DOI:
10.1039/D1BM01466H
(Paper)
Biomater. Sci., 2022,
10, 227-242
In situ mucoadhesive hydrogel capturing tripeptide KPV: the anti-inflammatory, antibacterial and repairing effect on chemotherapy-induced oral mucositis
Received
18th September 2021
, Accepted 14th October 2021
First published on 18th November 2021
Abstract
The self-healing of chemotherapy-induced oral mucositis is difficult in practice because of both local bacterial infection and severe inflammation. Herein, in situ mucoadhesive hydrogels (PPP_E) were successfully prepared by using temperature-sensitive PLGA-PEG-PLGA (PPP) as a matrix and epigallocatechin-3-gallate (EGCG) with inherent antibacterial activity as an adhesion enhancer. A series of PPP_E precursor solutions with various EGCG concentrations (1%, 2% and 5%) were prepared by fixing the PPP concentration at 25%. EGCG slightly decreased the sol–gel transition temperature and shortened the sol–gel transition time of the PPP hydrogel. Moreover, the incorporation of EGCG could significantly increase the tissue adhesion properties of the PPP hydrogel at 37 °C. PPP_2%E displayed a suitable gelation temperature (36.2 °C), gelation time (100 s) and storage modulus (48 Pa). Tripeptide KPV as a model drug was easily dissolved in cold PPP_2%E precursor solution to prepare KPV@PPP_2%E hydrogel. The anti-inflammatory activity and promotion of cell migration potential by KPV in PPP-2% E hydrogel were well maintained. Moreover, KPV@PPP_2%E exhibited strong antibacterial efficacy against S. aureus. PPP_2%E precursor solution rapidly transformed to a hydrogel and adhered to the wound surface for 7 hours when administrated to the gingival mucosa of rats. Treatment with KPV@PPP_2%E hydrogel greatly improved the food intake and body weight recovery of rats with chemotherapy-induced oral mucositis. Moreover, the tissue morphology of the ulcerated gingiva after application of KPV@PPP_E hydrogel was also well repaired by promoting CK10 and PCNA expression. In addition, the inflammatory cytokines including IL-1β and TNF-α were significantly inhibited by KPV@PPP_2%E hydrogel while IL-10 was up-regulated. KPV@PPP_2%E hydrogel also had an anti-bacterial effect on MRSA-infected gingival ulcer wounds, which resulted in the obvious inhibition of infiltration by inflammatory cells into submucosal tissues. Conclusively, KPV@PPP_E may be a promising practical application for cancer patients with chemotherapy-induced oral mucositis.
1 Introduction
Oral mucositis (OM) with increased risk of bacterial infection is an inflammatory, erosive and ulcerative process of the oral mucosa. Oral mucositis is a significant toxicity of systemic chemotherapy and of radiation therapy to the head and neck region.1 The incidence of OM in cancerous patients treated with chemotherapy is about 20 to 40 percent; this percentage rises to about 90% for head and neck cancer patients treated with both chemo- and radiotherapy.2 The morbidity of oral mucositis can include pain, nutritional compromise, impact on quality of life, alteration in cancer therapy, risk of infection, and economic costs.3 Management includes general symptomatic support, oral hygiene protocols and nutrition supplementation and targeted therapeutic interventions for the prevention or treatment of oral mucositis.4
KPV(Lys-Pro-Val) is a tripeptide derived from the C-terminal sequence of α-melanocyte-stimulating hormone (α-MSH), which possesses strong anti-inflammatory activity though regulating NF-κB signals.5 Moreover, the use of anti-inflammatory KPV to promote tissue repair has been broadly reported in various disease models, including cutaneous wounds and ulcers,6 ulcerative colitis7 and corneal epithelial wound.8 KPV is first recognized by the peptide transporter 1 (PepT1)9 which is greatly expressed in human epidermal cells, followed by its transport to the cytoplasm targets.10 Like most proteins or peptides, however, KPV is also susceptible to degradation when exposed to high temperature and enzymes, thereby displaying a short half-life and low bioavailability in vivo.11
Epigallocatechin-3-gallate (EGCG), a component extracted from green tea, has been proved to have multiple effects on human pathological and physiological processes. The biological activities of EGCG, such as antioxidant, anti-inflammatory and anti-bacterium effects, have been demonstrated in recent publications.12–14 In particular, EGCG mouthwash had been demonstrated to protect the mucosa from radiation-induced mucositis in head and neck cancer patients in a prospective phase I trial.15 The antioxidant mechanism of EGCG was due to its ability to quench free radicals or scavenge reactive oxygen species (ROS), such as hydroxyl radicals, singlet oxygen, peroxyl radicals, nitric oxide and nitrogen dioxide.16 Moreover, researchers have confirmed that EGCG could eradicate infectious agents and actually prevent infections because it disrupts the bacterial cell membrane.17 For example, EGCG-binding amyloid fibrils hydrogel has been demonstrated to retain the main binding functions of inducing bacterial agglomeration and immobilization on both Gram-positive and Gram-negative bacteria.18 The anti-bacterial activity might prevent bacterial infection at the wound site and accelerate tissue regeneration. In addition, EGCG has been reported to possess mucoadhesive potential, which has been widely exploited as an additive in pharmaceutical formulas.14 For instance, a new adhesive oral cavity patch containing EGCG has been developed.19 The adhesive mechanism of EGCG toward the oral mucosa was proved to be associated with its irreversible catechol–mucin interactions.
Topical therapies are reasonable choices both to control pain/bleeding and to obtain complete and rapid remission of OM.20 Moreover, topical formulations avoid the side effects associated with systemic treatments since the target tissue is easily accessible.21 Many anti-inflammatory or analgesic drugs have been developed into topical formulations, including mucoadhesive films,22 mucoadhesive hydrogel,23 buccal tablets24 and electrospun nanofibers (NFs)25 for OM treatments within recent years. Among them, several topical formulations, such as Caphosol®, MuGard®, Mucotrol™, Gelclair®, Episil® and Palifermin have been marketed.26 However, there are still many challenges, such as short retention time, rapid erosion and noneffective wound dressing for these commercial formulations due to saliva washing and enzymolysis in the oral cavity. Therefore, there is an urgent need to develop an efficient and convenient local mucosa adhesion delivery system to prolong drug retention time to cater to complex oral conditions for OM repair in cancerous patients.
Temperature-sensitive hydrogels are a promising drug delivery vehicle for OM management.27 The polymer precursor solutions together with bioactive molecules at room temperature can be sprayed on the irregular ulcerative wound and spontaneously undergo a sol–gel transition in situ at body temperature to dress the wound. As a physical protective barrier, the dressed hydrogel could relieve pain and discomfort.28 Among various temperature-sensitive polymers, triblock poly(lactic-co-glycolic acid)-poly(ethylene glycol)-poly(lactic-co-glycolic acid) (PLGA-PEG-PLGA) has been extensively used as a hydrogel-forming material due to its excellent biocompatibility and biodegradation.29 PLGA-PEG-PLGA hydrogel with a suitable gelling profile has been developed as a potential bandage for corneal wound repair.30 However, the low adhesion of PLGA-PEG-PLGA hydrogel means it can be rapidly cleared by saliva washing when applied to wounded oral mucosa, which greatly limits its application in buccal delivery systems.
In this study, an in situ mucoadhesive hydrogel has been designed by using PLGA-PEG-PLGA and EGCG as a backbone polymer and adhesion enhancer, respectively. Moreover, the anti-inflammatory tripeptide, KPV was directly dissolved in the cold EGCG/PLGA-PEG-PLGA (PPP_E) precursor solution. PPP_E hydrogel with KPV would be spontaneously formed in response to body temperature and adhere to the wound surface when administrated to the ulcerated oral mucosa. We hypothesized that bio-adhesive KPV@PPP_E hydrogel would achieve the rapid repair of oral chemotherapy-induced mucositis via an anti-inflammatory and antibacterial effect (Fig. 1). In this study a series of formulations with different EGCG amounts have been prepared and evaluated by sol–gel transition temperature and tissue adhesive properties. In addition, the antibacterial activity of KPV@PPP_E hydrogel against S. aureus was further demonstrated in vitro. Moreover, the anti-inflammatory and anti-oxidative effect of KPV@PPP_E was also detected by using human oral keratinocyte (HOK) cells. In situ retention of KPV@PPP_E hydrogel in the gingival ulcer zone was further investigated by an in vivo living imaging system. Finally, the repair effect of KPV@PPP_E hydrogel on chemotherapy-induced oral mucositis of SD rats was evaluated by macroscopic and histopathological analysis.
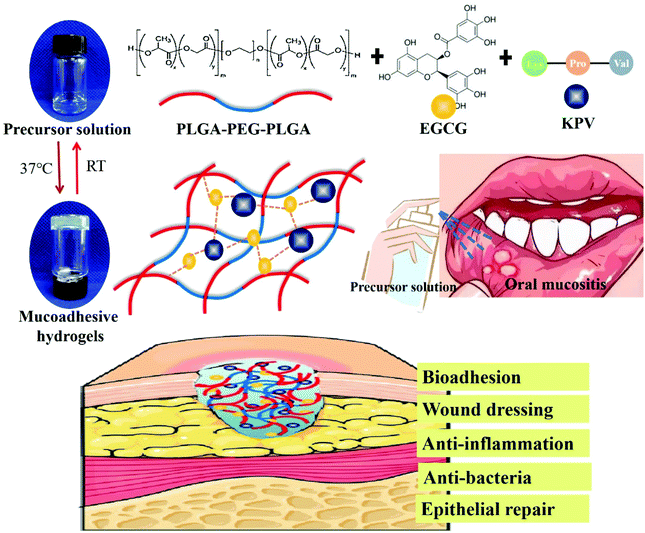 |
| Fig. 1 Scheme of mucoadhesive KPV@PPP_E hydrogel for chemotherapy-induced oral mucositis. | |
2 Materials and methods
2.1 Materials and reagents
PLGA-PEG-PLGA copolymer (mole ratio of LA/GA equals 3, PLGA segments with Mn of 1775 and PEG segment with Mn of 1000) was purchased from Daigang Biotechnology Limited Company (Jinan, China). EGCG was purchased from Shanghai Darui Fine Chemical Co., Ltd. Compound chlorhexidine dexamethasone film was purchased from Xian Kanghua Pharmacy Co., Ltd. KPV was purchased from GL Bio-Chem Co., Ltd (Shanghai, China). 3-[4,5-Dimethylthiazol-2-yl]-2,5-diphenyltetrazolium bromide (MTT) was obtained from Sigma-Aldrich (St Louis, MO, USA).
2.2 The preparation of PPP_E hydrogel
Aqueous PLGA-PEG-PLGA solutions (300 mg mL−1) were prepared by dissolving PLGA-PEG-PLGA in cold phosphate buffer solution (PBS, pH 7.4). Subsequently, EGCG powder was added to the PLGA-PEG-PLGA solutions under gentle stirring until the formation of a transparent solution. A series of EGCG/PLGA-PEG-PLGA precursor solutions were prepared by adjusting the amount of EGCG (1%, 2% and 5%) in the formulation. The aqueous EGCG/PLGA-PEG-PLGA precursor solutions were added into vials to form PPP_E hydrogels at 37 °C. As control, a PPP hydrogel was also prepared by a similar procedure but without addition of EGCG. To prepare KPV-loaded PPP_E hydrogels (KPV@PPP_E), KPV was directly dissolved in the cold EGCG/PLGA-PEG-PLGA precursor solution under gentle stirring, followed by gelation at 37 °C.
2.3 Rheological properties of PPP_E hydrogel
The apparent viscosity of the PPP_E hydrogels was first measured on a coaxial cylinder rheometer (NDJ-8S, China) using a No. 1 rotor and a small sample adapter. The temperature-dependent viscosity curves of the PPP_E hydrogels were detected in the temperature range from 20 °C to 50 °C. The gelation time of the cold PPP_E solutions was also detected by the vial tilting method, as described in a previous study.31 In addition, the rheological behavior of the PPP_E hydrogels was also detected by using a rheometer (Discovery HR-2) fitted with a flat plate (40 mm) with a 0.8 mm gap. All samples were dropped onto the parallel plates and the temperature sweep tests were carried out at the rate of 1 °C min−1 within the temperature range from 25 °C to 50 °C at an oscillatory frequency of 10 rad s−1. According to the temperature sweep tests, the gelation temperature was calculated at the crossover point of the storage modulus (G′) and the loss modulus (G′′). Oscillatory frequency sweeps were performed at 0.1% of strain with frequency increasing from 0.01 to 10 Hz.
2.4 Tissue adhesion properties of PPP_E hydrogel
The tissue adhesion properties of the PPP_E hydrogel precursor were measured with a universal testing machine (UTM, Instron 3343, USA). Briefly, two pieces of mouse dorsal skin tissue of comparable size were fixed on two glass slides. Hydrogel samples were used to cover the surface of the mouse skin at room temperature, and the counterpart glass slide was carefully put over the hydrogel sample. Afterwards, all sets were placed in a 37 °C incubator for 5 minutes. The test was performed with a universal testing machine (UTM, EZ-SX STD, Shimadzu, Japan) at a crosshead speed of 5 mm min−1 and a 20 N load cell. The displacement–force curves were plotted and the maximum force was calculated from the displacement–force curves. In order to further investigate the adhesion properties, different balance weights were placed on the glass slides until complete dislocation occurred.
2.5
In vitro antibacterial activity of PPP_E hydrogel
The in vitro antibacterial activity of the PPP_E hydrogels was assessed against Gram-positive methicillin-resistant Staphylococcus aureus (MRSA) according to the method depicted in a previous study.32 Briefly, sterilized LB agar plates and liquid LB medium were first prepared using a tryptone soy broth (TBS, CM0129, OXOID) and bacteriological agar (AGAR, LP0011, OXOID). Subsequently, individual bacterial colonies of MRSA were added to 5 mL of liquid LB medium and further incubated at 37 °C for 4 h. Afterwards, 10 μL of MRSA suspension was transplanted to centrifuge tubes containing 3 mL of liquid LB medium supplemented with hydrogel formulation and PBS was used as control. After further incubation for 2 h at 37 °C, the growth rates and bacterial concentrations were determined by measuring the optical density at 600 nm (OD600). Standard plate counting was also carried. After 2 h incubation, 100 μL suspensions were taken from each centrifuge tube, and serial dilution (1
:
10) was carried out. Then, 25 μL of bacterial suspension was spread onto LB agar plates at 37 °C overnight. Finally, the images were taken and the colonies were counted using Image J software.
2.6 The compatibility of KPV with PPP-E hydrogel
Human oral keratinocyte (HOK) cells from ScienCell Research Laboratory were cultured in MEM basal medium supplemented with 1% glutamine, 1% penicillin–streptomycin and 10% fetal bovine serum in the presence of 5% CO2 at 37 °C. The anti-inflammatory activity of KPV in KPV@PPP_E hydrogel against LPS-induced HOK cells was first evaluated by detecting levels of IL-1β, IL-6, and IL-10. Briefly, HOK cells with a density of 5 × 103 were seeded on 96-well plates, and further cultured for 12 h. The attached HOK cells were insulted by 10 μg mL−1 lipopolysaccharide (LPS) for 2 h, followed by treatment with KPV@PPP_E hydrogel for 6 h. Subsequently, HOK cells were fixed with 4% paraformaldehyde for 20 min, washed thrice with PBS, permeabilized with 0.1% Triton X-100 for 15 min, and blocked with 5% bovine serum albumin (BSA) for 1 h at room temperature. Immunofluorescent staining of inflammation-associated factors, including IL-1β, IL-6, and IL-10, was performed. Primary antibodies, including anti-IL-6 (DF6087, 1:200, Affinity), anti-IL-1β (ab9722, 1:200, Abcam) and anti-IL-10 (20850-1-AP, 1:200, Proteintech), were used. Goat anti-mouse IgG Alexa Fluor 488 (ab150117, 1:1000, Abcam) or goat anti-rabbit IgG Alexa Fluor 488 (ab150017, 1:1000, Abcam) were used as second antibodies. Meanwhile, the cells were further stained with DAPI. The fluorescent images were observed by a Nikon ECLIPSE 80i (Nikon, Japan).
The cell migrating bioactivity of KPV in KPV@PPP_E hydrogel was also detected by a scratch assay, as previously reported.33 HOK cells at a density of 5 × 104 cells per pore were seeded on 6-well plates and further cultured for 24 h. A scratch was created with a sterile clear tip and the detached cells were removed with fresh PBS. The cells were treated with KPV@PPP_E hydrogel in the presence of H2O2 (10 mM) for 6 h. Afterwards, the culture medium was replaced with fresh medium and further cultured for 72 h. The scratch was imaged using a Nikon ECLIPSE 80i (Nikon, Japan) microscope and the wound healing rate was quantitatively calculated as the relative percentage of initial size.
2.7 Chemotherapy-induced oral mucositis rat model
Male Sprague–Dawley rats (180 g–200 g) were provided from the Laboratory Animals Centre of Wenzhou Medical University. All rats were housed in stainless-steel cages under standard environmental conditions. All animal care was conducted under the guidelines for animal experimentation under the National Institutes of Health and approved by the Animal Care and Use Committee of Wenzhou Medical University.
A chemotherapy-induced oral mucositis rat model was induced by a modified protocol based on a previously established method.34 Briefly, SD rats received 30 mg kg−1 5-FU intraperitoneally (ip) on days 1, 3 and 5 before acetic acid insult. The control group was intraperitoneally injected with the same amount of saline. Subsequently, on day 6, a 6 mm diameter filter paper disk soaked with 30% acetic acid was used to create an ulcerated area on the gingival mucosa, and all the rats were treated with this for 45 s.
2.7.1
In vivo retention of PPP_E hydrogel.
To investigate in situ muco-adhesion of PPP_E hydrogel, indocyanine green (ICG) as a tracking probe replaced KPV to prepare the ICG/PPP_E hydrogel. ICG@PPP_E hydrogel precursor (200 μL) was dropped on the oral cavity. At a predetermined time point, all the rats were put into the in vivo imaging system (Cambridge Research & Instrumentation, Inc.) to observe the remaining fluorescence. A series of formulations, including ICG/PPP, ICG/PPP_1%E, ICG/PPP_2%E and ICG/PPP_5%E, were evaluated. ICG solution was used as a control.
2.7.2 Treatment regimens with KPV@PPP-E hydrogel.
OM rats were randomly divided into 5 groups: control group (saline, n = 6), positive control group (0.12% dexamethasone ointment, n = 6), and experimental groups treated with the blank PPP-E hydrogel, free KPV solution (100 μg mL−1) and KPV@PPP-E hydrogel (100 μg mL−1) (n = 6 per group). All test samples (200 μL each time) were sprayed on the ulcerated surface and administered twice each day for 5 days. The SD rats in each group were weighed daily and their food intake was also recorded for 5 days before and after treatment.
2.7.3 Macroscopic evaluation and histopathological analysis.
After treatment with different formulations, macroscopic images of the gingival ulcers were recorded using a camera from day 0 to day 5. On day 5, rats each group were sacrificed and the gingival mucosa around the ulcer were collected, and then the samples were fixed in 4% paraformaldehyde for 2 days. Next, the fixed samples were embedded in paraffin. Sections of 5 μm thickness were prepared for hematoxylin/eosin (H&E) and Masson's trichrome (MT) staining. All images were captured with a Nikon ECLIPSE 80i (Nikon, Japan).
2.7.4 Immunohistochemical staining.
Inflammation-associated cytokines, including IL-1β, TNF-α and IL-10, were further evaluated by immunohistochemical staining. Briefly, sections of 5 μm thickness were incubated in 3% hydrogen peroxide and treated with 10% BSA for 10 min to prevent nonspecific binding. Then anti-IL-1β (ab9722, 1:200, Abcam), anti-TNF-α (ab183218, 1:200, Abcam) and anti-IL-10(20850-1-AP, 1:200, Proteintech) primary antibodies in 1% BSA were applied to sections overnight at 4 °C to detect relevant proinflammatory cytokines. In addition, epithelial repair was also assessed by detecting CK10 and PCNA. Primary antibodies CK10 (18343-1-AP, 1:200, Proteintech) and PCNA (ab92552, 1:200, Abcam) were used. Sections were labelled using a Polink-1 HRP DAB detection system (GBI, USA) and counterstained with hematoxylin. All images were captured with a Nikon ECLIPSE 80i (Nikon, Japan).
2.7.5
In situ bacterial detection in wound.
To further detect the antibacterial effect of KPV@PPP_E hydrogel in vivo, the ulcer wound was previously administrated with 2 μL MRSA suspensions (OD600 ≈ 0.3). The next day, each rat was treated with KPV@PPP and KPV@PPP_2%E. On the second day, a medical cotton swab was used to dip the ulcer site and transferred to the liquid LB medium. Meanwhile, the rats were sacrificed and the gingival mucosa around the ulcer were harvested for hematoxylin/eosin (H&E) staining.
2.8 Statistical analysis
All experiments were performed at least in triplicate and all the results were analysed by Graph Pad Prism 5 software. Data were presented as the mean value ± standard deviation (SD). All the results were statistically analyzed by the unpaired Student's t-test and multiple groups were studied using one-way ANOVA analysis; p < 0.05 was considered statistically significant.
3 Results and discussion
3.1 Rheological and bio-adhesive properties of PPP_E hydrogel
PLGA-PEG-PLGA copolymer solution (PPP) at lower than the critical temperature would go through a sol–gel transition when the temperature increased.35 In this study, a PPP solution at a polymer concentration of 25% could dissolve well in PBS to form a uniform solution at room temperature, but the solution became gelled at 37 °C. To investigate whether EGCG affects the sol–gel transition of PPP solution, a series of PPP_E precursor solutions with a constant PPP concentration (25%) were prepared by adjusting the amount of EGCG (1%, 2% and 5%) in the formulations and the apparent viscosity of these PPP_E hydrogels was measured along with a temperature increase from 25 to 50 °C. The results are shown in Fig. 2A. The viscosity of these PPP_E precursor solutions rapidly increased to a maximum value as the temperature rose, followed by a gradual decrease due to the melting of the PEG or PLGA block. At a temperature of 36–42 °C, all these precursor solutions showed a significant increase in viscosity, suggesting that the sol–gel transition occurred in this temperature range. However, addition of EGCG slightly accelerated the temperature-responsive speed and slightly decreased the sol–gel transition temperature in comparison with PPP solution alone. Moreover, the more EGCG there was in the PPP_E precursor solution, the more obvious was an abrupt increase in viscosity.
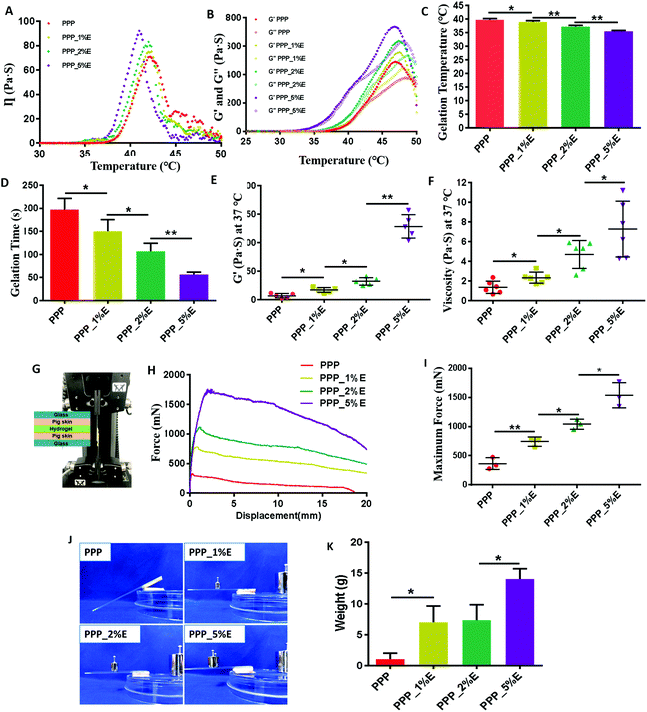 |
| Fig. 2 ( A) The apparent viscosity of PPP_E hydrogels with various EGCG concentrations as a function of temperature; (B) storage modulus (G′, solid symbols) and loss modulus (G′′, open symbols) of PPP_E hydrogels with various EGCG concentrations within the temperature range 25 °C to 50 °C; (C) the gelation temperature and (D) the gelation time of PPP_E hydrogels with various EGCG concentrations at 37 °C; (E) the storage modulus and (F) apparent viscosity of PPP_E hydrogels with various EGCG concentrations at 37 °C; (G) the test apparatus of the universal testing machine; (H) the representative force–displacement curve and (I) the quantitative maximum failure force of the PPP_E hydrogels; (J) images demonstrating the tissue adhesiveness of PPP, PPP_1%E, PPP_2%E and PPP_5%E sustaining different weights; (K) a quantification analysis of PPP, PPP_1%E, PPP_2%E and PPP_5%E sustaining weights (*p < 0.05, **p < 0.01, NS means no statistical significance, n = 3). | |
The viscoelastic moduli of the PPP_E precursor solution as function of temperature were further measured to obtain the accurate sol–gel transition temperature. Below the sol–gel transition temperature, the loss moduli (G′′) of all the precursors solution were higher than the storage moduli (G′), exhibiting their viscous behaviour. Both G′ and G′′ for all the precursor solutions increased as the temperature increased (Fig. 2B). The sol–gel transition temperature at which the G′ curve intersects with the G′′ curve was accurately measured and results are displayed in Fig. 2C. Truly, the sol–gel transition temperature of the PPP_E solution decreased as the concentration of EGCG in the formulations increased. The sol–gel transition temperature of the PPP_E solutions slightly decreased from 39.7 ± 0.1 °C for the PPP solution to 35.4 ± 0.3 °C for the PPP_5%E solution. At the physiological temperature (37 °C), the gelation time of the PPP_E precursor solutions was further detected by the vial tilting method. The gelation time of the PPP_E precursor solutions became shorter as the EGCG concentration increased. The PPP precursor solution takes about 200 ± 5 s to become gelled. But the gelation time of the PPP_5%E precursor solution shortened to 50 ± 10 s (Fig. 2D). Moreover, the viscosity and storage moduli (G′) of the PPP_E hydrogels at 37 °C had also obviously increased in comparison with the PPP hydrogel (Fig. 2E and F), indicating their higher mechanical strength. The PLGA-PEG-PLGA block copolymer is amphiphilic and self-assembled into micelles in aqueous solution below the critical gel temperature (CGT). As the temperature increased above the CGT, the assembled micelles became entangled with each other to form a PPP hydrogel.36 In this study, due to the physical interaction between EGCG and the PEG segments of the PPP copolymer, EGCG might act as a bridge between micelles of PLGA-PEG-PLGA and increase intermicellar interactions, leading to gelation at lower temperature and a shorter gelation time for the PPP_E hydrogels. A similar interaction of EGCG with PEGylated chitosan had been demonstrated in a previous study.37
The adhesive properties of PPP_E hydrogels against tissues were first evaluated by lap-shear tests with some modification in the previous study.14 As shown in Fig. 2G–I, the incorporation of EGCG could significantly increase the tissue adhesion properties of a PPP hydrogel. Moreover, the maximum adhesive force of the PPP_E hydrogels increased as a function of EGCG concentration in the formulations. The PPP hydrogel had a maximum adhesive force of 362.2 ± 100.6 mN while the PPP_5%E hydrogel presented the maximum adhesive force of 1535.8 ± 215.1 mN. In addition, the adhesive toughness was also evaluated with peel tests and similar results were reached. As shown in Fig. 2J and K, PPP_1%E, PPP_2%E and PPP_5%E hydrogels can resist weights of 7.0 ± 2.6 g, 7.33 ± 2.5 g and 14.0 ± 1.7 g, respectively, which were significantly higher than that of the PPP hydrogel. The higher adhesion strength of the PPP_E hydrogels was largely attributed to the strong interaction of tissue components with EGCG.14 Considering its suitable gelation temperature, gelation time and storage modulus, the KPV@PPP_2%E hydrogel was further used to evaluate the bioactive effect on a damaged cell line and the chemotherapy-induced oral mucositis rat model.
3.2 The compatibility of KPV with PPP-E hydrogel
To confirm whether the loading KPV was compatible with PPP-E hydrogel, the bioactivity of KPV in PPP-E hydrogel, which was usually reflected in its anti-inflammatory and promotion of cell migration activity, were detected by using in vitro cells line models. Our preliminary study showed that native KPV had no cell proliferation ability or migration potential against normal Caco-2 cells. But these bioactivities of KPV were obviously reviewed by using inflamed Caco-2 cells models in a previous publication.38 In this study, as an inflamed cell line model, HOK cells were firstly damaged by lipopolysaccharide (LPS, 10 μg mL−1) for 2 hours for a bioactivity test. As shown in Fig. 3A and B, the inflamed HOK cells in the PPP group showed a marked increase in terms of IL-1β, IL-6 and IL-10 in comparison with the CON group (normal HOK cells treated with PBS), indicating that the blank PPP hydrogels presented a negligible anti-inflammatory effect. Similar results were also observed in previous publications.39,40 Interestingly, the blank PPP_2%E hydrogel without KPV still displayed an obvious decrease in the level of proinflammatory cytokines, such as IL-1β or IL-6 levels, and accordingly increased the expression of anti-inflammatory cytokine, IL-10. These results suggested that the anti-inflammatory activity of the PPP_2%E hydrogel originated from EGCG. The anti-inflammatory properties of green tea extract and EGCG had also been demonstrated in an irinotecan-induced oral epithelial cell model.41 Among these treatments, KPV@PPP_2%E hydrogel resulted in the most obvious decrease in IL-1β and IL-6 expression, indicating that the bioactivity of KPV in the PPP-E hydrogel was well retained. Alternatively, the scratch method on HOK cells was further used to confirm the bioactivity of KPV in PPP_2%E. Fig. 3C and D represent the results of cell migration studies of blank PPP hydrogel, PPP_2%E, KPV@PPP or KPV@PPP_2%E hydrogel. A expected, the cell migration of KPV@PPP_2%E hydrogel was significantly higher than that of blank PPP hydrogel, PPP_2%E or KPV@PPP. Similarly, the remaining wound (%) in KPV@PPP_2%E hydrogel was 1.8-times lower than that in KPV@PPP (Fig. 3D), further indicating that the bioactivity of KPV was largely improved by PPP_2%E hydrogel. Collectively, the bioactivity of KPV was not compromised by PPP-E hydrogel and good compatibility between them was presented.
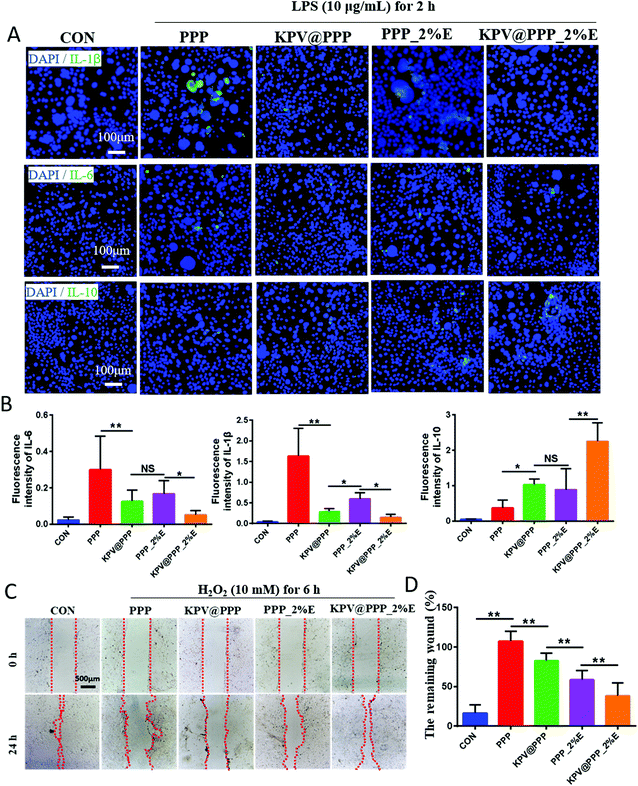 |
| Fig. 3 The bioactivity of KPV in PPP-E hydrogel: (A) immunofluorescence staining of IL-1β, IL-6 and IL-10 in LPS-insulted HOK cells in the presence of PPP-E hydrogel; (B) quantitative analysis of IL-1β, IL-6 and IL-10 based on immunofluorescence staining; (C) the scratched wound healing of H2O2-insulted HOK cells cultured with various formulations; (D) the quantitative statistics of wound scratching (*p < 0.05, **p < 0.01, NS, no significance, n = 3). | |
3.3
In situ retention of PPP_E hydrogel in the gingival ulcer zone
In order to investigate whether PPP_E hydrogel adheres to the gingival ulcer wound, an in vivo living imaging system was used to detect the retention of PPP_E hydrogel post administration. ICG was used as a fluorescent probe and encapsulated into the PPP_E hydrogel. As shown in Fig. 4A, ICG solution as the control was rapidly cleared from the gingival ulcer within 1 hour due to the saliva washing effect in the oral cavity. Although ICG fluorescence in all hydrogel groups gradually diffused from the ulcer area wound to the periphery, hydrogels, including ICG/PPP and various PPP_E hydrogels, were retained at the gingival ulcer for a long time. Moreover, the longer retention time of the PPP_E hydrogel was displayed in comparison with PPP hydrogel due to the EGCG-derived catechol taking part in adhesion.42 Therefore, the retention time of PPP_E hydrogel at the gingival ulcer was highly dependent on the EGCG concentration in the formula. The retention time of PPP_E hydrogel increased as a function of EGCG concentration. For instance, there was still strong fluorescence remaining at the gingival ulcer wound for PPP_2%E and PPP_5%E hydrogels even at 7 h post administration. The remaining fluorescence of the hydrogel was plotted as a function of time and the results are shown in Fig. 4B. At 4 h post administration, ca. 75% and 60% of the hydrogels still remained at the gingival ulcer wound for PPP_2%E and PPP_5%E hydrogels, respectively. Whereas there were only approximately 38% and 20% of the hydrogels remaining for PPP and PPP_1%E hydrogels.
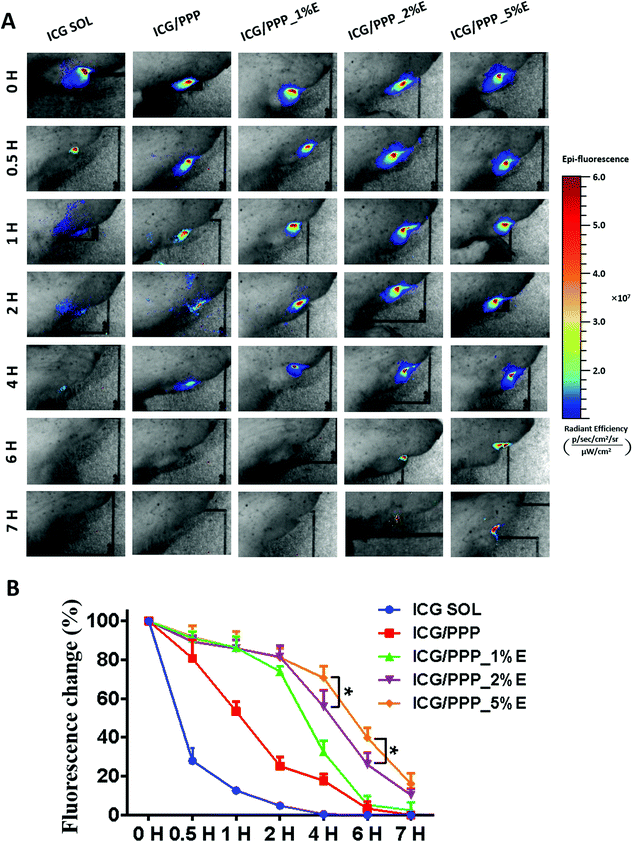 |
| Fig. 4 (A) In vivo imaging of the remaining hydrogel at the gingival ulcer wound at various times post administration (ICG-SOL: ICG solution; ICG/PPP; ICG/PPP_1%E; ICG/PPP_2%E and ICG/PPP_5%E); (B) quantitative analysis of the remaining hydrogel fluorescence normalized by fluorescence intensity at 0H (*p < 0.05, **p < 0.01, NS means no statistical significance, n = 3). | |
3.4 KPV@PPP_E hydrogel promoted the repair of oral gingival ulcers
5-Fu greatly impairs the normal condition of the oral mucosa in rats, which is very similar to the condition in a human after chemotherapy.43 A chemotherapy-induced oral mucositis model is usually established with 30% acetic acid (AA) insult on a 5-Fu-pretreated rat.44 The therapeutic effect of KPV@PPP_E hydrogel on the chemotherapy-induced oral mucositis model was evaluated in accordance with the experimental regimen depicted in Fig. 5A. The anti-inflammatory glucocorticoid, dexamethasone solution (DEX) available for clinical use was also evaluated as the positive drug. During the whole experiment, the body weight of the rats and daily food intake were monitored in each group. As shown in Fig. 5B and C, 5-Fu treatment led to a decrease in the body weight of the rats and in daily food intake. When the body weight of the rats decreased by approximately 15–20% after 3 cycles of 5-FU, an oral mucositis model was established by 30% acetic acid insult. An obvious decrease in food intake and body weight was observed in the 5 days following the acetic acid insult in the control group. By contrast, the body weight loss was significantly alleviated and the daily food intake slightly increased after administration of the blank PPP_2%E hydrogel, DEX solution, KPV solution or KPV@PPP_2%E hydrogel. Among these treatments, KPV@PPP_2%E hydrogel presented the best recovery of body weight and food intake, indicating the most effective alleviation of pain symptoms.
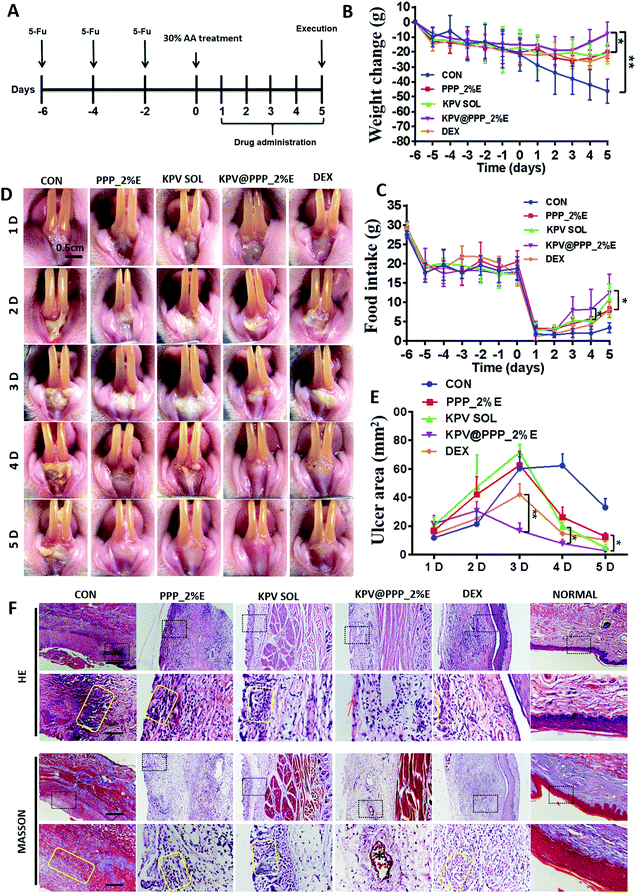 |
| Fig. 5 ( A) The therapeutic regime of KPV@PPP_E; (B) the daily food intake and (C) the body weights of rats during the whole treatment; (D) macroscopical images of oral ulcer wounds at predetermined time points and (E) analysis of ulcer wound area (*p < 0.05, **p < 0.01, NS means no statistical significance, n = 3); (F) HE and Masson's trichrome staining of the wound on day 5 after treatment (the yellow boxes indicate granulation tissue; the asterisk indicates a blood capillary). | |
The oral gingival ulcer wounds after treatment were monitored in real-time and macroscopic images are shown in Fig. 5D. On day 1 after AA insult, the oral gingiva of the rats show slight mucosal irritation and ulceration in each group. Obvious gingival ulcer wounds were formed on day 2 or day 3 due to epithelial necrosis. The ulcer wound area in each group after treatment was quantitatively analyzed and the results are displayed in Fig. 5E. The KPV@PPP_2%E hydrogel significantly attenuated the formation of gingival ulcer wounds. On day 3, the ulcer wound areas in groups given PPP_2%E, KPV or DEX was obviously larger than those of the KPV@PPP_2%E group. Moreover, KPV@PPP_2%E significantly reduced the ulcer wound area and accelerated re-epithelization within 3–5 days. On day 5, rats in each group were sacrificed and the gingival ulcer wounds were collected for histopathological morphology evaluation. The results are shown in Fig. 5F. Generally, self-repair for a normal oral ulcer would be completed within about 3 days in healthy rats.45 Even after 5 days of treatment, the oral mucosa were still broken and the squamous epithelium was broken off in each group, indicating that chemotherapy greatly slows down the rate of repair in our study. In the control group, a large amount of granulation tissue was infiltrated in the mucosal epithelium, the surface of which was covered with necrotic tissue. By contrast, rats treated with PPP_2%E, KPV SOL or KPV@PPP_2%E exhibited less granulation tissue or inflammatory cell infiltration. Nevertheless, after treatment with DEX, the inflammatory cell infiltration was even worse than in the PPP_2%E or KPV SOL group. Moreover, the basal lamina and blood capillaries had well recovered after KPV@PPP_2%E treatment, indicating that a combination of EGCG and KPV has a positive effect on the regeneration of oral gingival ulcers.
3.5 KPV@PPP_E hydrogel promoted the expression of CK10 and PCNA
Keratins are one major component of the epithelial cytoskeleton, which play important roles in wound healing in oral mucositis.46 The expression of keratins K10 (CK10) present in the gingival and epidermal tissues was stained in the ulcer wounds after treatment in this study. The results are displayed in Fig. 6A. CK10 was mainly expressed in the suprabasal layers of the sulcular epithelium in healthy rats (NORMAL), which was similar to the previous results.47 However, there was less expression of CK10 in the CON or DEX groups. After treatment with PPP_E, KPV SOL or KPV@PPP_2%E, the CK10 expression was obviously up-regulated. Moreover, among these treatments, CK10-positive areas were the highest in the KPV@PPP_2%E group (Fig. 6B). The robust stem cell (SC) population in the lamina propria was activated and proliferated in the self-repairing of oral mucosa via producing proliferating cell nuclear antigen (PCNA).48 Therefore, PCNA expression was also detected by immunohistochemical staining. Obvious PCNA expression was observed in the lamina propria of the epithelial mucosa in the KPV SOL or KPV@PPP_2%E groups, while PCNA was less expressed in the PPP_2%E or DEX groups. It is worth noting that the strongest expression of PCNA in the lamina propria was observed in the KPV@PPP_2%E group compared with the other groups (Fig. 6C). These results indicated that anti-inflammatory KPV can promote epithelial proliferation. Moreover, PPP_E hydrogel could improve the reparative effect of KPV on the damaged epithelium.
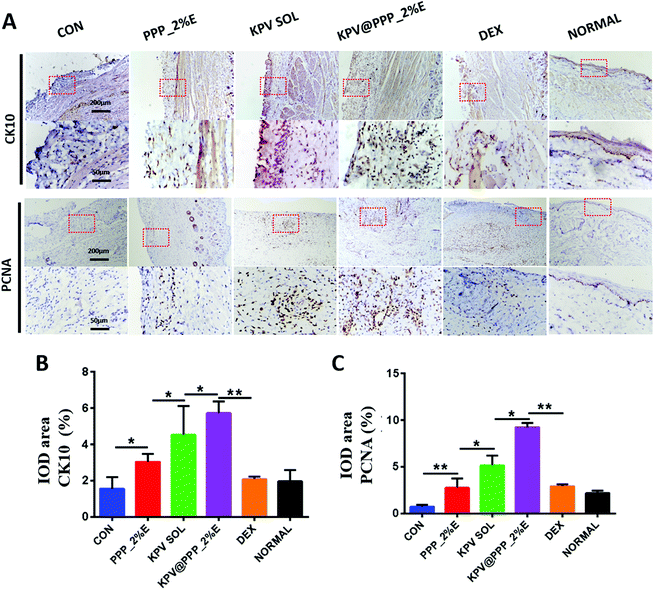 |
| Fig. 6 (A) Immunohistochemical staining of CK10 and PCNA for ulcer wounds on day 5 after treatment (brown indicates positive expression); quantitative analysis of (B) CK10 and (C) PCNA by Image J (IOD is the mean integrated optical density, *p < 0.05, **p < 0.01, n = 3). | |
3.6 KPV@PPP_E hydrogel alleviated inflammation
The levels of inflammation-associated cytokines, including IL-1β, TNF-α and IL-10 were evaluated by immunohistochemistry staining and the results are displayed in Fig. 7A. An excess of pro-inflammatory cytokine (IL-1β, TNF-α) was over-expressed in the mucosal epithelium while the anti-inflammatory cytokine (IL-10) was significantly down-regulated in the control group. After treatment with PPP_2%E, KPV SOL or KPV@PPP_2%E, the production of pro-inflammatory cytokines decreased to varying degrees in comparison with the control group. However, there was no obvious inhibition of inflammatory cytokines in the DEX group. Despite its anti-inflammatory effect in acute and delayed nausea and vomiting caused by various anticancer drugs, dexamethasone also causes B and T cell depletion and augments immunosuppression,49 thus leading to more inflammatory infiltration in the DEX group. KPV@PPP_2%E not only down-regulated the expression of IL-1β and TNF-α but also up-regulated the expression of IL-10. Moreover, KPV@PPP_2%E treatments presented the most obvious inflammation inhibition among these treatments (Fig. 7B–D). The anti-inflammation effect of KPV@PPP_2%E predominantly originated from the encapsulated KPV. KPV attenuating inflammatory cytokine production has also been demonstrated in skin wounds50 and inflammatory bowel disease.51 Moreover, it was previously reported that the anti-inflammatory mechanism of KPV was associated with suppressing NF-κB signaling via inhibition of p65RelA nuclear import and epithelial MC3R activation.52 Overall, KPV@PPP_E hydrogel has a strong effect on reducing the invasion of pro-inflammation cytokine and improving anti-inflammatory cytokine expression.
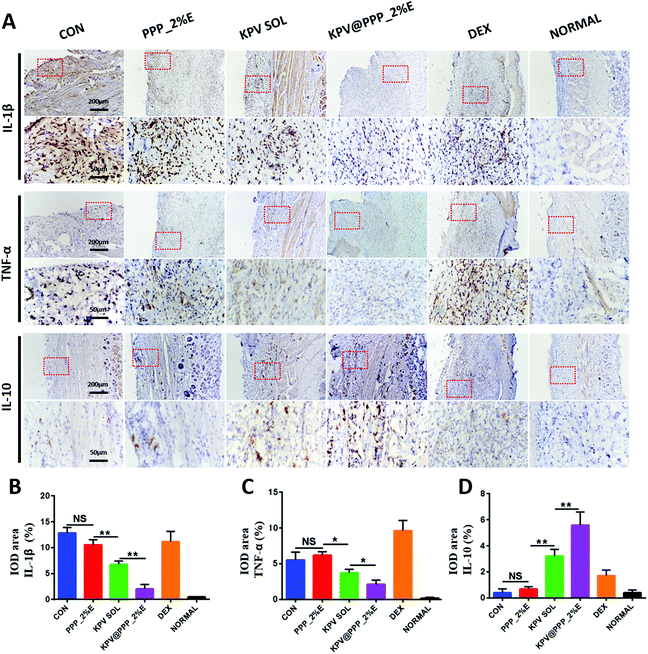 |
| Fig. 7 ( A) Immunohistochemical staining of IL-1β, TNF-α and IL-10 for ulcer wounds on day 5 after treatment (brown indicates positive expression); quantitative analysis of (B) IL-1β, (C) TNF-α and (D) IL-10 using Image J (IOD means integrated optical density, *p < 0.05, **p < 0.01, NS means no statistical significance, n = 3). | |
3.7 Antibacterial activity of KPV@PPP_E hydrogel
It has been demonstrated that more than 700 bacterial species can be found in the oral cavity.53 Cancer chemotherapy not only compromises the immune response against pathogenic bacteria but also disrupts the balance of the resident oral bacteria,54 resulting in bacterial infection in chemotherapy-induced oral mucositis. The important key for mucosal healing is to prevent the bacterial infection of the wound.55 Therefore, the antibacterial effects of KPV@PPP_E hydrogel was first evaluated in vitro. The results are displayed in Fig. 8A–D. A strong antibacterial effect of KPV@PPP_E against S. aureus was presented even when the EGCG concentration was as low as 1%. All KPV@PPP_E hydrogels showed comparable antibacterial activity to that of the positive control drug, amoxicillin. By contrast, the inhibitory growth of S. aureus was negligible for PPP hydrogel or KPV@PPP hydrogel without EGCG. These results demonstrated that the antibacterial effect of KPV@PPP_E hydrogel could be attributed to EGCG, which was similar to a previous result in the literature.56 Moreover, it has been shown that the mechanism of EGCG underlying its antibacterial action is highly associated with damaging the cell membrane of S. aureus.57
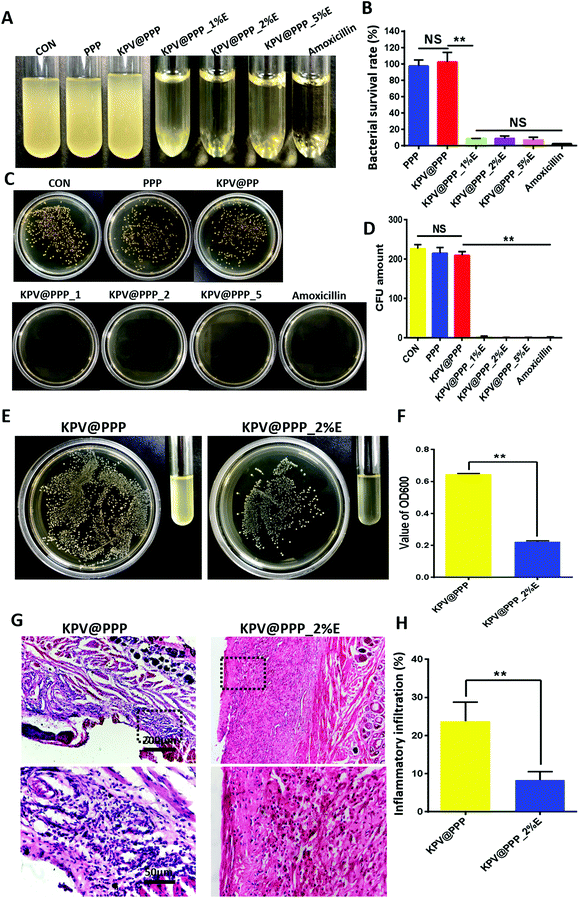 |
| Fig. 8 Antibacterial activity of KPV@PPP_E hydrogel in vivo and in vitro: (A) macroscopic image of the antibacterial effects of KPV@PPP_E in vitro; (B) bacterial survival rate of different formulations (normalized by the CON group); (C) photo and (D) quantitative analysis of a bacterial colony of S. aureus incubated with different formulations; (E) macroscopic image and (F) quantitative analysis of the residual MRSA bacteria at the wound zone after KPV@PPP or KPV@PPP_2%E treatment; (G) HE staining of the infected wound after KPV@PPP or KPV@PPP_2%E treatment and (H) statistics of inflammatory cell infiltration based on HE staining (*p < 0.05, **p < 0.01, NS means no statistical significance, n = 3). | |
To confirm the in vivo antibacterial effect of KPV@PPP_E hydrogel on ulcer wounds, the wound zone on day 3 was infected with MRSA suspension. Next day, the infected rat was treated with KPV@PPP_2%E or KPV@PPP. The residual number of MRSA bacteria was detected on the next day after treatment and the results are shown in Fig. 8A and B. In comparison with KPV@PPP, obvious inhibition of MRSA bacteria was presented by KPV@PPP_2%E. The residual number of MRSA bacteria at the wound zone after KPV@PPP_2%E treatment was 3.1-fold lower than that in KPV@PPP treatment, indicating the strong antibacterial effect of KPV@PPP_E hydrogel in vivo. Moreover, the antibacterial effect of KPV@PPP_E hydrogel arises from EGCG instead of the other ingredients in the hydrogel. The strong antibacterial activity of EGCG was also demonstrated in a previous publication.12 In addition, the infected wound was further stained to observe the infiltration of inflammatory cells and the results are shown in Fig. 8C and D. As expected, an excess of inflammatory cells infiltrated the submucosal tissues in the KPV@PPP group because of their severe MRSA infection. By contrast, the infiltration of inflammatory cells was largely inhibited after KPV@PPP_2%E treatment (Fig. 8D). These results may suggest that the antibacterial activity of EGCG also contributed to inflammation alleviation for KPV@PPP_2%E treatment. Collectively, the combination of EGCG and KPV in KPV@PPP_E hydrogel produced a positive effect on the wound repair of oral gingival ulcers.
4 Conclusions
In this study, in situ mucoadhesive hydrogels (PPP_E) were successfully prepared by using temperature-sensitive PLGA-PEG-PLGA (PPP) as a matrix and EGCG as an adhesion enhancer. A series of PPP_E precursor solutions with various EGCG concentrations (1%, 2% and 5%) were prepared by fixing the PPP concentration at 25%. The addition of EGCG slightly decreased the sol–gel transition temperature and shortened the sol–gel transition time. Moreover, the incorporation of EGCG could significantly increase the tissue adhesion properties of the PPP hydrogel at 37 °C. Tripeptide KPV as a model drug was easily dissolved in cold PPP_E precursor solution to prepare the KPV@PPP_E hydrogel. The anti-inflammatory activity and promotion of cell migration potential of KPV in the PPP-E hydrogel were well maintained. Moreover, KPV@PPP_E exhibited strong antibacterial efficacy against S. aureus. In vivo animal experiments showed that treatment with KPV@PPP_2%E hydrogel greatly improved the food intake and body weight recovery of rats with chemotherapy-induced oral mucositis. Moreover, the tissue morphology of the ulcerated gingiva after treatment with KPV@PPP_E hydrogel was also well repaired by promoting CK10 and PCNA expression. In addition, the inflammatory cytokines were significantly inhibited by KPV@PPP_E hydrogel. The KPV@PPP_E hydrogel also had an anti-bacterial effect on MRSA-infected gingival ulcer wounds, which resulted in obvious inhibition of inflammatory cell infiltration into submucosal tissues. In conclusion, KPV@PPP_E may have promising practical application for cancer patients with chemotherapy-induced oral mucositis.
Conflicts of interest
No conflict of interest exits in this study and manuscript is approved by all authors for publication.
Acknowledgements
This research was supported by Wenzhou Science and Technology Bureau (Y20210211), Wenzhou Scientific and Technological Innovation Project (Grant No. ZY2021019), Wenzhou Innovative Technology Project for High-level Talents (Y.-Z. Z.), Zhejiang Provincial Foundation for Health Department (Grant No. 2021KY198), Zhejiang Provincial Natural Science Foundation (Grant No. LY20H300002), Excellent Youth Talents of Wenzhou Medical University (H-L Xu) and Scientific and Technological Innovation Plan of College Students in Zhejiang Province (2021R413014).
References
- M. Cinausero, G. Aprile, P. Ermacora, D. Basile, M. G. Vitale and V. Fanotto,
et al., New Frontiers in the Pathobiology and Treatment of Cancer Regimen-Related Mucosal Injury, Front. Pharmacol., 2017, 8, 354 CrossRef.
- H. Jung, H. S. Kim, J. H. Lee, J. J. Lee and H. S. Park, Wound Healing Promoting Activity of Tonsil-Derived Stem Cells on 5-Fluorouracil-Induced Oral Mucositis Model, Tissue Eng. Regener. Med., 2020, 17, 105–119 CrossRef CAS.
- A. Trotti, L. Bellm, J. Epstein, D. Frame, H. Fuchs and C. Gwede,
et al., Mucositis incidence, severity and associated outcomes in patients with head and neck cancer receiving radiotherapy with or without chemotherapy: a systematic literature review, Radiother. Oncol., 2003, 66, 253–262 CrossRef.
- K. Yamaguchi, K. Ono, S. Hitomi, M. Ito, T. Nodai and T. Goto,
et al., Distinct TRPV1- and TRPA1-based mechanisms underlying enhancement of oral ulcerative mucositis-induced pain by 5-fluorouracil, Pain, 2016, 157, 1004–1020 CrossRef CAS.
- T. Brzoska, M. Böhm, A. Lügering, K. Loser and T. Luger, Terminal signal: anti-inflammatory effects of α-melanocyte-stimulating hormone related peptides beyond the pharmacophore, Adv. Exp. Med. Biol., 2010, 681, 107–116 CrossRef CAS.
- M. Böhm and T. Luger, Are melanocortin peptides future therapeutics for cutaneous wound healing?, Exp. Dermatol., 2019, 28, 219–224 CrossRef.
- M. Zeng, A. Shao, H. Li, Y. Tang, Q. Li and Z. Guo,
et al., Peptide Receptor-Targeted Fluorescent Probe: Visualization and Discrimination between Chronic and Acute Ulcerative Colitis, ACS Appl. Mater. Interfaces, 2017, 9, 13029–13036 CrossRef CAS PubMed.
- V. Bonfiglio, G. Camillieri, T. Avitabile, G. Leggio and F. Drago, Effects of the COOH-terminal tripeptide alpha-MSH(11-13) on corneal epithelial wound healing: role of nitric oxide, Exp. Eye Res., 2006, 83, 1366–1372 CrossRef CAS.
- G. Dalmasso, L. Charrier-Hisamuddin, H. Nguyen, Y. Yan, S. Sitaraman and D. Merlin, PepT1-mediated tripeptide KPV uptake reduces intestinal inflammation, Gastroenterology, 2008, 134, 166–178 CrossRef CAS PubMed.
- M. Kudo, K. Kobayashi-Nakamura, N. Kitajima and K. Tsuji-Naito, Alternate expression of PEPT1 and PEPT2 in epidermal differentiation is required for NOD2 immune responses by bacteria-derived muramyl dipeptide, Biochem. Biophys. Res. Commun., 2020, 522, 151–156 CrossRef CAS.
- S. Zhang, R. Langer and G. Traverso, Nanoparticulate Drug Delivery Systems Targeting Inflammation for Treatment of Inflammatory Bowel Disease, Nano Today, 2017, 16, 82–96 CrossRef CAS.
- S. Lee, G. Razqan and D. Kwon, Antibacterial activity of epigallocatechin-3-gallate (EGCG) and its synergism with β-lactam antibiotics sensitizing carbapenem-associated multidrug resistant clinical isolates of Acinetobacter baumannii, Phytomedicine, 2017, 24, 49–55 CrossRef CAS.
- F. Sun, Y. Bu, Y. Chen, F. Yang, J. Yu and D. Wu, An Injectable and Instant Self-Healing Medical Adhesive for Wound Sealing, ACS Appl. Mater. Interfaces, 2020, 12, 9132–9140 CrossRef CAS.
- S. Kim, K. Kim, B. Kim, Y. An, U. Lee and S. Lee,
et al., Fabrication of polyphenol-incorporated anti-inflammatory hydrogel via high-affinity enzymatic crosslinking for wet tissue adhesion, Biomaterials, 2020, 242, 119905 CrossRef CAS.
- W. Zhu, H. Mei, L. Jia, H. Zhao, X. Li and X. Meng,
et al., Epigallocatechin-3-gallate mouthwash protects mucosa from radiation-induced mucositis in head and neck cancer patients: a prospective, non-randomised, phase 1 trial, Invest. New Drugs, 2020, 38, 1129–1136 CrossRef CAS PubMed.
- S. Sang, J. Lambert, C. Ho and C. Yang, The chemistry and biotransformation of tea constituents, Pharmacol. Res., 2011, 64, 87–99 CrossRef CAS.
- T. Huang, H. Lu, Y. Ho, K. Lu, P. Wang and F. Mi, A smart and active film with tunable drug release and color change abilities for detection and inhibition of bacterial growth, Mater. Sci. Eng., C, 2021, 118, 111396 CrossRef CAS.
- B. Hu, Y. Shen, J. Adamcik, P. Fischer, M. Schneider and M. J. Loessner,
et al., Polyphenol-Binding Amyloid Fibrils Self-Assemble into Reversible Hydrogels with Antibacterial Activity, ACS Nano, 2018, 12, 3385–3396 CrossRef CAS.
- J. Ryu, J. Choi, E. Park, M. Eom, S. Jo and M. Lee,
et al., Chitosan oral patches inspired by mussel adhesion, J. Controlled Release, 2020, 317, 57–66 CrossRef CAS.
- A. Al-Taie, A. Al-Shohani, Z. Albasry and A. Altaee, Current topical trends and novel therapeutic approaches and delivery systems for oral mucositis management, J. Pharm. BioAllied Sci., 2020, 12, 94–101 CrossRef CAS PubMed.
- J. Xu, S. Strandman, J. Zhu, J. Barralet and M. Cerruti, Genipin-crosslinked catechol-chitosan mucoadhesive hydrogels for buccal drug delivery, Biomaterials, 2015, 37, 395–404 CrossRef CAS.
- Y. Murata, K. Kofuji, N. Nishida and R. Kamaguchi, Development of film dosage form containing allopurinol for prevention and treatment of oral mucositis, ISRN Pharm., 2012, 2012, 764510 Search PubMed.
- R. Allison, A. Ambrad, Y. Arshoun, R. Carmel, D. Ciuba and E. Feldman,
et al., Multi-institutional, randomized, double-blind, placebo-controlled trial to assess the efficacy of a mucoadhesive hydrogel (MuGard) in mitigating oral mucositis symptoms in patients being treated with chemoradiation therapy for cancers of the head and neck, Cancer, 2014, 120, 1433–1440 CrossRef.
- R. Gilhotra, M. Ikram, S. Srivastava and N. Gilhotra, A clinical perspective on mucoadhesive buccal drug delivery systems, J. Biomed. Res., 2014, 28, 81–97 Search PubMed.
- R. Reda, M. Wen and A. El-Kamel, Ketoprofen-loaded Eudragit electrospun nanofibers for the treatment of oral mucositis, Int. J. Nanomed., 2017, 12, 2335–2351 CrossRef CAS PubMed.
- N. Nagai, R. Seiriki, S. Deguchi, H. Otake, N. Hiramatsu and H. Sasaki,
et al., Hydrogel Formulations Incorporating Drug Nanocrystals Enhance the Therapeutic Effect of Rebamipide in a Hamster Model for Oral Mucositis, Pharmaceutics, 2020, 12, 532–544 CrossRef CAS.
- S. Xian and M. Webber, Temperature-responsive supramolecular hydrogels, J. Mater. Chem. B, 2020, 8, 9197–9211 RSC.
- D. Saunders, J. Epstein, S. Elad, J. Allemano, P. Bossi and M. van de Wetering,
et al., Systematic review of antimicrobials, mucosal coating agents, anesthetics, and analgesics for the management of oral mucositis in cancer patients, Supportive Care Cancer, 2013, 21, 3191–3207 CrossRef PubMed.
- Y. Chen, J. Shi, Y. Zhang, J. Miao, Z. Zhao and X. Jin,
et al., An injectable thermosensitive hydrogel loaded with an ancient natural drug colchicine for myocardial repair after infarction, J. Mater. Chem. B, 2020, 8, 980–992 RSC.
- C. Pratoomsoot, H. Tanioka, K. Hori, S. Kawasaki, S. Kinoshita and P. J. Tighe,
et al., A thermoreversible hydrogel as a biosynthetic bandage for corneal wound repair, Biomaterials, 2008, 29, 272–281 CrossRef CAS.
- L. F. Wang, J. W. Xu, P. P. Xue, J. Y. Liu, L. Z. Luo and D. L. Zhuge,
et al., Thermo-sensitive hydrogel with mussel-inspired adhesion enhanced the non-fibrotic repair effect of EGF on colonic mucosa barrier of TNBS-induced ulcerative colitis rats through macrophage polarizing, Chem. Eng. J., 2021, 416, 29221–29243 Search PubMed.
- C. Wang, Y. Wang, L. Zhang, R. Miron, J. Liang and M. Shi,
et al., Pretreated Macrophage-Membrane-Coated Gold Nanocages for Precise Drug Delivery for Treatment of Bacterial Infections, Adv. Mater., 2018, 30, e1804023 CrossRef PubMed.
- H. Xu, W. Yang, P. Chen, R. Chen, P. Xue and L. Wang,
et al., Bone-Inspired Tube Filling Decellularized Matrix of Toad Cartilage Provided an Osteoinductive Microenvironment for Mesenchymal Stem Cells to Facilitate the Radius Defect Repair of Rabbit, Biotechnol. J., 2020, 15, e2000004 CrossRef PubMed.
- J. W. Park, J. Oh, S. J. Ko, M. S. Chang and J. Kim, Effects of Onchung-eum, an Herbal Prescription, on 5-Fluorouracil-Induced Oral Mucositis, Integr. Cancer Ther., 2018, 17, 1285–1296 CrossRef PubMed.
- L. Feng, J. Ward, S. Li, G. Tolia, J. Hao and D. Choo, Assessment of PLGA-PEG-PLGA copolymer hydrogel for sustained drug delivery in the ear, Curr. Drug Delivery, 2014, 11, 279–286 CrossRef CAS.
- P. Wang, W. Chu, X. Zhuo, Y. Zhang, J. Gou and T. Ren,
et al., Modified PLGA-PEG-PLGA thermosensitive hydrogels with suitable thermosensitivity and properties for use in a drug delivery system, J. Mater. Chem. B, 2017, 5, 1551–1565 RSC.
- C. E. Echeverri-Cuartas, N. A. Agudelo and C. Gartner, Chitosan-PEG-folate-Fe(III) complexes as nanocarriers of epigallocatechin-3-gallate, Int. J. Biol. Macromol., 2020, 165, 2909–2919 CrossRef CAS PubMed.
- H. Laroui, G. Dalmasso, H. T. Nguyen, Y. Yan, S. V. Sitaraman and D. Merlin, Drug-loaded nanoparticles targeted to the colon with polysaccharide hydrogel reduce colitis in a mouse model, Gastroenterology, 2010, 138, 843–53 CrossRef CAS.
- S. Hobbs, M. Reynoso, A. Geddis, A. Mitrophanov and R. Matheny, LPS-stimulated NF-κB p65 dynamic response marks the initiation of TNF expression and transition to IL-10 expression in RAW 264.7 macrophages, Physiol. Rep., 2018, 6, e13914 Search PubMed.
- O. Pleguezuelos, S. Dainty, S. Kapas and J. Taylor, A human oral keratinocyte cell line responds to human heat shock protein 60 through activation of ERK1/2 MAP kinases and up- regulation of IL-1beta, Clin. Exp. Immunol., 2005, 141, 307–314 CrossRef CAS.
- K. Vaillancourt, A. Ben Lagha and D. Grenier, A green tea extract and epigallocatechin-3-gallate attenuate the deleterious effects of irinotecan in an oral epithelial cell model, Arch. Oral Biol., 2021, 126, 105135 CrossRef CAS PubMed.
- B. Singh, S. Shankar and R. Srivastava, Green tea catechin, epigallocatechin-3-gallate (EGCG): mechanisms, perspectives and clinical applications, Biochem. Pharmacol., 2011, 82, 1807–1821 CrossRef CAS PubMed.
- L. Sayadi, S. Varaei and M. Babazadeh Zanjani, The Effect of Family-Centered Supportive Program on Chemotherapy-Induced Symptoms in Patients with Acute Lymphoblastic Leukemia (ALL), Int. J. Hematol.-Oncol. Stem Cell Res., 2021, 15, 35–50 Search PubMed.
- I. Takeuchi, R. Kawamata and K. Makino, Effects of GGsTop on Collagen and Glutathione in the Oral Mucosa Using a Rat Model of 5-Fluorouracil-Induced Oral Mucositis, In Vivo, 2021, 35, 175–180 CrossRef CAS PubMed.
- X. Kong, J. Fu, K. Shao, L. Wang, X. Lan and J. Shi, Biomimetic hydrogel for rapid and scar-free healing of skin wounds inspired by the healing process of oral mucosa, Acta Biomater., 2019, 100, 255–269 CrossRef CAS PubMed.
- S. Groeger and J. Meyle, Oral Mucosal Epithelial Cells, Front. Immunol., 2019, 10, 208 CrossRef CAS.
- Q. Jiang and D. Li, [Cytokeratin expression in human junctional epithelium, oral epithelium and sulcular epithelium], Chin. J. Stomatol., 2005, 40, 298–301 CAS.
- K. Marynka-Kalmani, S. Treves, M. Yafee, H. Rachima, Y. Gafni and M. Cohen,
et al., The lamina propria of adult human oral mucosa harbors a novel stem cell population, Stem Cells, 2010, 28, 984–995 CrossRef CAS.
- M. Marinella, Routine antiemetic prophylaxis with dexamethasone during COVID-19: Should oncologists reconsider?, J. Oncol. Pharm. Pract., 2020, 26, 1482–1485 CrossRef CAS.
- M. Bohm and T. Luger, Are melanocortin peptides future therapeutics for cutaneous wound healing?, Exp. Dermatol., 2019, 28, 219–224 CrossRef PubMed.
- M. Zeng, A. Shao, H. Li, Y. Tang, Q. Li and Z. Guo,
et al., Peptide Receptor-Targeted Fluorescent Probe: Visualization and Discrimination between Chronic and Acute Ulcerative Colitis, ACS Appl. Mater. Interfaces, 2017, 9, 13029–13036 CrossRef CAS PubMed.
- S. C. Land, Inhibition of cellular and systemic inflammation cues in human bronchial epithelial cells by melanocortin-related peptides: mechanism of KPV action and a role for MC3R agonists, Int. J. Physiol., Pathophysiol. Pharmacol., 2012, 4, 59–73 CrossRef CAS.
- T. Larsen and N. Fiehn, Dental biofilm infections - an update, APMIS, 2017, 125, 376–384 CrossRef CAS.
- I. Fragkioudakis, M. Riggio and D. Apatzidou, Understanding the microbial components of periodontal diseases and periodontal treatment-induced microbiological shifts, J. Med. Microbiol., 2021, 70 DOI:10.1099/jmm.0.00124.
- K. Wang, J. Wang, L. Li, L. Xu, N. Feng and Y. Wang,
et al., Novel Nonreleasing Antibacterial Hydrogel Dressing by a One-Pot Method, ACS Biomater. Sci. Eng., 2020, 6, 1259–1268 CrossRef CAS PubMed.
- A. Renzetti, J. Betts, K. Fukumoto and R. Rutherford, Antibacterial green tea catechins from a molecular perspective: mechanisms of action and structure-activity relationships, Food Funct., 2020, 11, 9370–9396 RSC.
- A. Kitichalermkiat, M. Katsuki, J. Sato, T. Sonoda, Y. Masuda and K. I. Honjoh,
et al., Effect of epigallocatechin gallate on gene expression of Staphylococcus aureus, J. Glob. Antimicrob. Resist., 2020, 22, 854–859 CrossRef PubMed.
Footnote |
† The first three authors contributed equally to this work. |
|
This journal is © The Royal Society of Chemistry 2022 |
Click here to see how this site uses Cookies. View our privacy policy here.