DOI:
10.1039/D1FO03194E
(Paper)
Food Funct., 2022,
13, 121-130
Mogroside-rich extract from Siraitia grosvenorii fruits protects against the depletion of ovarian reserves in aging mice by ameliorating inflammatory stress†
Received
23rd September 2021
, Accepted 5th December 2021
First published on 6th December 2021
Abstract
Mogroside-rich extract (MGE), the main bioactive component of dried Siraitia grosvenorii fruit, has long been used as a natural sweetener and traditional Chinese medicine. This extract possesses various types of pharmacological activities, such as anti-inflammatory, antioxidative, hypoglycemic and hypolipemic activities. Moreover, we recently revealed that MGE has beneficial effects on female reproduction. Increasing maternal age leads to a rapid reduction in female fertility; in particular, it dramatically decreases ovarian function. Nevertheless, whether MGE can alleviate ovarian aging and the underlying mechanisms have not yet been explored. In this study, mice were treated with MGE by supplementation in drinking water from 10 to 44 weeks of age. Then, ovarian function and molecular changes were determined. Our findings showed that MGE treatment protected aged mice from estrous cycle disorder. Moreover, MGE treatment significantly increased the ovarian reserves of aged mice. RNA-seq data showed that MGE upregulated the expression of genes related to gonad development, follicular development, and hormone secretion in ovarian tissue. Additionally, inflammatory stress was induced, as indicated by upregulation of inflammation-related gene expression and elevated TNF-α levels in the ovarian tissues of aged mice; however, MGE treatment attenuated inflammatory stress. In summary, our findings demonstrate that MGE can ameliorate age-related estrous cycle disorder and ovarian reserve decline in mice, possibly by alleviating ovarian inflammatory stress.
1. Introduction
Increasing maternal age contributes to the decline in female fertility, which is mainly attributed to abnormalities in ovarian function.1,2 As the organs most sensitive to aging, the ovaries undergo significant functional decline after 35 years of age in humans.3 Maternal age-related ovarian dysfunction is associated with significant decreases in ovarian reserves and the quantity and quality of oocytes as well as irregular ovulatory cycles,4 which can lead to a series of severe gynecological symptoms, such as menstrual disorders, infertility, fetal anomalies, and obstetric complications.5 Given its severe impact on female reproduction, multiple therapeutic strategies have been employed to alleviate age-related impairment of ovarian function.6–8 Among them, strategies involving herbal extracts have attracted public attention due to the safety, convenience, and cost-effectiveness of these agents.9,10
Siraitia grosvenorii is an edible and medicinal plant principally cultivated in southern China,11 whose fruits are used to make traditional hot drinks for treating cough, sore throat, asthma, bronchitis, and constipation.12 Mogroside-rich extract (MGE), the main active ingredient of Siraitia grosvenorii fruits, is a natural zero-calorie sweetener with high sweetness and stable physical and chemical properties.13 Its main bioactive substances are a group of cucurbitane-type triterpenoid glycosides, of which mogroside V (MV) accounts for the largest proportion.14 Modern pharmacological studies have shown that MGE possesses anti-inflammatory,15 antioxidation,16 antiobesity,17 antidiabetic,18 and anticarcinogenic effects.19 MGE can ameliorate inflammatory injury in many organs; for example, it improves neuroinflammation,20 ulcerative colitis,15 and atopic dermatitis.21 Moreover, MGE can attenuate high-fat diet-induced obesity,22 diabetes,23 and nonalcoholic fatty liver disease24 through its strong hypoglycemic and hypolipidemic activities.
Regarding female reproduction, we have found that MV can promote porcine oocyte in vitro maturation and subsequent embryo development25 and ameliorate lipopolysaccharide (LPS)-induced porcine oocyte meiotic defects.26 Furthermore, MV alleviates meiotic defects and quality deterioration in oocytes from benzo(a)pyrene-exposed mice.27 More importantly, MV delays the deterioration of porcine oocyte quality during in vitro aging.28 Taken together, the data indicate that MGE not only attenuates inflammatory injury and metabolic disturbance but also ameliorates oocyte quality. However, the role of MGE in ovarian dysfunction due to physiological aging remains unknown.
Hence, we aimed to determine whether long-term MGE treatment can alleviate the decline in ovarian function in physiologically aging mice. We treated 10-week-old female mice with MGE until 44 weeks of age and then observed the estrous cycle and ovarian follicle reserves. To reveal the protective mechanisms of MGE, ovarian RNA was extracted to perform RNA sequencing (RNA-seq). Our work may provide a new reference for the treatment of age-related female infertility.
2. Materials and methods
2.1. Animals
The experiments were approved by the Institutional Animal Care and Use Committee (IACUC) of Guangxi University in Nanning and conducted in accordance with the National Institutes of Health Guidelines for the Care and Use of Laboratory Animals. Female Institute for Cancer Research (ICR) mice (8 to 9 weeks old) were purchased from Guangxi Medical University (GXU2020-015). The mice were housed at ambient temperature (22–25 °C) and 50% relative humidity with a standard 12 h light-dark cycle. Food and water were available ad libitum.
2.2. Experimental design and sample collection
After one week of adaptation, the mice were randomly divided into two groups: the Old and Old/MGE groups. The Old/MGE group mice received MGE (600 mg per kg per day) in drinking water until 44 weeks of age.29 The dose of MGE was selected based on a published report23,24 and our preliminary screening. Water bottles were covered with aluminum foil to protect solution from light. Meanwhile, the Old group mice were given only water without treatment. Ten-week-old female mice served as the Young group. At the end of treatment, mice in the Young, Old and Old/MGE groups were euthanized, and their ovaries were removed. The right ovaries were snap-frozen in liquid nitrogen and stored at −80 °C for further analyses, and the other ovaries were fixed in 10% neutral formalin for histological staining.
MGE was purchased from Layn Biotech (Guilin, China). Its main constituents were identified by the colorimetric method and HPLC method, which consisted of moisture 7.87%, total phenolics 0.61%, total mogrosides 79.62%, mogroside V 48.86%, 11-O-mogroside V 7.01%, mogroside IV 1.61%, mogroside III 0.30% and mogroside IIA2 0.05%. Commercial standards (purity ≥ 97%) were obtained from Chengdu Must Biotech (Chengdu, China).
2.3. Estrous cycle analysis
When the mice were 42 weeks old, the estrous cycle was assessed by cytological examination of vaginal smears for 8 consecutive days. Vaginal secretions were collected at 08:00–09:00 every morning for microscopic examination. The stages of the mouse estrous cycle were determined by analyzing the proportions of major cell types (epithelial cells, cornified cells, and leukocytes) according to previously described methods.30
2.4. Hematoxylin and eosin (H&E) staining and follicle counting
Ovarian tissues were fixed in 10% neutral formalin for 16 h at 4 °C and then dehydrated in gradient concentrations of ethanol, cleared in xylene, and embedded in paraffin. Sections (5 μm thickness) were stained with H&E to observe follicular morphology. Ovarian primordial and primary, secondary, preantral, antral, and atretic follicles were classified and counted as previously described.31
2.5. RNA isolation and sequencing
Total RNA of ovarian tissue was isolated using an RNeasy Mini Kit (Qiagen, Germany), and each group had three replicate samples. The technological processes of RNA quantification and qualification, library preparation and quality control, and RNA-seq were performed by Biomarker Technologies Corporation (Beijing, China). The cDNA library was sequenced on an Illumina high-throughput sequencing platform.
2.6. Identification of the differentially expressed genes (DEGs)
Before analyzing the data, high-quality clean data were obtained by removing the reads with low-quality bases and adapter sequences. The clean reads were then aligned to the GRCm38 reference genome using HISAT2 software. The DESeq2 package32 was used to identify DEGs, and a fold change (FC) ≥ 2 and a false discovery rate (FDR) < 0.05 were used as the screening criteria.
2.7. Gene ontology (GO) and kyoto encyclopedia of genes and genomes (KEGG) enrichment analyses
GO enrichment analysis of the DEGs was performed with the R package topGO.33 The Bonferroni method was used to correct the p values. KOBAS software34 was utilized to test the statistical enrichment of DEGs in KEGG pathways. In this process, the p value was obtained by the Benjamin and Hochberg method. GO terms and KEGG pathways with p values <0.05 were identified as significantly enriched.
2.8. Quantitative real time PCR (qRT-PCR)
Total RNA was isolated from the ovaries using an RNeasy Mini Kit (Qiagen, Germany). cDNA was synthesized using a first-strand cDNA synthesis kit (Bio-Rad, USA) following standard protocols. qRT-PCR was performed in a Bio-Rad CFX96 system using an UltraSYBR Mixture kit (CWBIO, China). The relative mRNA levels were determined by the 2−ΔΔCt method. All primer sequences are listed in ESI Table S1.†
2.9. Enzyme-linked immunosorbent assay (ELISA)
The contents of TNF-α in ovarian tissue were measured using commercial mouse ELISA kits (Meimian Biotech, Jiangsu, China) according to the manufacturer's instructions.
2.10. Statistical analyses
The data are shown as the mean ± SEM. Statistical analyses were carried out using Prism 7 software (GraphPad, San Diego, CA, USA). Statistical significance was determined by one-way analysis of variance (ANOVA). P < 0.05 was considered to indicate statistical significance.
3. Results
3.1. Effect of MGE on the estrous cycles of aged mice
To explore the potential impacts of MGE treatment on age-related estrous cycle disorder, we performed daily vaginal cytology for 8 consecutive days. Representative images of vaginal cytology for four different stages of the estrous cycle are shown in Fig. 1A. The detailed vaginal cytology data revealed that the young mice had a normal estrous cycle (4–5 days), while aged mice exhibited an irregular estrous cycle that was mostly stopped in the diestrus phase. However, the estrous cycles of MGE-treated aged mice were similar to those of young mice (Fig. 1B). These results indicate that MGE treatment can combat estrous cycle irregularity in aged mice.
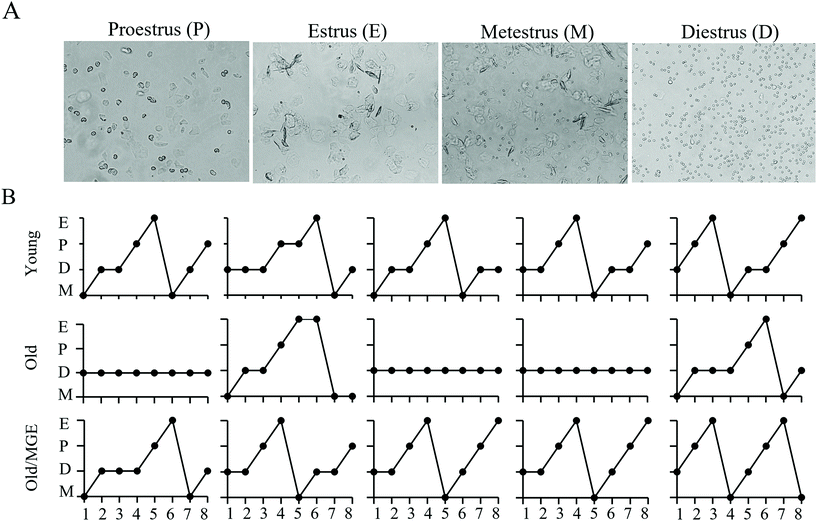 |
| Fig. 1 Effect of MGE on the estrous cycles of aged mice. (A) Example images of different estrous cycle phases: proestrus (P), estrus (E), metestrus (M), and diestrus (D). The estrous cycle stage was determined according to the relative proportion of each cell type. (B) Detailed changes in the estrous cycles of mice from the Young, old, and old/MGE groups. Each grid represents a mouse, and each dot represents a day (n = 5). | |
3.2 Effect of MGE on the ovarian follicle reserves of aged mice
We next assessed the ability of MGE to reverse the age-related decline in ovarian reserves. Representative histological sections of ovaries and the follicle counts showed that the follicle numbers in MGE-treated aged mice were significantly higher than those in nontreated aged mice (P < 0.01), as shown in Fig. 2A and B. Specifically, compared with young mice, aged mice exhibited significant declines in the numbers of primordial and primary follicles, secondary follicles, preantral follicles and antral follicles. And the numbers of atretic follicles of aged mice were significantly higher than those of young mice. However, MGE-treated aged mice showed a 47% increase in the number of primordial and primary follicles. The numbers of secondary and preantral follicles also displayed 1.25-fold and 2.4-fold increases, respectively, after MGE treatment. Antral follicle numbers were also increased by 2.8-fold in aged mice after MGE treatment. However, MGE treatment did not change the number of atretic follicles in aged mice (Fig. 2C). Taken together, our results demonstrate that MGE treatment can restore ovarian reserves in aged mice.
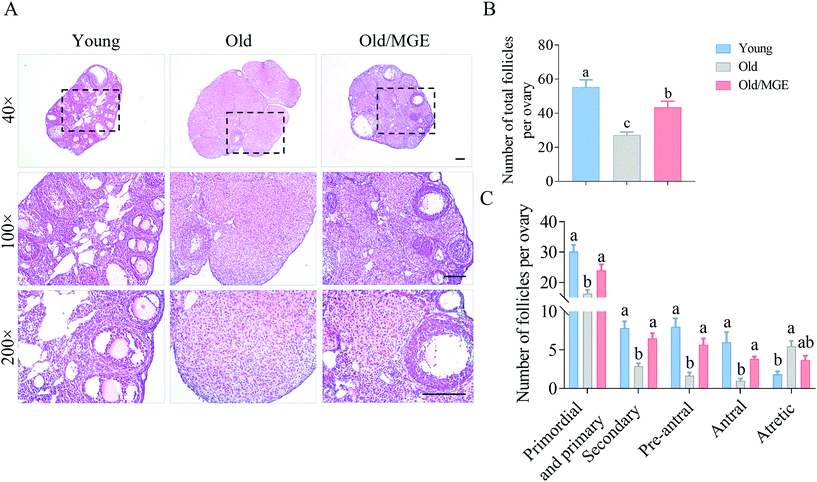 |
| Fig. 2 Effect of MGE on the ovarian follicle reserves of aged mice. (A) Representative H&E-stained images of ovarian tissue sections from the three groups. Scale bar = 100 μm. (B) The total follicle numbers of mice from the three groups (n = 6–9). (C) Follicle dynamics of the mice from the three groups (n = 6–9). The data are shown as the mean ± SEM, and different letters denote significant differences (P < 0.05). | |
3.3 Effects of MGE on the ovarian mRNA expression profiles of aged mice
To further investigate the effect of MGE on gene expression in aged ovaries, we performed RNA-seq on mouse ovaries. Principal component analysis (PCA) of the RNA-seq data revealed that biological replicates clustered together, suggesting that there was high reproducibility among the replicate samples. Moreover, samples from the Old/MGE and Young groups clustered together and were separate from Old group samples. These findings indicated that the transcriptome profiles of the Old/MGE group differed significantly from those of the Old group and were highly similar to those of the Young group (Fig. 3A).
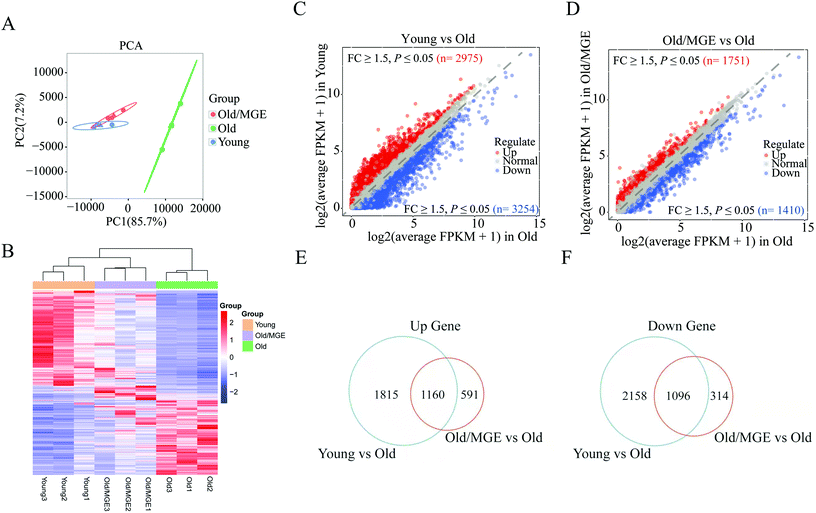 |
| Fig. 3 Effects of MGE on the ovarian mRNA expression profiles of aged mice. (A) Principal component analysis (PCA) of the ovarian transcriptomic data from the three groups (n = 3). (B) The heatmap shows all DEGs in mouse ovaries from the three groups. Red indicates genes with upregulated expression, and blue indicates genes with downregulated expression. (C and D) Scatter plots showing the DEGs identified in young vs. old and old/MGE vs. old. Red and blue dots represent upregulated and downregulated DEGs, respectively. (E and F) Venn diagram analysis of the numbers of overlapping upregulated and downregulated DEGs in the scatter plots for both the Young and old/MGE groups. | |
Heatmaps and volcano plots of the significant DEGs are shown in Fig. 3B–D. According to the transcriptome data, 2975 upregulated and 3254 downregulated DEGs were identified in ovaries from young mice compared to ovaries from aged mice, while 1751 upregulated and 1410 downregulated DEGs were identified in ovaries from MGE-treated aged mice compared to ovaries from aged mice. Venn diagram analysis of all upregulated and downregulated DEGs described above identified 1160 shared upregulated and 1096 shared downregulated DEGs between the Young and Old/MGE groups (Fig. 3E and F).
3.4 GO analysis of DEGs and validation of RNA-seq data by qRT-PCR
To identify the biological processes altered by MGE treatment, GO analysis was performed on shared DEGs. We found that a large number of shared upregulated DEGs were significantly enriched for GO terms associated with ovarian performance and female reproductive hormone secretion, such as gonadal development, ovarian follicle development, female gamete production and development, ovulation, fertilization, steroid hormone biosynthesis, and hormone secretion. Moreover, the shared downregulated DEGs were particularly enriched for inflammation-related GO terms, such as tumor necrosis factor production, leukocyte cell-cell adhesion, and T cell activation (Fig. 4A).
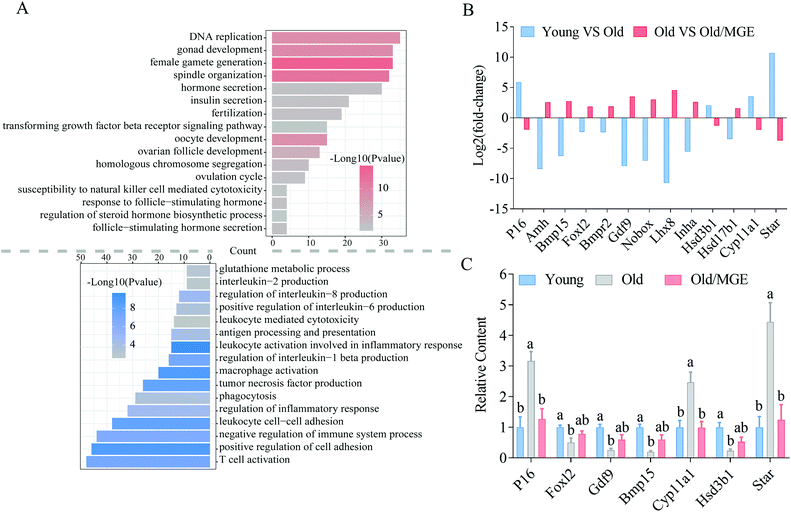 |
| Fig. 4 GO analysis of DEGs and validation of RNA-seq data by qRT-PCR. (A) GO enrichment analysis of the shared upregulated and downregulated DEGs. (B) RNA-seq results of genes related to ovarian performance in young ovaries compared to aged ovaries (blue) and aged ovaries compared to MGE-treated aged ovaries (red). (C) A total of 7 genes were selected to validate the relative mRNA expression by qRT-PCR (n = 4). Data are shown as the mean ± SEM, and different letters denote significant differences (P < 0.05). | |
We then analyzed RNA-seq data for the expression levels of several known genes related to ovarian performance and hormone secretion and found that MGE alleviated the aberrant expression of these genes in ovaries from aged mice (Fig. 4B). Moreover, the reliability and accuracy of the RNA-Seq data were further confirmed by qRT-PCR analysis. These genes were related to cellular senescence (P16), follicular development (Foxl2, Gdf9, and Bmp15), and hormone secretion (Cyp11α1, Hsd3b1, and Star) (Fig. 4C). Collectively, these results imply that MGE can improve ovarian follicle development and female reproductive hormone secretion and ameliorate ovarian inflammatory stress.
3.5 Identification of target effectors of MGE in aged ovaries by KEGG analysis
KEGG analysis was performed to further reveal the main signaling pathways affected by MGE treatment. As shown in Fig. 5A, shared upregulated DEGs were enriched in the cell cycle, progesterone-mediated oocyte maturation, oocyte maturation, the TGF-β signaling pathway, and the PI3K-Akt signaling pathway. The shared downregulated DEGs were mainly related to phagosomes, cell adhesion molecules, antigen processing and presentation, and viral protein interactions with cytokines and cytokine receptors. Moreover, some downregulated signaling pathways were associated with geriatric diseases, such as the AGE-RAGE signaling pathway. Of note, the downregulated KEGG pathways were strongly related to the inflammatory response.
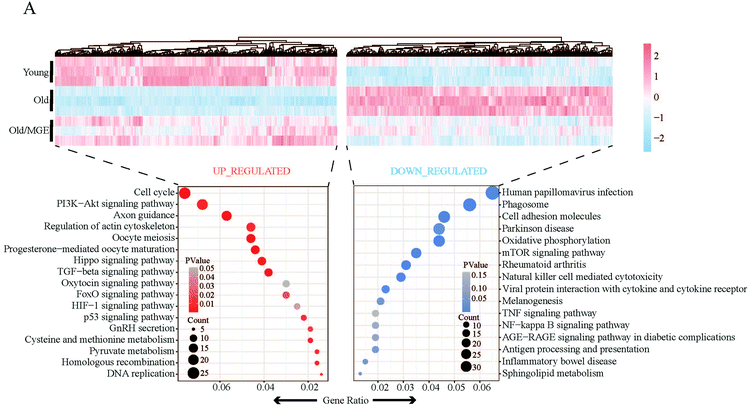 |
| Fig. 5 Identification of target effectors of MGE in aged ovaries by KEGG analysis. (A) Heatmap and KEGG enrichment analysis of the shared upregulated and downregulated DEGs. Red and blue indicate upregulated and downregulated DEGs and pathways, respectively. | |
3.6 MGE treatment ameliorates inflammatory stress in the ovaries of aged mice
Since the downregulated biological processes and signaling pathways were closely related to the inflammatory response, we investigated whether MGE potently protects against age-related ovarian inflammatory stress. The heatmap shown in Fig. 6A displays the expression levels of genes in inflammation-related signaling pathways, including cell adhesion molecule genes, NF-kappa B signaling pathway genes, and TNF signaling pathway genes. Interestingly, the expression levels of all these genes were upregulated in aged ovaries, but the upregulation was significantly alleviated by MGE treatment. The fold changes in the expression of specific inflammation-related genes had similar trends (Fig. 6B).
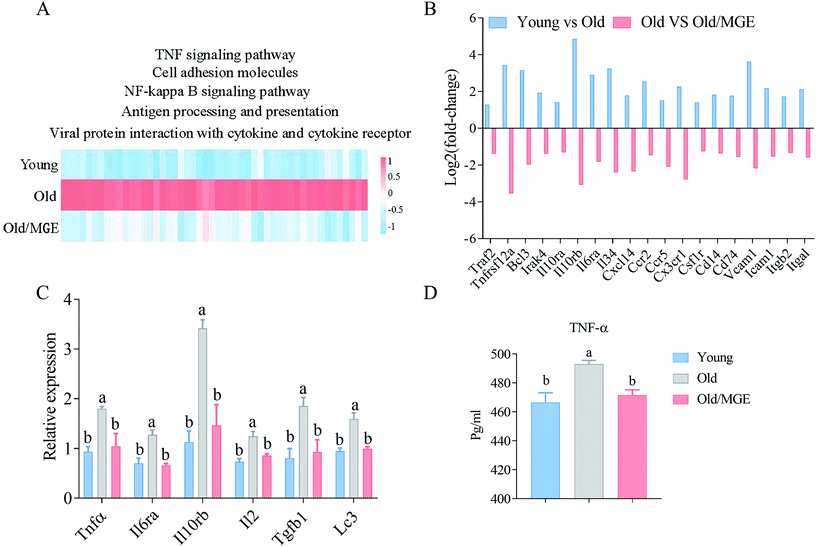 |
| Fig. 6 MGE treatment ameliorates inflammatory stress in the ovaries of aged mice. (A) The heatmap shows the DEGs in inflammation-related pathways of the KEGG analysis. Red and blue indicate upregulated and downregulated DEGs, respectively. (B) RNA-seq results of inflammation-related DEGs in young ovaries compared to aged ovaries (blue) and aged ovaries compared to MGE-treated aged ovaries (red). (C) qRT-PCR analysis of the expression levels of Tnfα, Il6ra, Il10rb, Il2, Tgfb1, and Lc3 in ovaries from the three groups (n = 4). (D) The TNF-α contents in the ovaries from the three groups (n = 5). The data are shown as the mean ± SEM, and different letters denote significant differences (P < 0.05). | |
Furthermore, the relative expression levels of several inflammation-related genes (Tnfα, Il6ra, Il10rb, Il2, Tgfb1, and Lc3) were quantified using qRT-PCR, and the results showed that MGE treatment significantly attenuated the age-related aberrant expression of these genes (Fig. 6C). In addition, the TNF-α levels in ovaries from MGE-treated aged mice were significantly lower than those in ovaries from aged mice (Fig. 6D). Collectively, these findings confirm that MGE alleviates age-related ovarian inflammatory stress in aged mice.
4. Discussion
Delaying ovarian aging is key to maintaining female fertility. In this study, we observed that MGE treatment ameliorated estrous cycle disorder and ovarian reserve decline in aged mice. Moreover, transcriptome data from ovarian tissues further supported a role for MGE in improving gonad development, follicular development, and hormone secretion. Importantly, further analysis confirmed that the abovementioned powerful effect of MGE was associated with the attenuation of inflammatory stress. Hence, we reveal a new function of MGE and preliminarily elucidate the underlying mechanism.
Irregularity of the estrous cycle is an important sign of ovarian failure and reproductive aging.35,36 Accordingly, our results showed that the estrous cycles of aged mice were irregular and arrested primarily at the diestrus stage, but MGE treatment restored the irregular estrous cycles to a considerable degree. Moreover, a drastic reduction in follicular reserves is associated with age-related ovarian dysfunction.37 In this study, we observed significant ovarian reserve decline in aged mice. Notably, MGE treatment increased the total number of follicles in aged mice. Specifically, the numbers of primordial/primary, secondary, preantral, and antral follicles were significantly increased in Old/MGE mice. These results illustrate that MGE can combat estrous cycle disorder and ovarian reserve decline in aged mice.
RNA-seq was performed to identify the potential effector targets of MGE. Our RNA-seq data showed that the gene expression profiles of the Old/MGE group were most similar to those of the Young group. Further GO analysis revealed that MGE upregulated the expression of genes related to gonad development, follicular development, hormone secretion, and female gamete generation in aged ovaries. Furthermore, KEGG analysis showed that these shared upregulated DEGs were enriched in the cell cycle, TGF-β signaling pathway, Hippo signaling pathway, and PI3K-Akt signaling pathway. A series of studies have shown that activation of these signaling pathways inhibits ovarian granulosa cell apoptosis and optimizes the follicle reserves.38–41 Moreover, several well-known genes are critical for cellular senescence (P16),42 follicular development (Foxl2, Gdf9, and Bmp15),43,44 and hormone secretion (Cyp11α1, Hsd3b1, and Star).45,46 As expected, MGE treatment alleviated the aberrant expression of these genes in aged mice. Collectively, the above results indicate that MGE alters gene expression profiles in the ovaries of aged mice and contributes to the improvement of ovarian function.
Ovaries undergo age-related chronic inflammation,47 which inhibits follicle development and ovulation and decreases gamete quality.48–50 Multiple studies have documented that the improvement of ovarian inflammatory conditions can effectively delay age-related ovarian dysfunction.9,51 Our findings showed that the most significantly downregulated GO terms and KEGG pathways after MGE treatment were related to cell adhesion molecules, immune responses, the inflammatory response, phagocytosis, T cell activation, and tumor necrosis factor production. Consistent with our observation, a published transcriptomic study on ovaries from aged mice reported activation of these terms and pathways.52 Additionally, the depletion of ovarian reserves over the reproductive lifespan correlates with increases in the levels of proinflammatory factors.53 As expected, our study showed that TNF-α levels and the relative expression levels of Tnfα, Il6ra, Il10rb, Il2, Tgfb1, and Lc3 were significantly reduced in ovaries from MGE-treated aged mice. Similarly, a previous study found that MGE inhibits LPS-induced inflammation in RAW 264.7 cells.54 In animal experiments, it has been reported that MGE can alleviate 12-O-tetradecanoylphorbol 13-acetate-induced atopic dermatitis,55 improve ovalbumin-induced pulmonary inflammation,56 and attenuate LPS-induced and amyloid β-peptide(1–42)-induced memory impairment and neuroinflammatory responses.20,57 Hence, our findings uncover a new physiological effect of MGE: inhibition of age-related ovarian inflammatory stress.
5. Conclusions
In conclusion, MGE treatment can ameliorate estrous cycle disorder and protect against ovarian reserve decline in aged mice. Our transcriptome data further reveal that MGE enhances gonad development and follicular development and improves steroid hormone biosynthesis. Moreover, the positive effects of MGE may be partly explained by inhibition of ovarian inflammatory stress. To our knowledge, this is the first study evaluating the protective effects of MGE on age-related ovarian dysfunction and preliminarily exploring the underlying mechanisms.
Author contributions
Y. D., X. Y. and X. L. conceived the study. Y. D., J. L., S. L., J. H., S. W., J. L., K. C., K. Y. and N. W. performed the experiments. Y. D., X. Y. and X. L. analyzed the data and wrote the manuscript. All the authors reviewed the manuscript and approved the final version of the paper.
Conflicts of interest
The authors declare that they have no conflicts of interest.
Acknowledgements
This work was supported by the National Natural Science Foundation of China (No. 82160287), the Scientific Research Foundation of Guangxi University (XTZ170099), the Guangxi Natural Science Foundation Program (2018GXNSFAA138125, 2020GXNSFAA159099), the One-hundred Talent Program of Guangxi and the Funding from State Key Laboratory for Conservation and Utilization of Subtropical Agro-bioresources (SKLCUSA-b201913).
References
- M. V. Sauer, Reproduction at an advanced maternal age and maternal health, Fertil. Steril., 2015, 103, 1136–1143 CrossRef PubMed
.
- B. Vollenhoven and S. Hunt, Ovarian ageing and the impact on female fertility, F1000Research, 2018, 7, 1835 CAS
.
- C. J. Li, L. T. Lin, H. W. Tsai, C. U. Chern, Z. H. Wen, P. H. Wang and K. H. Tsui, The Molecular Regulation in the Pathophysiology in Ovarian Aging, Aging Dis., 2021, 12, 934–949 CrossRef PubMed
.
- S. U. Park, L. Walsh and K. M. Berkowitz, Mechanisms of ovarian aging, Reproduction, 2021, 162, R19–R33 CAS
.
- F. J. Broekmans, M. R. Soules and B. C. Fauser, Ovarian aging: mechanisms and clinical consequences, Endocr. Rev., 2009, 30, 465–493 CrossRef CAS PubMed
.
- J. F. Kawwass and M. L. Badell, Maternal and Fetal Risk Associated With Assisted Reproductive Technology, Obstet. Gynecol., 2018, 132, 763–772 CrossRef PubMed
.
- J. Qiao, Z. B. Wang, H. L. Feng, Y. L. Miao, Q. Wang, Y. Yu, Y. C. Wei, J. Yan, W. H. Wang, W. Shen, S. C. Sun, H. Schatten and Q. Y. Sun, The root of reduced fertility in aged women and possible therapentic options: current status and future perspects, Mol. Aspects Med., 2014, 38, 54–85 CrossRef PubMed
.
- Z. Fazeli, A. Abedindo, M. D. Omrani and S. M. H. Ghaderian, Mesenchymal Stem Cells (MSCs) Therapy for Recovery of Fertility: a Systematic Review, Stem Cell Rev. Rep., 2018, 14, 1–12 CrossRef CAS PubMed
.
- Y. Jiang, Z. Zhang, L. Cha, L. Li, D. Zhu, Z. Fang, Z. He, J. Huang and Z. Pan, Resveratrol Plays a Protective Role against Premature Ovarian Failure and Prompts Female Germline Stem Cell Survival, Int. J. Mol. Sci., 2019, 20, 3605 CrossRef CAS PubMed
.
- S. H. Azami, H. Nazarian, M. A. Abdollahifar, F. Eini, M. A. Farsani and M. G. Novin, The antioxidant curcumin postpones ovarian aging in young and middle-aged mice, Reprod., Fertil. Dev., 2020, 32, 292–303 CrossRef CAS PubMed
.
- C. Li, L. M. Lin, F. Sui, Z. M. Wang, H. R. Huo, L. Dai and T. L. Jiang, Chemistry and pharmacology of Siraitia grosvenorii: a review, Chin. J. Nat. Med., 2014, 12, 89–102 CAS
.
- X. Gong, N. Chen, K. Ren, J. Jia, K. Wei, L. Zhang, Y. Lv, J. Wang and M. Li, The Fruits of Siraitia grosvenorii: A Review of a Chinese Food-Medicine, Front. Pharmacol., 2019, 10, 1400 CrossRef CAS PubMed
.
- D. D. Soejarto, E. M. Addo and A. D. Kinghorn, Highly sweet compounds of plant origin: From ethnobotanical observations to wide utilization, J. Ethnopharmacol., 2019, 243, 112056 CrossRef CAS PubMed
.
- C. Liu, L. Dai, Y. Liu, D. Dou, Y. Sun and L. Ma, Pharmacological activities of mogrosides, Future Med. Chem., 2018, 10, 845–850 CrossRef CAS PubMed
.
- H. Liang, R. Cheng, J. Wang, H. Xie, R. Li, K. Shimizu and C. Zhang, Mogrol, an aglycone of mogrosides, attenuates ulcerative colitis by promoting AMPK activation, Phytomedicine, 2021, 81, 153427 CrossRef PubMed
.
- Y. M. Zhu, L. C. Pan, L. J. Zhang, Y. Yin, Z. Y. Zhu, H. Q. Sun and C. Y. Liu, Chemical structure and antioxidant activity of a polysaccharide from Siraitia grosvenorii, Int. J. Biol. Macromol., 2020, 165, 1900–1910 CrossRef CAS PubMed
.
- L. Li, W. Zheng, C. Wang, J. Qi and H. Li, Mogroside V Protects against Hepatic Steatosis in Mice on a High-Fat Diet and LO2 Cells Treated with Free Fatty Acids via AMPK Activation, J. Evidence-Based Complementary Altern. Med., 2020, 2020, 7826874 Search PubMed
.
- Y. Zhang, Y. Peng, L. Zhao, G. Zhou and X. Li, Regulating the gut microbiota and SCFAs in the faeces of T2DM rats should be one of antidiabetic mechanisms of mogrosides in the fruits of Siraitia grosvenorii, J. Ethnopharmacol., 2021, 274, 114033 CrossRef CAS PubMed
.
- J. Chen, D. Jiao, Y. Li, C. Jiang, X. Tang, J. Song and Q. Chen, Mogroside V Inhibits Hyperglycemia-induced Lung Cancer Cells Metastasis through Reversing EMT and Damaging Cytoskeleton, Curr. Cancer Drug Targets, 2019, 19, 885–895 CrossRef CAS PubMed
.
- G. Chen, C. Liu, G. Meng, C. Zhang, F. Chen, S. Tang, H. Hong and C. Zhang, Neuroprotective effect of mogrol against Aβ(1–42) -induced memory impairment neuroinflammation and apoptosis in mice, J. Ethnopharmacol., 2019, 71, 869–877 CAS
.
- M. Weerawatanakorn, J. R. Yang, M. L. Tsai, C. S. Lai, C. T. Ho and M. H. Pan, Inhibitory effects of Momordica grosvenori Swingle extracts on 12-O-tetradecanoylphorbol 13-acetate-induced skin inflammation and tumor promotion in mouse skin, Food Funct., 2014, 5, 257–264 RSC
.
- Y. Zhang, G. Zhou, Y. Peng, M. Wang and X. Li, Anti-hyperglycemic and anti-hyperlipidemic effects of a special fraction of Luohanguo extract on obese T2DM rats, J. Ethnopharmacol., 2020, 247, 112273 CrossRef CAS PubMed
.
- H. Liu, X. Qi, K. Yu, A. Lu, K. Lin, J. Zhu, M. Zhang and Z. Sun, AMPK activation is involved in hypoglycemic and hypolipidemic activities of mogroside-rich extract from Siraitia grosvenorii (Swingle) fruits on high-fat diet/streptozotocin-induced diabetic mice, Food Funct., 2019, 10, 151–162 RSC
.
- X. Zhang, Y. Song, Y. Ding, W. Wang, L. Liao, J. Zhong, P. Sun, F. Lei, Y. Zhang and W. Xie, Effects of Mogrosides on High-Fat-Diet-Induced Obesity and Nonalcoholic Fatty Liver Disease in Mice, Molecules, 2018, 23, 1894 CrossRef PubMed
.
- J. Nie, K. Yan, L. Sui, H. Zhang, H. Zhang, X. Yang, S. Lu, K. Lu and X. Liang, Mogroside V improves porcine oocyte in vitro maturation and subsequent embryonic development, Theriogenology, 2020, 141, 35–40 CrossRef CAS PubMed
.
- K. Yan, K. Cui, J. Nie, H. Zhang, L. Sui, H. Zhang, X. Yang, C. L. Xu and X. Liang, Mogroside V Protects Porcine Oocytes From Lipopolysaccharide-Induced Meiotic Defects, Front. Cell. Dev. Biol., 2021, 9, 639691 CrossRef PubMed
.
- L. Sui, K. Yan, H. Zhang, J. Nie, X. Yang, C. L. Xu and X. Liang, Mogroside V Alleviates Oocyte Meiotic Defects and Quality Deterioration in Benzo(a)pyrene-Exposed Mice, Front. Pharmacol., 2021, 12, 722779 CrossRef CAS PubMed
.
- J. Nie, L. Sui, H. Zhang, H. Zhang, K. Yan, X. Yang, S. Lu, K. Lu and X. Liang, Mogroside V protects porcine oocytes from in vitro ageing by reducing oxidative stress through SIRT1 upregulation, Aging, 2019, 11, 8362–8373 CrossRef CAS PubMed
.
- H. 0Tamura, M. Kawamoto, S. Sato, I. Tamura, R. Maekawa, T. Taketani, H. Aasada, E. Takaki, A. Nakai, R. J. Reiter and N. Sugino, Long-term melatonin treatment delays ovarian aging, J. Pineal Res., 2017, 62, e12381 CrossRef PubMed
.
- S. L. Byers, M. V. Wiles, S. L. Dunn and R. A. Taft, Mouse estrous cycle identification tool and images, PLoS One, 2012, 7, e35538 CrossRef CAS PubMed
.
- B. Huang, C. Ding, Q. Zou, J. Lu, W. Wang and H. Li, Human Amniotic Fluid Mesenchymal Stem Cells Improve Ovarian Function During Physiological Aging by Resisting DNA Damage, Front. Pharmacol., 2020, 11, 272 CrossRef CAS PubMed
.
- M. I. Love, W. Huber and S. Anders, Moderated estimation of fold change and dispersion for RNA-seq data with DESeq2, Genome Biol., 2014, 15, 550 CrossRef PubMed
.
- M. D. Young, M. J. Wakefield, G. K. Smyth and A. Oshlack, Gene ontology analysis for RNA-seq: accounting for selection bias, Genome Biol., 2010, 11, R14 CrossRef PubMed
.
- C. Xie, X. Mao, J. Huang, Y. Ding, J. Wu, S. Dong, L. Kong, G. Gao, C. Y. Li and L. Wei, KOBAS 2.0: a web server for annotation and identification of enriched pathways and diseases, Nucleic Acids Res., 2011, 39, W316–W322 CrossRef CAS PubMed
.
- T. Umehara, T. Kawai, I. Kawashima, K. Tanaka, S. Okuda, H. Kitasaka, J. S. Richards and M. Shimada, The acceleration of reproductive aging in Nrg1(flox/flox); Cyp19-Cre female mice, Aging Cell, 2017, 16, 1288–1299 CrossRef CAS PubMed
.
- L. R. Bernstein, A. C. Mackenzie, D. C. Kraemer, J. E. Morley, S. Farr, C. L. Chaffin and I. Merchenthaler, Shortened estrous cycle length, increased FSH levels, FSH variance, oocyte spindle aberrations, and early declining fertility in aging senescence-accelerated mouse prone-8 (SAMP8) mice: concomitant characteristics of human midlife female reproductive aging, Endocrinology, 2014, 155, 2287–2300 CrossRef PubMed
.
- C. Yang, Q. Liu, Y. Chen, X. Wang, Z. Ran, F. Fang, J. Xiong, G. Liu, X. Li, L. Yang and C. He, Melatonin delays ovarian aging in mice by slowing down the exhaustion of ovarian reserve, Commun. Biol., 2021, 4, 534 CrossRef CAS PubMed
.
- Y. L. Chu, Y. R. Xu, W. X. Yang and Y. Sun, The role of FSH and TGF-β superfamily in follicle atresia, Aging, 2018, 10, 305–321 CrossRef CAS PubMed
.
- J. Grosbois and I. Demeestere, Dynamics of PI3K and Hippo signaling pathways during in vitro human follicle activation, Hum. Reprod., 2018, 33, 1705–1714 CrossRef CAS PubMed
.
- X. Du, L. Zhang, X. Li, Z. Pan, H. Liu and Q. Li, TGF-β signaling controls FSHR signaling-reduced ovarian granulosa cell apoptosis through the SMAD4/miR-143 axis, Cell Death Dis., 2016, 7, e2476 CrossRef CAS PubMed
.
- V. A. Ansere, S. Ali-Mondal, R. Sathiaseelan, D. N. Garcia, J. V. V. Isola, J. D. Henseb, T. D. Saccon, S. R. Ocañas, K. B. Tooley, M. B. Stout, A. Schneider and W. M. Freeman, Cellular hallmarks of aging emerge in the ovary prior to primordial follicle depletion, Mech. Ageing Dev., 2021, 194, 111425 CrossRef CAS PubMed
.
- J. Y. Liu, G. P. Souroullas, B. O. Diekman, J. Krishnamurthy, B. M. Hall, J. A. Sorrentino, J. S. Parker, G. A. Sessions, A. V. Gudkov and N. E. Sharpless, Cells exhibiting strong p16 (INK4a) promoter activation in vivo display features of senescence, Proc. Natl. Acad. Sci. U. S. A., 2019, 116, 2603–2611 CrossRef CAS PubMed
.
- A. Georges, A. Auguste, L. Bessière, A. Vanet, A. L. Todeschini and R. A. Veitia, FOXL2: a central transcription factor of the ovary, J. Mol. Endocrinol., 2014, 52, R17–R33 CAS
.
- M. J. Park, J. W. Ahn, K. H. Kim, J. Bang, S. C. Kim, J. Y. Jeong, Y. E. Choi, C. W. Kim and B. S. Joo, Prediction of
ovarian aging using ovarian expression of BMP15, GDF9, and C-KIT, Exp. Biol. Med., 2020, 245, 711–719 CrossRef CAS PubMed
.
- X. An, H. Ma, Y. Liu, F. Li, Y. Song, G. Li, Y. Bai and B. Cao, Effects of miR-101–3p on goat granulosa cells in vitro and ovarian development in vivo via STC1, J. Anim. Sci. Biotechnol., 2020, 11, 102 CrossRef CAS PubMed
.
- L. Hui, G. Shuangshuang, Y. Jianning and S. Zhendan, Systemic analysis of gene expression profiles in porcine granulosa cells during aging, Oncotarget, 2017, 8, 96588–96603 CrossRef PubMed
.
- Z. Zhang, F. Schlamp, L. Huang, H. Clark and L. Brayboy, Inflammaging is associated with shifted macrophage ontogeny and polarization in the aging mouse ovary, Reproduction, 2020, 159, 325–337 CAS
.
- J. E. Rowley, F. Amargant, L. T. Zhou, A. Galligos, L. E. Simon, M. T. Pritchard and F. E. Duncan, Low Molecular Weight Hyaluronan Induces an Inflammatory Response in Ovarian Stromal Cells and Impairs Gamete Development In Vitro, Int. J. Mol. Sci., 2020, 21, 1036 CrossRef CAS PubMed
.
- D. M. Duffy, C. Ko, M. Jo, M. Brannstrom and T. E. Curry, Ovulation: Parallels With Inflammatory Processes, Endocr. Rev., 2019, 40, 369–416 CrossRef PubMed
.
- C. Lliberos, S. H. Liew, A. Mansell and K. J. Hutt, The Inflammasome Contributes to Depletion of the Ovarian Reserve During Aging in Mice, Front. Cell. Dev. Biol., 2020, 8, 628473 CrossRef PubMed
.
- J. M. Navarro-Pando, E. Alcocer-Gómez, B. Castejón-Vega, E. Navarro-Villarán, M. Condés-Hervás, M. Mundi-Roldan, J. Muntané, A. J. Pérez-Pulido, P. Bullon, C. Wang, H. M. Hoffman, A. Sanz, G. Mbalaviele, B. Ryffel and M. D. Cordero, Inhibition of the NLRP3 inflammasome prevents ovarian aging, Sci. Adv., 2021, 7, eabc7409 CrossRef CAS PubMed
.
- L. Ma, H. Lu, R. Chen, M. Wu, Y. Jin, J. Zhang and S. Wang, Identification of Key Genes and Potential New Biomarkers for Ovarian Aging: A Study Based on RNA-Sequencing Data, Front. Genet., 2020, 11, 590660 CrossRef CAS PubMed
.
- C. Lliberos, S. H. Liew, P. Zareie, N. L. La Gruta, A. Mansell and K. Hutt, Evaluation of inflammation and follicle depletion during ovarian ageing in mice, Sci. Rep., 2021, 11, 278 CrossRef CAS PubMed
.
- R. Di, M. T. Huang and C. T. Ho, Anti-inflammatory activities of mogrosides from Momordica grosvenori in murine macrophages and a murine ear edema model, J. Agric. Food Chem., 2011, 59, 7474–7481 CrossRef CAS PubMed
.
- Y. Y. Sung, H. J. Yuk, W. K. Yang, S. H. Kim and D. S. Kim, Siraitia grosvenorii Residual Extract Attenuates Atopic Dermatitis by Regulating Immune Dysfunction and Skin Barrier Abnormality, Nutrients, 2020, 12, 3638 CrossRef CAS PubMed
.
- Y. Y. Sung, S. H. Kim, H. J. Yuk, W. K. Yang, Y. M. Lee, E. Son and D. S. Kim, Siraitia grosvenorii residual extract attenuates ovalbumin-induced lung inflammation by down-regulating IL-4, IL-5, IL-13, IL-17, and MUC5AC expression in mice, Phytomedicine, 2019, 61, 152835 CrossRef CAS PubMed
.
- H. Wang, G. L. Meng, C. T. Zhang, H. Wang, M. Hu, Y. Long, H. Hong and S. S. Tang, Mogrol attenuates lipopolysaccharide (LPS)-induced memory impairment and neuroinflammatory responses in mice, J. Asian Nat. Prod. Res., 2020, 22, 864–878 CrossRef CAS PubMed
.
Footnote |
† Electronic supplementary information (ESI) available: Table S1: Primer sequences used for real–time RT-PCR. Table S2: RNA-seq data. See DOI: 10.1039/d1fo03194e |
|
This journal is © The Royal Society of Chemistry 2022 |