DOI:
10.1039/D1FO03208A
(Paper)
Food Funct., 2022,
13, 113-120
A functional polysaccharide from Eriobotrya japonica relieves myocardial ischemia injury via anti-oxidative and anti-inflammatory effects
Received
24th September 2021
, Accepted 25th November 2021
First published on 25th November 2021
Abstract
We herein report a food-derived polysaccharide (EJP) with the effect of relieving myocardial ischemia reperfusion injury (MIRI). This novel polysaccharide was isolated from the leaf of Eriobotrya japonica, and we first found its myocardium protective effects in vitro. Then, we firstly characterized EJP with a series of analytical technologies and further tested its effect on myocardial ischemia reperfusion injury (MIRI) with the illustration of the potential mechanisms in vivo. Interestingly, in the murine model of MIRI, administration of EJP effectively improved post-I/R heart contraction and limited the infarct size. Moreover, EJP significantly attenuated IR-induced oxidative damage and inflammatory reaction, as evidenced by decreasing MDA, IL-6, and TNF-α contents and increasing SOD activity and GSH-Px expression. In addition, we proved that EJP not only had no nephrotoxicity but also demonstrated a protective effect on the kidneys through HE staining and biochemical analysis. In sum, EJP, with a significant protective effect against myocardial I/R injury by showing anti-inflammatory and anti-oxidative activities, may become a meaningful drug candidate for the treatment of myocardial I/R injury.
1. Introduction
Polysaccharides derived from nature are commonly found to exert various biological activities including anti-tumor, anti-viral, anti-oxidative, and anti-aging effects, and so on.1–5 Among these, a unique role of natural polysaccharides in cellular signaling is to mediate the functions of immune cells in vitro and in vivo.6,7 They can enhance macrophage phagocytosis, strengthen lymphocyte functions, and heighten immunosurveillance against tumors.8–10 They can modulate the inflammatory response via interacting with macrophages and dendritic cells (DCs) expressing abundant carbohydrate receptors including mannose receptors (MRs), dectin-1 and macrophage galactose-type lectin (MGL).11,12 Among these, their different molecular weights, monosaccharide components, and glycosidic linkage patterns directly affect the immunoregulatory activities of polysaccharides, and further influence the secretion of inflammatory cytokines.13 With the revelation of the side effects of some synthetic drugs for treating inflammations in clinics, there is increasing interest in searching for safe, low toxicity, and effective natural drug candidates. Such an interesting feature of polysaccharides inspires the development of natural drugs for treating diseases induced by inflammations.14
Currently, acute myocardial infarction (AMI) is the main cause of morbidity and mortality in cerebrovascular diseases.15 With the application of reperfusion therapy for AMI in clinics, myocardial ischemia reperfusion injury (MIRI) has become an inevitable clinical challenge, which attracts extensive attention. At present, calcium channel blockers and antioxidants are the two main therapeutic drugs for MIRI.16 However, both of them have clinical limitations to a certain extent including side effects such as acute kidney injury, arrhythmia, hypertension, and low bioavailability.17 Based on this, potential therapeutic agents with fewer side effects have promising prospects in clinics. Recently, numerous studies have indicated that oxidative stress and inflammation play vital roles in the pathogenesis of MIRI.18 It is generally believed that when ischemia or myocardial infarction occurs, reactive oxygen species (ROS) triggers the chain lipid peroxidation, altering the structural and functional integrity of cells and inducing cell necrosis and apoptosis.19 Furthermore, ROS is a stimulatory signal of nuclear factor κB (NF-κB) activation which can induce inflammation and cause damage through up-regulating tumor necrosis factor-α (TNF-α) and interleukin 6 (IL-6).20 Therefore, exploration of appropriate anti-inflammatory and antioxidant agents will be of great value for treating MIRI in clinics. As mentioned above, a series of natural polysaccharides may be developed as anti-inflammatory and antioxidative agents for a series of therapeutic applications. Thereby, we can explore the polysaccharides with clear molecular weights, monosaccharide compositions and glycosidic linkage patterns for the discovery of new therapeutic agents for MIRI.
In this study, we report the discovery of a galacturonic acid (GalA)-presenting polysaccharide that possesses anti-inflammatory and anti-oxidative properties both in vitro and in vivo. This acidic heteroglycan, namely EJP, was isolated from the leaf of Eriobotrya japonica L., which is a high-quality fruit widely distributed in several countries. After obtaining this polysaccharide (EJP), we tested the effect of EJP on MIRI in mice. Then we characterized this polysaccharide and further observed the anti-oxidative and anti-inflammatory activities and potential protective effects in ischemia-reperfusion mice. Our findings may provide insights into the exploration of new therapeutic agents based on this polysaccharide.
2. Experimental
2.1 Materials and reagents
The leaves of Eriobotrya japonica L. (Chuannong8 cultivar) were purchased from the market of Chengdu, Sichuan Province and sent for third-party verification at Sichuan agricultural university. The monosaccharide standards including D-glucose (Glc), D-galactose (Gal), L-rhamnose (Rha), L-arabinose (Ara), D-mannose (Man), D-xylose (Xyl), D-galacturonic acid (D-GalA), and trifluoroacetic acid (TFA) were purchased from Sigma-Aldrich. All reagents were of the highest grade available. Dulbecco's modified Eagle's medium (DMEM) and fetal bovine serum (FBS) were purchased from Life Technologies/Gibco Laboratories (Grand Island, NY); ELISA kits for IL-6, TNF-α, MDA, GSH-Px, SOD, CK-MB, BUN and creatinine were purchased from Neobioscience (Shenzhen, China); and rabbit antibodies for GAPDH, pro-caspase3, cleaved-caspase3, and Bcl-2 were purchased from Cell Signaling Technology (USA). MTT assay, JC-1 and Annexin V-FITC/PI assay kits were purchased from Beyotime (China).
2.2 Preparation of EJP polysaccharide
We obtained the leaves of Eriobotrya japonica L. from Chengdu, Sichuan Province, and then the leaves were washed, cut into small pieces, and dried using microwaves at 400 W. Furthermore, the leaves were ground and extruded through a 60-mesh sieve. After defatting with ultrasonication for 30 min in 80% ethanol, the residue was extracted with ultrasound assisted extraction (442 W for 21 min each time) in ultrapure water (the volume ratio of sample
:
ultrapure water is 1
:
42). After centrifugation at 4000g for 20 min, the supernatant was retained and the residue was extracted for the second time. After extraction and centrifugation at 4000g for 20 min, the supernatant was also retained. Then, 95% ethanol was added into the supernatant slowly at room temperature using a magnetic stirrer, and the crude polysaccharide was precipitated overnight at 4 °C. After centrifugation, the obtained product was washed and the protein was removed by Sevag's method (chloroform/n-butanol, 4
:
1, v/v). The collected aqueous phase was subsequently purified by ultrafiltration at a cut-off of 8000–14
000 Da and lyophilized in order to obtain the polysaccharide.21 Then, the morphology of the obtained polysaccharide was observed using a scanning electron microscope (JSM-7500F, Japan).22
2.3 The molecular weight of EJP
The molecular weight (Mw) and polydispersity (Mw/Mn) of EJP were measured using a high-performance size exclusion chromatography system with a multi-angle laser scattering and refractive index detector (HPSEC-MALLS-RID, Wyatt Technology Co., Santa, USA) according to a published protocol.21 Briefly, the MW of EJP was detected using a HPSEC-MALLS-RID system with an Agilent 1260 series liquid chromatography system (Agilent Technologies, on a multi-angle laser scattering detector, USA). The polysaccharide was eluted with 0.9% NaCl aqueous solution using a Shodex OHpak SB-806 M HQ column (300 mm × 8.0 mm, i.d.). We selected 0.15 mL g−1 as the dn/dc value of EJP. The Astra software was used for data collection and analysis, and the molecular weight of EJP was calculated by the Zimm static light scattering method.
2.4 The monosaccharide compositions of EJP
We detected the monosaccharide compositions of EJP, which were measured by high performance liquid chromatography.21 Briefly, 4.0 mg of polysaccharide was hydrolyzed with 2.0 M trifluoroacetic acid (2.0 mL) at 95 °C for 10 h. After hydrolysis, the hydrolysate was mixed with 1 mL of methanol and evaporated on a rotary evaporator under vacuum. The addition of methanol was repeated twice as mentioned above. Subsequently, the dried hydrolysate was dissolved in 1 mL of deionized water for 1-phenyl-3-methyl-5-pyrazolone (PMP) derivatization. Then, the mixture was incubated at 70 °C for 100 min, and it was neutralized with hydrochloric acid solution. After shaking vigorously with 1 mL of chloroform, the organic layer was discarded. Finally, the solution was filtered through a 0.22 μm membrane for HPLC analysis. Meanwhile, standard monosaccharides containing Rha, Man, GlcA, GalA, Glc, Gal, Xyl, and Ara were analysed following the same procedure. PMP derivatives were analyzed using the Agilent 1260 series liquid chromatography system (Agilent Technologies, Palo Alto, CA, USA) with a ZORBAX Eclipse XDB-C18 column (4.6 × 250 mm, id 5 μm, Agilent Technologies Inc., CA, USA). The mobile phase is a mixture solution of 0.1 M phosphate buffer (pH = 6.7) and acetonitrile (83
:
17, v/v).
2.5 The FT-IR of EJP
The Fourier Transform Infrared (FT-IR) analysis of EJP was performed according to a published method with some minor revisions. Briefly, each sample (1.0 mg) was mixed with 80 mg of dried KBr powder and then pressed into a disc for analysis. The spectrum was measured with a Nicolet iS 10 FT-IR spectrometer (Thermo Fisher, USA) in the frequency range of 4000–500 cm−1.21
2.6 The rheological characterization of EJP
The rheological characterization of EJP was performed according to a reported method. It was determined with a Discovery Hybrid Rheometer-1 (DHR-1, TA Instruments, New Castle, DE, USA) equipped with parallel steel plates at a certain concentration (0.2% and 0.4%, w/v).23
2.7
In vitro evaluation of EJP on myocardial ischemia injury
2.7.1 Cell culture.
H9c2 cells (American Type Culture Collection) were cultured in Dulbecco's modified Eagle's medium supplemented with 10% fetal bovine serum, 100 U mL−1 penicillin, 100 μg L−1 streptomycin and 110 mg L−1 sodium pyruvate under a humidified atmosphere containing 5% CO2 at 37 °C.
2.7.2 MTT assay.
H9c2 cells were seeded in 96-well plates at a density of 4 × 103 cells per well. MTT (0.5 mg m−1) was added and the cells were incubated for 4 h at 37 °C prior to the addition of dimethyl sulfoxide. The absorbance was measured at a wavelength of 490 nm according to the manufacturer's protocol (SpectraMax® M2/M2e Multiskan; Molecular Devices LLC, Sunnyvale, CA, USA). All experiments were performed in quintuplicate.
2.7.3 Annexin V-FITC/PI flow cytometry for apoptosis detection.
After apoptosis induction, the cells were digested with 0.25 % trypsin and centrifuged at 2000g for 3 min at 4 °C, resuspended in pre-chilled PBS, and centrifuged again at 2000g for 3 min at 4 °C. The supernatant was discarded, and Annexin V-FITC, PI, and binding buffer were added to the cell precipitate. After incubation for 10 min at 4 °C protected from light, cell fluorescence was detected by flow cytometry. For data analysis, Annexin V-FITC+PI-subpopulation was considered early-stage apoptosis and Annexin V-FITC+PI+ was late-stage apoptosis or necrosis. Cell proportion comparisons between treatment and control groups were done in both early- and late-stage subpopulations separately and in total.
2.7.4 Western blot analysis.
After extracted proteins were quantified, equivalent protein amounts were separated by SDS-PAGE and subsequently transferred to a PVDF membrane. After the blocking step, the membranes were incubated with primary antibodies at 4 °C overnight. Then membranes were incubated with secondary antibodies for 2 h at room temperature. Finally, chemiluminescence signals were detected with a ChemiDoc™ Imager.
2.7.5 Mitochondrial membrane potential assay.
The mitochondrial membrane potential (MMP) was assessed using a commercial kit (Beyotime, China) following the manufacturer's instructions.
2.8
In vivo evaluation of EJP on myocardial ischemia injury
2.8.1 Ischemic reperfusion model.
Male C57BL/6 mice (10 weeks old) were provided by Jinan Pengyue Experimental Animal Breeding Co. (Jinan, China). All procedures were performed in strict accordance with the NIH guidelines for the care and use of laboratory animals (NIH Publication no. 8023, revised 1978) and followed the regulations for the Care and Use of Laboratory Animals of the National Institute of Animal Health and the Guidance by the ethics committee of Qingdao University (animal welfare assurance number: 14-0027). The mice were divided into different groups including the control, I/R, and I/R and EJP groups (20 and 50 mg kg−1, i.g.). EJP was administered by intragastric administration (i.g.) 1 h before I/R operation. The mice were anesthetized and the left anterior descending coronary arteries were occluded by a knot to create ischemia for 30 min followed by 6 h of reperfusion. At the end of reperfusion, blood samples were collected, and the organs including the heart and kidneys were also isolated for further experiments.
2.8.2 Measurement by echocardiography.
After 24 h of I/R surgery, transthoracic echocardiography was performed non-invasively with a high-resolution ultrasound imaging system (Vevo2100; VisualSonics, Inc., Canada) equipped with a 30 MHz mechanical transducer. The mice were lightly anesthetized with 2% isoflurane/100% oxygen and placed on a warming platform for the duration of the recordings. Two-dimensional guided M-mode echocardiography was performed in the parasternal long-axis view. Ejection fraction (EF), fractional shortening (FS), left ventricular end-diastole volume (LVEDV) and the left ventricular end-systolic volume (LVESV) were measured. M-Mode and Doppler measurement data represent 3–6 averaged cardiac cycles from at least two scans per mouse.
2.8.3 TTC & Evans blue double-staining.
After reperfusion for 24 h, Evans blue dye (1 ml of a 2.0% solution; Sigma-Aldrich, St Louis, MO, USA) was injected into the jugular vein. Then, the hearts were rapidly excised and sectioned at 1 mm thickness with incubation in 1.0% 2,3,5-triphenyltetrazolium chloride (Sigma-Aldrich, St Louis, MO, USA) for 15 min at 37 °C. The infarcted myocardium is stained white, with the risk zone stained red, and the normal tissue remained blue. The areas of infarction (INF) and nonischemic left ventricle (LV) were determined by computerized planimetry using an image analysis software (NIH 1.57 Image Software).
2.8.4 H&E staining.
The tissues of the heart and kidneys were harvested and fixed in 10% formaldehyde, then they were embedded in paraffin and sliced after dehydration in gradient concentrations of alcohol. The tissue sections were stained with H&E according to the instructions and then captured using a light microscope (Olympus IX73, Japan).
2.8.5 Detection of biochemical indexes.
The blood samples from the abdominal aortic arteries were centrifuged (3000g) at 4 °C for 20 min. Then, a series of biochemical indexes including myocardial LDH, CK-MB, SOD, MDA, GSH-Px, BUN and creatinine were measured using commercial kits according to the instructions from the manufacturers. The levels of TNF-α and IL-6 in serum and in the supernatant were also measured using the corresponding ELISA kits (4A Biotech Co., Ltd, Beijing).
2.9 Statistical analysis
Data are presented as mean ± standard deviation (SD). Statistical analyses were performed using two-way ANOVA (GraphPad Prism 7). Data significance was set at *, p < 0.05, **, p < 0.01 and ***, p < 0.001, respectively.
3. Results and discussion
3.1 Molecular weight of EJP
According to our previously published protocol, we isolated a polysaccharide from the leaf of EJ and removed proteins using the Sevag method several times. We obtained the polysaccharide fraction after purification with ultrafiltration at 8000–14
000 kDa (Fig. 1A). We acquired the polysaccharide, namely EJP. The morphology of EJP was observed by SEM, shown in Fig. 1B. Then, the determination of molecular weights was performed, which could help investigate the purification of EJP. The results in Fig. 1C and D demonstrated that EJP was composed of two components. The molecular weight of the first component was 0.53 × 106 Da and that of the second component was 0.099 × 106 Da. The molecular weight distributions were 1.78 and 1.12, which corresponded to the molecular weight spectrum.
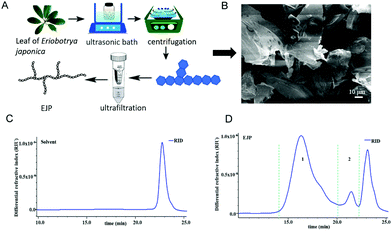 |
| Fig. 1 (A) The scheme to obtain EJP polysaccharide; (B) the SEM graph of EJP; (C) and (D) high performance size exclusion chromatogram of EJP. RIU – refractive index unit. | |
3.2 Compositional analysis of EJP
After the molecular weight of EJP was determined, we set out to examine the monosaccharide composition of EJP. In the results, the retention time of each peak was compared to that of a standard monosaccharide, which revealed the monosaccharide composition of EJP. After analysis and calculation of the results, we confirmed that EJP was mainly composed of mannose, arabinose, rhamnose, galactose, galacturonic acid and glucose, with a molar ratio of 0.82
:
0.9
:
1
:
0.61
:
0.72
:
0.51 (Fig. 2 and Table 1).
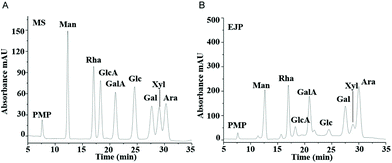 |
| Fig. 2 The high performance liquid chromatograms of standard monosaccharides (A) and EJP polysaccharide (B). | |
Table 1 Molecular weight (Mw), polydispersity (Mw/Mn), and constituent monosaccharides of EJP
|
EJP |
Total polysaccharides (%) |
95.1 |
M
w × 106 (Da) |
|
Fraction 1 |
0.531 (±0.168%) g |
Fraction 2 |
0.099 (±1.154%) g |
M
w/Mn |
|
Fraction 1 |
1.78 (±0.379%) |
Fraction 2 |
1.12 (±1.654%) |
Monosaccharides and molar ratios |
|
Rha |
1.00 |
Man |
0.82 |
GalA |
0.72 |
Ara |
0.90 |
Gal |
0.61 |
GlcA |
0.16 |
Glc |
0.51 |
Xyl |
0.24 |
3.3 FT-IR analysis and rheological properties of EJP
The IR spectrum of EJP was measured and analyzed. The results showed that the absorption peaks of 3200 cm−1 and 3600 cm−1 belonged to –OH. The absorption peaks in the range of 3000–2800 cm−1 belonged to C–H, including CH, CH2 and CH3 tensile vibrations. The absorption peak at 1733.06 cm−1 was related to the tensile vibration of the esterified carboxyl group (–COOR). In addition, the strong absorption peak appearing at 1616.94 cm−1 corresponded to the asymmetric stretching of COO–C
O, indicating that EJP was an acid polysaccharide. The absorption peak at 1417.49 cm−1 was attributed to the bending vibration of C–H or O–H. In addition, the absorption peak at 1250.04 cm−1 corresponded to asymmetric COC tensile vibration, indicating the presence of –OCH3. No typical protein absorption peaks were detected at 1651 cm−1 and 1555 cm−1, which indicated that there was little protein in EJP (Fig. 3A).
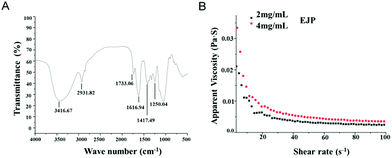 |
| Fig. 3 FT-IR spectra (A) and apparent viscosity (B) of EJP polysaccharide. | |
The rheological properties of EJP were measured and the EJP solution showed non-Newtonian shear thinning behavior in a low shear rate range (0.01–50 s−1). However, the Newtonian flow characteristics (50–100 s−1) are almost reached at high shear rates. With higher concentration, the solution showed higher viscosity (Fig. 3B).
3.4 Protective effect of EJP against oxidative damage in vitro
Myocardial infarction is accompanied by the cellular death of a segment of the heart muscle. Many studies have focused on apoptotic cell death in the heart. In our study, H2O2 (500 μM) was selected to induce myocyte damage for 4 h. First, we found that EJP with a 200 μM concentration has no cell cytotoxicity on H9c2 cells (Fig. 4A) and was selected for the following experiments. Then, EJP protected H9c2 death in response to H2O2 stimulation identified by a series of assays. Firstly, cells’ apoptotic ratio was evaluated by Annexin V/PI double-staining assay and apoptosis-associated proteins were detected by western blotting technology. As shown in results, in the control group, the membrane integrity of H9c2 remains intact (Annexin V−/PI−) while the membrane integrity was significantly disrupted with H2O2 stimulation (Annexin V+/PI+) and partially restored with EJP pretreatment. Furthermore, H2O2 induced cleavage of caspase 3 and decreased expression of Bcl-2, which were obviously reversed by EJP pretreatment (Fig. 4B–D). Besides, JC-1 staining was carried out to detect the mitochondrial membrane potential (ΔΨm) (MMP). In H2O2-damaged cells, decreased red fluorescence and increased green fluorescence were observed indicating the decrease of MMP, while this phenomenon was partially reversed with EJP pretreatment (Fig. 4E).
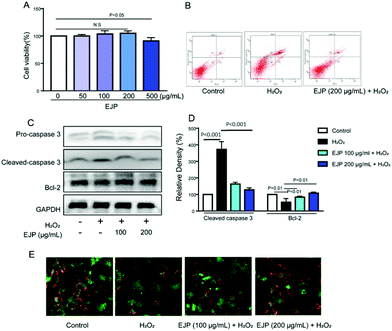 |
| Fig. 4 The effect of EJP polysaccharide on cell viability (A) and cell apoptosis (B) by using flow cytometry; the related proteins including caspase 3 and Bcl-2 (C) were detected by western blotting; the quantitative analysis was also performed (D); the mitochondrial membrane permeabilization was detected with JC-1 staining (E). | |
3.5 Protective effect of EJP against MIRI in vivo
Food-derived polysaccharides show versatile pharmacological activities and do not arouse allergic reactions. Due to the unique advantages, polysaccharides have drawn much attention in many fields.24,25 In our study, according to the network pharmacology prediction, we can conclude that EJ has promising potential for relieving heart diseases (Fig. 5A), and the role of EJP in the pathogenesis of myocardial infarction was studied. Firstly, the area of myocardial necrosis (Fig. 5D and E) and the levels of CK-MB isoenzyme (Fig. 5B) and LDH (Fig. 5C) were detected in I/R mice. The results indicated that EJP exhibited a stronger effect on relieving myocardial infarction with the increasing concentration of EJP. Except for reducing infarct size, EJP could also suppress the release of risk factors and ameliorate myocardial morphological changes (Fig. 5D and E).
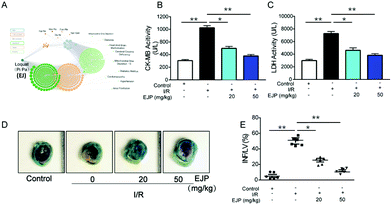 |
| Fig. 5 Data from the network pharmacology analysis of Eriobotrya japonica (A); mice were subjected to 45 min ischemia and 24 h reperfusion. Then serum was collected and CK-MB (B) and LDH (C) were detected with biochemical test kits; hearts were isolated, and infarct size was analyzed by TTC staining (D) and quantified using computerized planimetry (E). | |
3.6 The detection of cardiac function
We further investigated cardiac function after I/R injury. Echocardiography is an accurate noninvasive method for objectively quantifying regional myocardial function including fractional shortening (FS), ejection fraction (EF), left ventricular end-systolic volume (LVESV) and left ventricular end-diastolic volume (LVEDV). As shown in Fig. 6, the representative ultrasonic cardiogram indicated that ventricular systolic function recovered in the EJP group (Fig. 6A). Decreased EF (Fig. 6B) and FS values (Fig. 6C) as well as elevated LVEDV and LVESV values (Fig. 6D and E) were observed in I/R mice hinting the high risk of heart failure. After EJP pretreatment, these phenomena were noticeably reversed. In sum, our results suggested that EJP is protective against I/R injury in mice.
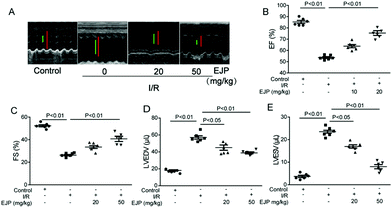 |
| Fig. 6 The cardiovascular ultrasound imaging (A) was performed and cardiac function evaluation indexes EF (B), FS (C), LVEDV (D) and LVESV (E) were detected by transthoracic echocardiography. | |
3.7 Antioxidant and anti-inflammatory effects of EJP in vivo
For ischemia, a lot of oxidative free radicals were observed immediately after the recovery of blood supply in the ischemic myocardium.26,27 Oxygen species are highly reactive and finally aggravate myocardial injury and cardiomyocyte death.28,29 In this process, SOD and GSH-Px are two vital indicators of free radical scavenging while MDA is a biomarker for evaluating oxidative stress.30 We detected the relative indexes and found that in I/R mice, EJP could increase SOD and GSH-Px activities (Fig. 7D and E) and decrease the level of MDA (Fig. 7C). The result showed that EJP significantly rebalanced these changes and exerted a cardioprotective effect associated with reduced oxidant stress. Meanwhile, the secretion of major inflammatory cytokines TNF-α (Fig. 7A) and IL-6 (Fig. 7B) was significantly decreased in serum after EJP pretreatment, which demonstrated its potential anti-inflammatory activity.
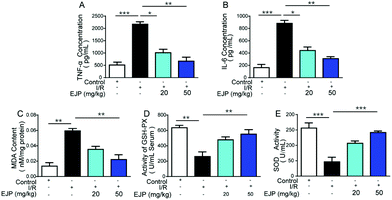 |
| Fig. 7 The effect of EJP on oxidative stress and inflammation induced by I/R. The blood samples were collected from the abdominal aortic artery and the major inflammatory cytokines TNF-α (A) and IL-6 (B) were detected with ELISA kits, and oxidative stress markers MDA (C), GSH-Px (D), and SOD (E) were evaluated with biochemical test kits. | |
At present, a large volume of research suggests that immune-regulatory polysaccharides may interact with macrophages and regulate these cells in a diverse way. Some polysaccharides interact with the TLR4/MD-2 complex, which leads to the activation of intracellular signaling pathways (MAPKs and NF-κB). Other polysaccharides may interact with CR3 and mannose receptors. In our research, we speculated that the potential mechanism of anti-inflammatory activity of EJP was mainly through the activation of the above proteins. In addition, EJP showed an antioxidant effect, which may be attributed to the reductive groups of the structure. Meanwhile, EJP, as a polysaccharide, showed different effects with the change of dose, which exerted the anti-oxidative and anti-inflammatory effects in vitro and in vivo in a dose dependent manner.
3.8 Protective effect of EJP against acute renal injury induced by IR in mice
More studies demonstrated that reperfusion not only aggravated irreversible cardiomyocyte death and enlarged infarcted zone but also induced serious complications. Among these, acute kidney injury is one inevitable and serious syndrome.31,32 To evaluate the effect of EJP on kidney injury, the levels of BUN and creatinine reflecting the renal function were tested. The results showed that the levels were higher in the I/R group than in the control group, while EJP significantly decreased their secretion (Fig. 8B and C). In addition, H&E staining was performed to detect the histologic changes of kidneys (Fig. 8A). Compared with the normal kidney tissues, glomerular atrophy and vacuolization were obviously observed in the I/R group while EJP evidently attenuated I/R-induced tubular injury. The results showed that EJP had a potential effect on alleviating nephrotoxicity after the myocardial I/R injury.
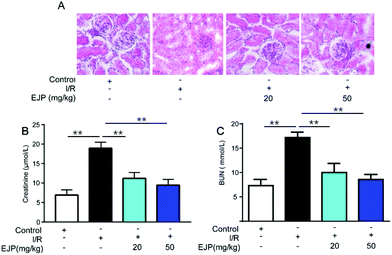 |
| Fig. 8 The effect of EJP on acute renal injury secondary to I/R injury. The morphological changes of the kidneys were assessed by HE staining (A) and the renal function was evaluated by detecting creatinine (B) and BUN (C). BUN, blood urea nitrogen; I/R, ischemia reperfusion. | |
4. Conclusions
In our research, we obtained a food-derived polysaccharide, namely EJP, and confirmed its cardioprotective effects. We first characterized the physicochemical properties of this polysaccharide, and further validated its cardioprotective effects both in vitro and in vivo. EJP not only alleviated oxidative damage in vitro, but also reduced myocardial infarction sizes and improved cardiac function in ischemia-reperfusion mice via anti-oxidation and anti-inflammation. In sum, EJP, with demonstrated cardioprotective ability, has the potential to become a valuable candidate as a cardioprotective drug.
Author contributions
Conceptualization – Qiu Li; data curation – Xiaoli Huang, Wei Pan and Ranran Hou; formal analysis – Xiaoli Huang, Wei Pan, Ranran Hou and Dingtao Wu; writing – original draft – Xiaoli Huang, Wei Pan, Ranran Hou and Qiu Li.
Conflicts of interest
There are no conflicts to declare.
Acknowledgements
This study was financially supported by the National Natural Science Foundation of China (NSFC 82003922), the Key Research and Discovery Program of Shandong Province (2019GSF107072), the Natural Science Foundation of Shandong (ZR2020QH322), and the High-Level Talent Research Foundation of Qingdao Agricultural University, China (1119007).
References
- M. Yin, Y. Zhang and H. Li, Advances in research on immunoregulation of macrophages by plant polysaccharides, Front. Immunol., 2019, 10, 145 CrossRef CAS PubMed.
- F. Chen and G. Huang, Preparation and immunological activity of polysaccharides and their derivatives, Int. J. Biol. Macromol., 2018, 112, 211–216 CrossRef CAS PubMed.
- G. Liu, J. Zhang, T. Hou, S. An, B. Guo, C. Liu, L. Hu, Y. Huang, S. Zhang, M. J. F. Song and Function, Extraction kinetics, physicochemical properties and immunomodulatory activity of the novel continuous phase transition extraction of polysaccharides from Ganoderma lucidum, Food Funct., 2021, 12, 9708–9718 RSC.
- N. Liu, X. Chen, J. Song, M. Chen, P. Gong, W. Jia, G. J. F. Li and Function, Hypoglycemic effects of Auricularia auricula polysaccharides on high fat diet and streptozotocin-induced diabetic mice using metabolomics analysis, Food Funct., 2021, 12, 9994–10007 RSC.
- D.-T. Wu, Y. He, M.-X. Fu, R.-Y. Gan, Y.-C. Hu, L.-X. Peng, G. Zhao and L. Zou, Structural characteristics and biological activities of a pectic-polysaccharide from okra affected by ultrasound assisted metal-free Fenton reaction, Food Hydrocolloids, 2022, 122, 107085 CrossRef CAS.
- C. Tang, R. Ding, J. Sun, J. Liu, J. Kan and C. Jin, The impacts of natural polysaccharides on intestinal microbiota and immune responses–a review, Food Funct., 2019, 10, 2290–2312 RSC.
- G. Pang, F. Wang and L. W. Zhang, Dose matters: Direct killing or immunoregulatory effects of natural polysaccharides in cancer treatment, Carbohydr. Polym., 2018, 195, 243–256 CrossRef CAS PubMed.
- Y. Zhao, B. Yan, Z. Wang, M. Li and W. Zhao, Natural polysaccharides with immunomodulatory activities, Mini-Rev. Med. Chem., 2020, 20, 96–106 CrossRef CAS PubMed.
- L. Huang, M. Shen, G. A. Morris and J. Xie, Sulfated polysaccharides: Immunomodulation and signaling mechanisms, Trends Food Sci. Technol., 2019, 92, 1–11 CrossRef CAS.
- R. Zhong, X. Wan, D. Wang, C. Zhao, D. Liu, L. Gao, M. Wang, C. Wu, S. M. Nabavid and M. Daglia, Polysaccharides from marine Enteromorpha: Structure and function, Trends Food Sci. Technol., 2020, 99, 11–20 CrossRef CAS.
- Y. Yu, M. Shen, Z. Wang, Y. Wang, M. Xie and J. Xie, Sulfated polysaccharide from Cyclocarya paliurus enhances the immunomodulatory activity of macrophages, Carbohydr. Polym., 2017, 174, 669–676 CrossRef CAS PubMed.
- Y. Wang, M. Kwak, P. C.-W. Lee and J.-O. Jin, Rehmannia glutinosa polysaccharide promoted activation of human dendritic cells, Int. J. Biol. Macromol., 2018, 116, 232–238 CrossRef CAS PubMed.
- G. Benchamas, S. Huang and G. Huang, The influence of traditional and new processing technologies on the structure and function of food polysaccharide, Food Funct., 2020, 11, 5718–5725 RSC.
- C. Cao, B. Zhu, Z. Liu, X. Wang, C. Ai, G. Gong, M. Hu, L. Huang and S. Song, An arabinogalactan from Lycium barbarum attenuates DSS-induced chronic colitis in C57BL/6J mice associated with the modulation of intestinal barrier function and gut microbiota, Food Funct., 2021, 12, 9829–9843 RSC.
- J. L. Anderson and D. A. Morrow, Acute myocardial infarction, N. Engl. J. Med., 2017, 376, 2053–2064 CrossRef CAS PubMed.
- D. Sueta, N. Tabata and S. Hokimoto, Clinical roles of calcium channel blockers in ischemic heart diseases, Hypertens. Res., 2017, 40, 423–428 CrossRef CAS PubMed.
- S. Vallabhajosyula, L. Ya'Qoub, S. M. Dunlay, S. Vallabhajosyula, S. Vallabhajosyula, P. R. Sundaragiri, A. S. Jaffe, B. J. Gersh and K. Kashani, Sex disparities in acute kidney injury complicating acute myocardial infarction with cardiogenic shock, ESC Heart Fail., 2019, 6, 874–877 CrossRef PubMed.
- Y. Zhang, C. Li, H. Meng, D. Guo, Q. Zhang, W. Lu, Q. Wang, Y. Wang and P. Tu, BYD ameliorates oxidative stress-induced myocardial apoptosis in heart failure post-acute myocardial infarction via the P38 MAPK-CRYAB signaling pathway, Front. Physiol., 2018, 9, 505 CrossRef PubMed.
- L. Hou, J. Guo, F. Xu, X. Weng, W. Yue and J. Ge, Cardiomyocyte dimethylarginine dimethylaminohydrolase1 attenuates left-ventricular remodeling after acute myocardial infarction: involvement in oxidative stress and apoptosis, Basic Res. Cardiol., 2018, 113, 1–12 CrossRef CAS PubMed.
- S. J. Forrester, D. S. Kikuchi, M. S. Hernandes, Q. Xu and K. K. Griendling, Reactive oxygen species in metabolic and inflammatory signaling, Circ. Res., 2018, 122, 877–902 CrossRef CAS PubMed.
- Y. Fu, Q. Yuan, S. Lin, W. Liu, G. Du, L. Zhao, Q. Zhang, D.-R. Lin, Y.-T. Liu, W. Qin, D.-Q. Li and D.-T. Wu, Physicochemical characteristics and biological activities of polysaccharides from the leaves of different loquat (Eriobotrya japonica) cultivars, Int. J. Biol. Macromol., 2019, 135, 274–281 CrossRef CAS PubMed.
- X. Huang, N. Guan and Q. Li, A Marine-Derived Anti-Inflammatory Scaffold for Accelerating Skin Repair in Diabetic Mice, Mar. Drugs, 2021, 19, 496 CrossRef CAS PubMed.
- X.-R. Nie, H.-Y. Li, G. Du, S. Lin, R. Hu, H.-Y. Li, L. Zhao, Q. Zhang, H. Chen, D.-T. Wu and W. Qin, Structural characteristics, rheological properties, and biological activities of polysaccharides from different cultivars of okra (Abelmoschus esculentus) collected in China, Int. J. Biol. Macromol., 2019, 139, 459–467 CrossRef CAS.
- V. Jesumani, H. Du, P. Pei, C. Zheng, K. Cheong and N. Huang, Unravelling property of polysaccharides from Sargassum sp. as an anti-wrinkle and skin whitening property, Int. J. Biol. Macromol., 2019, 140, 216–224 CrossRef CAS.
- S. Vandghanooni and M. Eskandani, Electrically conductive biomaterials based on natural polysaccharides: Challenges and applications in tissue engineering, Int. J. Biol. Macromol., 2019, 141, 636–662 CrossRef CAS PubMed.
- T. Carbonell and A. Gomes, MicroRNAs in the regulation of cellular redox status and its implications in myocardial ischemia-reperfusion injury, Redox Biol., 2020, 36, 101607 CrossRef CAS PubMed.
- C. Hu, X. Zhang, N. Zhang, W. Wei, L. Li, Z. Ma and Q. Tang, Osteocrin attenuates inflammation, oxidative stress, apoptosis, and cardiac dysfunction in doxorubicin-induced cardiotoxicity, Clin. Transl. Med., 2020, 10(3), e124 Search PubMed.
- C. Sinning, D. Westermann and P. Clemmensen, Oxidative stress in ischemia and reperfusion: current concepts, novel ideas and future perspectives, Biomarkers Med., 2017, 11, 11031–11040 CrossRef PubMed.
- T. Zhou, E. Prather, D. Garrison and L. Zuo, Interplay between ROS and Antioxidants during Ischemia-Reperfusion Injuries in Cardiac and Skeletal Muscle, Int. J. Mol. Sci., 2018, 19(2), 417 CrossRef PubMed.
- J. Zińczuk, M. Maciejczyk, K. Zaręba, A. Pryczynicz, V. Dymicka-Piekarska, J. Kamińska, O. Koper-Lenkiewicz, J. Matowicka-Karna, B. Kędra, A. Zalewska and K. Guzińska-Ustymowicz, Pro-Oxidant Enzymes, Redox Balance and Oxidative Damage to Proteins, Lipids and DNA in Colorectal Cancer Tissue. Is Oxidative Stress Dependent on Tumour Budding and Inflammatory Infiltration?, Cancers, 2020, 12(6), 1636 CrossRef PubMed.
- Y. Hu, Z. Mao, L. Xu, L. Yin, X. Tao, Z. Tang, Y. Qi, P. Sun and J. Peng, Protective effect of dioscin against intestinal ischemia/reperfusion injury via adjusting miR-351-5p-mediated oxidative stress, Pharmacol. Res., 2018, 137, 56–63 CrossRef CAS PubMed.
- C. Tang, Y. Hu, J. Gao, J. Jiang, S. Shi, J. Wang, Q. Geng, X. Liang and X. Chai, Dexmedetomidine pretreatment attenuates myocardial ischemia reperfusion induced acute kidney injury and endoplasmic reticulum stress in human and rat, Life Sci., 2020, 257, 118004 CrossRef CAS PubMed.
|
This journal is © The Royal Society of Chemistry 2022 |