DOI:
10.1039/D1RA08631F
(Paper)
RSC Adv., 2022,
12, 2662-2667
Guaiane sesquiterpenes from the gorgonian Echinogorgia flora collected in the South China Sea†
Received
25th November 2021
, Accepted 11th December 2021
First published on 19th January 2022
Abstract
Echinoflorine (1), a new dimethylamino-substituted guaipyridine alkaloid with a novel γ-lactone-cyclohepta[c]pyridine fused skeleton, and three new guaiane sesquiterpene lactones, echinofloranolides A–C (2–4), together with eight known guaiane sesquiterpenes were isolated from the gorgonian Echinogorgia flora collected in the South China Sea. Their structures were elucidated by 1D and 2D NMR, HRESIMS, calculated ECD and DP4+ probability analyses.
Introduction
The guaipyridine alkaloid, featured by a cyclohepta-pyridine fused skeleton and strong bioactivities against hepatocarcinoma and inflammation, is the representative of the sesquiterpene alkaloid. However, there are just 18 natural guaipyridine alkaloids, all of which possess the cyclohepta[b]pyridine skeletons, and were obtained from the terrestrial Chinese traditional medicine plants Artemisia rupestris L.,1–3 Cananga odorata4 and pachouli oil.5 Potential bioactivity and rare existence of this kind of compounds in nature make it necessary to isolate new members with an effective method, although the synthesis of a similar type of these alkaloids has been reported.6–8 As the analogues of guaipyridine, guaiane sesquiterpenes are widely distributed among marine organisms, including gorgonians,9 soft corals,10 sponges11 and nudibranchs.12 These marine-derived guaianes, mostly featured with the α,β-unsaturated-β-methyl-γ-lactone moiety, partly show inhibitory effects on the generation of superoxide anion,13 antimicrobial,14 antioxidant and cytotoxic activities.15 Notably, for guaianes, no compounds contained syn-hydroxyl groups at C-1 and C-8 but three 1,8-epoxy-guaianes.9,16,17 Only one of them showed anti-inflammatory activity by inhibiting NO production.17
We recently obtained a new guaipyridine alkaloid, echinoflorine (1), with an unprecedented γ-lactone-cyclohepta[c]pyridine skeleton, and three new guaiane sesquiterpene lactones with the rare 1,8-epoxy moiety and diverse stereochemical feature, echinofloranolides A–C (2–4) (Fig. 1), from the gorgonian Echinogorgia flora collected in the South China Sea. Through a comprehensive analysis of spectroscopic data of 1D and 2D NMR, MS, experimental and calculated ECD, and DP4+ probability analyses, their structures were fully assigned. Now, we report the isolation and structural elucidation of these secondary metabolites.
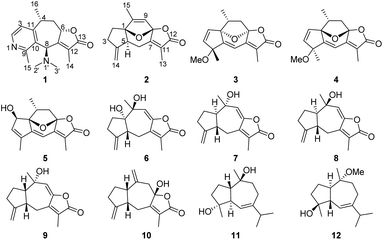 |
| Fig. 1 Structures of compounds 1–12. | |
Results and discussion
Echinoflorin A (1) was obtained as a light yellow oil, and its molecular formula was established as C17H22N2O2 by HRESIMS. The UV absorption maxima at 195, 229 and 260 nm (Fig. S15†) were characteristic of a typical guaipyridine alkaloid with the lactone group.4,5 The 13C NMR (DEPT) spectra displayed 17 carbon resonances, comprising five methyls, one methylene, five methines, six nonprotonated carbons including five olefinic carbons (one downfield imine) and one carbonyl. The 1H NMR revealed the presence of two pyridine protons at δH 7.10 (1H, d, J = 5.2 Hz) and 8.38 (1H, d, J = 5.2 Hz), one acyloxy-bearing methine proton at δH 5.29 (1H, dd, J = 9.5, 6.5 Hz), one nitrogen-bearing methine proton at δH 4.67 (1H, s), one methine proton at δH 4.11 (1H, m), two methylene protons at δH 2.44 (1H, dd, J = 12.7, 6.5 Hz) and 1.20 (1H, m), two olefinic methyl groups at δH 2.77 (3H, s) and 1.92 (3H, s), one dimethylamino group at δH 2.15 (6H, s), and one methyl group at δH 1.41 (3H, d, J = 7.1 Hz). Five of the eight degrees of unsaturation in 1 were accounted for one ester carbonyl and four olefinic bonds, which required the compound to be tricyclic.
The α,β-unsaturated-β-methyl-γ-lactone moiety was deduced by the HMBC correlations from H2-5 to C-6/C-7, from H-8 to C-6/C-7/C-12, and from H3-14 to C-7/C-12/C-13 (Fig. 2), together with the NMR resonances at δC 80.7/δH 5.29 and δC 173.7, and the IR absorption bands at 1759 and 1648 cm−1. The COSY correlation of H-2/H-3, and the HMBC correlations from H3-15 to C-9 and C-10, from H-8 to C-9, C-10 and C-11 and from H-2 to C-3, C-11 and C-9, and the deshielded shift at 8.38 (H-2) indicated the presence of the pyridine ring, which was also supported by the IR spectrum (1556, 1453 cm−1).5 The connection of the pyridine ring and lactone moieties was defined by the HMBC correlations from H-8 to C-7 and C-10 and from H3-16 to C-11, C-4 and C-5, combined with the COSY correlations of H3-16/H-4/H2-5/H-6. Furthermore, the location of the dimethylamino group at C-8 was confirmed by the HMBC correlations from H3-2′ and H3-3′ to C-8 (Fig. 2). Therefore, the planar structure of echinoflorin was determined.
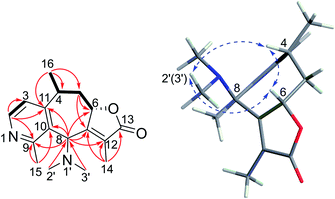 |
| Fig. 2 Key COSY (bold), HMBC (arrows), and NOESY (dashed blue arrows) correlations of 1. | |
The present structure of compound 1 showed a quasi-rigid skeleton with only slight flexibility on the two fragments of C11–C4–C5–C6 and C7–C8–C10, which were carried out in the MMFF minimization force field in the Spartan 10 software package (Fig. S1†). Thus, the pairwise NOESY correlations among H-4, H3-2′(3′) and H-6 indicated the relative configuration as 4R*, 6S*, 8S* (Fig. 2). In addition, for 4R*, 6S*, 8S*-configurations, the calculations of 1H and 13C NMR data deduced the high fitting degree (Fig. 3 and S2†) and 100% probability based on DP4+ analysis (Fig. S3†). Notably, the deshielded shift of H-4 at δH 4.11 is not reasonable as usual. The NMR calculations combined with the optimized conformation analysis (Table S4†) suggested that this shift may be caused by N-1′. The ECD calculations (Fig. 4), using the time-dependent density functional theory (TDDFT) method at the RB3LYP/DGDZVP level,18 established the absolute configuration of compound 1 as 4R, 6S, 8S.
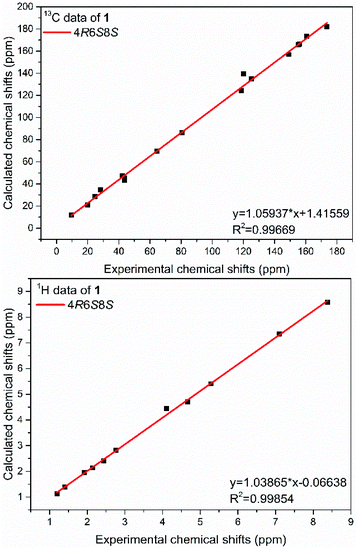 |
| Fig. 3 The fitting lines of experimental and calculated NMR data of 1. | |
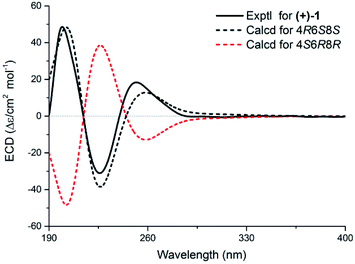 |
| Fig. 4 Experimental and calculated ECD spectra of 1. | |
Echinofloranolide A (2) was obtained as a colorless oil. Its molecular formula of C15H18O4 was determined by HRESIMS. The NMR data showed typical features of the guaiane-type sesquiterpene.16 The γ-lactone moiety was confirmed by the HMBC correlations and IR spectrum as in 1. Moreover, the bicyclo[7.5.0]decadiene skeleton was deduced by the COSY correlations of H2-2/H2-3 and H-5/H2-6, combined with the HMBC correlations from H2-14 to C-3, C-4 and C-5, from H-6 to C-1, C-4, C-5, C-7 and C-8, from H-9 to C-8, from H3-15 to C-1, C-9 and C-10, and from H2-3 to C-1 (Fig. 5). Taking into account the molecular formula of 2 and the deshielded shifts of C-1 (δC 95.8) and C-8 (δC 108.5), the connection of C-1 and C-8 through an ether bridge was suggested.9,16,19 In addition, to confirm the presence of the ether bridge rather than the dihydroxy and determine the relative configuration of 2, the NMR shift calculations and DP4+ probability analysis based on the gauge independent atomic orbital (GIAO) method at the PCM/b3lyp/6-311+G(d,p) level20 of all target conformations (Fig. S4†) were operated. The result showed that 1R*, 5S* 8S*-configurations with the ether bridge accounted for the most DP4+ probability of 99.89% (Fig. S5†). Ultimately, the absolute configuration was confirmed as 1R, 5S, 8S by the ECD calculation (Fig. 6).
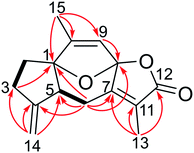 |
| Fig. 5 Key COSY (bold), and HMBC (arrows) correlations of 2. | |
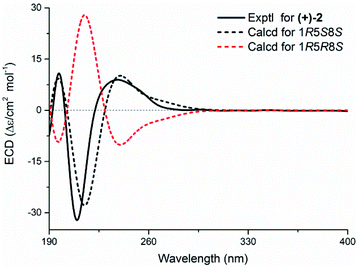 |
| Fig. 6 Experimental and calculated ECD spectra of 2. | |
Echinofloranolide B (3) was obtained as a colorless oil. Its molecular formula of C16H18O4 was suggested by HRESIMS. The NMR spectra displayed that 3 possessed the same carbon skeleton as 2, except for the methoxy group at C-4 and the double bands of Δ2 and Δ9 in 3, which was deduced from the COSY and HMBC correlations. The 1D and 2D NMR spectra indicated that compound 4 (echinofloranolide C) was the epimer of 3. The NOESY correlations of H-10/H3-14 and H-2/H3-15 indicated that compound 3 had 1S*, 4R*, 8R*, 10R*-configurations (Fig. 7). Finally, the absolute configuration of 3 was deduced as 1S, 4R, 8R, 10R by ECD calculations (Fig. 8).18 Similarly, the 1R, 4S, 8S, 10R-configurations of 4 were also confirmed in the same way (Fig. 9).
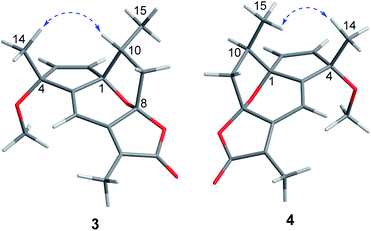 |
| Fig. 7 NOESY (dashed blue arrows) correlations of 3 and 4. | |
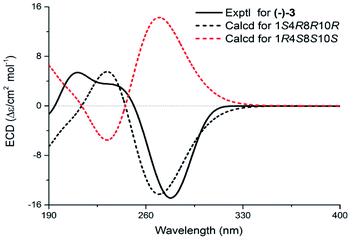 |
| Fig. 8 Experimental and calculated ECD spectra of 3. | |
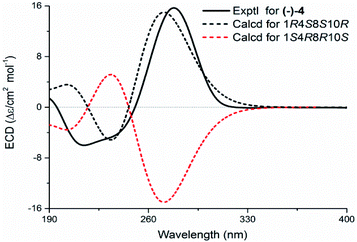 |
| Fig. 9 Experimental and calculated ECD spectra of 4. | |
Interestingly, the experimental ECD spectra of compounds 3 and 4 were highly symmetric (Fig. S6†). To explain this phenomenon, the optimal conformations of 3 and 4e (the enantiomer of 4) and their MOs (molecular orbitals) were analyzed (Fig. 10). The results displayed a high coincidence of the two optimal conformations and the nearly same wave functions in per molecular orbital, which revealed that the relative position at C-10 had little effect on the wave functions in these structures.
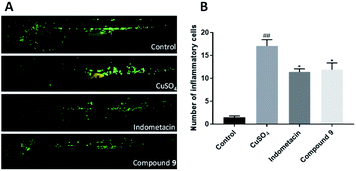 |
| Fig. 10 (A) Images of the number of macrophages surrounding neuromast sites in transgenic fluorescent zebrafish [Tg:zlyz-EGFP] treated with compound 9, using indomethacin as the positive control; (B) the analysis of macrophages surrounding neuromast in zebrafish treated with the positive control, compound 9. The data are represented as the mean ± SE. ##p < 0.01 vs. the control group, *p < 0.05 vs. the CuSO4-include group. | |
The anti-inflammatory activity of compounds 1–12 in vivo was indicated in transgenic fluorescent zebrafish (Tg:zlyz-EGFP) with the infiltration of macrophages around neuromast under the stimulation of CuSO4, which could make the neural mast cells and mechanical sensory cells of the zebrafish lateral line produce a strong acute inflammatory response.21 Compound 9
22 could decrease the number of macrophages as the positive control of indomethacin (Fig. 10).
Conclusions
In conclusion, the present study of the gorgonian Echinogorgia flora resulted in one novel guaipyridine alkaloid, three new and eight known guaiane sesquiterpene lactones. Echinoflorine (1) is the first marine guaiane cyclohepta[c]pyridine alkaloid, whose skeleton differs from the cyclohepta[b]pyridines from terrestrial organisms. Echinofloranolides A–C (2–4) possess the 1,8-epoxy moieties, which were only isolated from the gorgonian Menella woodin.9 In addition, without X-ray crystals, the configurations of these multichiral compounds depended on multiple strategies. The NOESY experiments should be used combined with a comprehensive conformational study, and the quantum chemical based calculations mainly including NMR (such as CP3,23 DP4+20 and J-DP4
24) and ECD are gradually perfect and indispensable, especially for the rigid and quasi-rigid structures. Compound 9 displayed anti-inflammatory activity in the zebrafish essay.
Experimental section
General experimental procedures
Optical rotations were obtained on a Jasco P-1020 polarimeter. UV spectra and circular dichroism were recorded on a Jasco J-815 CD Spectropolarimeter. IR spectra were taken on a Nicolet NEXUS 470 spectrophotometer, KBr disks. NMR spectra were measured on a JEOL JNM-ECP600 (1H, 600 MHz; 13C, 151 MHz) spectrometer. The 7.26 and 77.2 ppm resonances of CDCl3, and the 2.50 and 39.5 ppm resonances of DMSO were used as internal references for 1H and 13C NMR spectra, respectively. HRESIMS data were measured on a Thermo LTQ-Orbitrap. Reversed-phase (RP) HPLC was performed on an Agilent 1100 series liquid chromatography equipped with a DAD G1315A detector, equipped with a semi-preparative reversed-phase column (YMC-packed C18, 5 μm, 250 × 10 mm, 1.5 mL min−1) or an analytic chiral column DAICEL IC-3. Silica gel (300–400 mesh) and Sephadex LH-20 (Amersham Pharmacia Biotech AB) were used for column chromatography, and precoated silica gel plates (GF254) were used for TLC.
Coral material
The gorgonian E. flora was collected from Weizhou Island in the South China Sea, and was identified by Prof. RenLin Zou of the South China Sea Institute of Oceanology. The voucher specimen (WZD-2010-07), frozen at −20 °C, was deposited at the School of Medicine and Pharmacy, Ocean University of China, P. R. China.
Extraction and isolation
The whole fresh specimen of E. flora (7.2 kg, wet weight) was crushed and extracted with MeOH three times (each time for 3 days) at room temperature. The combined extract (130.0 mg) was subjected to silica gel column chromatography eluting with a gradient of petroleum ether/acetone (from 0
:
1 to 1
:
0, v/v) to obtain ten fractions (A–J). Fraction C (2.2 g) was subjected to a Sephadex LH-20 column eluted with CH2Cl2/MeOH (1
:
1, v/v), to give two subfractions C-1 and C-2. C-2 (1.5 g) was further separated by reversed-phase silica gel CC eluting with gradient mixtures of MeOH/H2O (1
:
4, 1
:
1, 4
:
1 and 10
:
0, v/v) and obtained five portions C-2-1–C-2-5. The portion C-2-3 (400 mg) eluted with MeOH/H2O (1
:
1, v/v) was then chromatographed on silica gel CC eluted by petroleum ether/acetone (10
:
1, v/v), and finally purified by semi-preparative HPLC (ODS, 5 μm, 250 × 10 mm; MeOH/H2O, 80/20, v/v; 2.0 mL min−1) to afford compound echinoflorine (2.0 mg). The portion D (7.6 g) was subsequently separated into two subfractions (C-1 and C-2) by a Sephadex LH-20 column eluted with CH2Cl2/MeOH (1
:
1, v/v) to afford two subfractions D-1 and D-2. D-2 (4.1 g) was divided to four subfractions (D-2-1–D-2-5) by silica gel CC (petroleum ether/acetone, 50/1 40/1, 30/1, 0/1, v/v). D-2-4 (1.1 g) purified by reversed-phase silica gel CC (MeOH/H2O, 40/1, v/v) and semi-preparative HPLC (ODS, 5 μm, 250 × 10 mm; MeOH/H2O, 60/40, v/v; 1.5 mL min−1) to give echinofloran A (3.5 mg) and the mixture of echinoflorans B and C, which could not be separated in the above semi-preparative HPLC and was divided into echinoflorans B (2.0 mg) and C (1.1 mg) by chiral phase HPLC (IC, 5 μm, 250 × 4.6 mm, n-hexane/isopropanol, 80
:
20, v/v).
Echinoflorine (1). Colorless oil; [α]24D +73 (c 5.0, MeOH); UV (MeOH) λmax (log
ε) 195 (4.05), 229 (3.45), 260 (3.10) nm; ECD (1.75 mM, MeOH) λmax (Δε) 199 (48.50), 226 (−31.10), 252 (18.39) nm; IR (KBr) νmax 3439, 2924, 2853, 1759, 1648, 1556, 1453, 1095, 1031 cm−1; 13C and 1H NMR data, Table 1; HRESIMS m/z 287.1756 [M + H]+ (calcd for C17H23N2O2, 287.1754).
Table 1 13C and 1H data of compounds 1–4
Position |
1 |
2 |
3 |
4 |
δCa |
δHb (mult, J in Hz) |
δCa |
δHb (mult, J in Hz) |
δCc |
δHd (mult, J in Hz) |
δCc |
δHd (mult, J in Hz) |
Recorded at 151 MHz in CDCl3. Recorded at 600 MHz in CDCl3. Recorded at 151 MHz in DMSO. Recorded at 600 MHz in DMSO. |
1 |
|
|
95.8 |
|
91.3 |
|
93.8 |
|
2 |
149.2 |
8.38 (d, 5.2) |
29.8 |
a 2.59 (m) |
141.7 |
6.21 (d, 6.0) |
141.6 |
6.13 (d, 6.0) |
b 2.65 (m) |
3 |
118.8 |
7.10 (d, 5.2) |
29.7 |
a 1.96 (m) |
131.4 |
6.23 (d, 6.0) |
132.6 |
6.15 (d, 6.0) |
b 1.99 (m) |
4 |
28.4 |
4.11 (m) |
149.7 |
|
83.7 |
|
84.4 |
|
5 |
42.2 |
a 1.20 (m) |
41.5 |
2.46 (m) |
156.4 |
|
158.0 |
|
b 2.44 (dd, 12.7, 6.5) |
6 |
80.7 |
5.29 (dd, 9.5, 6.5) |
21.8 |
a 2.65 (m) |
110.0 |
6.53(s) |
113.7 |
6.68 (s) |
|
|
|
|
b 2.93 (d, 17.1) |
|
|
|
|
7 |
160.6 |
|
154.2 |
|
160.8 |
|
158.2 |
|
8 |
64.5 |
4.67 (s) |
108.5 |
|
106.9 |
|
106.5 |
|
9 |
156.0 |
|
124.5 |
5.78 (s) |
39.0 |
a 1.79 (dd, 13.2, 3.6) |
37.7 |
a 1.67 (m) |
b 2.38 (dd, 13.2, 3.6) |
b 2.52 (m) |
10 |
130.1 |
|
147.8 |
|
38.9 |
2.20 (m) |
39.2 |
2.61 (m) |
11 |
155.2 |
|
120.0 |
|
116.7 |
|
117.1 |
|
12 |
125.0 |
|
172.5 |
|
171.2 |
|
171.2 |
|
13 |
173.7 |
|
12.7 |
1.79 (s) |
7.9 |
1.86 (s) |
8.0 |
1.87 (s) |
14 |
9.5 |
1.92 (s) |
107.0 |
a 4.93 (s) |
26.4 |
1.39 (s) |
24.6 |
1.44 (s) |
b 5.07 (s) |
15 |
24.7 |
2.77 (s) |
12.8 |
1.89 (s) |
18.8 |
1.07 (d, 7.0) |
16.0 |
0.85 (d, 7.0) |
16 |
20.0 |
1.41 (d, 7.1) |
|
|
51.1 |
2.96 (s) |
50.6 |
2.88 (s) |
Me-2′/3′ |
43.7 |
2.15 (s) |
|
|
|
|
|
|
Echinofloranolide A (2). Colorless oil; [α]24D +13 (c 2.5, MeOH); UV (MeOH) λmax (log
ε) 198 (4.43), 229 (4.20), 255 (3.83) nm; ECD (0.82 mM, MeOH) λmax (Δε) 197 (10.80), 210 (−32.18), 238 (8.93) nm; IR (KBr) νmax 2924, 2853, 1777, 1698, 1436, 1252, 1114, 1032, 1004, 979 cm−1; 13C and 1H NMR data, Table 1; HRESIMS m/z 267.0996 [M + Na]+ (calcd for C15H16O3Na, 267.0992).
Echinofloranolide B (3). Colorless oil; [α]24D −18 (c 1.2, MeOH); UV (MeOH) λmax (log
ε) 200 (4.14), 270 (4.43) nm; ECD (0.73 mM, MeOH) λmax (Δε) 211 (5.38), 233 (3.54), 278 (−14.88) nm; IR (KBr) νmax 2926, 2854, 1776, 1670, 1457, 1298, 1186, 1100, 1039, 963 cm−1; 13C and 1H NMR data, Table 1; HRESIMS m/z 275.1278 [M + H]+ (calcd for C16H19O4, 275.1278).
Echinofloranolide C (4). Colorless oil; [α]24D −14 (c 2.3, MeOH); UV (MeOH) λmax (log
ε) 196 (4.31), 273 (4.03) nm; ECD (1.02 mM, MeOH) λmax (Δε) 215 (−6.00), 278 (15.69) nm; IR (KBr) νmax 2926, 2854, 1776, 1670, 1457, 1298, 1186, 1100, 1039, 964 cm−1; 13C and 1H NMR data, Table 1; HRESIMS m/z 275.1278 [M + H]+ (calcd for C16H19O4, 275.1278).
Computational section
Conformational analyses were carried out in the MMFF minimization force field by the Spartan 10 software package. The resulting conformers were optimized using DFT at the B3LYP/6-31+G(d,p) level in the gas phase by the GAUSSIAN 09 program. The optimized conformations, whose Boltzmann distributions of Gibbs free energies were more than 1.0 percent, were used for the ECD calculations using the TD-DFT method with the basis set RB3LYP/DGDZVP, or the NMR calculations using the GIAO method at the PCM/b3lyp/6-311+G(d,p) level.18,20
Anti-inflammatory assay
A model of transgenic zebrafish [Tg:zlyz-EGFP] for expressing enhanced green fluorescent protein (EGFP) was used to evaluate the effect of the compounds on anti-inflammatory activity. Zebrafish were cultivated by the Biology Institute, Qilu University of Technology (Shandong Academy of Sciences), Jinan, China. Healthy zebrafish embryos were selected into 24-well plates (n = 10/well) in a 2 mL final volume of embryo medium 3 days post fertilization (dpf) and divided into 16 groups: a control group (fresh fish water), a model group (40 μM CuSO4), a positive drug group (20 μM indomethacin, Solarbio, China) and 12 drug groups (20 μM compounds 1–12). CuSO4 was added to the drug groups and incubated for 1 h after treatment with different compounds for 2 h. A fluorescence microscope (AXIO, ZOom.v16) was used to take photos of each zebrafish embryo, and Image-Pro Plus software count the number of macrophages.21
Conflicts of interest
There are no conflicts to declare.
Acknowledgements
This work was supported by the National Natural Science Foundation of China (No. 41876161, 41776136, 21861142006, and 81991522), the National Key Research and Development Program of China (No. 2018YFC0310903), NSFC-Shandong Joint Fund for Marine Science Research Centers (No. U1606403), National Science and Technology Major Project for Significant New Drugs Development (2018ZX09735004). And Special thanks are given to Dr Renlin Zou (South China Sea Institute of Oceanology, Chinese Academy of Sciences, Guangzhou 510301, China), for the gorgonian species identification, and the Center for High Performance Computing and System Simulation (Pilot National Laboratory for Marine Science and Technology) for the computer calculations.
Notes and references
- F. He, A. E. Nugroho, C. P. Wong, Y. Hirasawa, O. Shirota, H. Morita and H. A. Aisa, Chem. Pharm. Bull., 2012, 60, 213–218 CrossRef CAS PubMed.
- Z. Su, H. K. Wu, F. He, U. Slukhan and H. A. Aisa, Helv. Chim. Acta, 2010, 93, 33–38 CrossRef CAS.
- Z. Su, H. Wu, Y. Yang, H. A. Aisa, U. Slukhan and S. Aripova, J. Sep. Sci., 2008, 31, 2161–2166 CrossRef CAS PubMed.
- T. J. Hsieh, F. R. Chang, Y. C. Chia, C. Y. Chen, H. F. Chiu and Y. C. Wu, J. Nat. Prod., 2001, 64, 616–619 CrossRef CAS PubMed.
- G. Buechi, I. M. Goldman and D. W. Mayo, J. Am. Chem. Soc., 1966, 88, 3109–3113 CrossRef CAS.
- P. M. M. Shelton, S. M. Grosslight, B. J. Mulligan, H. V. Spargo, S. S. Saad and J. R. Vyvyan, Tetrahedron, 2020, 76, 131500 CrossRef CAS.
- D. Craig and G. D. Henry, Eur. J. Org. Chem., 2006, 2006, 3558–3561 CrossRef.
- A. Van der Gen, L. M. Van der Linde and J. G. Witteveen, Recl. Trav. Chim. Pays-Bas, 1972, 91, 1433–1440 CrossRef CAS.
- C. N. Diep, E. G. Lyakhova, D. V. Berdyshev, A. I. Kalinovsky, V. A. Tu, N. X. Cuong, N. H. Nam, C. V. Minh and V. A. Stonik, Tetrahedron Lett., 2015, 56, 7001–7004 CrossRef CAS.
- C. S. Phan, T. Kamada, T. Ishii, T. Hamada and C. S. Vairappan, Nat. Prod. Commun., 2018, 13, 15–16 CrossRef.
- S. Kozawa, H. Ishiyama, J. Fromont and J. I. Kobayashi, J. Nat. Prod., 2008, 71, 445–447 CrossRef CAS PubMed.
- E. Manzo, M. L. Ciavatta, M. Gavagnin, E. Mollo, Y. W. Guo and G. Cimino, J. Nat. Prod., 2004, 67, 1701–1704 CrossRef CAS PubMed.
- S. Y. Kao, J. H. Su, T. L. Hwang, J. H. Sheu, Y. D. Su, C. S. Lin, Y. C. Chang, W. H. Wang, L. S. Fang and P. J. Sung, Tetrahedron, 2011, 67, 7311–7315 CrossRef CAS.
- Y. Seo, J. R. Rho, N. Geum, J. B. Yoon and J. Shin, J. Nat. Prod., 1996, 59, 985–986 CrossRef CAS PubMed.
- G. W. Zhang, X. Q. Ma, J. Y. Su, K. Zhang, H. Kurihara, X. S. Yao and L. M. Zeng, Nat. Prod. Res., 2006, 20, 659–664 CrossRef CAS PubMed.
- M. G. Phan, T. T. N. Tran, T. S. Phan, K. Matsunami and H. Otsuka, Phytochem. Lett., 2014, 9, 137–140 CrossRef CAS.
- Y. Liu, J. H. Ma, Q. Zhao, C. R. Liao, L. Q. Ding, L. X. Chen, F. Zhao and F. Qiu, J. Nat. Prod., 2013, 76, 1150–1156 CrossRef CAS PubMed.
- X. Luo, Q. Wang, X. Tang, J. Xu, M. Wang, P. Li and G. Li, J. Nat. Prod., 2021, 84, 61–70 CrossRef CAS PubMed.
- S. Liang, Y. H. Shen, Y. Feng, J. M. Tian, X. H. Liu, Z. Xiong and W. D. Zhang, J. Nat. Prod., 2010, 73, 532–535 CrossRef CAS PubMed.
- N. Grimblat, M. M. Zanardi and A. M. Sarotti, J. Org. Chem., 2015, 80, 12526–12534 CrossRef CAS PubMed.
- J. J. Li, Y. Zhang, L. W. Han, Q. P. Tian, Q. X. He, X. M. Wang, C. Sun, J. Han and K. C. Liu, Fish Shellfish Immunol., 2018, 83, 205–212 CrossRef CAS PubMed.
- L. Li, C. Y. Wang, H. Huang, E. Mollo, G. Cimino and Y. W. Guo, Helv. Chim. Acta, 2008, 91, 111–117 CrossRef CAS.
- S. G. Smith and J. M. Goodman, J. Org. Chem., 2009, 74, 4597–4607 CrossRef CAS PubMed.
- N. Grimblat, J. A. Gavin, A. Hernandez Daranas and A. M. Sarotti, Org. Lett., 2019, 21, 4003–4007 CrossRef CAS PubMed.
Footnotes |
† Electronic supplementary information (ESI) available: ECD calculations, detailed DP4+ probability, HRESIMS, 1D and 2D NMR, IR and UV spectra of compounds 1–4. See DOI: 10.1039/d1ra08631f |
‡ X. Luo, R. Wu and X. Han contributed equally to this work. |
|
This journal is © The Royal Society of Chemistry 2022 |
Click here to see how this site uses Cookies. View our privacy policy here.