DOI:
10.1039/D1QO01087E
(Research Article)
Org. Chem. Front., 2022,
9, 25-31
Visible light-promoted aerobic oxidative cleavage and cyclization of olefins to access 3-hydroxy-isoindolinones†
Received
24th July 2021
, Accepted 24th September 2021
First published on 29th September 2021
Abstract
A convenient and environmentally friendly synthetic route from 2-vinylbenzimide to 3-hydroxy-isoindolinones through visible light-promoted transformations via iron/disulfide catalysis and molecular oxygen oxidation has been developed. A range of 3-hydroxy-isoindolinones was obtained in moderate to good yields, which exhibit excellent functional group compatibility and broad substrate scope. Further mechanistic investigations proved that dioxetane might be a key intermediate being involved in the reaction.
Introduction
Isoindolinone derivatives, a class of potential biological and pharmacologically active molecules, serve as critical structural motifs in natural products and many drugs.1 The structural units of isoindolinones are widely present in bioactive molecules, such as fumadensine which is an alkaloidal separated from rhizomes of Fumaria densiflora, thalidomide (Contergan®) that acts as a therapeutic drug for painful inflammations associated with leprosy, CRR-271, a synthetic compound which was confirmed as a PARP-1 inhibitor, etc. (Fig. 1).2 In addition, the isoindolinone skeleton could also represent intermediates in organic synthesis.3 This means that various novel synthesis strategies of isoindolinones have emerged as being of continuing interest with vigorous development and industrialization.4
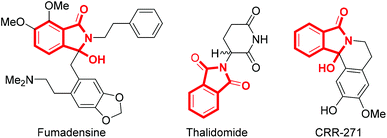 |
| Fig. 1 Natural product and pharmacological molecules with the isoindolinone nucleus. | |
In fact, the most common synthetic strategy is still selective reduction of phthalimide.5 However, the application of strong reducing agents (NaBH4, B2H6 and LiAlH4) and sub-zero temperature limits the functional group tolerance and causes potential safety hazards, significant amounts of waste, etc.6 Recently, transition metal-catalyzed cross-coupling reactions have been employed to synthesize isoindolinone derivatives by forming C–C bonds in cyclization/annulation processes (Scheme 1a).7 In 2018, Zhang's group reported a method involving carbonylation of benzylamine with carbon monoxide to provide the target product (Scheme 1b).8 In 2009, Kanazawa and co-workers proposed a feasible strategy of intramolecular cyclization of o-alkynylbenzamide derivatives (Scheme 1c).9 Unfortunately, all of these methodologies somehow require excessive expensive metals, strong oxidants, high temperature, and toxic raw materials, which might pollute the environment and contaminate the final products. Obviously, it is necessary to develop an environmentally friendly, safe-to-operate and low-cost approach to form isoindolinones.
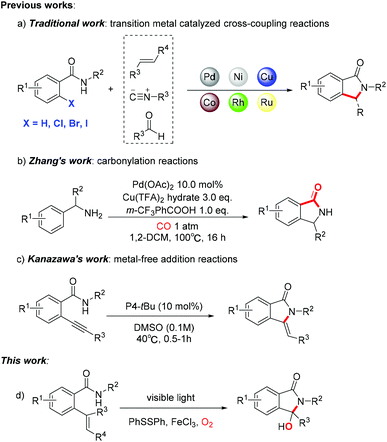 |
| Scheme 1 A wide variety of synthetic strategies for isoindolinones. | |
The oxidative cleavage of olefins (OCO) is an effective transformation, since it can be used to synthesize oxygen-containing groups such as ketones and aldehydes.10 Recently, the process of OCO which is promoted by irradiation of visible light has been reported.10b,c,11 The method adopted cheap and readily available thiophenol or disulfide as catalyst instead of other expensive photo-catalysts, and phenylthio radical could play an important role in selective attack on olefins. Simultaneously, a feasible route is iron-catalyzed oxidation with molecular oxygen, but this suffers from low chemo- and regio-selectivity, low efficiency, and poor functional-group tolerance.11,12 Encouraged by these previous studies, a mild strategy of synthesizing 3-hydroxy-isoindolinones through the synergistic catalysis of diphenyl disulfide and iron salt has been designed. This strategy features mild conditions, high efficiency and environmental benignity, and visible light-promoted aerobic oxidative cleavage and cyclization of olefins reaction has not been reported until now. This usage of low-energy visible light to activate the olefin reaction is more appealing. And this protocol provides a simple and general way for preparing 3-hydroxy-isoindolinones via synergistic catalysis with diphenyl disulfide and iron salt.
Results and discussion
Initially, our investigation began with N-tosyl-2-vinylbenzamide 1a as the standard substrate to optimize the conditions (Table 1). Under blue LED irradiation, the reaction was examined for 10 h at room temperature without any catalysts. Gratifyingly, the target product 3-hydroxy-2-tosylisoindolin-1-one 2a was obtained in 41% yield (Table 1, entry 1). Next, the experiments were carried out with a range of solvents and different volume ratios, and the results indicated that co-solvents of CH3CN/CH3OH (5/1 v/v) was a good choice (Table 1, entries 2–6). A series of different iron sources were loaded into the system because vinyl oxidative cleavage can be promoted by iron salts. The results showed that ferric chloride could improve the efficiency of the reaction (Table 1, entries 7–10). Then, the amount of ferric chloride was investigated, the results indicating that either decreasing or increasing the amount would reduce the yield of product (Table 1, entries 11 and 12). Similarly, adjusting the amount of diphenyl disulfide could influence the performance, and led to a significant reduction in yield (Table 1, entries 13–16). What surprised us was that the product 2a was still detected when the reaction was conducted without diphenyl disulfide (Table 1, entry 13). Due to the strategy whereby iron could trap oxygen species, vinyl would be selectively oxidized under an iron-containing environment.12 Moreover, the reaction failed in the dark during the exploration of the effect of different light sources (Table 1, entry 17). Compared with blue light, exposure to white and green light gave the target product 3a at an extremely low conversion rate (Table 1, entries 18 and 19). Finally, experiments were conducted under nitrogen or oxygen atmosphere (Table 1, entries 20 and 21). And the yield of 3-hydroxy-isoindolinones was increased under an oxygen atmosphere, while the reaction was inhibited completely in a nitrogen atmosphere.
Table 1 Optimization of reaction conditionsa
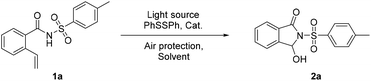
|
Entry |
Light source |
PhSSPh (equiv.) |
Cat. (equiv.) |
Solvent |
Yieldb (%) |
Reaction conditions: N-tosyl-2-vinylbenzamide 1a (0.3 mmol), light source, PhSSPh, Cat., solvent (3 mL), 10 h, room temperature.
Isolated yield.
Under nitrogen atmosphere.
Under oxygen atmosphere.
|
1 |
Blue |
0.5 |
— |
CH3CN |
41 |
2 |
Blue |
0.5 |
— |
CH3OH |
37 |
3 |
Blue |
0.5 |
— |
1,4-Dioxane |
24 |
4 |
Blue |
0.5 |
— |
CH3CN/CH3OH (5/1) |
44 |
5 |
Blue |
0.5 |
— |
CH3CN/CH3OH (5/1) |
49 |
6 |
Blue |
0.5 |
— |
CH3CN/CH3OH (5/1) |
45 |
7 |
Blue |
0.5 |
FeCl2 (0.1) |
CH3CN/CH3OH (5/1) |
63 |
8 |
Blue |
0.5 |
FeCl3 (0.1) |
CH3CN/CH3OH (5/1) |
68 |
9 |
Blue |
0.5 |
Fe(OAc)3 (0.1) |
CH3CN/CH3OH (5/1) |
26 |
10 |
Blue |
0.5 |
Ferrocene (0.1) |
CH3CN/CH3OH (5/1) |
34 |
11 |
Blue |
0.5 |
FeCl3 (0.05) |
CH3CN/CH3OH (5/1) |
58 |
12 |
Blue |
0.5 |
FeCl3 (0.2) |
CH3CN/CH3OH (5/1) |
44 |
13 |
Blue |
— |
FeCl3 (0.1) |
CH3CN/CH3OH (5/1) |
31 |
14 |
Blue |
0.1 |
FeCl3 (0.1) |
CH3CN/CH3OH (5/1) |
43 |
15 |
Blue |
0.2 |
FeCl3 (0.1) |
CH3CN/CH3OH (5/1) |
47 |
16 |
Blue |
1.0 |
FeCl3 (0.1) |
CH3CN/CH3OH (5/1) |
50 |
17 |
— |
0.5 |
FeCl3 (0.1) |
CH3CN/CH3OH (5/1) |
Trace |
18 |
White |
0.5 |
FeCl3 (0.1) |
CH3CN/CH3OH (5/1) |
10 |
19 |
Green |
0.5 |
FeCl3 (0.1) |
CH3CN/CH3OH (5/1) |
Trace |
20 |
Blue |
0.5 |
FeCl3 (0.1) |
CH3CN/CH3OH (5/1) |
Tracec |
21
|
Blue
|
0.5
|
FeCl
3
(0.1)
|
CH
3
CN/CH
3
OH (5/1)
|
75
|
With the optimal reaction conditions in hand, we investigated the scope of this visible light-promoted oxidative cleavage reaction of 2-vinylbenzamides followed by cyclization (Table 2). Firstly, for the substrate scope, 2-vinylbenzamides with different ortho-, meta-, or para-substituted benzenesulfonamides bearing electron-donating groups (Me, t-Bu, MeO) or electron-withdrawing groups (OCF3, CF3, F, Cl, Br, CN, NO2) could be stably transformed into corresponding products in 65–88% yields under standard reaction conditions (Table 2, 2a–2o). And the molecular structure of 2n was identified by single-crystal X-ray diffraction (CCDC 2088807, Fig. S1, see the ESI†). To our delight, substrates with N-mesyl or N-ethanesulfonyl groups also reacted smoothly to give the desired products in good yields of 71% and 76%, respectively (Table 2, 2p, 2q). Moreover, substrates with N-tosyl-2-vinylbenzamide with different types and positions of substituents of R2 groups successfully generated the desired products in good yields from 67% to 86% (Table 2, 2r–2w).
Table 2 Substrate scope of visible light-promoted oxidative cleavage reaction of N-sulfonamide-2-vinylbenzamides followed by cyclizationa,b
Reaction conditions: O2, blue LED (426 nm, 30 W), 1 (0.3 mmol, 1.0 eq.), PhSSPh (0.15 mmol, 0.5 eq.), FeCl3 (0.03 mmol, 0.1 eq.), MeCN/MeOH (3 mL, 5/1 v/v), room temperature, 10 h, pressure cylindrical.
Isolated yield.
|
|
Subsequently, the scope of substituents on the N atom under standard conditions was investigated. The results showed that N-alkoxy-2-vinylbenzamide (MeO, i-PrO, t-BuO, BnO) were successfully applied to this reaction system and provided corresponding products in moderate to good yields (Table 3, 2x–2aa). Meanwhile, there is no desired product detected while amide without any substituents (Table 3, 2ab). The substrates of methylamine and aniline showed poor tolerance under the optimized conditions after reacting for 15 h, and the raw materials were reacted incompletely when the reaction lasted 10 h (Table 3, 2ac and 2ad). N-Tosylbenzamide with 2-(1-propenyl) or 2-(1-phenylvinyl) could also afford the targeted compounds in the reaction system (Table 3, 2ae and 2af). And compared with 2-(1-propenyl), 2-(1-phenylvinyl)-N-tosylbenzamide possesses a large steric hindrance and inhibits the process of cyclization.13 As expected, both 1ag and 1ah were also found to be more efficient oxidative cleavage compounds, and the desired product 2a was isolated in 84% and 77% yield, respectively.
Table 3 Substrate scope of visible light-promoted oxidative cleavage reaction of olefinic amides with different substituentsa,b
Reaction conditions: O2, blue LED (426 nm, 30 W), 1 (0.3 mmol, 1.0 eq.), PhSSPh (0.15 mmol, 0.5 eq.), FeCl3 (0.03 mmol, 0.1 eq.), MeCN/MeOH (3 mL, 5/1 v/v), room temperature, 10 h, pressure cylindrical.
Isolated yield.
Response time 15 h.
|
|
In order to evaluate the practical applicability of the visible-light-promoted oxidative cleavage, a 5 mmol scale-up reaction between 1a and 1,2-diphenyldisulfane was completed (Scheme 2). The reaction indicated that N-tosyl-2-vinylbenzamide performed well under standard conditions in the flask, and the product 2a was obtained in 66% yield. The results showed that this green and environmentally friendly photo-promoted strategy could have great potential industrial value.
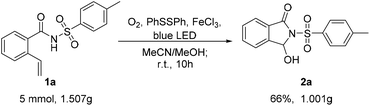 |
| Scheme 2 Gram-scale synthesis of 1a. | |
Finally, several control experiments were implemented to gain a better understanding of the mechanism of this strategy (Scheme 3). When substrate 1a was treated with different gas atmospheres, such as air, N2 and O2, desired product 2a was detected in both air and O2, but not in N2 atmosphere, which implied that air or O2 was necessary for this reaction system (Scheme 3a). Next, we found that the reaction yield was only 48% when carried out without adding ferric chloride, but the substrate was converted completely. While target product was detected with a 31% yield, and the conversion rate of substrate was 31% only (Scheme 3b). These results showed that the mechanism of the reaction may involve two different routes: one is metal photo-catalysis, and the other is disulfide catalysis. However, the two kinds of routes were obviously inhibited by adding 3 equivalents of radical scavenger 2,2,6,6-tetramethyl-1-piperidinyloxy (TEMPO) into the system. A trace amount of the product was obtained when loading 3 equivalents of superoxide radical quencher p-benzoquinone in the reaction, similarly (Scheme 3c). When 1,1-diphenylethylene was added into this reaction system, the reaction was suppressed and two different intermediates 3a and 4a were captured by high-resolution mass spectroscopy (Scheme 3d; Fig. S2 and S3, see the ESI†). These phenomena suggested that the reaction proceeds through a free radical pathway, and superoxide radicals might be the main reactive oxygen species. Then, 2-(1,2-dihydroxyethyl)-N-tosylbenzamide 6a was observed via high-resolution mass spectroscopy when methionine (1 equiv.) and water (1 mL) were added into the reaction system as the trapping reagents for dioxetane under the standard conditions,10b,c,14 which indicated that extremely unstable dioxetane 3a might be a key intermediate (Scheme 3e; Fig. S4, see the ESI†). There is no reaction when N-tosylbenzamide 1ai reacts with benzaldehyde under standard conditions, and product 7ai was detected by high-resolution mass spectroscopy while 1ai reacts with styrene under the same conditions (Scheme 3f; Fig. S5, see the ESI†). These results showed that aldehyde might not be the mid-product and the process involved the cleavage of dioxetane 5a and amino cyclization simultaneously.
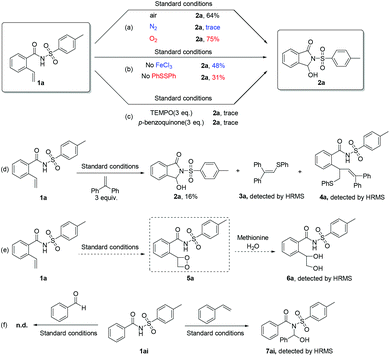 |
| Scheme 3 Several control experiments. | |
Based on the above experimental results and previous reports,10–12,15 a plausible mechanism for visible light-promoted OCO followed by cyclization is shown in Scheme 4. Initially, two molecules of phenyl sulfide radicals A are provided by diphenyl disulfide being irradiated by visible light. Next, free radical B is readily produced through radical A attacking C
C bond with high regioselectivity. Besides, superoxide radical O2˙− attacks free radical B to afford intermediate C with catalysis by iron and visible light. And, dioxetane D is the key intermediate, which is obtained by the abstraction and substitution of the thiyl radical, as with other OCO processes.10b,c,11 Then, intermediate F is gained through the fracture of dioxetane immediately with imine nucleophilic attack of dioxetane. Ultimately, target product 2a could be obtained by hydrogen proton rearrangement. In addition, there might be two different routes worked by ferric chloride or diphenyl disulfide independently (for details, see Scheme S1 in the ESI†).
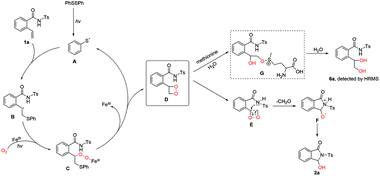 |
| Scheme 4 Plausible reaction mechanism. | |
Conclusions
In summary, a mild and visible-light-mediated strategy to prepare 3-hydroxy-isoindolinones through aerobic oxidative cleavage and cyclization of olefins has been developed. The reaction employed molecular oxygen as oxidant, and ferric chloride and diphenyl disulfide as synergistic catalysts. In addition, the good substrate compatibility and the achievement of gram-scale reaction demonstrate potential industrial application. Control experiments point out that dioxetane might be the critical intermediate, and related experiments of further research are ongoing in our laboratory.
Conflicts of interest
There are no conflicts to declare.
Acknowledgements
The research was supported by the National Natural Science Foundation of China (grant no. 21776130, 21878145 and 22078150).
Notes and references
-
(a) J. Wrobel, A. Dietrich, S. A. Woolson, J. Millen, M. McCaleb, M. C. Harrison, T. C. Hohman, J. Sredy and D. Sullivan, Novel Spirosuccinimides with Incorporated Isoindolone and Benzisothiazole 1,1-Dioxide Moieties as Aldose Reductase Inhibitors and Antihyperglycemic Agents, J. Med. Chem., 1992, 35, 4613 CrossRef CAS;
(b) I. R. Hardcastle, J. Liu, E. Valeur, A. Watson, S. U. Ahmed, T. J. Blackburn, K. Bennaceur, W. Clegg, C. Drummond, J. A. Endicott, B. T. Golding, R. J. Griffin, J. Gruber, K. Haggerty, R. W. Harrington, C. Hutton, S. Kemp, X. Lu, J. M. McDonnell, D. R. Newell, M. E. Noble, S. L. Payne, C. H. Revill, C. Riedinger, Q. Xu and J. Lunec, Isoindolinone inhibitors of the murine double minute 2 (MDM2)-p53 protein-protein interaction: structure-activity studies leading to improved potency, J. Med. Chem., 2011, 54, 1233 CrossRef CAS;
(c) K. Speck and T. Magauer, The chemistry of isoindole natural products, Beilstein J. Org. Chem., 2013, 9, 2048 CrossRef;
(d) Z. Q. Yang, Y. Shu, L. Ma, M. Wittmann, W. J. Ray, M. A. Seager, K. A. Koeplinger, C. D. Thompson, G. D. Hartman, M. T. Bilodeau and S. D. Kuduk, Discovery of naphthyl-fused 5-membered lactams as a new class of m1 positive allosteric modulators, ACS Med. Chem. Lett., 2014, 5, 604 CrossRef CAS PubMed.
-
(a) M. H. Abu Zarga, S. S. Sabri, S. Firdous and M. Shamma, Fumadensine a phthalideisoquinoline from Fumaria densiflora, Phytochemistry, 1987, 26, 1233–1234 CrossRef CAS;
(b) T. Ito, H. Ando, T. Suzuki, T. Ogura, K. Hotta, Y. Imamura, Y. Yamaguchi and H. Handa, Identification of a Primary Target of Thalidomide Teratogenicity, Science, 2010, 327, 1345 CrossRef CAS PubMed;
(c) I. Takahashi, T. Kawakami, E. Hirano, H. Yokota and H. Kitajima, Novel Phthalimidine Synthesis. Mannich Condensation of o-Phthalaldehyde with Primary Amines using 1,2,3-1H-Benzotriazole and 2-Mercaptoethanol as Dual Synthetic Auxiliaries, Synlett, 1996, 353 CrossRef CAS;
(d) U. C. Yoon, Y. X. Jin, S. W. Oh, C. H. Park, J. H. Park, C. F. Campana, X. Cai, E. N. Duesler and P. S. Mariano, A Synthetic Strategy for the Preparation of Cyclic Peptide Mimetics Based on SET-Promoted Photocyclization Processes, J. Am. Chem. Soc., 2003, 125, 10664 CrossRef CAS;
(e) D. Augner, O. Krut, N. Slavov, D. C. Gerbino, H. G. Sahl, J. Benting, C. F. Nising, S. Hillebrand, M. Kronke and H. G. Schmalz, On the antibiotic and antifungal activity of pestalone, pestalachloride A, and structurally related compounds, J. Nat. Prod., 2013, 76, 1519 CrossRef CAS PubMed;
(f) I. R. Hardcastle, S. U. Ahmed, H. Atkins, A. H. Calvert, N. J. Curtin, G. Farnie, B. T. Golding, R. J. Griffin, S. Guyenne, C. Hutton, P. Kallblad, S. J. Kemp, M. S. Kitching, D. R. Newell, S. Norbedo, J. S. Northen, R. J. Reid, K. Saravanan, H. M. Willems and J. Lunec, Isoindolinone-based inhibitors of the MDM2-p53 protein-protein interaction, Bioorg. Med. Chem. Lett., 2005, 15, 1515 CrossRef CAS PubMed;
(g) I. K. Lee, S. E. Kim, J. H. Yeom, D. W. Ki, M. S. Lee, J. G. Song, Y. S. Kim, S. J. Seok and B. S. Yun, Daldinan A, a novel isoindolinone antioxidant from the ascomycete Daldinia concentrica, J. Antibiot., 2012, 65, 95 CrossRef CAS;
(h) M. C. Mavroudi and C. P. Kapnissi-Christodoulou, Evaluation of amino acid ester-based ionic liquids as buffer additives in CE for the separation of 2-arylpropionic acids nonsteroidal anti-inflammatory drugs, Electrophoresis, 2014, 35, 2573 CrossRef CAS;
(i) A. Suyavaran, C. Ramamurthy, R. Mareeswaran, Y. V. Shanthi, J. Selvakumar, S. Mangalaraj, M. S. Kumar, C. R. Ramanathan and C. Thirunavukkarasu, Synthesis and biological evaluation of isoindoloisoquinolinone, pyroloisoquinolinone and benzoquinazolinone derivatives as poly(ADP-ribose) polymerase-1 inhibitors, Bioorg. Med. Chem., 2015, 23, 488–498 CrossRef CAS PubMed.
-
(a) A. J. Basson and M. G. McLaughlin, Synthesis of Functionalized Isoindolinones via Calcium Catalyzed Generation and Trapping of N-Acyliminium Ions, J. Org. Chem., 2020, 85, 5615 CrossRef CAS;
(b) S. Hu, L. Yuan, H. Yan and Z. Li, Design, synthesis and biological evaluation of Lenalidomide derivatives as tumor angiogenesis inhibitor, Bioorg. Med. Chem. Lett., 2017, 27, 4075 CrossRef CAS;
(c) P. C. Patil, F. A. Luzzio and J. M. Ronnebaum, Selective alkylation/oxidation of N-substituted isoindolinone derivatives: synthesis of N-phthaloylated natural and unnatural alpha-amino acid analogues, Tetrahedron Lett., 2017, 58, 3730 CrossRef CAS PubMed;
(d) A. Suneja, R. A. Unhale and V. K. Singh, Enantioselective Hydrophosphonylation of in Situ Generated N-Acyl Ketimines Catalyzed by BINOL-Derived Phosphoric Acid, Org. Lett., 2017, 19, 476 CrossRef CAS;
(e) F. Yagishita, H. Ishikawa, T. Onuki, S. Hachiya, T. Mino and M. Sakamoto, Total spontaneous resolution by deracemization of isoindolinones, Angew. Chem., Int. Ed., 2012, 51, 13023 CrossRef CAS;
(f) R. K. Bhatia, Isoindole Derivatives: Propitious Anticancer Structural Motifs, Curr. Top. Med. Chem., 2017, 17, 189 CrossRef CAS.
-
(a) C. Min, Y. Lin and D. Seidel, Catalytic Enantioselective Synthesis of Mariline A and Related Isoindolinones via a Biomimetic Approach, Angew. Chem., Int. Ed., 2017, 56, 15353 CrossRef CAS PubMed;
(b) R. Savela and C. Mendez-Galvez, Isoindolinone Synthesis via One-Pot Type Transition Metal Catalyzed C-C Bond Forming Reactions, Chemistry, 2021, 27, 5344 CrossRef CAS;
(c) N. Topolovcan and M. Gredicak, Synthesis and stereoselective catalytic transformations of 3-hydroxyisoindolinones, Org. Biomol. Chem., 2021, 19, 4637 RSC;
(d) M. C. Reddy and M. Jeganmohan, Ruthenium-catalyzed cyclization of aromatic nitriles with alkenes: stereoselective synthesis of (Z)-3-methyleneisoindolin-1-ones, Org. Lett., 2014, 16, 4866–4869 CrossRef CAS PubMed;
(e) M. C. Reddy and M. Jeganmohan, Total synthesis of aristolactam alkaloids via synergistic C-H bond activation and dehydro-Diels-Alder reactions, Chem. Sci., 2017, 8, 4130–4135 RSC.
-
(a) Z.-I. Horii, C. Iwata and Y. Tamura, Reduction of Phthalimides with Sodium Borohydride, J. Org. Chem., 1961, 26, 2273 CrossRef CAS;
(b) K. Matoba and T. Yamazaki, Reduction of O-Alkylated Imides, Chem. Pharm. Bull., 1974, 22, 2999 CrossRef CAS;
(c) E.-C. Wang, H.-F. Chen, P.-K. Feng, Y.-L. Lin and M.-K. Hsu, A new synthesis of 3-alkyl-1-isoindolinones, Tetrahedron Lett., 2002, 43, 9163 CrossRef CAS.
-
(a) M. H. Norman, D. J. Minick and G. C. Rigdon, Effect of Linking Bridge Modifications on the Antipsychotic Profile of Some Phthalimide and Isoindolinone Derivatives, J. Med. Chem., 1996, 39, 149 CrossRef CAS PubMed;
(b) A. Arizpe, F. J. Sayago, A. I. Jiménez, M. Ordóñez and C. Cativiela, Synthesis of Phosphoproline Derivatives with an Octahydroisoindole Structure, Eur. J. Org. Chem., 2011, 6732 CrossRef CAS;
(c) J. R. Cabrero-Antonino, R. Adam, V. Papa, M. Holsten, K. Junge and M. Beller, Unprecedented selective homogeneous cobalt-catalysed reductive alkoxylation of cyclic imides under mild conditions, Chem. Sci., 2017, 8, 5536 RSC;
(d) J. G. Pierce, D. L. Waller and P. Wipf, Synthesis of Functionalized Isoindolinones: Addition of In Situ Generated Organoalanes to Acyliminium Ions, J. Organomet. Chem., 2007, 692, 4618 CrossRef CAS PubMed;
(e) K. Xu, S. Zhang, Y. Hu, Z. Zha and Z. Wang, Asymmetric Michael reaction catalyzed by proline lithium salt: efficient synthesis of L-proline and isoindoloisoquinolinone derivatives, Chem. – Eur. J., 2013, 19, 3573 CrossRef CAS.
-
(a) J. W. Wrigglesworth, B. Cox, G. C. Lloyd-Jones and K. I. Booker-Milburn, New heteroannulation reactions of N-alkoxybenzamides by Pd(II) catalyzed C-H activation, Org. Lett., 2011, 13, 5326 CrossRef CAS PubMed;
(b) W. Hao, J. Tian, W. Li, R. Shi, Z. Huang and A. Lei, Nickel-Catalyzed Oxidative C-H/N-H Isocyanide Insertion: An Efficient Synthesis of Iminoisoindolinone Derivatives, Chem. – Asian J., 2016, 11, 1664 CrossRef CAS PubMed;
(c) T. K. Hyster, K. E. Ruhl and T. Rovis, A coupling of benzamides and donor/acceptor diazo compounds to form gamma-lactams via Rh(III)-catalyzed C-H activation, J. Am. Chem. Soc., 2013, 135, 5364 CrossRef CAS PubMed;
(d) R. Manoharan and M. Jeganmohan, Synthesis of isoindolinones via a ruthenium-catalyzed cyclization of N-substituted benzamides with allylic alcohols, Chem. Commun., 2015, 51, 2929 RSC;
(e) F. W. Patureau, T. Besset and F. Glorius, Rhodium-catalyzed oxidative olefination of C-H bonds in acetophenones and benzamides, Angew. Chem., Int. Ed., 2011, 50, 1064 CrossRef CAS;
(f) K. Takamatsu, K. Hirano and M. Miura, Copper-Catalyzed Formal [4 + 1] Cycloaddition of Benzamides and Isonitriles via Directed C-H Cleavage, Org. Lett., 2015, 17, 4066 CrossRef CAS;
(g) X. Wu, B. Wang, S. Zhou, Y. Zhou and H. Liu, Ruthenium-Catalyzed Redox-Neutral [4 + 1] Annulation of Benzamides and Propargyl Alcohols via C-H Bond Activation, ACS Catal., 2017, 7, 2494 CrossRef CAS;
(h) S. W. Youn, T. Y. Ko, Y. H. Kim and Y. A. Kim, Pd(II)/Cu(II)-Catalyzed Regio-and Stereoselective Synthesis of (E)-3-Arylmethyleneisoindolin-1-ones Using Air as the Terminal Oxidant, Org. Lett., 2018, 20, 7869 CrossRef CAS PubMed;
(i) Q. Yu, N. Zhang, J. Huang, S. Lu, Y. Zhu, X. Yu and K. Zhao, Efficient synthesis of hydroxyl isoindolones by a Pd-mediated C-H activation/annulation reaction, Chem. – Eur. J., 2013, 19, 11184 CrossRef CAS;
(j) H. Zhao, X. Shao, Z. Qing, T. Wang, X. Chen, H. Yang and H. Zhai, Cobalt-Catalyzed 2-(1-Methylhydrazinyl)pyridine-Assisted Direct C-H/N-H Functionalization of Benzoyl Hydrazide with Isocyanide: Efficient Synthesis of Iminoisoindolinone Derivatives, Adv. Synth. Catal., 2019, 361, 1678 CrossRef CAS;
(k) X. X. Zheng, C. Du, X. M. Zhao, X. Zhu, J. F. Suo, X. Q. Hao, J. L. Niu and M. P. Song, Ni(II)-Catalyzed C(sp(2))-H Alkynylation/Annulation with Terminal Alkynes under an Oxygen Atmosphere: A One-Pot Approach to 3-Methyleneisoindolin-1-one, J. Org. Chem., 2016, 81, 4002 CrossRef CAS PubMed;
(l) X. Zhou, H. Xu, Q. Yang, H. Chen, S. Wang and H. Zhao, Co(ii)/Cu(ii)-cocatalyzed oxidative C-H/N-H functionalization of benzamides with ketones: a facile route to isoindolin-1-ones, Chem. Commun., 2019, 55, 8603 RSC.
- C. Zhang, Y. Ding, Y. Gao, S. Li and G. Li, Palladium-Catalyzed Direct C–H Carbonylation of Free Primary Benzylamines: A Synthesis of Benzolactams, Org. Lett., 2018, 20, 2595 CrossRef CAS.
- C. Kanazawa and M. Terada, Dichotomous control of E/Z-geometry in intramolecular cyclization of o-alkynylbenzamide derivatives catalyzed by organic superbase P4-tBu in the presence/absence of water, Chem. – Asian J., 2009, 4, 1668 CrossRef CAS PubMed.
-
(a) A. Rajagopalan, M. Lara and W. Kroutil, Oxidative Alkene Cleavage by Chemical and Enzymatic Methods, Adv. Synth. Catal., 2013, 355, 3321 CrossRef CAS;
(b) Y. Deng, X. J. Wei, H. Wang, Y. Sun, T. Noël and X. Wang, Disulfide-Catalyzed Visible-Light-Mediated Oxidative Cleavage of C=C Bonds and Evidence of an Olefin-Disulfide Charge-Transfer Complex, Angew. Chem., Int. Ed., 2016, 56, 832 CrossRef;
(c) X. Wang, Y. Li and Z. Li, Thiol-initiated photocatalytic oxidative cleavage of the C=C bond in olefins and its extension to direct production of acetals from olefins, Catal. Sci. Technol., 2021, 11, 1000 RSC;
(d) J. P. Wan, Y. Gao and L. Wei, Recent Advances in Transition-Metal-Free Oxygenation of Alkene C=C Double Bonds for Carbonyl Generation, Chem. – Asian J., 2016, 11, 2092 CrossRef CAS;
(e) A. Itoh, A. Fujiya, A. Kariya, T. Nobuta, N. Tada and T. Miura, Photooxidative Cleavage of Aromatic Alkenes into Aldehydes Using Catalytic Iodine and Molecular Oxygen under Visible Light Irradiation, Synlett, 2014, 25, 884 CrossRef.
- B. Xiong, X. Zeng, S. Geng, S. Chen, Y. He and Z. Feng, Thiyl radical promoted chemo- and regioselective oxidation of C=C bonds using molecular oxygen via iron catalysis, Green Chem., 2018, 20, 4521 RSC.
-
(a) M. J. Rak, M. Lerro and A. Moores, Hollow iron oxide nanoshells are active and selective catalysts for the partial oxidation of styrene with molecular oxygen, Chem. Commun., 2014, 50, 12482 RSC;
(b) X. Huang and J. T. Groves, Oxygen Activation and Radical Transformations in Heme Proteins and Metalloporphyrins, Chem. Rev., 2018, 118, 2491 CrossRef CAS;
(c) H. Hong, L. Hu, M. Li, J. Zheng, X. Sun, X. Lu, X. Cao, J. Lu and H. Gu, Preparation of Pt@Fe2O3 nanowires and their catalysis of selective oxidation of olefins and alcohols, Chem. – Eur. J., 2011, 17, 8726 CrossRef CAS PubMed;
(d) X. Zhou and H. Ji, Highly Efficient Oxidative Cleavage of Carbon-Carbon Double Bond overmeso-Tetraphenyl Cobalt Porphyrin Catalyst in the Presence of Molecular Oxygen, Chin. J. Chem., 2012, 30, 2103 CrossRef CAS;
(e) U. R. Pillai, E. Sahle-Demessie, V. V. Namboodiri and R. S. Varma, An efficient and ecofriendly oxidation of alkenes using iron nitrate and molecular oxygen, Green Chem., 2002, 4, 495 RSC;
(f) A. Gonzalez-de-Castro and J. Xiao, Green and Efficient: Iron-Catalyzed Selective Oxidation of Olefins to Carbonyls with O2, J. Am. Chem. Soc., 2015, 137, 8206 CrossRef CAS.
-
(a) J.-M. Barbe, F. Burdet, E. Espinosa, C. P. Gros and R. Guilard, New insights into the synthesis of porphyrin-corrole and biscorrole systems, J. Porphyrins Phthalocyanines, 2012, 07, 365 CrossRef;
(b) X. Zhang, Y. Liu, G. Chen, G. Pei and S. Bi, Theoretical Insight into C(sp3)-F Bond Activations and Origins of Chemo- and Regioselectivities of “Tunable” Nickel-Mediated/-Catalyzed Couplings of 2-Trifluoromethyl-1-alkenes with Alkynes, Organometallics, 2017, 36, 3739 CrossRef CAS.
- W. Adam, S. G. Bosio, N. J. Turro and B. T. Wolff, Enecarbamates as selective substrates in oxidations: chiral-auxiliary-controlled mode selectivity and diastereoselectivity in the [2 + 2] cycloaddition and ene reaction of singlet oxygen and in the epoxidation by DMD and m-CPBA, J. Org. Chem., 2004, 69, 1704 CrossRef CAS PubMed.
-
(a) D. H. R. Barton and B. M. Chabot, The selective functionalization of saturated hydrocarbons. Part 33. Further reactions which take place in the FeII-FeIV manifold, Tetrahedron, 1996, 52, 10287 CrossRef CAS;
(b) J. Hua, M. Bian, T. Ma, M. Yang, W. He, Z. Yang, C. Liu, Z. Fang and K. Guo, The sunlight-promoted aerobic selective cyclization of olefinic amides and diselenides, Catal. Sci. Technol., 2021, 11, 2299 RSC;
(c) Y. Nosaka and A. Y. Nosaka, Generation and Detection of Reactive Oxygen Species in Photocatalysis, Chem. Rev., 2017, 117, 11302 CrossRef CAS PubMed;
(d) S. Fukuzumi and K. Ohkubo, Selective photocatalytic reactions with organic photocatalysts, Chem. Sci., 2013, 4, 561 RSC;
(e) L. B. Zhang, Z. C. Wang, S. Z. Sun, S. F. Ni, L. R. Wen and M. Li, Metal-Free Catalyzed Cyclization of N-Methoxybenzamides to Construct Quaternary Carbon-Containing Isoindolinones, Chin. J. Chem., 2021, 39, 903 CrossRef CAS;
(f) H. Vosooghian and M. H. Habibi, Photooxidation of Some Organic Sulfides under UV Light Irradiation Using Titanium Dioxide Photocatalyst, Int. J. Photoenergy, 2007, 2007, 1 CrossRef;
(g) C. Zhang, J. McClure and C. J. Chou, Silver-catalyzed direct thiolation of quinones by activation of aryl disulfides to synthesize quinonyl aryl thioethers, J. Org. Chem., 2015, 80, 4919 CrossRef CAS PubMed;
(h) I. Arslan-Alaton, G. Tureli and T. Olmez-Hanci, Treatment of azo dye production wastewaters using Photo-Fenton-like advanced oxidation processes: Optimization by response surface methodology, J. Photochem. Photobiol., A, 2009, 202, 142 CrossRef CAS;
(i) Y. Chen, A. J. Spiering, S. Karthikeyan, G. W. Peters, E. W. Meijer and R. P. Sijbesma, Mechanically induced chemiluminescence from polymers incorporating a 1,2-dioxetane unit in the main chain, Nat. Chem., 2012, 4, 559 CrossRef CAS PubMed;
(j) R. M. O'Connor and A. Greer, How Tryptophan Oxidation Arises by “Dark” Photoreactions from Chemiexcited Triplet Acetone, Photochem. Photobiol., 2021, 97, 456 CrossRef.
Footnote |
† Electronic supplementary information (ESI) available. CCDC 2088807. For ESI and crystallographic data in CIF or other electronic format see DOI: 10.1039/d1qo01087e |
|
This journal is © the Partner Organisations 2022 |