DOI:
10.1039/D1QI00226K
(Research Article)
Inorg. Chem. Front., 2022,
9, 70-77
Solvent-dependent textural properties of defective UiO-66 after acidic and basic treatment†
Received
22nd February 2021
, Accepted 1st November 2021
First published on 2nd November 2021
Abstract
UiO-66 is one of the most chemically stable metal–organic frameworks (MOFs) available. However, little is known about its stability in organic solvents. In this study, we synthesized a highly defective UiO-66 (HD-UiO-66) and a less defective one (D-UiO-66) and explored how their properties change when exposed to weak and strong acids, both organic and inorganic, and dissolved in different solvents: water, dichloromethane (DCM), and tetrahydrofuran (THF). Exposing both defective UiO-66 materials to weak acids and bases, such as acetic acid and triethylamine, maintains their crystalline structure and porosity, irrespective of the solvent. Sulphuric acid decomposes D-UiO-66 in all solvents studied and HD-UiO-66 in DCM and THF, but not in water. Trifluoroacetic acid decomposes the frameworks only in DCM. Tetramethylguanidine decomposes HD-UiO-66 and D-UiO-66 in organic solvents but maintains some of the MOFs’ porosity and crystalline structure in water, whereas potassium carbonate damages the MOFs to a greater extent in water than in organic solvents. Our results show that the acid/base properties of the solvent modulate the strength of acids and bases and its polarity determines the extent of their solvation, thus playing a crucial role in altering the MOF's textural properties. This systematic investigation highlights the central role played by the solvent in tuning the properties of MOFs, which is relevant for liquid-phase applications in acidic and basic environments, such as catalysis and adsorption.
Introduction
Metal–organic frameworks (MOFs) are porous coordination polymers constituted by inorganic nodes and organic linkers.1 Their unique features have drawn attention for applications in many different fields, including catalysis, gas separation and storage, and drug-delivery.2 A MOF of particular interest for the scientific community is UiO-66, first reported by Cavka et al. in 2008,3 consisting of [Zr6(OH)4O4]12+ nodes and terephthalate linkers. Its thermal,4 chemical5 and mechanical6 stability is attributed to the high connectivity and nuclearity of the inorganic node and promoted the development of a large family of “Zr6 MOFs”, including NU-10007 and MOF-808,8 with tetradentate and tridentate carboxylate linkers, respectively. Isoreticular MOFs were synthesized by using linkers with various functional groups4 and different size,3 post-synthetic modification (PSM),9,10 and post-synthetic exchange (PSE) of the linker11 and of the nodes.12
The simulated BET surface area of a defect-free UiO-66 structure is 1100 m2 g−1.13 The addition of acids as modulators during the synthesis of the framework promotes the formation of “missing linker” and/or “missing cluster” defects.14–17 Defects result in different properties of the framework, such as increased chemical reactivity in PSM,18 increased Lewis acidity,19 increased surface area20 and improved gas sorption properties,21 but may cause a reduction in the thermal stability of the compound.22
MOFs can decompose upon interaction with water via hydrolysis of the metal-linker bond.23 This phenomenon is extremely important for gas phase applications, such as carbon dioxide and water capture, and the stability of MOFs towards water vapour has been studied extensively.24 Similar mechanisms of decomposition may take place in liquid phase applications, such as catalysis. UiO-66 is stable upon treatment with aqueous solutions of different pH and with common solvents, retaining its crystalline structure.4,5,25 This may not be due necessarily to the framework's inertness, but rather to a dynamic process of ligand exchange.11 More recently, Leus et al. proved that a significant loss of the porosity of MOFs can take place without affecting their diffraction patterns,26 highlighting how nitrogen physisorption and diffraction provide complementary insight into the stability of MOFs upon chemical treatment. Bůžek et al. reported a detailed investigation of the stability of UiO-66 to aqueous buffer solutions.27 The reactivity of UiO-66 with acidic and basic solutions in organic solvents is extremely important for its applications. Its stability was, for the most part, proved indirectly: UiO-66-NH2 underwent PSM treatments with anhydrides both in solution of dichloromethane or chloroform or in gas phase, preserving crystallinity and porosity;9,10,28 and a UiO-67 derivative functionalized with palladium retained its structure after being used in Suzuki–Miyaura coupling.29 The role that defects in UiO-66 play in the chemical stability towards acids, bases and solvents remains largely unexplored. A recent contribution from Feng et al. showed that highly defective UiO-66 containing 4-sulfonatobenzoate as hemilabile linker shows a higher thermal stability than a defect-free material.30
In this work, we synthesized a highly defective and a defective UiO-66 (HD-UiO-66 and D-UiO-66, respectively) and studied their reactivity towards acids and bases in different solvents. By studying the textural properties of the samples with nitrogen physisorption and powder X-ray diffraction, we demonstrate that the solvent has a dramatic influence on modulating the interaction of the framework with such chemicals.
Results
We synthesized HD-UiO-66 with acetic acid as a modulator, giving a crystalline material with PXRD pattern showing a UiO-66 topology (Fig. S1, ESI†) and with a BET surface area larger than 2000 m2 g−1 (Fig. S2, ESI†), among the highest values reported for this material.31 Thermal Gravimetric Analysis (TGA) shows a linker-to-node ratio of around 4.1, indicative of a high defectivity of the sample. The TGA curve (Fig. S3, ESI†) shows the decomposition of the framework between 480 and 500 °C, comparable to that reported elsewhere.14 With a similar procedure, (see ESI†) we prepared D-UiO-66, which showed a lower BET surface area, comprised between 1550 and 1730 m2 g−1 between different batches. We explored how the textural properties of the defective UiO-66 frameworks would change, depending (a) on the polarity of the solvent from protic (water), to aprotic and polar (THF) and apolar (DCM) and (b) on the nature and strength of the acid and the base from inorganic (H2SO4 and K2CO3), strong organic (trifluoroacetic acid (TFA) and tetramethylguanidine (TMG)) and weak organic (AcOH and Et3N).
Treatment of HD-UiO-66 in aqueous solutions
The PXRD of the material formed after treatment of HD-UiO-66 with pure water showed reflections belonging to the UiO-66 framework, broader and of lower intensity than in the pristine material (Fig. 1, left, red and black curves). The BET surface area of the material reduced to 1590 m2 g−1 (Table S1, entry 2, ESI†), and the nitrogen adsorption isotherm showed a lower uptake than the pristine material at p/p0 > 0.1 (Fig. 1, right, red and black squares).
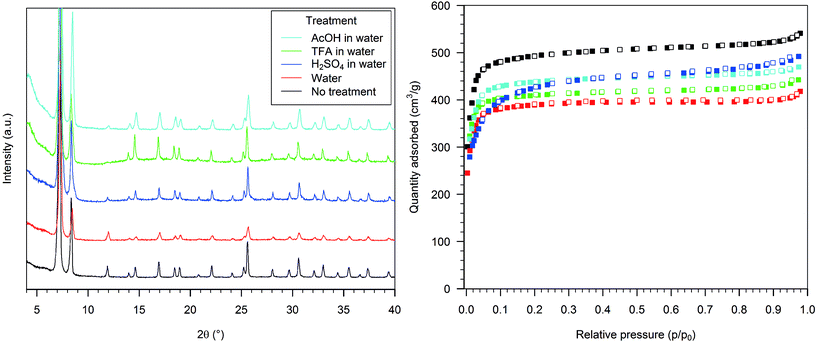 |
| Fig. 1 (left) PXRD patterns of as synthesized HD-UiO-66 (black) and of HD-UiO-66 after treatment with water (red) and with acidic aqueous solutions of H2SO4 (blue), TFA (green) and AcOH (light blue). (right) Corresponding nitrogen physisorption isotherms, adsorption represented by filled squares, desorption by open squares. | |
Treating the MOF with 0.1 M H2SO4 gave an almost identical PXRD pattern as that of the parent material (Fig. 1, left, blue curve). The nitrogen physisorption isotherm featured a steeper increase at p/p0 > 0.1 than the pristine material and resulted in a BET surface area of 1630 m2 g−1 (Fig. 1, right, blue squares). The material after exposure to a 0.1 M TFA solution showed an increased baseline in the PXRD pattern (Fig. 1, left, green curve); the nitrogen adsorption isotherm maintained a similar shape to the starting material but with reduced uptake capacity and a BET surface area of 1700 m2 g−1 (Fig. 1, right, green squares). Exposure to 0.1 M AcOH resulted only in slight PXRD peak broadening (Fig. 1, left, light blue curve), and the nitrogen adsorption capacity was reduced by a small extent; the BET surface area was 1820 m2 g−1 (Fig. 1, left, light blue squares).
Upon exposure to 0.1 M aqueous K2CO3 the sample lost its diffraction pattern (Fig. 2, left, blue curve), and the nitrogen uptake capacity was significantly reduced, with a BET surface area of 540 m2 g−1 (Fig. 2, right, blue squares). The diffraction pattern of the sample treated with a 0.1 M TMG solution showed only a few broad reflections, corresponding to the most intense signals (Fig. 2, left, green curve), and the BET surface area was reduced to 980 m2 g−1 (Fig. 2, right, green squares). The treatment with 0.1 M aqueous Et3N caused only a slight reduction in the intensity of the PXRD signals and a minor increase in the baseline (Fig. 2, left, light blue), but the nitrogen physisorption isotherm was similar to that of the previous sample, with a BET surface area of 1020 m2 g−1 (Fig. 2, right, light blue squares).
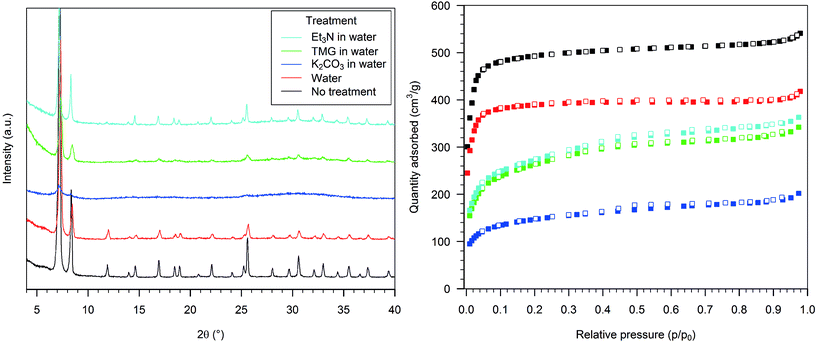 |
| Fig. 2 (left) PXRD patterns of as synthesized HD-UiO-66 (black) and of HD-UiO-66 after treatment with water (red) and with basic aqueous solutions of K2CO3 (blue), TMG (green) and Et3N (light blue). (right) Corresponding nitrogen physisorption isotherms, adsorption represented by filled squares, desorption by open squares. | |
Treatment of HD-UiO-66 in tetrahydrofuran solutions
The treatment of HD-UiO-66 with pure THF resulted in a slight broadening of the PXRD peaks (Fig. 3, left, red curve) and a decrease in the nitrogen uptake, with a BET surface area of 1620 m2 g−1 (Fig. 3, right, red squares).
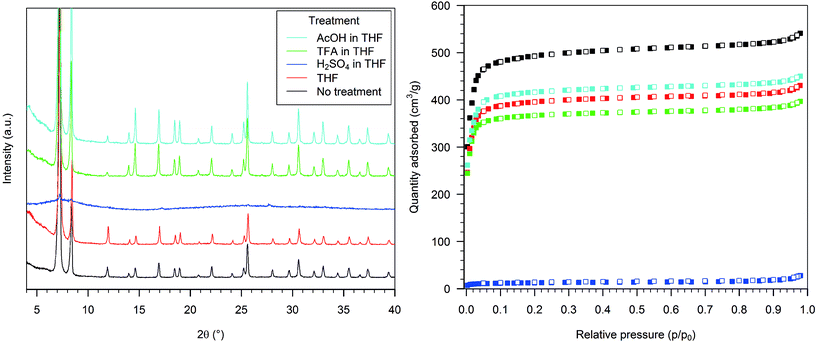 |
| Fig. 3 (left) PXRD patterns of as synthesized HD-UiO-66 (black) and of HD-UiO-66 after treatment with THF (red) and with acidic solutions of H2SO4 (blue), TFA (green) and AcOH (light blue) in THF. (right) Corresponding nitrogen physisorption isotherms, adsorption represented by filled squares, desorption by open squares. | |
Treating HD-UiO-66 with a 0.1 M solution of H2SO4 in THF caused the loss of the diffraction pattern (Fig. 3, left, blue curve) and of the nitrogen uptake capacity of the material, resulting in a BET surface area of 50 m2 g−1 (Fig. 3, right, blue squares). The material obtained after exposure to a TFA solution had a diffraction pattern comparable to that of the pristine material (Fig. 3, left, green curve) and a similar nitrogen physisorption isotherm, with a BET surface area of 1510 m2 g−1 (Fig. 3, right, green squares). HD-UiO-66 treated with 0.1 M AcOH showed a minimally affected PXRD pattern (Fig. 3, left, light blue curve) and nitrogen physisorption isotherm, with a BET surface area of 1700 m2 g−1 (Fig. 3, right, light blue squares).
The PXRD pattern of HD-UiO-66 after treatment with 0.1 M K2CO3 in THF was similar to that of the starting material but with two additional reflections, attributed to residues of the base (Fig. 4, left, blue curve). The BET surface area was reduced to 1060 m2 g−1 (Fig. 4, right, blue squares), a value that is underestimated because of the presence of a non-porous solid in the mixture. The material obtained after exposure of the MOF to a TMG solution showed only a broad reflection in the diffractogram (Fig. 4, left, green curve) and a low nitrogen physisorption isotherm, with a BET surface area of 470 m2 g−1 (Fig. 4, right, green squares). Treatment with 0.1 M Et3N did not affect the PXRD pattern (Fig. 4, left, light blue curve) and caused only a slight reduction in nitrogen uptake, with a decrease in BET surface area to 1760 m2 g−1 (Fig. 4, right, light blue squares).
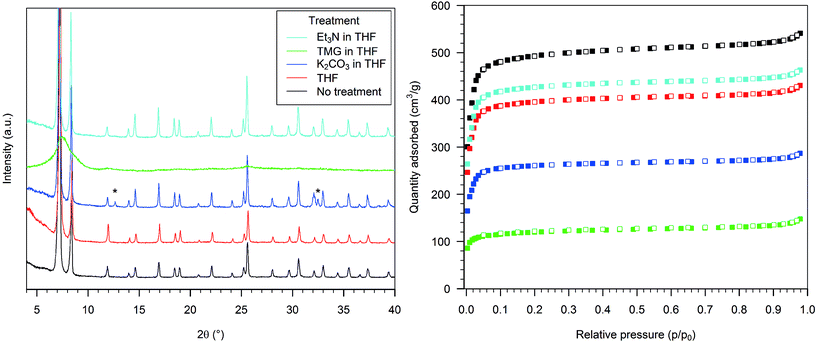 |
| Fig. 4 (left) PXRD patterns of as synthesized HD-UiO-66 (black) and of HD-UiO-66 after treatment with THF (red) and with basic solutions of K2CO3 (blue), TMG (green) and Et3N (light blue) in THF. Residues of K2CO3 are indicated by a star. (right) Corresponding nitrogen physisorption isotherms, adsorption represented by filled squares, desorption by open squares. | |
Treatment of HD-UiO-66 in dichloromethane solutions
Soaking HD-UiO-66 in pure DCM had a minimal effect on its diffraction pattern (Fig. 5, left, red curve) and nitrogen physisorption isotherm, with a reduction of the BET surface area to 1850 m2 g−1 (Fig. 5, right, red squares).
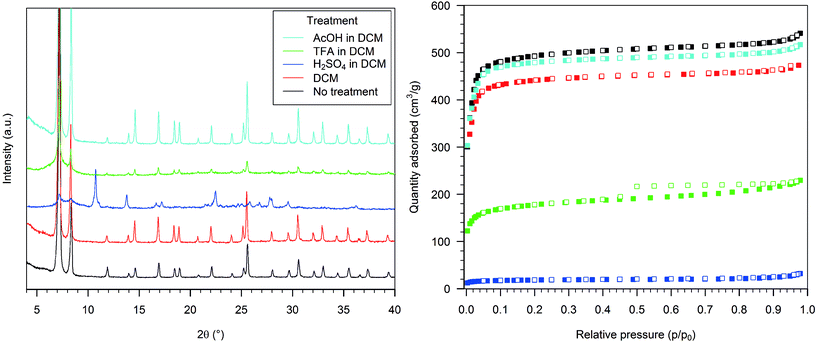 |
| Fig. 5 (left) PXRD patterns of as synthesized HD-UiO-66 (black) and of HD-UiO-66 after treatment with DCM (red) and with acidic solutions of H2SO4 (blue), TFA (green) and AcOH (light blue) in DCM. (right) Corresponding nitrogen physisorption isotherms, adsorption represented by filled squares, desorption by open squares. | |
The material that formed after treatment of HD-UiO-66 with 0.1 M H2SO4 in this solvent had different PXRD pattern than the starting material (Fig. 5, left, blue curve) and showed no nitrogen uptake, with a BET surface area of 70 m2 g−1 (Fig. 5, right, blue squares). Exposure to a TFA solution resulted in a diffraction pattern with weak reflections belonging to the starting material and a broad signal between 5° and 9° (Fig. 5, left, green curve) and the BET surface area decreased to 680 m2 g−1 (Fig. 5, right, green squares). After treatment with 0.1 M AcOH, the material retained its PXRD pattern (Fig. 5, left, light blue curve) and nitrogen physisorption isotherm, with a BET surface area of 1950 m2 g−1 (Fig. 5, right, light blue squares).
The diffraction pattern of the material obtained after treating HD-UiO-66 with K2CO3 in DCM contains weak and broad reflections of the starting material as well as those of the base residues (Fig. 6, left, blue curve); its BET surface area was reduced to 680 m2 g−1 (Fig. 6, right, blue squares), an underestimated value. Treatment with a 0.1 M TMG solution resulted in the loss of the framework reflections from the PXRD pattern, which contains only a broad peak (Fig. 6, left, green curve), and in a lower nitrogen physisorption isotherm with a BET surface area of 640 m2 g−1 (Fig. 6, right, green squares). Exposing the MOF to a Et3N solution did not change the PXRD pattern (Fig. 6, left, light blue curve) and reduced the BET surface area to 1710 m2 g−1 (Fig. 6, right, light blue squares).
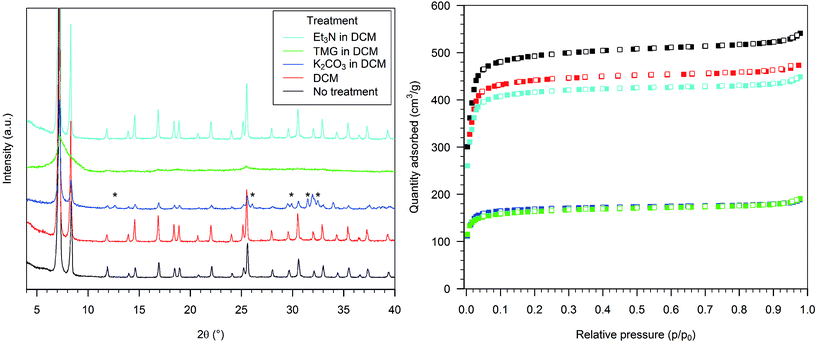 |
| Fig. 6 (left) PXRD patterns of as synthesized HD-UiO-66 (black) and of HD-UiO-66 after treatment with DCM (red) and with basic solutions of K2CO3 (blue), TMG (green) and Et3N (light blue) in DCM. Residues of K2CO3 are indicated by a star. (right) Corresponding nitrogen physisorption isotherms, adsorption represented by filled squares, desorption by open squares. | |
Chemical treatment of D-UiO-66
The behaviour of D-UiO-66 in the conditions above was in most cases comparable to that of HD-UiO-66, resulting in the formation of materials with similar textural properties. Significant deviations were observed in the following cases. Treatment with aqueous H2SO4 formed a material with broad and weak diffraction peaks of UiO-66 and surface area of 130 m2 g−1. Upon reaction of D-UiO-66 with K2CO3 in DCM, the resulting solid showed the crystalline phases of UiO-66 and K2CO3 in the PXRD and had surface area of 120 m2 g−1. Treatment with TMG, in both THF and DCM, the materials derived from D-UiO-66 showed a crystalline diffraction pattern with surface area of 540 m2 g−1 and 550 m2 g−1 respectively.
Discussion
Fig. 7 schematically summarizes our observations on the acidic and basic chemical treatment of HD-UiO-66 and D-UiO-66. The textural properties of the MOF are impacted considerably, both by the solvent and the presence of an acid or a base. HD-UiO-66 shows similar behaviour in water as the corresponding defect-free material, being more resistant to acidic than to basic solutions.4,5 By comparing the effect of solvents alone, we found that polar solvents have a stronger effect on reduction of crystallite size, mesoporosity (Fig. 1, 3 and 5, red samples) and BET surface area (Table S1, entries 2, 9 and 16, ESI†). As Kim et al. demonstrated, suspension in solvents promotes the dissolution and recrystallization of this material, with a higher rate found for polar solvents.11
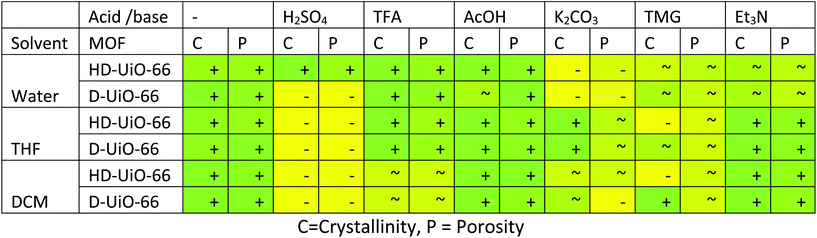 |
| Fig. 7 Summary on the retention of textural properties of HD-UiO-66 and D-UiO-66, according to both characterization techniques. Colour gradient: green = complete retention and yellow = complete loss of textural properties. Symbols: + = good retention, ∼ = partial retention and − = loss of textural property. | |
While HD-UiO-66 retains its textural properties after treatment with weak acids and bases in all solvents, it is strongly affected by the solvent in the treatment with strong acids and bases. In comparing the effect of strong acid solutions in water and in DCM, it is clear that H2SO4 and TFA have a minimal effect on the integrity of HD-UiO-66 in aqueous solution, losing around 20% of its surface area (Table S1, entries 3 and 4, ESI†), while in DCM there is decomposition of the MOF (Fig. 5, blue and green curves). Both H2SO4 and TFA dissociate to H3O+ and the corresponding conjugated base in water. The acid H3O+ is the one that likely reacts with the framework. The situation is different in the apolar solvent DCM, which does not have basic sites. In that case the strongest proton acceptor in the reaction mixture is the framework: protonation of terephthalate linkers by H2SO4 and TFA is a plausible explanation for the decomposition of the MOF in DCM.24 In THF, which is still polar but not protic, the results were intermediate: a H2SO4 solution causes the decomposition of the framework, while a TFA solution has only minor effects on its textural properties (Fig. 3, blue and green curves).
The situation with the textural properties of HD-UiO-66 treated with basic water solutions is more complex. TMG has a pKa value of 13, while K2CO3 and Et3N have a similar pKa, around 10.5 in water, showing that the integrity of the framework is not exclusively affected by the strength of the base. Even though TMG is a much stronger base than K2CO3, it damages the framework to a lesser extent and reduces the surface area as much as a solution of Et3N. This shows that the nature and size of the base play important roles in the reactivity of HD-UiO-66 in water and that the concentration of OH− is not the only determining factor in MOF decomposition. Whereas water solutions of the organic bases have a similar effect on the framework, in other solvents the effects differ significantly. Solutions of TMG in organic solvents destroy the framework; solutions of Et3N leave it unaffected. The different basic strengths may not be the only explanation; other properties of the reaction mixture, e.g. the ligand properties of the bases and how they are affected by solvation, must be considered. Water, with its hydrogen-bond donor capacity, can efficiently solvate the bases and modulate their interaction with zirconium ions. In solution of THF or DCM, the bases are “naked” and can interact directly with the framework's nodes.
D-UiO-66 showed a comparable behaviour to HD-UiO-66 in most of the conditions studied with some differences. The surface area reduction was more pronounced in the treatment with H2SO4 in water, to 8% for D-UiO-66 and 81% for HD-UiO-66 (Table S2,† entry 2). This difference shows that higher defectivity, in addition to increasing thermal stability,30 enhances the retention of surface area of UiO-66 after treatment in aqueous H2SO4. Reaction with K2CO3 in DCM reduced the surface area to 8% for D-UiO-66 and to 37% for HD-UiO-66 (Table S2,† entry 19). The samples derived by treating D-UiO-66 with TMG in organic solvents appeared to be more crystalline than those derived from HD-UiO-66, despite a comparable reduction in porosity.
Conclusions
In conclusion, the retention of textural properties of defective UiO-66 depends on the solvent, especially upon treatment with strong acids and strong bases, and its defectivity in the case of strong acids in water. The role of the solvent is to solvate the acid or base and their conjugated species and act as a mediator of the acid and base strength. Whereas in water the acids and bases produce H3O+ and OH−, this is not the case in organic solvents, which leave the acids and bases more “naked”, enabling different reactivity with the framework. The treatment of HD-UiO-66 and D-UiO-66 with mild acids and bases minimally changes their textural properties in all solvents. These results will be useful in the MOF community, since the conditions studied are relevant for catalysis and other liquid-phase applications.
Conflicts of interest
There are no conflicts to declare.
Acknowledgements
The authors thank Dr. Dennis Palagin and Ms. Marcia Schoenberg for proofreading. We also thank F. Hoffmann-La Roche AG for funding.
References
- W. Lu, Z. Wei, Z.-Y. Gu, T.-F. Liu, J. Park, J. Park, J. Tian, M. Zhang, Q. Zhang, T. Gentle III, M. Bosch and H.-C. Zhou, Tuning the structure and function of metal–organic frameworks via linker design, Chem. Soc. Rev., 2014, 43, 5561–5593 RSC.
- H. Furukawa, K. E. Cordova, M. O'Keeffe and O. M. Yaghi, The Chemistry and Applications of Metal-Organic Frameworks, Science, 2013, 341, 1230444 CrossRef PubMed.
- J. H. Cavka, S. Jakobsen, U. Olsbye, N. Guillou, C. Lamberti, S. Bordiga and K. P. Lillerud, A New Zirconium Inorganic Building Brick Forming Metal Organic Frameworks with Exceptional Stability, J. Am. Chem. Soc., 2008, 130, 13850–13851 CrossRef.
- M. Kandiah, M. H. Nilsen, S. Usseglio, S. Jakobsen, U. Olsbye, M. Tilset, C. Larabi, E. A. Quadrelli, F. Bonino and K. P. Lillerud, Synthesis and Stability of Tagged UiO-66 Zr-MOFs, Chem. Mater., 2010, 22, 6632–6640 CrossRef CAS.
- J. B. DeCoste, G. W. Peterson, H. Jasuja, T. G. Glover, Y.-g. Huang and K. S. Walton, Stability and degradation mechanisms of metal–organic frameworks containing the Zr6O4(OH)4 secondary building unit, J. Mater. Chem. A, 2013, 1, 5642–5650 RSC.
- H. Wu, T. Yildirim and W. Zhou, Exceptional Mechanical Stability of Highly Porous Zirconium Metal–Organic Framework UiO-66 and Its Important Implications, J. Phys. Chem. Lett., 2013, 4, 925–930 CrossRef CAS.
- J. E. Mondloch, W. Bury, D. Fairen-Jimenez, S. Kwon, E. J. DeMarco, M. H. Weston, A. A. Sarjeant, S. T. Nguyen, P. C. Stair, R. Q. Snurr, O. K. Farha and J. T. Hupp, Vapor-Phase Metalation by Atomic Layer Deposition in a Metal–Organic Framework, J. Am. Chem. Soc., 2013, 135, 10294–10297 CrossRef CAS PubMed.
- H. Furukawa, F. Gándara, Y.-B. Zhang, J. Jiang, W. L. Queen, M. R. Hudson and O. M. Yaghi, Water Adsorption in Porous Metal–Organic Frameworks and Related Materials, J. Am. Chem. Soc., 2014, 136, 4369–4381 CrossRef CAS PubMed.
- M. Kandiah, S. Usseglio, S. Svelle, U. Olsbye, K. P. Lillerud and M. Tilset, Post-synthetic modification of the metal–organic framework compound UiO-66, J. Mater. Chem., 2010, 20, 9848–9851 RSC.
- S. J. Garibay and S. M. Cohen, Isoreticular synthesis and modification of frameworks with the UiO-66 topology, Chem. Commun., 2010, 46, 7700–7702 RSC.
- M. Kim, J. F. Cahill, Y. Su, K. A. Prather and S. M. Cohen, Postsynthetic ligand exchange as a route to functionalization of ‘inert’ metal–organic frameworks, Chem. Sci., 2012, 3, 126–130 RSC.
- M. Kim, J. F. Cahill, H. Fei, K. A. Prather and S. M. Cohen, Postsynthetic Ligand and Cation Exchange in Robust Metal–Organic Frameworks, J. Am. Chem. Soc., 2012, 134, 18082–18088 CrossRef CAS PubMed.
- M. J. Katz, Z. J. Brown, Y. J. Colón, P. W. Siu, K. A. Scheidt, R. Q. Snurr, J. T. Hupp and O. K. Farha, A facile synthesis of UiO-66, UiO-67 and their derivatives, Chem. Commun., 2013, 49, 9449–9451 RSC.
- G. C. Shearer, S. Chavan, S. Bordiga, S. Svelle, U. Olsbye and K. P. Lillerud, Defect Engineering: Tuning the Porosity and Composition of the Metal–Organic Framework UiO-66 via Modulated Synthesis, Chem. Mater., 2016, 28, 3749–3761 CrossRef CAS.
- M. Taddei, When
defects turn into virtues: The curious case of zirconium-based metal-organic frameworks, Coord. Chem. Rev., 2017, 343, 1–24 CrossRef CAS.
- M. Taddei, R. J. Wakeham, A. Koutsianos, E. Andreoli and A. R. Barron, Post-Synthetic Ligand Exchange in Zirconium-Based Metal-Organic Frameworks: Beware of The Defects!, Angew. Chem., Int. Ed. Engl., 2018, 57, 11706–11710 CrossRef CAS.
- L. Liu, Z. Chen, J. Wang, D. Zhang, Y. Zhu, S. Ling, K.-W. Huang, Y. Belmabkhout, K. Adil, Y. Zhang, B. Slater, M. Eddaoudi and Y. Han, Imaging defects and their evolution in a metal–organic framework at sub-unit-cell resolution, Nat. Chem., 2019, 11, 622–628 CrossRef CAS PubMed.
- G. C. Shearer, J. G. Vitillo, S. Bordiga, S. Svelle, U. Olsbye and K. P. Lillerud, Functionalizing the Defects: Postsynthetic Ligand Exchange in the Metal Organic Framework UiO-66, Chem. Mater., 2016, 28, 7190–7193 CrossRef CAS.
- F. Vermoortele, B. Bueken, G. Le Bars, B. Van de Voorde, M. Vandichel, K. Houthoofd, A. Vimont, M. Daturi, M. Waroquier, V. Van Speybroeck, C. Kirschhock and D. E. De Vos, Synthesis Modulation as a Tool To Increase the Catalytic Activity of Metal–Organic Frameworks: The Unique Case of UiO-66(Zr), J. Am. Chem. Soc., 2013, 135, 11465–11468 CrossRef CAS PubMed.
- H. Wu, Y. S. Chua, V. Krungleviciute, M. Tyagi, P. Chen, T. Yildirim and W. Zhou, Unusual and Highly Tunable Missing-Linker Defects in Zirconium Metal–Organic Framework UiO-66 and Their Important Effects on Gas Adsorption, J. Am. Chem. Soc., 2013, 135, 10525–10532 CrossRef CAS PubMed.
- A. W. Thornton, R. Babarao, A. Jain, F. Trousselet and F. X. Coudert, Defects in metal–organic frameworks: a compromise between adsorption and stability?, Dalton Trans., 2016, 45, 4352–4359 RSC.
- G. C. Shearer, S. Chavan, J. Ethiraj, J. G. Vitillo, S. Svelle, U. Olsbye, C. Lamberti, S. Bordiga and K. P. Lillerud, Tuned to Perfection: Ironing Out the Defects in Metal–Organic Framework UiO-66, Chem. Mater., 2014, 26, 4068–4071 CrossRef CAS.
- J. J. Low, A. I. Benin, P. Jakubczak, J. F. Abrahamian, S. A. Faheem and R. R. Willis, Virtual High Throughput Screening Confirmed Experimentally: Porous Coordination Polymer Hydration, J. Am. Chem. Soc., 2009, 131, 15834–15842 CrossRef CAS PubMed.
- S. Yuan, L. Feng, K. Wang, J. Pang, M. Bosch, C. Lollar, Y. Sun, J. Qin, X. Yang, P. Zhang, Q. Wang, L. Zou, Y. Zhang, L. Zhang, Y. Fang, J. Li and H.-C. Zhou, Stable Metal–Organic Frameworks: Design, Synthesis, and Applications, Adv. Mater., 2018, 30, 1704303 CrossRef PubMed.
- C. G. Piscopo, A. Polyzoidis, M. Schwarzer and S. Loebbecke, Stability of UiO-66 under acidic treatment: Opportunities and limitations for post-synthetic modifications, Microporous Mesoporous Mater., 2015, 208, 30–35 CrossRef CAS.
- K. Leus, T. Bogaerts, J. De Decker, H. Depauw, K. Hendrickx, H. Vrielinck, V. Van Speybroeck and P. Van Der Voort, Systematic study of the chemical and hydrothermal stability of selected “stable” Metal Organic Frameworks, Microporous Mesoporous Mater., 2016, 226, 110–116 CrossRef CAS.
- D. Bůžek, S. Adamec, K. Lang and J. Demel, Metal–organic frameworks vs. buffers: case study of UiO-66 stability, Inorg. Chem. Front., 2021, 8, 720–734 RSC.
- M. Servalli, M. Ranocchiari and J. A. Van Bokhoven, Fast and high yield post-synthetic modification of metal–organic frameworks by vapor diffusion, Chem. Commun., 2012, 48, 1904–1906 RSC.
- H. Fei and S. M. Cohen, A robust, catalytic metal–organic framework with open 2,2′-bipyridine sites, Chem. Commun., 2014, 50, 4810–4812 RSC.
- X. Feng, J. Hajek, H. S. Jena, G. Wang, S. K. P. Veerapandian, R. Morent, N. De Geyter, K. Leyssens, A. E. J. Hoffman, V. Meynen, C. Marquez, D. E. De Vos, V. Van Speybroeck, K. Leus and P. Van Der Voort, Engineering a Highly Defective Stable UiO-66 with Tunable Lewis- Brønsted Acidity: The Role of the Hemilabile Linker, J. Am. Chem. Soc., 2020, 142, 3174–3183 CrossRef CAS.
- K. Wang, C. Li, Y. Liang, T. Han, H. Huang, Q. Yang, D. Liu and C. Zhong, Rational construction of defects in a metal–organic framework for highly efficient adsorption and separation of dyes, Chem. Eng. J., 2016, 289, 486–493 CrossRef CAS.
Footnote |
† Electronic supplementary information (ESI) available. See DOI: 10.1039/d1qi00226k |
|
This journal is © the Partner Organisations 2022 |
Click here to see how this site uses Cookies. View our privacy policy here.