DOI:
10.1039/D1QO01124C
(Research Article)
Org. Chem. Front., 2022,
9, 19-24
Diastereodivergent synthesis of fully disubstituted spiro[indoline-3,2′-pyrrolidin]-2-ones via tuneable Lewis base/Brønsted base-promoted (3 + 2) cycloadditions†
Received
30th July 2021
, Accepted 26th September 2021
First published on 27th September 2021
Abstract
Herein we report a diastereodivergent synthesis of fully disubstituted spiro[indoline-3,2′-pyrrolidin]-2-ones through base-promoted (3 + 2) cycloadditions. Importantly, the catalysts are found to have full control over the configuration of the stereocenters. When a Lewis base (PCy3) is used as a catalyst, good yields and excellent diastereoselectivities are obtained, regardless of the properties of the substituents, whereas spiro[indoline-3,2′-pyrrolidin]-2-ones of a different diastereoisomer are produced in good yields when a Brønsted base (K2CO3) is used. ESI-MS experiments proved the existence of key zwitterionic intermediates.
Spiro[indoline-3,2′-pyrrolidin]-2-ones are found as core motifs in an array of biologically active natural products, pharmaceuticals, and their analogues.1 Their absolute and relative configurations have been proven to play a key role in the biological activity of spirocyclic compounds. As such, the diastereodivergent synthesis of a target spirocyclic compound not only results in the formation of spirocyclic compounds with varied biological activities but also allows the exploration of configuration–activity relationships.2 Nevertheless, precise access to different diastereomers of the product is incredibly difficult because diastereochemical behaviour is primarily dominated by the inherent structural and stereoelectronic nature of substrates. Most of the strategies currently available rely on using different catalysts, switching of solvents or additives, and using dual catalysis to selectively access complementary diastereomers.3 Despite all this, utilizing two distinct catalysts for the complete diastereoisomeric control of products bearing multiple stereocenters is highly desired but remains an unmet synthetic challenge.4
Lewis base catalysis, which mainly includes tertiary phosphine and amine catalysts, has recently become one of the most powerful methods for the chemoselective synthesis of cyclic and heterocyclic compounds.5 For example, in 2011, Kwon and Guo reported allene-dependent (3 + 2), (3 + 3), (4 + 3), and (3 + 2 + 3) annulations that were carried out by changing the substrates.6 In 2016, Lu and colleagues described phosphine-catalyzed regiodivergent γ-additions wherein C-2- and C-4-selective γ-additions of oxazolones to 2,3-butadienoates were realized by using different phosphine catalysts.7 In 2017, Huang and co-workers achieved the divergent synthesis of hydropyridine derivatives via Lewis base mediated (4 + 2) cyclizations.8 More recently, our group developed phosphine-catalyzed enantioselective (4 + 2) annulation reactions of γ-benzyl allenoates wherein two diastereoisomeric phosphine catalysts functioned as pseudoenantiomers, providing a wide range of 3,3′-spirocyclic oxindoles with opposite absolute configurations.9 Although numerous advances have been made in controlling the chemoselectivity and enantioselectivity of products, the divergent synthesis of two different diastereomers from identical starting materials in the field of Lewis base catalysis remains highly desirable.
Phosphine-catalyzed annulation reactions of electron-deficient olefins with imines offer a very reliable strategy for the construction of valuable heterocycles.10 With regard to the reaction mechanism, the nucleophilic addition of a phosphine catalyst to the electrophilic β-carbon of electron-deficient olefin results in the generation of a zwitterionic intermediate, which subsequent undergoes a Michael addition reaction with imine (Scheme 1A). In 2020, Zhou and co-workers reported a phosphine-catalyzed cascade annulation reaction between aldimine esters and δ-acetoxy allenoates, which involved a different reaction mechanism (Scheme 1B).11 Inspired by this, we envisioned that a change in the reaction pathway might regulate the relative configuration and steric hindrance of the transient state, and the divergent synthesis of two different diastereomers from identical starting materials could thus be realized. Recently, we developed a new conjugated diene derived from β,γ-unsaturated α-ketoester, which produced a new zwitterionic intermediate and might lead to the unpredictable prospect of a new reaction discovery.12 In addition, N-2,2,2-trifluoroethylisatin ketimines have been employed as azomethine ylide precursors undergoing a cycloaddition reaction with electron-deficient alkenes for the construction of spirocyclic oxindole derivatives bearing a CF3 group.13 Herein, we wish to report our research on this subject. This report shows that we are the first to realize the divergent synthesis of two different diastereomers from identical starting materials by applying a different achiral Lewis base (PCy3)/Brønsted base (K2CO3) catalytic system (Scheme 1C).
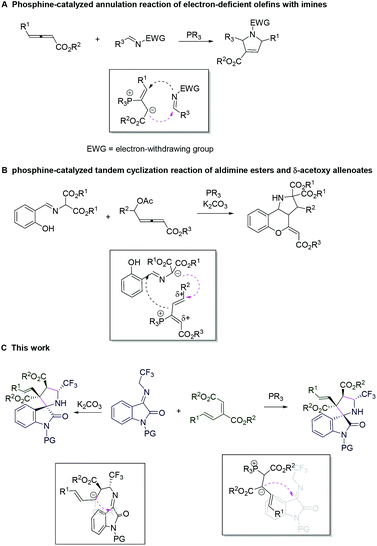 |
| Scheme 1 Strategy for the divergent synthesis of two different diastereomers. | |
We began our study by choosing N-2,2,2-trifluoroethylisatin ketimine 1a with conjugated diene 2a as the model substrates. The screening was initiated by the examination of different phosphine catalysts (Table 1 entries 1–5). The result showed that PCy3 was the optimal catalyst, giving the products endo-3a in 21% yield and exo′-4a in 15% yield, respectively. Subsequently, the influence of the solvent was examined, and acetonitrile showed the best diastereoselectivity (Table 1, entries 6–9). Upon prolonging the reaction time to 60 h, the product was obtained in an increased yield of 59% with excellent diastereoselectivity (Table 1, entry 10). It is noteworthy that the best yield of endo-3a was observed when PCy3 (25 mol%) was used (66% yield; Table 1, entry 11). Tertiary amine catalysts were also examined, and it was found that DBU was a poor catalyst (Table 1, entries 12–14). Interestingly, when Brønsted bases (Cs2CO3 and K2CO3) were used, the diastereoisomer exo′-4a was obtained as the major product (Table 1, entries 15 and 16). When the solvent was changed to CHCl3 in the presence of K2CO3, the diastereoselectivity was fully reversed, producing only the diastereoisomer exo′-4a in 78% yield (Table 1, entry 17). Finally, an improvement in the yield (90%) was obtained when the loading of the Brønsted base was increased to 100 mol% (Table 1, entries 17–19).
Table 1 Optimization of the reaction conditionsa
Having established the optimized reaction conditions, we explored the substrate scope of the phosphine-catalyzed diastereoselective (3 + 2) annulation reaction of N-2,2,2-trifluoroethylisatin ketimines with conjugated dienes, as shown in Scheme 2, and we are the first to do so. First, the conjugated dienes having electron-neutral (endo-3a), electron-withdrawing (endo-3b–endo-3d and endo-3g) or electron-donating (endo-3e and endo-3f) functional groups on the phenyl ring reacted with 1a to generate the desired products endo-3 with up to >20
:
1 diastereoselectivity in 43–66% yields (Scheme 2, endo-3a–endo-3g). Then various N-2,2,2-trifluoroethylisatin ketimines 1, including 5-Me, 5-Cl, 5-Br, 6-Cl, 7-Me, and 5,7-Me2, were examined, providing the corresponding spiro[indoline-3,2′-pyrrolidin]-2-ones 3 in 47–96% yields with >20
:
1 diastereoselectivities (Scheme 2, endo-3h–endo-3m). The conjugated dienes bearing different ester groups reacted smoothly with 1a, giving the corresponding products endo-3 in 64%–71% yields (Scheme 2, endo-3n–endo-3p), but low diastereoselectivities were obtained when R3 = R4 = Et and Bn (Scheme 2, endo-3n and endo-3o). Finally, various N-protection groups were accommodated, leading to good yields and diastereoselectivities (Scheme 2, endo-3q and endo-3r). Interestingly, when N-Ac of 1 was used, the deprotection product endo-3q was obtained in 97% yield (Scheme 2, endo-3q). Furthermore, the relative configuration of endo-3a was determined by X-ray crystallographic analysis of a single crystal of the pure sample.14
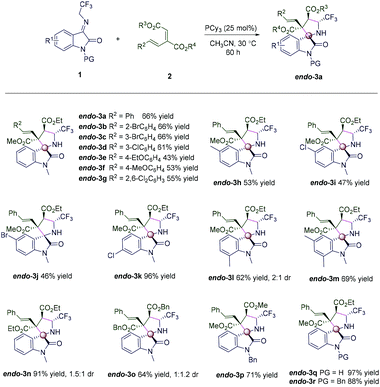 |
| Scheme 2 Reaction scope in the presence of PCy3. Reaction conditions: unless otherwise noted, the reactions were conducted with 1 (0.15 mmol) and 2 (0.23 mmol) in 2.0 mL of CH3CN for 60 h. Isolated yield, dr > 20 : 1; the two isomers could be isolated by flash column chromatography. | |
We then set out to explore the substrate scope in the presence of K2CO3, as shown in Scheme 3. Under the optimized reaction conditions, various N-protection groups (Me, allyl, Ac, and Bn) on N1 of N-2,2,2-trifluoroethylisatin ketimines 1 were examined, leading to good yields and diastereoselectivities (Scheme 3, exo′-4a–exo′-4e). Curiously, the deprotection product exo′-4c was obtained in 59% yield in the presence of N-Ac protection (Scheme 3, exo′-4c). Substrates 2 bearing different ester groups were all tolerated in this transformation, affording their respective products exo′-4f–exo′-4k in moderate to high yields with excellent diastereoselectivities (Scheme 3, exo′-4f–exo′-4k). Besides, the reaction showed good tolerance towards the electronic properties of aromatic substituents; the R2 substituent groups of conjugated dienes with electron-withdrawing or electron-donating groups worked well, giving the desired products exo′-4l–exo′-4t in moderate to high yields with excellent diastereoselectivities (Scheme 3, exo′-4l–exo′-4t). A substrate bearing a strong electron-withdrawing group (2,6-Cl2) formed the product exo′-4u with excellent diastereoselectivity but with a modest yield (Scheme 3, exo′-4u). To further explore the scope, substituted N-2,2,2-trifluoroethylisatin ketimines 1 (including 5,7-Me2, 5-Cl, 4-Cl, and 7-Me) were also used, and we were pleased to obtain products exo′-4v–exo′-4x bearing vicinal quaternary stereogenic carbon centers in 46–65% yields with >20
:
1 dr (Scheme 3, exo′-4v–exo′-4x). The relative configuration of exo′-4a was determined by X-ray crystallographic analysis of a single crystal of the pure sample.15
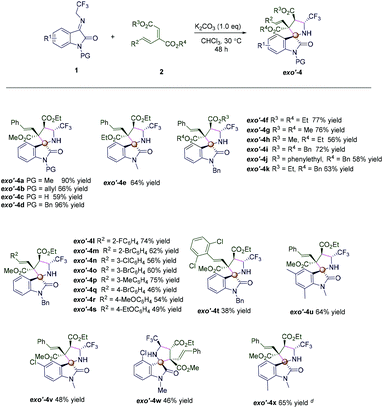 |
| Scheme 3 Reaction scope in the presence of K2CO3. Reaction conditions: unless otherwise noted, the reactions were conducted with 1 (0.15 mmol) and 2 (0.23 mmol) in 2.0 mL of CHCl3 for 48 h. Isolated yield, dr > 20 : 1; the two isomers could be isolated by flash column chromatography. cK2CO3 (40 mol%). | |
In order to demonstrate the practicality of our method, we performed the reaction on a large scale. When N-2,2,2-trifluoroethylisatin ketimine 1a and conjugated diene 2a were used in the presence of PCy3 under the optimal reaction conditions, the reaction proceeded smoothly to afford the desired adduct endo-3a in 66% yield. When a Brønsted base (K2CO3) was used, 64% yield of the product exo′-4a was obtained under the optimal reaction conditions (Scheme 4).
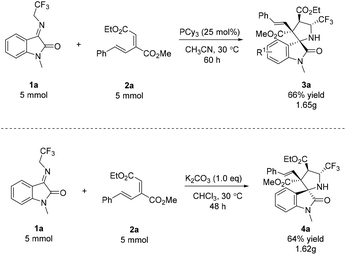 |
| Scheme 4 Large scale preparation. | |
Based on the above experimental observations and on literature precedents,16 we propose a plausible mechanism for the reaction, as shown in Scheme 5. When PBu3 was used as the catalyst, initially, the nucleophilic addition of the phosphine catalyst to conjugated diene 2a afforded zwitterionic intermediate A. The subsequent stereospecific nucleophilic addition of intermediate A to N-2,2,2-trifluoroethylisatin ketimine 1a resulted in the formation of intermediate C, which was detected by HRMS analysis (see the ESI, Fig. S1†). Next, an SN2 substitution reaction occurred, forming the desired product endo-3a and regenerating the phosphine catalyst. When K2CO3 was used as the Brønsted base, intermediate E was the first to be obtained; the Michael addition of intermediate E to conjugated diene 2a then occurred to give intermediate F. Subsequently, the stereospecific ring closure and protonation afforded the corresponding product exo′-4a (Scheme 5).
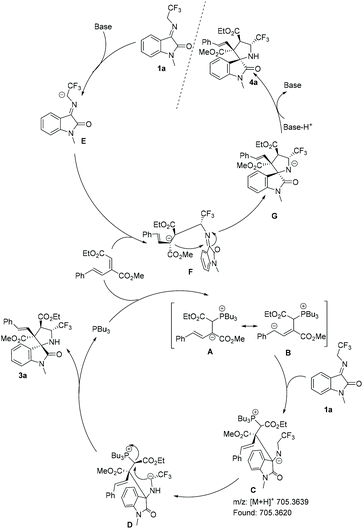 |
| Scheme 5 Plausible reaction mechanism. | |
In summary, we have developed unique base-promoted diastereoselective (3 + 2) cycloadditions. A diastereodivergent synthesis of fully disubstituted spiro[indoline-3,2′-pyrrolidin]-2-ones was realized by tuning a Lewis base (PCy3)/Brønsted base (K2CO3). The reaction achieved near perfect diastereodivergence in most cases. Significantly, ESI-MS studies were carried out to present a full picture of the reaction processes, and a plausible reaction mechanism is proposed. Further exploration of this diastereodivergent strategy for the construction of biologically valuable molecules is currently underway in our laboratory and will be reported in due course.
Author contributions
K. Li, Z. Zhang, J. Zhu, and Y. Wang performed the experiments. J. Zhao performed the HRMS analysis. E.-Q. and Zheng conceived and directed the project and wrote the paper. All authors discussed the results and commented on the manuscript.
Conflicts of interest
There are no conflicts to declare.
Acknowledgements
We are grateful to the National Natural Science Foundation of China (21702189, 21672193, and 21272218), the Key Scientific and Technological Project of Henan Province (202102310004), the China Ministry of Industry and Information Technology (Z135060009002), and Zhengzhou University (JC21253007) of China for financial support of this research.
Notes and references
- For selected examples, see:
(a) J. W. H. Li and J. C. Vederas, Drug Discovery and Natural Products: End of an Era or an Endless Frontier?, Science, 2009, 325, 161–165 CrossRef;
(b) A. L. Harvey, Natural products in drug discovery, Drug Discovery Today, 2008, 13, 894–901 CrossRef CAS;
(c) C. V. Galliford and K. A. Scheidt, Pyrrolidinyl-Spirooxindole Natural Products as Inspirations for the Development of Potential Therapeutic Agents, Angew. Chem., Int. Ed., 2007, 46, 8748–8758 CrossRef CAS;
(d) K. Ding, Y. Lu, Z. Nikolovska-Coleska, G. Wang, S. Qiu, S. Shangary, W. Gao, D. Qin, J. Stuckey, K. Krajewski, P. P. Roller and S. Wang, Structure-Based Design of Spiro-oxindoles as Potent, Specific Small-Molecule Inhibitors of the MDM2-p53 Interaction, J. Med. Chem., 2006, 49, 3432–3435 CrossRef CAS;
(e) C. Marti and E. M. Carreira, Construction of Spiro[pyrrolidine-3,3′-oxindoles]-Recent Applications to the Synthesis of Oxindole Alkaloids, Eur. J. Org. Chem., 2003, 2209–2219 CrossRef CAS;
(f) C. B. Cui, H. Kakeya and H. Osada, Novel mammalian cell cycle inhibitors, spirotryprostatins A and B, produced by Aspergillus fumigatus, which inhibit mammalian cell cycle at G2/M phase, Tetrahedron, 1996, 52, 12651–12666 CrossRef CAS;
(g) B. Gu, S. Wu, H. Xu, W. Yang, Z. Liu and W. Deng, Organocatalytic asymmetric [3+3] annulation of isatin N,N’-cyclic azomethine imines with enals: Efficient approach to functionalized spiro N-heterocyclic oxindoles, Chin. Chem. Lett., 2021, 32, 672–675 CrossRef CAS.
- X. Huo, J. Zhang, J. Fu, R. He and W. Zhang, Ir/Cu Dual Catalysis: Enantio- and Diastereodivergent Access to α,α-Disubstituted α-Amino Acids Bearing Vicinal Stereocenters, J. Am. Chem. Soc., 2018, 140, 2080–2084 CrossRef CAS PubMed.
-
(a) Q. Cheng, F. Zhang, Y. Cai, Y.-L. Guo and S.-L. You, Stereodivergent Synthesis of Tetrahydrofuroindoles through Pd-Catalyzed Asymmetric Dearomative Formal (3+2) Cycloaddition, Angew. Chem., Int. Ed., 2018, 57, 2134–2138 CrossRef CAS;
(b) F. A. Cruz and V. M. Dong, Stereodivergent Coupling of Aldehydes and Alkynes via Synergistic Catalysis Using Rh and Jacobsen's Amine, J. Am. Chem. Soc., 2017, 139, 1029–1103 CrossRef CAS PubMed;
(c) X. Jiang, J. J. Beiger and J. F. Hartwig, Stereodivergent Allylic Substitutions with Aryl Acetic Acid Esters by Synergistic Iridium and Lewis Base Catalysis, J. Am. Chem. Soc., 2017, 139, 87–90 CrossRef CAS;
(d) D. Uraguchi, K. Yoshioka and T. Ooi, Complete diastereodivergence in asymmetric 1,6-addition reactions enabled by minimal modification of a chiral catalyst, Nat. Commun., 2017, 8, 14793–14805 CrossRef;
(e) X. Huo, R. He, X. Zhang and W. Zhang, An Ir/Zn Dual Catalysis for Enantio- and Diastereodivergent α-Allylation of α-Hydroxyketones, J. Am. Chem. Soc., 2016, 138, 11093–11096 CrossRef CAS PubMed;
(f) S.-L. Shi, Z. L. Wong and S. L. Buchwald, Copper-catalysed enantioselective stereodivergent synthesis of amino alcohols, Nature, 2016, 532, 353–356 CrossRef CAS;
(g) S. Krautwald, D. Sarlah, M. A. Schafroth and E. M. Carreira, Enantio- and Diastereodivergent Dual Catalysis: α-Allylation of Branched Aldehydes, Science, 2013, 340, 1065–1068 CrossRef CAS;
(h) A. Nojiri, N. Kumagai and M. Shibasaki, Linking Structural Dynamics and Functional Diversity in Asymmetric Catalysis, J. Am. Chem. Soc., 2009, 131, 3779–3784 CrossRef CAS PubMed.
-
(a) I. P. Beletskaya, C. Nájera and M. Yus, Stereodivergent Catalysis, Chem. Rev., 2018, 118, 5080–5200 CrossRef CAS PubMed;
(b) G. Zhan, W. Du and Y.-C. Chen, Switchable divergent asymmetric synthesis via organocatalysis, Chem. Soc. Rev., 2017, 46, 1675–1692 RSC;
(c) F. Cheng, S. J. Kalita, Z.-N. Zhao, X. Yang, Y. Zhao, U. Schneider, N. Shibata and Y.-Y. Huang, Diastereodivergent asymmetric 1,3-dipolar cycloaddition of azomethine ylides and β-fluoroalkyl vinylsulfones: low copper(II) catalyst loading and theoretical studies, Angew. Chem., Int. Ed., 2019, 58, 16637–16643 CrossRef CAS;
(d) S. J. Kalita, F. Cheng, Q.-H. Fan, N. Shibata and Y.-Y. Huang, Diastereodivergent synthesis of chiral 4-fluoropyrrolidines (exo and exo′) based on the Cu(II)-catalyzed asymmetric 1,3-dipolar cycloaddition, J. Org. Chem., 2021, 86, 8695–8705 CrossRef CAS;
(e) M.-S. Mei, Y.-J. Wang, G.-S. Zhang, J.-Y. Tang, P. Tian and Y.-H. Wang, Catalyst-Controlled Diastereoselectivity Switch in the Asymmetric [3 + 2] Annulation of Isatin-Derived MBH Carbonates and 5–Alkenylthiazol-4(5H)–ones, Org. Lett., 2021 DOI:10.1021/acs.orglett.1c02421;
(f) G. Zhan, M.-L. Shi, Q. He, W.-J. Lin, Q. Ouyang, W. Du and Y.-C. Chen, Catalyst-controlled switch in chemo- and diastereoselectivities: annulations of Morita–Baylis–Hillman carbonates from isatins, Angew. Chem., Int. Ed., 2016, 55, 2147–2151 CrossRef CAS.
- Selected reviews:
(a) C. Xie, A. J. Smaligo, X.-R. Song and O. Kwon, Phosphorus-Based Catalysis, ACS Cent. Sci., 2021, 7, 536–558 CrossRef CAS PubMed;
(b) L. Wu, B. Yu and E.-Q. Li, Recent Advances in Organocatalyst-Mediated Benzannulation Reactions, Adv. Synth. Catal., 2020, 362, 4010–4026 CrossRef CAS;
(c) E.-Q. Li and Y. Huang, Recent advances in phosphine catalysis involving γ-substituted allenoates, Chem. Commun., 2020, 56, 680–694 RSC;
(d) H. Guo, Y. Fan, Z. Sun, Y. Wu and O. Kwon, Phosphine Organocatalysis., Chem. Rev., 2018, 118, 10049–10293 CrossRef CAS;
(e) Y. Wei and M. Shi, Applications of Chiral Phosphine-Based Organocatalysts in Catalytic Asymmetric Reactions, Chem. – Asian J., 2014, 9, 2720–2734 CrossRef CAS;
(f) Y. C. Fan and O. Kwon, Advances in nucleophilic phosphine catalysis of alkenes, allenes, alkynes, and MBHADs, Chem. Commun., 2013, 49, 11588–11619 RSC;
(g) B. J. Cowen and S. J. Miller, Enantioselective catalysis and complexity generation from allenoates, Chem. Soc. Rev., 2009, 38, 3102–3116 RSC;
(h) L.-W. Ye, J. Zhou and Y. Tang, Phosphine-triggered synthesis of functionalized cyclic compounds, Chem. Soc. Rev., 2008, 37, 1140–1152 RSC;
(i) X. Lu, C. Zhang and Z. Xu, Reactions of Electron-Deficient Alkynes and Allenes under Phosphine Catalysis, Acc. Chem. Res., 2001, 34, 535–544 CrossRef CAS PubMed.
- R. Na, C. Jing, Q. Xu, H. Jiang, X. Wu, J. Shi, J. Zhong, M. Wang, D. Benitez, E. Tkatchouk, W. A. Goddard, H. Guo and O. Kwon, Phosphine-Catalyzed Annulations of Azomethine Imines: Allene-Dependent (3+2), (3+3), (4+3), and (3+2+3) Pathways, J. Am. Chem. Soc., 2011, 133, 13337–13348 CrossRef CAS PubMed.
- T. Wang, Z. Yu, D. L. Hoon, C. Y. Phee, Y. Lan and Y. Lu, Regiodivergent Enantioselective γ-Additions of Oxazolones to 2,3-Butadienoates Catalyzed by Phosphines: Synthesis of α,α-Disubstituted α-Amino Acids and N,O-Acetal Derivatives, J. Am. Chem. Soc., 2016, 138, 265–271 CrossRef CAS PubMed.
- H. Jin, E. Li and Y. Huang, Divergent synthesis of hydropyridine derivatives via nitrogen-containing Lewis base mediated regioselective (4+2) cyclizations, Org. Chem. Front., 2017, 4, 2216–2220 RSC.
- S. Jia, M. Ma, E.-Q. Li, Z. Duan and F. Mathey, Design of 1-Phosphanorbornene Derivatives as Chiral Organocatalysts for Enantioselective (4+2) Annulation Reactions of γ-Benzyl Allenoates, Org. Lett., 2021, 23, 3337–3342 CrossRef CAS PubMed.
- Selected recent examples:
(a) X. Ren, H. Wu, M. Zhang, W. Zhao and G. Huang, Origins of catalyst-controlled enantiodivergent hydroamination of enones with pyridazinones: A computational study, Chin. Chem. Lett., 2021 DOI:10.1016/j.cclet.2021.02.049;
(b) N. Li and Y. Huang, Phosphine-Catalyzed Sequential (3+3)/Aza-6π-Electrocyclization Reaction of Cross-Conjugated Azatrienes and δ-Sulfonamido-Allenoates, Org. Lett., 2020, 22, 9392–9397 CrossRef CAS;
(c) J. Feng, Y. Chen, W. Qin and Y. Huang, Phosphine-Catalyzed (3+2)/(3+2) Sequential Annulation of γ-Vinyl Allenoates: Access to Fused Carbocycles, Org. Lett., 2020, 22, 433–437 CrossRef CAS PubMed;
(d) H. Qiu, X. Chen and J. Zhang, Design, synthesis and application of a new type of bifunctional Le-Phos in highly enantioselective γ-addition reactions of N-centered nucleophiles to allenoates, Chem. Sci., 2019, 10, 10510–10515 RSC;
(e) M. Wu, Z. Han, K. Li, J. Wu, K. Ding and Y. Lu, Cyclohexyl-Fused, Spirobiindane-Derived, Phosphine-Catalyzed Synthesis of Tricyclic γ-Lactams and Kinetic Resolution of γ-Substituted Allenoates, J. Am. Chem. Soc., 2019, 141, 16362–16373 CrossRef CAS;
(f) X. He, Y. Tang, Y. Wang, J.-B. Chen, S. Xu, J. Dou and Y. Li, Phosphine-Catalyzed Activation of Alkylidenecyclopropanes: Rearrangement to Form Polysubstituted Furans and Dienones, Angew. Chem., Int. Ed., 2019, 58, 10698–10702 CrossRef CAS PubMed;
(g) H. Ni, X. Tang, W. Zheng, W. Yao, N. Ullah and Y. Lu, Enantioselective Phosphine-Catalyzed Formal (4+4) Annulation of α,β-Unsaturated Imines and Allene Ketones: Construction of Eight-Membered Rings, Angew. Chem., Int. Ed., 2017, 56, 14222–14226 CrossRef CAS;
(h) X.-Y. Wu, H.-Z. Gui, H. Jangra, Y. Wei, H. Zipse and M. Shi, Phosphine-catalyzed (3+2) annulation of 2-aminoacrylates with allenoates and mechanistic studies, Catal. Sci. Technol., 2020, 10, 3959–3964 RSC;
(i) Y.-N. Gao and M. Shi, Phosphine-mediated enantioselective synthesis of carbocycles and heterocycles, Chin. Chem. Lett., 2017, 28, 493–502 CrossRef CAS.
- Z. Dai, J. Zhu, W. Su, W. Zeng, Z. Liu, M. Chenand and Q. Zhou, Phosphine-Catalyzed Stereoselective Tandem Annulation Reaction for the Synthesis of Chromeno[4,3-b]pyrroles, Org. Lett., 2020, 22, 7008–7012 CrossRef CAS PubMed.
-
(a) K. Li, Z. Gan, E.-Q. Li and Z. Duan, Phosphine-Catalyzed (4+2) Cycloaddition of Conjugated Dienes with Enones and Its Asymmetric Variant, Org. Lett., 2021, 23, 3094–3099 CrossRef CAS PubMed;
(b) Z. Gan, Y. Gong, Y. Chu, E.-Q. Li, Y. Huang and Z. Duan, Phosphine-catalyzed regiodivergent annulations of γ-substituted allenoates with conjugated dienes, Chem. Commun., 2019, 55, 10120–10123 RSC.
- Selected examples:
(a) G. Zhang, A. Yu, Y. Lei, X. Meng and L. Zhang, Synthesis of novel pyridinium 1,5-zwitterions and their reactivity with isatin-based α-(trifluoromethyl)imines: a sulfur-controlled domino reaction, Org. Chem. Front., 2021, 8, 3718–3723 RSC;
(b) C. Zou, Y. Han, C. Zeng, T. Y. Zhang, J. Ye and G. Song, Remote regioselective organocatalytic asymmetric (3+2) cycloaddition of N-2,2,2-trifluoroethyl isatin ketimines with cyclic 2,4-dienones, Chin. Chem. Lett., 2020, 31, 377–380 CrossRef CAS;
(c) B. Niu, X.-Y. Wu, Y. Wei and M. Shi, Palladium-Catalyzed Diastereoselective Formal (5+3) Cycloaddition for the Construction of Spirooxindoles Fused with an Eight-Membered Ring, Org. Lett., 2019, 21, 4859–4863 CrossRef CAS PubMed;
(d) Y. You, W.-Y. Lu, Z.-H. Wang, Y.-Z. Chen, X.-Y. Xu, X.-M. Zhang and W.-C. Yuan, Organocatalytic Asymmetric (3+2) Cycloaddition of N-2,2,2-Trifluoroethylisatin Ketimines with β-Trifluoromethyl Electron-Deficient Alkenes: Access to Vicinally Bis(trifluoromethyl)-Substituted 3,2′-Pyrrolidinyl Spirooxindoles, Org. Lett., 2018, 20, 4453–4457 CrossRef CAS PubMed.
- CCDC (3a) 2057244† contains the supplementary crystallographic data for this paper.
- CCDC (4a) 2050077† contains the supplementary crystallographic data for this paper.
-
(a) Y. Wang, M. Song, E.-Q. Li and Z. Duan, Auto-tandem palladium/phosphine cooperative catalysis: synthesis of bicyclo[3.1.0]hexenes by selective activation of Morita-Baylis-Hillman carbonates, Org. Chem. Front., 2021, 8, 3366–3371 RSC;
(b) Y. Wang, S. Jia, E.-Q. Li and Z. Duan, Phosphine/Palladium Cooperative Catalysis: (4+3) Annulations of Morita–Baylis–Hillman Carbonates and Vinyl Benzoxazinanones, J. Org. Chem., 2019, 84, 15323–15330 CrossRef CAS PubMed;
(c) S. Li, H. Tang, Y. Wang, Y. Zhu, D.-C. Fang, D. Wei and M. Tang, A DFT study on the competing mechanisms of PPh3-catalyzed (3+3) and (3+2) annulations between 5-acetoxypenta-2,3-dienoate and 1C,3O-bisnucleophiles, J. Mol. Catal. A: Chem., 2015, 407, 137–146 CrossRef CAS;
(d) Y. Liang, S. Liu, Y. Xia, Y. Li and Z.-X. Yu, Mechanism, Regioselectivity, and the Kinetics of Phosphine-Catalyzed [3+2] Cycloaddition Reactions of Allenoates and Electron-Deficient Alkenes, Chem. – Eur. J., 2008, 14, 4361–4373 CrossRef CAS;
(e) E. Mercier, B. Fonovic, C. Henry, O. Kwon and T. Dudding, Phosphine triggered (3+2) allenoate–acrylate annulation: a mechanistic enlightenment, Tetrahedron Lett., 2007, 48, 3617–3620 CrossRef CAS;
(f) Y. Xia, Y. Liang, Y. Chen, M. Wang, L. Jiao, F. Huang, S. Liu, Y. Li and Z.-X. Yu, An Unexpected Role of a Trace Amount of Water in Catalyzing Proton Transfer in Phosphine-Catalyzed (3+2) Cycloaddition of Allenoates and Alkenes, J. Am. Chem. Soc., 2007, 129, 3470–3471 CrossRef CAS;
(g) X.-F. Zhu, C. E. Henry and O. Kwon, Stable Tetravalent Phosphonium Enolate Zwitterions, J. Am. Chem. Soc., 2007, 129, 6722–6723 CrossRef CAS PubMed.
Footnotes |
† Electronic supplementary information (ESI) available. CCDC 2057244 and 2050077. For ESI and crystallographic data in CIF or other electronic format see DOI: 10.1039/d1qo01124c |
‡ These authors contributed equally to this work. |
|
This journal is © the Partner Organisations 2022 |