255 CrossRef CAS PubMed;
(h) V. Lillo, A. Prieto, A. Bonet, M. M. Diazrequeojo, J. Ramirez, P. J. Perez and E. Fernandez, Organometallics, 2009, 28, 659–662 CrossRef CAS;
(i) M. Gao, S. B. Thorpe and W. L. Santos, Org. Lett., 2009, 15, 3478–3481 CrossRef PubMed;
(j) I. H. Chen, L. Yin, W. Itano, M. Kanai and M. Shibasaki, J. Am. Chem. Soc., 2009, 131, 11664–11665 CrossRef CAS PubMed.
- For recent select examples, see:
(a) Y. Sasaki, Y. Horita and C. Zhong, Angew. Chem., Int. Ed., 2011, 50, 2778–2782 CrossRef CAS PubMed;
(b) Y. Lee and A. H. Hoveyda, J. Am. Chem. Soc., 2009, 131, 3160–3161 CrossRef CAS PubMed.
- For recent select examples of hydroboration of alkynes, see:
(a) H. Jang, A. R. Zhugralin, Y. Lee and A. H. Hoveyda, J. Am. Chem. Soc., 2011, 133, 7859–7871 CrossRef CAS PubMed;
(b) F. Gao and A. H. Hoveyda, J. Am. Chem. Soc., 2010, 132, 10961–10963 CrossRef CAS PubMed;
(c) Y. Lee, H. Yang and A. H. Hoveyda, J. Am. Chem. Soc., 2009, 131, 18234–18235 CrossRef CAS PubMed;
(d) H. R. Kim, I. G. Jung, K. Yoo, K. Jang, E. S. Lee, J. Yun and S. U. Son, Chem. Commun., 2010, 46, 758–760 RSC;
(e) J. E. Lee, J. Kwon and J. Yun, Chem. Commun., 2008, 44, 733–734 RSC.
- For recently copper-promoted boration reactions with diboron, see:
(a) K. Takahashi, T. Ishiyama and N. Miyaura, J. Organomet. Chem., 2001, 625, 47–53 CrossRef CAS;
(b) H. Ito, Y. Sasaki and M. Sawamura, J. Am. Chem. Soc., 2008, 130, 15774–15775 CrossRef CAS PubMed;
(c) H. Ito, S. Ito, Y. Sasaki, K. Matsuura and M. Sawamura, J. Am. Chem. Soc., 2007, 129, 14856–14857 CrossRef CAS PubMed . For recently rhodium-catalyzed boration reactions with diboron, see: ;
(d) A. Kondoh and T. F. Jamison, Chem. Commun., 2010, 46, 907–909 RSC.
- W. J. Teo, C. Wang, Y. W. Tan and S. Ge, Angew. Chem., Int. Ed., 2017, 56, 4328–4332 CrossRef CAS PubMed.
-
(a) P. Zhang, J. M. Suarez, T. Driant, E. Derat, Y. Zhang, M. Menand, S. Roland and M. Sollogoub, Angew. Chem., Int. Ed., 2017, 129, 10961–10965 CrossRef;
(b) A. Bismuto, S. P. Thomas and M. J. Cowley, Angew. Chem., Int. Ed., 2016, 55, 15336 CrossRef PubMed;
(c) C. Lee, W. Shih, J. Zhou, J. H. Reibenspies and O. V. Ozerov, Angew. Chem., Int. Ed., 2015, 127, 14209–14213 CrossRef;
(d) D. Gao, Y. Xiao, M. Liu, Z. Liu, M. K. Karunananda, J. S. Chen and K. M. Engle, ACS Catal., 2018, 8, 3650–3654 CrossRef CAS PubMed;
(e) A. D. Ibrahim, S. W. Entsminger and A. R. Fout, ACS Catal., 2017, 7, 3730–3734 CrossRef CAS;
(f) T. Chen, C. Zhao and L. Han, J. Am. Chem. Soc., 2018, 140, 3139–3155 CrossRef CAS PubMed;
(g) A. Guthertz, M. Leutzsch, L. M. Wolf, P. Gupta, S. M. Rummelt, R. Goddard, C. Fares, W. Thiel and A. Furstner, J. Am. Chem. Soc., 2018, 140, 3156–3169 CrossRef CAS PubMed;
(h) N. Gorgas, L. G. Alves, B. Stoger, A. M. Martins, L. F. Veiros and K. Kirchner, J. Am. Chem. Soc., 2017, 139, 8130–8133 CrossRef CAS PubMed;
(i) J. R. Smith, B. S. L. Collins, M. J. Hesse, M. A. Graham, E. L. Myers and V. K. Aggarwal, J. Am. Chem. Soc., 2017, 139, 9148–9151 CrossRef CAS PubMed;
(j) J. Guo, B. Cheng, X. Shen and Z. Lu, J. Am. Chem. Soc., 2017, 139, 15316–15319 CrossRef CAS PubMed.
-
(a) T. P. Loh, G.-Q. Cao and J. Pei, Tetrahedron Lett., 1998, 39, 1453–1456 CrossRef CAS;
(b) T. P. Loh, G. L. Chua, J. J. Vittal and M. W. Wong, Chem. Commun., 1998, 861–862 RSC;
(c) T. P. Loh, J.-R. Zhou and Z. Yin, Org. Lett., 1999, 1, 1855–1857 CrossRef CAS;
(d) T. P. Loh, Z. Yin, H.-Y. Song and K. L. Tan, Tetrahedron Lett., 2003, 44, 911–914 CrossRef CAS;
(e) T. P. Loh and X.-R. Li, Angew. Chem., Int. Ed., 1997, 36, 980–982 CrossRef CAS;
(f) Z.-L. Shen and T. P. Loh, Org. Lett., 2007, 9, 5413–5416 CrossRef CAS PubMed;
(g) Z.-L. Shen, H. L. Cheong and T. P. Loh, Chem.–Eur. J., 2008, 14, 1875–1880 CrossRef CAS PubMed;
(h) Z.-L. Shen, Y. L. Yeo and T. P. Loh, J. Org. Chem., 2008, 73, 3922–3924 CrossRef CAS PubMed;
(i) J. Lu, F. Liu and T. P. Loh, Adv. Synth. Catal., 2008, 350, 1781–1784 CrossRef CAS;
(j) Z.-L. Shen, S. J. Ji and T. P. Loh, Tetrahedron, 2008, 64, 8159–8163 CrossRef CAS;
(k) Z.-L. Shen, H. L. Cheong and T. P. Loh, Tetrahedron Lett., 2009, 50, 1051–1054 CrossRef CAS;
(l) Y.-S. Yang, Z.-L. Shen and T. P. Loh, Org. Lett., 2009, 11, 1209–1212 CrossRef CAS PubMed;
(m) Y.-S. Yang, Z.-L. Shen and T. P. Loh, Org. Lett., 2009, 11, 2213–2215 CrossRef CAS PubMed;
(n) J. S. da Costa, R. K. Braun, P. A. Horn, D. S. Lüdtke and A. V. Moro, RSC Adv., 2016, 6, 59935–59938 RSC.
- The formation of different isomers when using THF as solvent may be due to the reversible insertion and elimination of palladium hydride to the triple bond or the allene intermediate
.
Footnotes |
† Electronic supplementary information (ESI) available: Experimental procedures, analytical data, and copies of 1H, 13C spectra (PDF). See DOI: 10.1039/d1ra09136k |
‡ These authors contributed equally to this work. |
|
This journal is © The Royal Society of Chemistry 2022 |