DOI:
10.1039/D1RA07641H
(Paper)
RSC Adv., 2022,
12, 6869-6875
Analysis method development and health risk assessment of pesticide and heavy metal residues in Dendrobium Candidum†
Received
15th October 2021
, Accepted 22nd February 2022
First published on 1st March 2022
Abstract
Herbal medicines that are widely used worldwide are easily contaminated by pesticides and heavy metals, threatening human health. In this study, a modified QuEChERS pre-treatment method combined with HPLC/GC-MS/MS was established for the determination of 24 pesticide residues in Dendrobium candidum. The average recoveries of 24 pesticides in D. candidum were 76.9–110.0% with the relative standard deviation (RSD) of 0.28–11.40%, and their limits of detection (LOD) and limits of quantitation (LOQ) were 0.005–10 and 0.011–22 μg kg−1, respectively. The results showed that 83.33% of all samples had detected pesticide residues with the concentrations of 0.06–312.83 μg kg−1. Meanwhile, microwave digestion combined with ICP-MS was used to detect the residues of 8 heavy metals in D. candidum. The average recoveries of 8 heavy metals were 82.7–108.1% with an RSD of 1.4–8.0%, and their LOD and LOQ were 0.0001–0.05 mg kg−1 and 0.0003–0.2 mg kg−1, respectively. The results indicated that 8 heavy metals were all detected in all samples, and the highest concentration of Zn was 11.97 mg kg−1. Furthermore, the health risk assessment showed that the risk of the detected pesticides and heavy metals in samples to humans, specifically to the general population including adults and children, was acceptable.
1. Introduction
Safety and pollution problems of herbal medicines are global issues where pesticide and heavy metal residues are significant factors threatening human health. It has been confirmed by the WHO that approximately 80% of the world population use medicinal plants.1 Dendrobium candidum, a perennial herb of Dendrobium in Ochidaceae, is one of the rare herbal medicines in China, whose medicinal parts are fresh or dry stems.2 Oral administration of D. candidum powder or extract is a traditional Chinese medicine treatment and health care method.3 Many studies have shown that some components of D. candidum have positive effects on health, such as inhibiting cancer, promoting body fluid production and reducing blood sugar.4,5 The use of D. candidum in traditional Chinese medicine has brought health, and also brought considerable production and consumption. Due to the lack of wild D. candidum, artificial cultivation of D. candidum has developed continuously since the 1970s.6 In 2017, the output of D. candidum in China was 27
000 tons, and the output value exceeded 1.5 billion dollars.7 From the perspective of production distribution, D. candidum is mainly distributed in Zhejiang province, Yunnan province, Guangdong province, Guangxi province and other places in China. Among them, the production of D. candidum in Zhejiang is the largest, accounting for about 60%.8
Pesticides are often used in large quantities to prevent and control pests and diseases in the herbal medicine production process, resulting in pesticide residue pollution. Luo et al.9 have reported that 88.03% of the tested 1771 herbal medicine samples contained pesticide residues, and 59.01% of them were above the limit of European Pharmacopoeia. Pesticide residues may cause liver toxicity and lipid metabolism disorders in zebrafish.10 More importantly, it may enter the human body through food and water, thereby harming health. First of all, pesticide residues in food may cause acute and chronic poisoning.11 Additionally, exposure to some pesticides, such as BHC, DDT, procymidone and other endocrine disruptors, have a harmful impact on human reproduction and development.12 They can be passed through the food web and accumulated in cells and organs, causing abnormalities in the endocrine system, nervous system, reproductive system and immune system.13,14 In particular, many insecticides such as pyrethroid pesticides target the nervous system of insects, but also have neurotoxicity to humans.15 Therefore, it is necessary to improve the extraction and detection analysis methods of pesticide residues in D. candidum, and comprehensively evaluate their dietary risks.
Meanwhile, herbal medicine can absorb and enrich heavy metals from soil, water and air when they are planted, processed and transported.16 Among all kinds of medicinal materials, herb-type medicinal materials such as D. candidum had the highest risk of heavy metal content.17 Chronic oral exposures to some heavy metals can cause detrimental effects in humans. Very heavy metals (e.g. cerium) are not efficiently absorbed.18 Long-term exposure to arsenic increased the risk of skin cancer, lung cancer and other cancers.19,20
In this study, the residues of 24 pesticides and 8 heavy metals were detected in D. candidum from different production areas in China. This study aims to (1) establish a modified QuEChERS-HPLC/GC-MS/MS analysis method for detecting 24 pesticides in D. candidum; (2) analyse the residual characteristics of 24 representative pesticides in D. candidum and assess their dietary risks; and (3) detect the residues of 8 typical heavy metals in D. candidum by ICP-MS combined with microwave digestion and evaluate their health risk.
2. Experimental
2.1 Chemicals and reagents
All pesticides standards including α-BHC(100 mg L−1), β-BHC (100 mg L−1), γ-BHC (100 mg L−1), δ-BHC (100 mg L−1), p,p′-DDE (100 mg L−1), o,p′-DDT (100 mg L−1), p,p′-DDT (100 mg L−1), p,p′-DDD (100 mg L−1), azoxystrobin (99.0%), carbendazim (99.0%), chlorothalonil (98.9%), chlorpyrifos (99.5%), cypermethrin (98.2%), chlorantraniliprole (98.2%), dichlorvos (99.7%), difenoconazole (99.2%), fenpropathrin (98.9%), isocarbophos (98.9%), procymidone (99.5%), prochloraz (99.2%), pyraclostrobin (98.1%), thiophanate-methyl (99.3%), tebuconazole (99.5%) and λ-cyhalothrin (93.5%) were purchased from Dr Ehrenstorfer GmbH (Augsburg, Germany). Acetonitrile (HPLC grade) were provided by Bangyi Chemical Co., Ltd. (Hangzhou, China). Anhydrous magnesium sulphate, anhydrous sodium sulphate and n-hexane were of analytical grade. Primary secondary amine (PSA) was provided by Agela Technologies.
Each single element stock solution (1000 mg L−1) of Cu, Zn, Cr, Ni, Pb, As, Cd, and Hg were purchased from National Standard Material Research Centre (Beijing, China). Guaranteed reagent nitric acid (HNO3) was provided by Fanhong Trading Co., Ltd. (Guangzhou, China).
2.2 Standard solution preparation
Pesticides were divided into two groups where group I consisted of α-BHC, β-BHC, γ-BHC, δ-BHC, p,p′-DDE, o,p′-DDT, p,p′-DDE, p,p′-DDD, azoxystrobin, chlorothalonil, chlorpyrifos, dichlorvos, isocarbophos, fenpropathrin, λ-cyhalothrin, difenoconazole and cypermethrin, while group II included carbendazim, thiophanate-methyl, chlorantraniliprole, tebuconazole, procymidone, prochloraz and pyraclostrobin. For group I, stock solutions (1000 mg L−1) for each pesticide were prepared by n-hexane and mixed for the multi-residue pesticide standard solution (10 mg L−1), and then dilute the mixed standard solution with n-hexane to 0.1, 1, and 10 mg L−1, and stored at −20 °C until analysis. For group II, the mixed standard solution was prepared according to the above procedure, but all solvents were replaced with acetonitrile. In addition, the single element stock solution of Cu, Zn, Cr, Ni, Pb, As, Cd and Hg was diluted step by step with 5% HNO3 solution to prepare appropriate mixed standard working solution.
2.3 Sample preparation
2.3.1 Extraction and purification of pesticide residues. Fresh D. candidum was collected by five-spot-sampling method from the experimental plots (Table S1†) and storied at −20 °C. The extraction method of pesticide residues was modified on the basis of QuEChERS. After homogenization, 10.0 g of D. candidum were accurately weighed into a 50 mL centrifuge tube containing 2.0 g NaCl and 3.0 mL distilled. After standing for 30 min, pesticide residues were extracted with 15 mL acetonitrile and 5.0 g anhydrous MgSO4 under shaking for 10 min, and separated by centrifugation for 15 min at 5500 rpm. And then 8 mL of supernatant was collected in a 10 mL centrifuge tube including 60 mg PSA and 250 mg anhydrous MgSO4. Pesticide residues were separated by vortex for 2 min and centrifugation for 5 min at 4500 rpm. The supernatant was evaporated to dryness under a stream of nitrogen and redissolved in 1.0 mL n-hexane for GC analysis or 1.0 mL acetonitrile for HPLC analysis. The extracts were passed through 0.22 μm organic filter membrane before chromatographic analysis.
2.3.2 Microwave digestion. The dry D. candidum sample (0.3000 g) was added into a microwave digestion tank containing HNO3. The samples were soaked in HNO3 overnight at 160 °C for 6 h. After the tank got to room temperature, heat it up to evaporate HNO3 until it was almost dry. Microwave digestion tank was washed by 1% HNO3 solution three times. Then all solutions were fully transferred and fixed to 25 mL which was ready to be tested for ICP-MS.
2.4 Instrumental analysis
2.4.1 Analyses of pesticide residues. An Agilent 6890N GC-ECD equipped with a HP-5 capillary column (30 m × 0.32 mm × 0.25 μm) was used for the determination of pesticide residues from group I. The temperatures of the injection port and detector were 260 °C and 290 °C, respectively. Injection volume was 1 μL with a splitless mode. The GC oven temperature program was as follows: 60 °C for 2 min and then increased by 15 °C min−1 to 150 °C for 1 min. Afterwards temperature increased by 5 °C min−1 to 170 °C for 1 min then by 2 °C min−1 to 210 °C for 1 min. After that GC was heat up to 230 °C for 1 min by 5 °C min−1 and finally to 290 °C by 10 °C min−1 for 5 min.An Agilent 1260 with a diode-array detector (DAD) was used for the determination of pesticide residues from group II. A Waters C18 column (150 mm × 4.6 mm, 5 μm) was served as stationary phase while mobile phase consisted of acetonitrile and pure water (60
:
40, v/v). Injection volume was 10 μL and the flow rate was 1 mL min−1. The GC-MS/MS and HPLC-MS/MS were used to verify the results according to the methods described by Wong et al. 21 and Lozano et al.22
2.4.2 Heavy metal analysis. Thermo X Series II ICP-MS (Thermo Fisher Scientific, USA) was utilized for the determination of heavy metals in this study. Blanks and duplicate samples were analysed for quality control. The optimum instrumental operating conditions for ICP-MS measurements are presented in Table S2.†
2.5 Method validation
The validation of modified QuEChERS method was evaluated by accuracy, precision and sensitivity. The accuracy, expressed by recoveries, was assessed by three replicated blank samples and three fortified pesticide mixture samples at 0.01, 0.1, and 1 mg kg−1. The relative standard deviation (RSD) was served to evaluate the precision, and the sensitivity was assessed by the limit of detection (LOD) and limit of quantification (LOQ).
Similarly, the LOD, LOQ and recoveries of 8 heavy metals and the internal standard based on microwave digestion combined with ICP-MS method were determined to verify whether the method meets the requirements of heavy metal residue analysis.
2.6 Determination of heavy metal content
Content of heavy metal elements in samples was computed by following eqn (1): |
X = ((ρ − ρ0) × V × f)/(m × 1000)
| (1) |
where, X is the content of elements in samples (mg kg−1 or mg L−1), ρ is mass concentration of elements in sample solution (mg L−1), similarly ρ0 is mass concentration of elements in blank solution (mg L−1), V represents volume of digestive juice (mL), f is dilution ratio, and m is the sample quality (g or kg).
2.7 Health risk assessments
2.7.1 Dietary intake risk. The dietary intake risk of each pesticide was calculated as eqn (2) and (3): |
NEDI = (∑(STMRi × Fi))/bw
| (2) |
where, NEDI is the national estimated daily intake (μg per kg per bw per day), STMRi is the standard test of residual values (mg kg−1), Fi is the average food consumption (kg), bw is the average body weight (kg).where, ADI is the acceptable daily intake and RQ is the risk quotient value (the dietary risk is acceptable only when RQ values ≤1).In this study, ADI referred to Chinese Pharmacopoeia Commission (2015)2 and the National Food Safety Standard of China (GB2763-2019).23 According to the investigation and calculation, the daily intake (Fi) of Chinese herbal medicine for adults with a standard weight (bw) of 55.9 kg is 0.000531 kg.22
2.7.2 Target hazard quotient (THQ). The THQ was used to assess the risk of heavy metals ingested by human body through food, using the following eqn (4) according to USEPA:24 |
THQ = (c × ED × EF × IRD)/(bw × AT × RfD)
| (4) |
where, c is mass concentration of elements in sample (mg kg−1), ED is the exposure duration (30 year), EF is the exposure frequency (30 day per year), IRD is the intake rate (12 and 6 g per day for adults and children, respectively),2 bw represents body weight (55.9 and 32.7 kg for adults and children, respectively),25 AT is the average time of exposure under heavy metals (365 day × 70 year) and RfD is the reference oral dose of heavy metals (μg per (kg per day); Cu, 40; As, 0.3; Cd, 1; Cr, 3; Hg, 0.3; Ni, 20; Zn, 300).26,27 The RfD of Pb (40 μg per (kg per day)) was calculated from the weekly tolerable intake limit provided by FAO/WHO.28 The exposure may do harm to human health when THQ values exceed 1. In contrast, when THQ values are less than 1, it is unnecessary to worry about exposure risks.29
2.7.3 Cancer risk (CR). CR indicates the probability of a population developing any type of cancer due to exposure to heavy metals, which is calculated using following eqn (5) according to USEPA:23 |
CR = (c × EF × ED × IRD × CSF)/(bw × AT)
| (5) |
where CSF is the cancer slope factor (1.5 mg per (kg per day) for As and 8.5 × 10−6 mg per (kg per day) for Hg).30 The acceptable lifetime cancer risk range is lower than 10−6.26
3. Results & discussion
3.1 Determination of pesticide residues in D. candidum
The chromatograms of 24 pesticides are shown in Fig. 1a and b, and they had satisfactory separation. The LOD and LOQ of 24 pesticides were 0.005–10 μg kg−1 and 0.011–22 μg kg−1, respectively (Table 1). The mean recoveries of all pesticides by the modified QuEChERS method were 76.9–110.0% as well as the RSD values were 0.28–11.40%, which demonstrated that the analysis method was satisfactory for the requirements of pesticide residues. Moreover, GC-MS/MS and HPLC-MS/MS were used to identify pesticide residues in D. candidum.
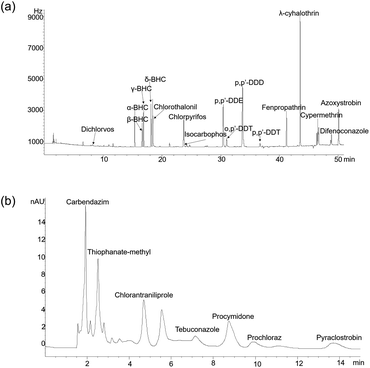 |
| Fig. 1 The chromatograms of 24 pesticides in Dendrobium candidum. (a) GC-ECD chromatogram of 17 pesticides for group I; (b) HPLC-DAD chromatogram of 7 pesticides for group II. | |
Table 1 The LOD, LOQ, recovery, and RSD of 24 pesticides in Dendrobium candidum
Pesticide |
LOD (μg kg−1) |
LOQ (μg kg−1) |
D. candidum-spiked level (mg kg−1) |
0.01 |
0.1 |
1 |
Recovery (%) |
RSD (%) |
Recovery (%) |
RSD (%) |
Recovery (%) |
RSD(%) |
α-BHC |
0.005 |
0.011 |
78.97 |
3.11 |
81.92 |
1.85 |
87.74 |
3.70 |
β-BHC |
0.05 |
0.11 |
82.97 |
8.06 |
84.79 |
6.96 |
86.43 |
2.28 |
γ-BHC |
0.011 |
0.022 |
91.43 |
7.89 |
92.32 |
11.37 |
80.61 |
3.42 |
δ-BHC |
0.005 |
0.011 |
93.91 |
3.48 |
80.62 |
11.40 |
93.88 |
8.95 |
p,p′-DDD |
0.005 |
0.011 |
87.24 |
8.15 |
92.09 |
5.84 |
94.12 |
9.01 |
p,p′-DDE |
0.011 |
0.022 |
87.36 |
2.13 |
89.15 |
10.55 |
91.31 |
8.15 |
o,p′-DDT |
0.011 |
0.022 |
82.50 |
6.67 |
85.25 |
4.83 |
89.37 |
5.43 |
p,p′-DDT |
0.005 |
0.011 |
94.60 |
4.17 |
92.73 |
9.14 |
92.14 |
4.63 |
Azoxystrobin |
0.011 |
0.022 |
85.76 |
7.16 |
109.97 |
4.42 |
104.08 |
6.54 |
Carbendazim |
10 |
22 |
86.24 |
13.09 |
85.11 |
3.55 |
87.05 |
3.31 |
Chlorothalonil |
0.005 |
0.011 |
83.30 |
8.82 |
82.08 |
9.77 |
85.17 |
8.67 |
Chlorpyrifos |
0.005 |
0.011 |
76.87 |
6.93 |
87.39 |
6.07 |
103.49 |
4.61 |
Cypermethrin |
0.011 |
0.022 |
92.27 |
1.09 |
103.58 |
4.74 |
106.24 |
1.04 |
Chlorantraniliprole |
10 |
22 |
85.32 |
5.13 |
83.35 |
9.31 |
91.65 |
7.49 |
Dichlorvos |
10 |
22 |
83.12 |
8.14 |
78.43 |
4.76 |
82.65 |
3.85 |
Difenoconazole |
0.05 |
0.11 |
103.08 |
5.90 |
104.94 |
3.65 |
106.88 |
2.19 |
Fenpropathrin |
0.011 |
0.022 |
77.76 |
2.06 |
89.41 |
5.16 |
97.26 |
0.98 |
Isocarbophos |
0.05 |
0.11 |
77.99 |
9.24 |
78.15 |
7.38 |
99.16 |
7.70 |
Prochloraz |
1 |
2.2 |
78.61 |
3.81 |
87.55 |
8.87 |
96.75 |
3.43 |
Pyraclostrobin |
5 |
11 |
82.10 |
7.70 |
89.22 |
7.47 |
91.24 |
6.58 |
Procymidone |
10 |
22 |
84.58 |
9.70 |
87.19 |
5.80 |
87.30 |
8.55 |
Thiophanate-methyl |
1 |
2.2 |
86.78 |
7.17 |
83.29 |
3.75 |
92.36 |
8.43 |
Tebuconazole |
10 |
22 |
77.28 |
7.56 |
83.14 |
6.30 |
93.60 |
7.36 |
λ-Cyhalothrin |
0.011 |
0.022 |
99.65 |
0.28 |
98.68 |
2.43 |
102.36 |
1.46 |
Fig. 2a and Table S3† showed that 83.33% samples contained at least one pesticide residue. Only 3 samples (SY-1, HQYT and YLB-2) were below the LOD. Five different pesticide residues were detected in the WZTY sample which had the most kinds of pesticides. As shown in Fig. 2b, 7 pesticides in group I and 5 pesticides in group II were detected in all samples, ranging from 0.06 μg kg−1 to 312.83 μg kg−1 (Table S3†). The highest detection frequency of pesticide residues in D. candidum was prochloraz (44.4%), followed by pyraclostrobin (38.89%), azoxystrobin (33.33%), difenoconazole (16.67%), chlorothalonil, chlorpyrifos, chlorantraniliprole, procymidone and carbendazim (11.11%), β-BHC, fenpropathrin and λ-cyhalothrin (5.56%). In general, half of D. candidum samples were exposed to more than one pesticide, and the residual levels of pesticides in all samples varied significantly with their sampling location. In consideration of few standards for pesticide residues in herbal medicine in China, both Ch. P. 2015 (ref. 2) and GB2763-2019 (ref. 21) which stipulates maximum pesticide residues in food and drugs were referred. After comparison, it is found that all of the pesticide residues detected in D. candidum samples were under the maximum residual limits (MRLs) values by Ch. P. 2015 (ref. 2) and GB2763-2019. 21
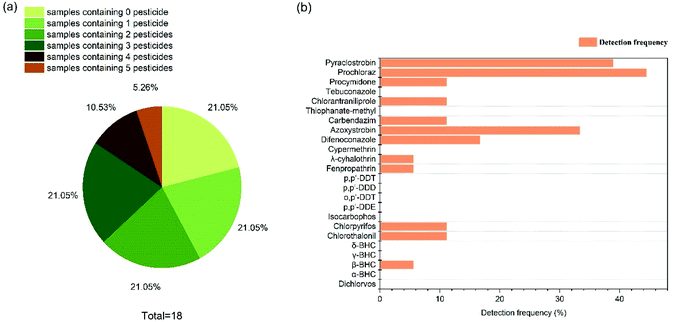 |
| Fig. 2 The residues of 24 pesticides in Dendrobium candidum samples. (a) Proportion of pesticide residues in single Dendrobium candidum sample, 0–5 represents the number of pesticide residue types; (b) detection frequency of 24 pesticide residues in Dendrobium candidum samples. | |
3.2 Residual levels of heavy metals in D. candidum
On the basis of microwave digestion-ICP-MS, the LOD, LOQ, average recoveries, and RSD of 8 heavy metals were 1.0 × 10−4 to 5.0 × 10−2 mg kg−1, 3.0 × 10−4 to 2.0 × 10−1, 82.7–108.1%, and 1.4–8.0%, respectively.
The concentration of 8 heavy metals in D. candidum samples collected from different areas in China is listed in Table 2. 8 heavy metal elements could be detected in all D. candidum samples. The maximum residues detected in D. candidum samples were 5.74 × 10–3 mg kg−1 (Hg), 0.66 mg kg−1 (Cr), 0.40 mg kg−1 (Ni), 6.85 mg kg−1 (Cu),42.66 mg kg−1 (Zn), 0.09 mg kg−1 (As), 0.14 mg kg−1 (Cd) and 0.48 mg kg−1 (Pb), respectively. It can be concluded that the concentration of most heavy metals in samples did not vary greatly except for Zn. As shown in Table 2, the content of Zn fluctuated significantly in different samples, and the maximum level (42.66 mg kg−1) was found in the NB sample but the minimum level (2.99 mg kg−1) was found in the LXX sample, which may be related to the local cultivation environment. Although heavy metals were commonly found in D. candidum samples in this study, all of them did not exceed the MRLs values of Ch. P. 2015.2
Table 2 The concentration of heavy metals in Dendrobium candidum samples
Sample |
Cu (mg kg−1) |
Zn (mg kg−1) |
Cr (mg kg−1) |
Ni (mg kg−1) |
As (mg kg−1) |
Cd (mg kg−1) |
Pb (mg kg−1) |
Hg (mg kg−1) |
YLB |
1.85 |
27.85 |
0.40 |
0.34 |
0.02 |
0.08 |
0.21 |
1.82 × 10−3 |
SY |
6.85 |
31.49 |
0.54 |
0.38 |
0.09 |
0.14 |
0.25 |
5.74 × 10−3 |
NB |
1.40 |
42.66 |
0.37 |
0.21 |
0.02 |
0.04 |
0.18 |
1.64 × 10−3 |
WC |
0.65 |
12.79 |
0.46 |
0.30 |
0.06 |
0.06 |
0.48 |
5.31 × 10−3 |
HQ |
0.45 |
7.11 |
0.26 |
0.17 |
0.01 |
0.03 |
0.03 |
1.97 × 10−3 |
LXX |
0.40 |
2.99 |
0.30 |
0.10 |
0.01 |
0.03 |
0.01 |
1.69 × 10−3 |
SY-1 |
3.72 |
8.41 |
0.25 |
0.15 |
0.03 |
0.04 |
0.02 |
8.91 × 10−4 |
WZTD |
0.53 |
9.28 |
0.21 |
0.15 |
0.01 |
0.02 |
0.02 |
4.13 × 10−4 |
GXD |
0.40 |
4.88 |
0.23 |
0.15 |
0.04 |
0.02 |
0.02 |
8.31 × 10−4 |
WJX-W |
0.52 |
18.31 |
0.24 |
0.13 |
0.01 |
0.02 |
0.06 |
6.19 × 10−4 |
KEB |
0.72 |
5.46 |
0.21 |
0.15 |
0.04 |
0.01 |
0.03 |
8.33 × 10−4 |
XRC |
2.89 |
9.06 |
0.18 |
0.13 |
0.06 |
0.02 |
0.02 |
7.22 × 10−4 |
SXG |
0.50 |
5.30 |
0.19 |
0.13 |
0.01 |
0.02 |
0.06 |
9.18 × 10−4 |
TH |
0.73 |
6.59 |
0.66 |
0.40 |
0.01 |
0.01 |
0.20 |
1.94 × 10−3 |
GXS |
0.18 |
7.22 |
0.23 |
0.15 |
0.02 |
0.01 |
0.08 |
1.12 × 10−3 |
TFTS |
0.62 |
12.52 |
0.28 |
0.16 |
0.01 |
0.02 |
0.17 |
1.70 × 10−3 |
WZTY |
0.45 |
10.02 |
0.27 |
0.14 |
0.01 |
0.02 |
0.11 |
1.70 × 10−3 |
GXL |
0.20 |
6.39 |
0.23 |
0.14 |
0.04 |
0.01 |
0.17 |
2.12 × 10−3 |
TFTH |
0.87 |
13.52 |
0.31 |
0.14 |
0.02 |
0.05 |
0.25 |
2.41 × 10−3 |
H1 |
0.58 |
3.86 |
0.30 |
0.14 |
0.01 |
0.04 |
0.03 |
8.07 × 10−4 |
H6 |
0.60 |
5.76 |
0.32 |
0.19 |
0.01 |
0.07 |
0.04 |
8.88 × 10−4 |
3.3 Health risk assessment
The dietary intake risk of pesticide residues was evaluated according to the eqn (2). The standard median residue test (STMR) was the highest residue detected in D. candidum samples, which was used to calculate risk quotient (RQ). As shown in Table 3, RQ values ranged from 1.88 × 10−8 to 7.25 × 10−5, far less than 1, indicating that pesticide residues in D. candidum were within an acceptable range.
Table 3 The dietary intake risk assessment of pesticide residues in Dendrobium candidum
Pesticide |
MRL (mg kg−1) |
Fi (kg) |
STMR (mg kg−1) |
EDI (mg) |
Bw (kg) |
ADI (mg kg−1 bw) |
RQ |
Chlorpyrifos |
2 |
5.31 × 10−4 |
8.50 × 10−5 |
9.29 × 10−7 |
55.9 |
0.01 |
8.07 × 10−8 |
Fenpropathrin |
5 |
5.31 × 10−4 |
6.00 × 10−5 |
2.40 × 10−7 |
55.9 |
0.03 |
1.90 × 10−8 |
λ-Cyhalothrin |
15 |
5.31 × 10−4 |
2.30 × 10−4 |
2.25 × 10−6 |
55.9 |
0.02 |
1.09 × 10−7 |
Difenoconazole |
10 |
5.31 × 10−4 |
3.40 × 10−4 |
3.65 × 10−7 |
55.9 |
0.01 |
3.23 × 10−7 |
Azoxystrobin |
1 |
5.31 × 10−4 |
3.95 × 10−4 |
4.43 × 10−7 |
55.9 |
0.2 |
1.88 × 10−8 |
β-BHC |
0.2 |
5.31 × 10−4 |
1.10 × 10−4 |
8.03 × 10−7 |
55.9 |
0.005 |
2.09 × 10−7 |
Chlorothalonil |
10 |
5.31 × 10−4 |
2.20 × 10−4 |
5.93 × 10−7 |
55.9 |
0.02 |
1.05 × 10−7 |
Carbendazim |
1 |
5.31 × 10−4 |
3.04 × 10−3 |
1.89 × 10−6 |
55.9 |
0.03 |
9.63 × 10−7 |
Chlorantraniliprole |
0.5 |
5.31 × 10−4 |
1.41 × 10−1 |
1.40 × 10−4 |
55.9 |
2 |
6.69 × 10−7 |
Procymidone |
5 |
5.31 × 10−4 |
1.66 × 10−1 |
1.87 × 10−4 |
55.9 |
0.1 |
1.58 × 10−5 |
Prochloraz |
15 |
5.31 × 10−4 |
7.62 × 10−2 |
1.20 × 10−4 |
55.9 |
0.01 |
7.25 × 10−5 |
Pyrazoxystrobin |
10 |
5.31 × 10−4 |
2.23 × 10−2 |
7.49 × 10−4 |
55.9 |
0.03 |
7.05 × 10−6 |
The health risk of 8 heavy metals was assessed by target hazard quotient (THQ) and carcinogenic risk (CR). The non-carcinogenic health risk was evaluated by mean concentration of heavy metals in D. candidum based on THQ. According to USEPA, there is a threat to human health when THQ exceeds 1.
Moreover, exposure or ingestion of heavy metals may cause liver cancer and skin cancer. Therefore, USEPA stipulated only if CR value for As and Pb were below 10–6, the lifetime cancer risk can be inconsequential. As shown in Table 4, the THQ of single heavy metal for adults and children ranged from 4.33 × 10–5 to 7.76 × 10–4 and 3.70 × 10–5 to 6.64 × 10–4, respectively. Among them, Cr had the highest THQ and Hg had lowest THQ to both adults and children. Meanwhile, by summing the THQ values of each heavy metal, the total THQ values of 8 heavy metals to adults and children were 0.0029 and 0.0025. Both each THQ and the total THQ were all below 1, indicating that exposure to single and combined heavy metals posed a low risk to human health. Furthermore, the CR values of As and Pb were all below 10–6, indicating that the dietary intake of D. candidum had no significant hazard of carcinogenesis.
Table 4 Target hazard quotient (THQ) and cancer risk (CR) for heavy metals
Heavy metal |
Adults |
Children |
THQ |
CR |
THQ |
CR |
Hg |
4.33 × 10−5 |
— |
3.70 × 10−5 |
— |
Cr |
7.76 × 10−4 |
— |
6.64 × 10−4 |
— |
Ni |
7.14 × 10−5 |
— |
6.11 × 10−5 |
— |
Cu |
2.26 × 10−4 |
— |
1.93 × 10−4 |
— |
Zn |
3.02 × 10−4 |
— |
2.58 × 10−4 |
— |
As |
6.55 × 10−4 |
2.95 × 10−7 |
5.60 × 10−4 |
2.52 × 10−7 |
Cd |
2.73 × 10−4 |
— |
2.33 × 10−4 |
— |
Pb |
2.15 × 10−5 |
7.46 × 10−9 |
1.88 × 10−5 |
6.38 × 10−9 |
4. Conclusions
The results obtained in this study showed that a modified QuEChERS-HPLC/GC-MS/MS method was established to efficiently determine 24 pesticide residues in D. candidum from different sites in China. The LOQ was within the range of 0.011–22 μg kg−1. The average recoveries of 24 pesticides spiked into three different blank samples at three levels of 0.01, 0.1, and 1 mg kg−1 were in the range of 76.9–110.0% with RSD of 0.28–11.40%. The detection rate of pesticide residues was 83.33%, and 57.89% of all samples detected two or more pesticides. All RQ values were far less than 1, indicating that these pesticide residues in these D. candidum samples did not pose the potential risk to consumers. Meanwhile, the residual levels of 8 typical heavy metals were also detected by microwave digestion-ICP-MS method and then estimated their potential health risk. Although all 8 heavy metals were detected, the residual amount was relatively low. THQ and CR also suggested that the heavy metals in these D. candidum samples were safe to human. In general, this study provided the simple, efficient, and stable pre-treatment method for the extraction and detection of typical pesticide and heavy metal residues in D. candidum, which may provide a technical support for the pollution monitoring of herbal medicines.
Conflicts of interest
The authors declared that there are no conflicts of interest to this work.
Acknowledgements
This work was supported by the Zhejiang Provincial Key Research and Development Program of China (No. 2018C02034), the Key Program of Natural Science Foundation of Zhejiang Province of China (No. LZ21B070002), and the National Nature Science Foundation of China (No. 41877144 and 42177252).
Notes and references
- J. M. Kong, N. K. Goh, L. S. Chia and T. F. Chia, Acta Pharmacol. Sin., 2003, 24, 7–21 CAS.
- C. P. Commission, Pharmacopoeia of the People's Republic of China, China Medical Science and Technology Press, Beijing, 2015 Search PubMed.
- C. C. Wong, H. B. Li, K. W. Cheng and F. Chen, Food Chem., 2006, 97, 705–711 CrossRef CAS.
- T. B. Ng, J. Liu, J. H. Wong, X. Ye, S. C. W. Sze, Y. Tong and K. Y. Zhang, Appl. Microbiol. Biotechnol., 2012, 93, 1795–1803 CrossRef CAS PubMed.
- Z. Guo, Y. Zhou, J. Yang and X. Shao, Biomed. Pharmacother., 2019, 110, 371–379 CrossRef CAS PubMed.
- J. Cheng, P. P. Dang, Z. Zhao, L. C. Yuan, Z. H. Zhou, D. Wolf and Y. B. Luo, J. Ethnopharmacol., 2019, 229, 81–88 CrossRef PubMed.
- C. Fang, G.-Z. Xin, S.-L. Wang, M.-M. Wei, P. Wu, X.-M. Dong, G.-Q. Song, T. Xie and J.-L. Zhou, J. Pharm. Biomed. Anal., 2020, 182, 113118 CrossRef CAS PubMed.
- X. Cai, X. He, T. Lu, G. Lu and S. Chen, Pharmacol. Clin. Chin. Mater. Med., 2019, 35, 191–196 Search PubMed.
- L. Luo, L. Dong, Q. Huang, S. Ma, P. Fantke, J. Li, J. Jiang, M. Fitzgerald, J. Yang, Z. Jia, J. Zhang, H. Wang, Y. Dai, G. Zhu, Z. Xing, Y. Liang, M. Li, G. Wei, J. Song, J. Wei, C. Peng, H. Zhang, W. Zhang, S. Wang, K. Mizuno, A. A. G. Marco, L. Wu, J. Xu, C. Xiong and S. Chen, Chemosphere, 2021, 262, 127477 CrossRef CAS PubMed.
- J. Jiang, L. Chen, S. Wu, L. Lv, X. Liu, Q. Wang and X. Zhao, Environ. Pollut., 2020, 265, 114844 CrossRef CAS PubMed.
- F. Kamel and J. A. Hoppin, Environ. Health Perspect., 2004, 112, 950–958 CrossRef CAS PubMed.
- L. N. Vandenberg, T. Colborn, T. B. Hayes, J. J. Heindel, D. R. Jacobs Jr, D.-H. Lee, T. Shioda, A. M. Soto, F. S. vom Saal, W. V. Welshons, R. T. Zoeller and J. P. Myers, Endocr. Rev., 2012, 33, 378–455 CrossRef CAS PubMed.
- A. Ahmad and M. Ahmad, Pestic. Biochem. Physiol., 2017, 143, 127–134 CrossRef CAS PubMed.
- A. Bergman, J. Heindel, S. Jobling, K. Kidd and R. T. Zoeller, Toxicol. Lett., 2012, 211, S3 CrossRef.
- L. G. Costa, Handb. Clin. Neurol., 2015, 131, 135–148 Search PubMed.
- P. C. Nagajyoti, K. D. Lee and T. V. M. Sreekanth, Environ. Chem. Lett., 2010, 8, 199–216 CrossRef CAS.
- Y. Chen, J. Zou, H. Sun, J. Qin and J. Yang, Ecotoxicol. Environ. Saf., 2021, 207, 111311 CrossRef CAS PubMed.
- X. Liu, Q. Song, Y. Tang, W. Li, J. Xu, J. Wu, F. Wang and P. C. Brookes, Sci. Total Environ., 2013, 463, 530–540 CrossRef PubMed.
- L. Jarup, Br. Med. Bull., 2003, 68, 167–182 CrossRef PubMed.
- S. Kapaj, H. Peterson, K. Liber and P. Bhattacharya, J. Environ. Sci. Health, Part A: Toxic/Hazard. Subst. Environ. Eng., 2006, 41, 2399–2428 CrossRef CAS PubMed.
- J. W. Wong, K. Zhang, K. Tech, D. G. Hayward, A. J. Krynitsky, I. Cassias, F. J. Schenck, K. Banerjee, S. Dasgupta and D. Brown, J. Agric. Food Chem., 2010, 58, 5884–5896 CrossRef CAS PubMed.
- A. Lozano, L. Rajski, N. Belmonte-Valles, A. Ucles, S. Ucles, M. Mezcua and A. R. Fernandez-Alba, J. Chromatogr. A, 2012, 1268, 109–122 CrossRef CAS PubMed.
- Ministry of Agriculture of the People's Republic of China, 2019, GB2763.
- U.S. Environmental Protection Agency, Risk assessment guidance for superfund, EPA/540/1–89/002, Washington, DC, 1989 Search PubMed.
- K. Y. Ge, The Status of Nutrient and Meal of Chinese in the 1990s, People's Hygiene Press, Beijing, 1992 Search PubMed.
- M. Varol, G. K. Kaya and A. Alp, Sci. Total Environ., 2017, 599, 1288–1296 CrossRef PubMed.
- A. A. Taylor, J. S. Tsuji, M. R. Garry, M. E. McArdle, W. L. Goodfellow, W. J. Adams and C. A. Menzie, Environ. Manage., 2020, 65, 131–159 CrossRef PubMed.
- Food and Agriculture Organization of the World Health Organization, Joint FAO/WHO Food Standards Programme Codex Committee on Contaminants in Foods, The Netherlands, 2011 Search PubMed.
- U.S. Environmental Protection Agency, Guidelines for Carcinogen Risk Assessment, EPA/630/P-03/001F, Washington, DC, 2005 Search PubMed.
- U.S. Environmental Protection Agency, Integrated risk information system, accessed, November, 2020 Search PubMed.
Footnotes |
† Electronic supplementary information (ESI) available. See DOI: 10.1039/d1ra07641h |
‡ Equal contribution. |
|
This journal is © The Royal Society of Chemistry 2022 |
Click here to see how this site uses Cookies. View our privacy policy here.