DOI:
10.1039/D1QI01014J
(Research Article)
Inorg. Chem. Front., 2022,
9, 78-82
Controlled assembly and reversible transformation of tuneable luminescent Mo8-R6G hybrids†
Received
10th August 2021
, Accepted 29th October 2021
First published on 29th October 2021
Abstract
Fluorescent dyes such as rhodamine R6G usually suffer from quenching caused by aggregation in the solid state. Herein, we enhance the solid state luminescence of R6G by controlling assemblies of rhodamine R6G and [Mo8O26]4− based on the synergies between the anchoring and diluting effects. In addition, the hybrids represent reversible transformation and tunable luminescence by different loadings of the rhodamine 6G dye within the [Mo8O26]4− architecture. This work provides new ideas for the structural design, synthesis and transformation of POM-based hybrid materials, opening the way towards the design of novel multifunctional hybrid systems.
Introduction
Fluorescence is critical to applications in optical materials including OLEDs and photonics.1–4 Fluorescence is also readily seen in many classes of dye molecules that display bright emissions in dilute solutions, which has motivated their widespread use as biolabels;5,6 however, the electronic coupling between fluorescent dyes in the solid state quenches their emission, preventing their reliable translation to applications. Despite the growing advances in crystal engineering, it remains almost impossible to predict the packing, coupling, and the resulting optical properties of fluorescent dyes in materials. Thus, the challenge of transferring the optical properties from high-performance dyes to programmed materials with superior optical properties requires predictable and controllable strategies to circumvent electronic coupling and emission quenching. New strategies have emerged to design high-performance solid-state emitters by favorably directing the Aggregation-Induced Emission/Aggregation-Caused Quenching (AIE/ACQ) balance with the use of noncovalent interactions. Many strategies to circumvent quenching rely on fluorophore isolation.7 Installation of bulky substituents on fluorophores is one potential approach to isolation but it demands time-consuming modifications of each and every dye.8 Recent efforts to address structural ordering of molecular building blocks rely on the principles of self-assembly. Tang et al. recently reported that anion-π+ interactions between cationic AIE oxazoliums and classical PF6− counter-ions acting as spacers efficiently reduce the detrimental ACQ effect.9 To go further in favorably directing the AIE/ACQ balance in these molecular systems, the use of highly nucleophilic anions able to develop strong H-bonding interactions with luminogens could be envisioned to strengthen their rigidity and hence to exalt the AIE effect.
Polyoxometalates (POMs), a class of negatively charged inorganic metal oxygen clusters, have broad application prospects in the fields of catalytics,10,11 medicine,12 optics,13 electricity14 and magnetism.15 In fact, POMs have great potential in supramolecular self-assembly materials as subcomponents owing to their wide range of compositions, structures (cage,16,17 sphere,18 ring,19 wheel20 shape, etc.) and charge distributions.21 Therein, cationic dyes form well-defined lattices by co-crystallization with the wide-band-gap nano-sized anion receptors called POMs to turn-on emission and transfer the optical properties from solutions to solids. However, the study of POM hybrids functionalized by organic dyes is still in its infancy. In 2012, Peng et al. reported fluorescent microtubes based on a Keggin tungstosilicate and fluorescein (SiW12-F), which presented tunable photoluminescence from sky blue to green to red by varying excitation light.22 But they did not obtain the exact molecular structure of the luminescent materials, so it is difficult to understand the mechanism of the luminescence properties of the materials. In 2018, Dessapt et al. reported two new supramolecular fluorescent hybrid materials based on POM [M6O19]2− (M = Mo, W) and the AIE active organic molecule 1-methyl-1,2,3,4,5-pentaphenyl-phospholium.23 Herein, we focused on the self-assembly of the hybrid of ACQ organic molecules (rhodamine R6G dye) and POM clusters ([Mo8O26]4−), which have not been reported yet. The self-assembly strategy has tackled the long-standing constraints of quenching the emission of R6G in the solid state, by preparing assemblies of rhodamine R6G and [Mo8O26]4−. Two hybrids with various solid fluorescence intensities based on the Mo8 cluster and R6G have been designed. Interestingly, the two assemblies can be controllably synthesized and their reversible conversion can also be achieved. The controlled synthesis and the regulation of corresponding properties have always been the goal pursued by chemists and material scientists. And the controlled synthesis of crystalline materials which are thermodynamically stable is still a challenge at present. Single crystal structures show that they possess different numbers of R6G molecules, in which Mo8-(R6G)2 has one Mo8 cluster, two TBA and two R6G, while Mo8-(R6G)4 has one Mo8 cluster and four R6G. Besides, steady-state photoluminescence (PL) spectra of R6G, Mo8-(R6G)2 and Mo8-(R6G)4 show that three samples display PL emission around 660 nm and the fluorescence intensity shows Mo8-(R6G)2 > Mo8-(R6G)4 > R6G.
In the light of the high fluorescence quantum yield and strong fluorescence emission characteristics of the R6G solution, we explored the possibility of R6G squeezing away different numbers of TBA in Mo8 to obtain complexes with various solid state fluorescence intensities. With this strategy, two assemblies with the participation of Mo8 clusters and R6G were obtained successfully. Mo8-(R6G)2 represents two R6G squeezing away two TBA, and Mo8-(R6G)4 means four R6G squeezed away four TBA. But no matter how reaction conditions (the ratio or concentration of Mo8 and R6G or organic solvents) were changed, assemblies with R6G squeezing three TBA or one TBA were still unavailable (Scheme 1).
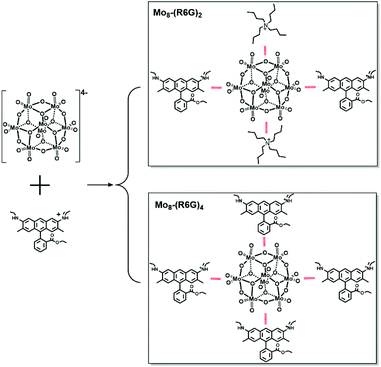 |
| Scheme 1 Representations of the assemblies of R6G with Mo8. | |
Results and discussion
Crystal structure
The single-crystal X-ray diffraction analysis reveals that Mo8-(R6G)2 crystallizes in the triclinic space group P
, and its asymmetric unit consists of one {Mo4O13}, one TBA and one C28H31N2O3 (abbreviated as R6G). As shown in Scheme 1, Mo8 consists of six ring shaped {MoO6} octahedra via edge-sharing and two {MoO4} tetrahedra occupied on the top and bottom of the ring, in which eight Mo atoms are connected by six two-bridged oxygens and six three-bridged oxygens to form the {Mo8O26} clusters. Besides, two TBA and two R6G cations interact with Mo8via electrostatic and C–H⋯O as well as N–H⋯O contacts. Single-crystal X-ray diffraction analysis reveals that Mo8-(R6G)4 also crystallizes in the triclinic space group P
, and its asymmetric unit consists of one {Mo4O13} and two R6G. As shown in Fig. 1b, the {Mo8O26} interacts with four R6G cations via electrostatic and C–H⋯O as well as N–H⋯O contacts.
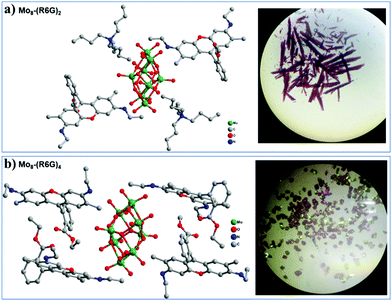 |
| Fig. 1 The ball-and-stick representations of Mo8-(R6G)2 (a) Mo8-(R6G)4 (b) and the digital pictures of the two kinds of crystals. Hydrogen bonds are omitted for clarity. | |
Optical properties
The absorption spectra of Mo8-(R6G)2 and Mo8-(R6G)4 dissolved in a CH3CN solution are the same as that of R6G in an acetonitrile solution, and the absorption spectrum of R6G in the 200–300 nm range overlaps with that of Mo8. In addition, Fig. S11 and S12† presented solid-state diffuse reflectance spectra. For Mo8, the absorption band is located in the 200–400 nm range, corresponding to colorless crystals, and the maximum absorption at 290 nm is assigned to a ligand-to-metal charge-transfer (LMCT) transition. The color of R6G, Mo8-(R6G)2 and Mo8-(R6G)4 is reddish brown; thus there exists an absorption band in the 500–700 nm range. This composite hybrid material between the organic dye and POM extends the absorption spectrum of POM from the ultraviolet region to the visible region.
As shown in Fig. 2a, under 365 nm ultraviolet light, both kinds of solids are capable of emitting red fluorescence with distinctive intensities, yet solids of R6G do not fluoresce. With the naked eye, it is easy to distinguish three samples based on brightness. The steady-state PL spectra of R6G, Mo8-(R6G)2 and Mo8-(R6G)4 are shown in Fig. 2b. At an excitation of 590 nm with maximum absorption in the solid state, the emission wavelengths of the three solid samples are around 660 nm, but their intensity shows a big discrepancy. Compared to R6G, the intensity for Mo8-(R6G)2 and Mo8-(R6G)4 increases by 11 times and 2 times, respectively.
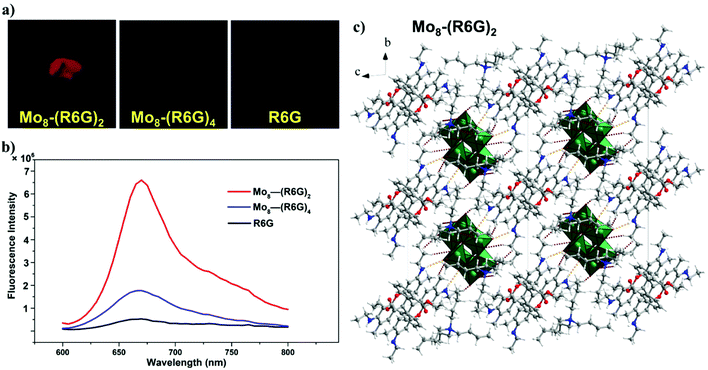 |
| Fig. 2 The digital pictures of three kinds of solids of Mo8-(R6G)2, Mo8-(R6G)4 and R6G under 365 nm irradiation (a), the comparison of steady-state PL spectra for three solid samples under 590 nm excitation (b) and the stacking pattern of Mo8-(R6G)2 (c). | |
The fluorescence lifetime is related to the microenvironment in which the substance is located, and the changes in the studied system can be understood through fluorescence lifetime analysis. Fig. S23–S26† show the fluorescence lifetimes of R6G, Mo8-(R6G)2 and Mo8-(R6G)4. The fluorescence lifetime with the single exponential fitting calculation for Mo8-(R6G)2 is 3.2 ns, but for Mo8-(R6G)4, the fluorescence lifetime is 1.4 ns. The intensity decay time of Mo8-(R6G)2 and Mo8-(R6G)4 is longer than that of R6G at 0.64 ns, indicating that intramolecular motions of R6G are significantly restricted, and that radiative decay is favored in the POM-R6G hybrid material.23
The Mo6 was reported to serve the function of immobilizing and diluting the dye molecules and minimizing quenching caused by the aggregation of dyes,23 and the phenomenon is known as anchoring and diluting effect luminescence. We speculate that the cationic R6G dye molecules confined by anionic Mo8 have restricted intramolecular torsional motion and restrained π–π interactions, which significantly diminish the aggregation-caused quenching effect observed in the solid R6G dye.24 As shown in Fig. 2c, the highly nucleophilic POM surfaces create strong N–H⋯O and C–H⋯O contacts with the organic luminophores, first. Next, the bulk [Mo8O26]4− anions act as large spacers that dilute R6G in the crystal lattice. The fluorescence intensity of Mo8-(R6G)2 is stronger than that of Mo8-(R6G)4, which may be due to the incorporation of TBA that can play a role of interval and dilution for R6G. As shown in Fig. S3–S5†, the crystal packing diagram of Mo8-(R6G)2 and Mo8-(R6G)4 in three directions along the a, b, and c axes shows that Mo8-(R6G)2 with two TBA has a looser structure. Furthermore, the shortest C⋯C distance between adjacent R6G cations in Mo8-(R6G)2 is longer than that for Mo8-(R6G)4, indicating weaker intermolecular π–π stacking interactions. The synergies between the anchoring and diluting effects on luminogens of R6G efficiently inhibits the aggregation and self-quenching on luminogens of R6G.
Controlled synthesis and reversible transformation
Mo8-(R6G)2 with two TBA and Mo8-(R6G)4 with no TBA has been synthesized from the one pot reaction of an acetonitrile mixture of Mo8 and R6G, and the reddish-brown single crystals have been isolated by evaporation. Moreover, the crystal shapes for Mo8-(R6G)2 and Mo8-(R6G)4 are rod and block, respectively, which are conducive to better differentiation and identification. Surprisingly, the synthesis of these two compounds can be controlled by adjusting the ratio between the reactants, achieving step-by-step synthesis or individual synthesis. For convenience, control experiments are achieved by adding different volumes of Mo8; the volume and concentration for R6G are 2 mL, 0.01 g/10 ml in acetonitrile, respectively while the concentration of Mo8 is 0.1 g/10 ml in acetonitrile.
As shown in Fig. 3, when the molar ratio of n(R6G)
:
n(Mo8) is less than or equal to 0.1, only rod crystals of Mo8-(R6G)2 were isolated; however, when the n(R6G)
:
n(Mo8) is greater than 1, only block crystals of Mo8-(R6G)4 were isolated. Once the molar ratio of n(R6G)
:
n(Mo8) was changed to a range of 0.1–1, both Mo8-(R6G)2 and Mo8-(R6G)4 were obtained in the same bottle at different times, in which Mo8-(R6G)4 with more R6G molecules crystallizes first, and then Mo8-(R6G)2 crystallizes out after filtration. This may be because the amount of R6G is relatively high at the beginning, so R6G can squeeze away all the TBA in Mo8. As Mo8-(R6G)4 crystallizes, the amount of R6G decreases, so Mo8-(R6G)2 with fewer R6G available will begin to crystallize.
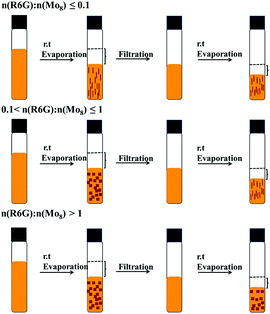 |
| Fig. 3 Schematic representation of the synthesis control of Mo8-(R6G)2 and Mo8-(R6G)4. | |
Inspired by controlled synthesis, we proposed an idea where Mo8-(R6G)2 and Mo8-(R6G)4 can be converted to each other as shown in Fig. 4. At first, we hoped that the transformation between Mo8-(R6G)2 and Mo8-(R6G)4 could be realized in a single crystal by simply soaking Mo8-(R6G)2 in R6G or Mo8-(R6G)4 in TBA to obtain Mo8-(R6G)4 or Mo8-(R6G)2, respectively, but it did not succeed because single crystal to single crystal transformation is not easy to achieve due to the retention of three-dimensional order. For the purpose of conversion, we employed another relatively easy way, where Mo8-(R6G)2 (rod) was dissolved in acetonitrile or methanol to transform to Mo8-(R6G)4 (block) by adding R6G solution (Fig. 4, top), while Mo8-(R6G)4 was converted to Mo8-(R6G)2 by means of dissolving it in acetonitrile with Mo8 solution (Fig. 4, middle).
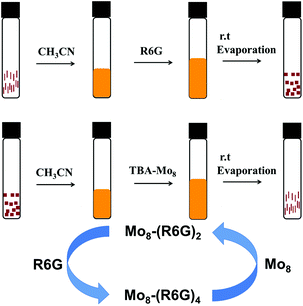 |
| Fig. 4 Schematic representation of reversible transformation of Mo8-(R6G)2 and Mo8-(R6G)4. | |
Conclusions
In summary, two new assemblies were successfully synthesized combining [Mo8O26]4− and the organic fluorescent cationic dye R6G. This new assembly strategy allows for replacing the TBA cations in {Mo8O26} clusters with organic fluorescent dyes, and obtaining the assemblies of Mo8-(R6G)2 with two R6G and Mo8-(R6G)4 with four R6G. The controllable synthesis and reversible conversion of these two assemblies have been achieved. Furthermore, fluorescence intensity in the solid state for them is enhanced compared with that of R6G, and Mo8-(R6G)2 is stronger than Mo8-(R6G)4, which is attributed to the anchoring and diluting effect of Mo8 to luminogens of R6G. This current study provides new ideas for the structural design, synthesis and transformation of POM-based complex materials, opening the way towards the design of novel multifunctional hybrid systems.
Conflicts of interest
There are no conflicts to declare.
Acknowledgements
We acknowledge the support of this work by the National Natural Science Foundation of China (NSFC 21801226) and the Zhejiang Provincial Natural Science Foundation of China (LY20B010002) and the Zhejiang Normal University Fund.
Notes and references
- V. Gray, D. Dzebo, A. Lundin, J. Alborzpour, M. Abrahamsson, B. Albinsson and K. Moth-Poulsen, Photophysical characterization of the 9,10-disubstituted anthracene chromophore and its applications in triplet-triplet annihilation photon upconversion, J. Mater. Chem. C, 2015, 3, 11111–11121 RSC.
- M. A. Baldo, D. F. O'Brien, Y. You, A. Shoustikov, S. Sibley, M. E. Thompson and S. R. Forrest, Highly efficient phosphorescent emission from organic electroluminescent devices, Nature, 1998, 395, 151–154 CrossRef CAS.
- D. Di, A. S. Romanov, L. Yang, J. M. Richter, J. P. H. Rivett, S. Jones, T. H. Thomas, M. A. Jalebi, R. H. Friend, M. Linnolahti, M. Bochmann and D. Credgington, High-performance light-emitting diodes based on carbene-metal-amides, Science, 2017, 356, 159–163 CrossRef CAS.
- T. N. Singh-Rachford, A. Haefele, R. Ziessel and F. N. Castellano, Boron dipyrromethene chromophores: next generation triplet acceptors/annihilators for low power upconversion schemes, J. Am. Chem. Soc., 2008, 130, 16164–16165 CrossRef CAS.
- G. Grynkiewicz, M. Poenie and R. Y. Tsien, A new generation of Ca2+ indicators with greatly improved fluorescence properties, J. Biol. Chem., 1985, 260, 3440–3450 CrossRef CAS.
- E. L. Que, D. W. Domaille and C. J. Chang, Metals in neurobiology: probing their chemistry and biology with molecular imaging, Chem. Rev., 2008, 108, 1517–1549 CrossRef CAS PubMed.
- K. Li and B. Liu, Polymer-encapsulated organic nanoparticles for fluorescence and photoacoustic imaging, Chem. Soc. Rev., 2014, 43, 6570–6597 RSC.
- H. S. Kim, S.-R. Park and M. C. Suh, Concentration quenching behavior of thermally activated delayed fluorescence in a solid film, J. Phys. Chem. C, 2017, 121, 13986–13997 CrossRef CAS.
- J. G. Wang, X. G. Gu, P. F. Zhang, X. B. Huang, X. Y. Zheng, M. Chen, H. T. Feng, R. T. K. Kwok, J. W. Y. Lam and B. Z. Tang, Ionization and Anion−π+ Interaction: A New Strategy for Structural Design of Aggregation-Induced Emission Luminogens, J. Am. Chem. Soc., 2017, 139, 16974–16979 CrossRef CAS.
- H. M. Zeng, Z. G. Jiang, H. W. Zhang, W. T. Mao, X. H. Gao and C. H. Zhan, An Extraordinary OER Electrocatalyst Based on the Co−Mo Synergistic 2D Pure Inorganic Porous Framework, Eur. J. Inorg. Chem., 2021, 2021(26), 2606–2610 CrossRef CAS.
- D. D. Li, B. H. Cen, C. T. Fang, X. Y. Leng, W. Y. Wang, Y. J. Wang, J. Chen and M. F. Luo, High performance cobalt nanoparticle catalysts supported by carbon for ozone decomposition: the effects of the cobalt particle size and hydrophobic carbon support, New J. Chem., 2021, 45, 561–568 RSC.
- J. T. Rhule, C. L. Hill, D. A. Judd and R. F. Schinazi, Polyoxometalates in Medicine, Chem. Rev., 1998, 98, 327–358 CrossRef CAS.
- Y. Wang, H. M. Zeng, W. T. Mao, X. J. Wang, Z. G. Jiang, C. H. Zhan and Y. L. Feng, The synthesis and photoluminescence of three porous metal-organic frameworks, Inorg. Chem. Commun, 2021, 129, 108613–108619 CrossRef CAS.
- X. Y. Yang, P. P. Zhu, X. L. Ma, W. J. Li, Z. L. Tan and J. Q. Sha, Graphite-like polyoxometalate-based metal–organic framework as an efficient anode for lithium ion batteries, CrystEngComm, 2020, 22, 1340–1345 RSC.
- C. H. Zhan, C. Busche, D. L. Long, P. I. Molina, R. S. Winter and L. Cronin, Encapsulation of a {Cu16} cluster containing four [Cu4O4] cubanes within an isopolyoxometalate {W44} cluster, Chem. Commun., 2017, 53, 7076–7079 RSC.
- J. M. Lin, N. Li, S. P. Yang, M. J. Jia, J. Liu, X. M. Li, L. An, Q. Tian, L. Z. Dong and Y. Q. Lan, Self-Assembly of Giant Mo240 Hollow Opening Dodecahedra, J. Am. Chem. Soc., 2020, 142, 13982–13988 CrossRef CAS.
- H. M. Gan, N. Xu, C. Qin, C. Y. Sun, X. L. Wang and Z. M. Su, Equi–size nesting of Platonic and Archimedean metal–organic polyhedra into a twin capsid, Nat. Commun., 2020, 11, 4103–4110 CrossRef CAS PubMed.
- S. G. Mitchell, C. Streb, H. N. Miras, T. Boyd, D. L. Long and L. Cronin, Face-directed self-assembly of an electronically active Archimedean polyoxometalate architecture, Nat. Chem., 2010, 2, 308–312 CrossRef CAS PubMed.
- C. H. Zhan, Q. Zheng, D. L. Long, L. Vilà-Nadal and L. Cronin, Controlling the Reactivity of the [P8W48O184]40− Inorganic Ring and Its Assembly into POMZite Inorganic Frameworks with Silver Ions, Angew. Chem., Int. Ed., 2019, 58, 17282–17286 CrossRef CAS PubMed.
- A. Müller and C. Serain, Soluble Molybdenum Blues-“des Pudels Kern”, Acc. Chem. Res., 2000, 33, 2–10 CrossRef.
- Z. G. Jiang, W. T. Mao, D. P. Huang, Y. Wang, X. J. Wang and C. H. Zhan, A nonconventional host–guest cubic assembly based on γ-cyclodextrin and a Keggin-type polyoxometalate, Nanoscale, 2020, 12, 10166–10171 RSC.
- H. Q. Zhang, J. Peng, Y. Shen, X. Yu, F. Zhang, J. Mei, B. Li and L. M. Zhang, Hybrid microtubes of polyoxometalate and fluorescence dye with tunable photoluminescence, Chem. Commun., 2012, 48, 4462–4462 RSC.
- P. Bolle, Y. Cheret, C. Roiland, L. Sanguinet, E. Faulques, H. Serier-Brault, P. A. Bouit, M. Hissler and R. Dessapt, Strong Solid-state Luminescence Enhancement in Supramolecular Assemblies of Polyoxometalate and “Aggregation-Induced Emission”-active Phospholium, Chem. – Asian J., 2019, 14, 1642–1646 CrossRef CAS.
- J. C. Yu, Y. J. Cui, H. Xu, Y. Yang, Z. Y. Wang, B. L. Chen and G. D. Qian, Confinement of pyridinium hemicyanine dye within an anionic metal-organic framework for two-photon-pumped lasing, Nat. Commun., 2013, 4, 2719–2725 CrossRef.
Footnote |
† Electronic supplementary information (ESI) available. CCDC 2090079 and 2090082. For ESI and crystallographic data in CIF or other electronic format see DOI: 10.1039/d1qi01014j |
|
This journal is © the Partner Organisations 2022 |