DOI:
10.1039/D1RA07266H
(Paper)
RSC Adv., 2021,
11, 39934-39939
Ligand-enabled and magnesium-activated hydrogenation with earth-abundant cobalt catalysts†
Received
29th September 2021
, Accepted 9th December 2021
First published on 15th December 2021
Abstract
Replacing expensive noble metals like Pt, Pd, Ir, Ru, and Rh with inexpensive earth-abundant metals like cobalt (Co) is attracting wider research interest in catalysis. Cobalt catalysts are now undergoing a renaissance in hydrogenation reactions. Herein, we describe a hydrogenation method for polycyclic aromatic hydrocarbons (PAHs) and olefins with a magnesium-activated earth-abundant Co catalyst. When diketimine was used as a ligand, simple and inexpensive metal salts of CoBr2 in combination with magnesium showed high catalytic activity in the site-selective hydrogenation of challenging PAHs under mild conditions. Co-catalyzed hydrogenation enabled the reduction of two side aromatics of PAHs. A wide range of PAHs can be hydrogenated in a site-selective manner, which provides a cost-effective, clean, and selective strategy to prepare partially reduced polycyclic hydrocarbon motifs that are otherwise difficult to prepare by common methods. The use of well-defined diketimine–ligated Co complexes as precatalysts for selective hydrogenation of PAHs and olefins is also demonstrated.
Earth-abundant metal-catalyzed hydrogenation provides a cost-effective and atom-economic strategy for sustainable chemical synthesis; thus, it has attracted considerable interest among the synthetic community.1–4 Several inexpensive first-row transition metals such as iron,5 nickel,6 cobalt,7 and manganese8 have been shown to have appealing catalytic activities in the hydrogenation of olefins, ketones, imines, and even amides and esters.9 In contrast, polycyclic aromatic hydrocarbons (PAHs) have rarely been hydrogenated by earth-abundant metal catalysts, probably due to their stability caused by aromaticity.10 Catalytic hydrogenation of PAHs has been dominated by precious metals such as ruthenium, rhodium, palladium, and platinum via heterogeneous catalysis (Scheme 1).11 Potassium bis(anthracene)cobaltate has been reported by Jacobi von Wangelin et al.7a for the hydrogenation reactions of unsaturated molecules (alkenes, arenes, carbonyl compounds). In addition, they have also reported the synthesis of potassium bis(η4-anthracene) metalates of Fe and Co for catalytic hydrogenation of alkenes, ketones and imines at ambient H2 pressure and temperatures.7i
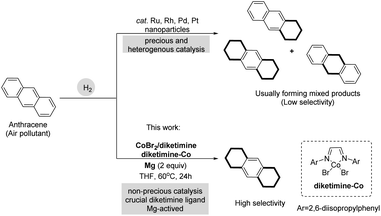 |
| Scheme 1 Transition-metal-catalyzed hydrogenation of PAH (anthracene). | |
Site selectivity in PAH hydrogenation has long been an important issue. Mixed products are usually formed upon hydrogenation of aromatic scaffolds at different sites, with limited PAH scope.12 The use of earth-abundant metals for catalytic hydrogenation of PAHs with site-selective and cost-effective method would be of greater value for partially hydrogenated feedstock chemicals synthesis.
In nonprecious-metal-promoted catalysis, an activation strategy involving the reduction of simple, low-cost and commercially available metal salts for in situ formation of active metal catalysts has been established. It offer the advantages of easy handling and potential applications in large-scale manufacturing, with minimal associated hazards in the purification of metal catalysts.13,14
We hypothesized whether it will be possible to use earth-abundant metal salts as precatalysts to replace expensive metal catalysts, using an in situ activation strategy for achieving the hydrogenation of PAHs with controllable selectivity. Hydrogenation using robust Fe, Co, and Mn catalysts has been reported. However, the hydrogenation of PAHs using these inexpensive metal catalysts has been rather limited.15,16 Zeng's group has developed low-valent cobalt in situ using methylmagnesium bromide as a reducing agent for the hydrogenation of fused PAHs to give two terminal aromatic carbocycles.17
Herein, we report a ligand-enabled and magnesium-activated hydrogenation of PAHs and olefins with in-expensive metal Co catalysis. Site selectivity in the hydrogenation of PAHs can be controlled by these two different, earth-abundant metal catalysts. A combination of CoBr2 and diketimine or a diketimine–ligated Co complex with magnesium as a reductant showed good catalytic activity in the selective hydrogenation of two side aromatics of PAHs (Scheme 1). Preactivation with Mg was performed by placing Mg turnings and CoBr2 in Schlenk tube and heating under vacuum for 5–8 minutes using a heat gun.
In our efforts to form low-oxidation-state active Co species in situ, we commenced our studies by selecting metallic magnesium as reductant, to test its reactivity in the hydrogenation of anthracene (Table 1).18 Initially, we selected CoCl2 salt as the catalyst and examined it under various reaction conditions. In the absence of external ligands, the low-cost CoCl2 salt with magnesium as reductant was unable to promote the hydrogenation (entry 1). Screening of ligands showed that 2,2′-bipyridine was inefficient in the hydrogenation (entry 2). Interestingly, Co-catalyzed hydrogenation occurred when using dtbpy as ligand; a product with one reduced aromatic side arm was formed (entry 3), only a trace amount of the two-side-aromatic-reduced product 3a was detected. We recognized that the ligand could play an important role in promoting hydrogenation with Co. Other ligands such as IPr, dppp, Xantphos, and diamino L1 were inefficient in the promotion of the Co-catalyzed hydrogenation (entries 4–7). N-2,6-Diisopropylphenyl-bearing diketimine greatly enhanced the catalytic activity of Co to afford 3a in 59% yield (entry 8). However, with 2,3-dimethyl-substituted diketimine, a trace amount of product 3a was detected (entry 9). Encouraged by the results, we chose different cobalt salts as catalysts. When using Co(acac)2 salt, no hydrogenation took place (entry 10). To our delight, the salt of CoBr2 exhibited high reactivity; it resulted in catalytic hydrogenation of two side aromatics of anthracene in the formation of product 3a (entry 11). Manganese and zinc could not serve as efficient reductants in Co catalysis (entry 12).
Table 1 Studying the effect of metal salts, ligands and reductants on selective hydrogenation of anthracenea
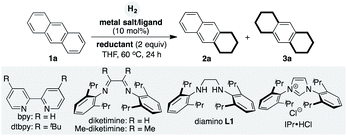
|
Entry |
Metal salt |
Ligand |
Reductant |
[2a %] |
[3a %] |
Conditions: 1a (0.2 mmol), metal salt (0.02 mmol), ligand (0.02 mmol), reductant (0.4 mmol), H2 (8 MPa), THF (2 mL), 60 °C, 48 h. 1H NMR yields using 1,3,5-trimethoxybenzene as internal standard. Not detected. Isolated yield. dppp = 1,3-bis(diphenylphosphino)propane. Xantphos = 4,5-bis(diphenyl phosphino)-9,9-dimethylxanthene. Without H2. |
1 |
CoCl2 |
None |
Mg |
ndb |
ndb |
2 |
CoCl2 |
Bpy |
Mg |
ndb |
ndb |
3 |
CoCl2 |
Dtbpy |
Mg |
38 |
Trace |
4 |
CoCl2 |
IPr·HCl |
Mg |
ndb |
ndb |
5 |
CoCl2 |
Dppp |
Mg |
ndb |
ndb |
6 |
CoCl2 |
Xantphos |
Mg |
ndb |
ndb |
7 |
CoCl2 |
Diamino L1 |
Mg |
ndb |
ndb |
8 |
CoCl2 |
Diketimine |
Mg |
26 |
59 |
9 |
CoCl2 |
Me-diketimine |
Mg |
37 |
Trace |
10 |
Co(acac)2 |
Diketimine |
Mg |
ndb |
ndb |
11 |
CoBr2 |
Diketimine |
Mg |
ndb |
90 [84]c |
12 |
CoBr2 |
Diketimine |
Mn or Zn |
ndb |
ndb |
13 |
CoBr2 |
No |
Mg |
ndb |
ndb |
14 |
CoBr2 |
Diketimine |
No |
ndb |
ndb |
15 |
CoBr2 |
Diketimine |
CH3Li |
ndb |
ndb |
16 |
CoBr2 |
Diketimine |
NaOtBu |
ndb |
ndb |
17 |
CoBr2 |
Diketimine |
NaHBEt3 |
ndb |
ndb |
18 |
CoBr2 |
Diketimine |
NaOtBu/HBPin |
ndb |
ndb |
19d |
CoBr2 |
Diketimine |
NaOtBu/HBPin |
ndb |
ndb |
Once we had the optimal hydrogenation conditions with CoBr2 and diketimine ligand in hand, we were curious as to whether well-defined diketimine–ligated Co complexes could serve as precatalysts to promote the hydrogenation of PAH. According to known procedures,19 we prepared the diketimine–ligated Co complexes and tested their reactivities in the hydrogenation of anthracene. Briefly, diketimine–ligated Co complexes were prepared by mixing equimolar anhydrous CoBr2 and diketimine in THF and stirred for 24 h at room temperature, filtered, washed with hexane, and dried in vacuum. The diketimine–Co complex afforded the product 3a in almost equal conversion to that using CoBr2/diketimine (Scheme 2). Catalytic hydrogenation of PAHs was carried out by heating Mg turnings (10 mg, 0.4 mmol) and CoBr2 (5 mg, 0.02 mmol) in a Schlenk tube using a heat gun at around 300 °C under vacuum for 5 min. The mixture was cooled to room temperature in nitrogen atmosphere, PAH (0.2 mmol), diketimine (8 mg, 0.02 mmol) and THF (2.0 mL) were added. The resulting mixture was heated at 80 °C for 2 hours. Then the resulting mixture was quickly moved to a high-pressure autoclave. The reaction mixture was stirred under an atmosphere of H2 (8 Mpa) at 60 °C for 50 h and then quenched with saturated NH4Cl/H2O (4 mL). The crude product was extracted with EtOAc (3 × 4 mL). The combined organic phases were dried over anhydrous Na2SO4 and concentrated under vacuum. After removal of the volatiles under vacuum, the crude products were purified by column chromatography to afford the desired hydrogenation compounds.
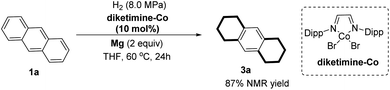 |
| Scheme 2 Testing the catalytic activities of well-defined diketimine–Co complexes in hydrogenation of anthracene. | |
With optimal reaction conditions in hand, CoBr2/diketimine was further used as a precatalyst to explore the scope of Co-catalyzed hydrogenation for the synthesis of two-side-aromatic-reduced motifs (Scheme 3).
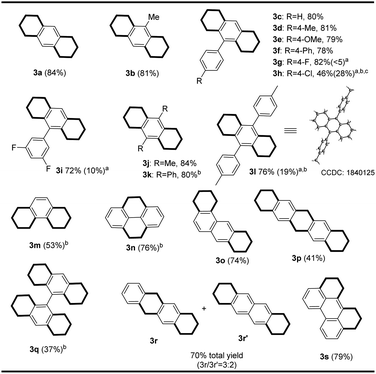 |
| Scheme 3 Co-catalyzed selective hydrogenation of PAHs. PAH (0.2 mmol), CoBr2 (0.02 mmol), diketimine (0.02 mmol), Mg (0.4 mmol), H2 (8 MPa), THF (2 mL), 60 °C, 48 h. Isolated yields. aYields of compounds that were formed by reducing one side arenes of PHAs. b60 h. cMg (3.5 equiv.) was used. | |
1,2,3,4,5,6,7,8-Octahydroanthracene derivatives bearing methyl and substituted phenyl substituents at the C9/C10 positions were prepared by selectively reducing two side carbocycles (3b–3l). As expected, the hydrogenation of phenanthrene readily took place with Co catalysis (3m). When using pyrene, we found that internal carbocycles were reduced to afford 4,5,9,10-tetrahydropyrene (3n). This protocol was extended to the preparation of partially reduced compounds of 1,2,3,4,8,9,10,11-octahydrotetraphene and 1,2,3,10,11,12-hexahydroperylene, by the reduction of related PAH precursors (3o and 3s). It was interesting to note that the hydrogenation of pentacene unexpectedly afforded 1,2,3,4,6,8,9,10,11,13-decahydropentacene 3p by selective reduction of three carbocycles. A further interesting observation was that four side carbocycles in bianthracene were hydrogenated to form hexadecahydro-9,9′-bianthracene (3q). This differs from what was observed in the Cr catalysis protocol; the formation of a mixed regioisomer (3r and 3r′) was found in the hydrogenation of tetracene by Co catalysis. This reaction was not tolerable for substrates bearing reducible groups such as Br, COOR, COOH, CHO, CN and NO2.
The diketimine–Co-catalyzed hydrogenation protocols can be utilized to reduce substituted olefins (Scheme 4).5c,5d,7a,7b The double bonds were reduced, with retention of the phenyl and naphthyl groups, affording linear or branched alkylarene motifs in good yields. Moreover, olefin hydrogenation using diketimine–ligated Co complexes took place smoothly (Scheme 5).
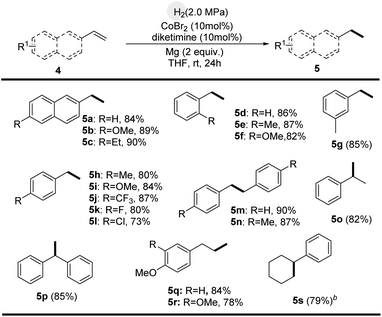 |
| Scheme 4 Diketimine–Co-catalyzed hydrogenation of olefins. | |
 |
| Scheme 5 Testing the catalytic activities of diketimine–Co complexes in hydrogenation of olefins. | |
To examine whether a low-oxidation-state Co species is formed by the reduction of a CoBr2 salt with magnesium, we performed X-ray photoelectron spectroscopy (XPS) analysis of the oxidation states of Co in the stoichiometric reactions of CoBr2 with diketimine in the absence or presence of magnesium. Downshift signals with a shift distance of 0.89 eV for the Co 2p components in the related spectra were observed when conducting the reaction with magnesium in THF (Fig. 1). This shows that low-oxidation-state Co species are formed in situ by the reduction of CoBr2 with magnesium, which then serve as active species to initiate the hydrogenation. The π-acceptors (arenes, olefins etc.) are able to stabilize low oxidation state reactive metal spices. Previously, it has been reported that Co catalyzed reaction were initiated by the substitution of the labile arenes molecules by π-acceptor substrates.7i Jacobi von Wangelin et al. have reported arene–Co and arene–Fe catalysts for hydrogenations of olefins, alkenes, etc. and found that slow hydrogenation of anthracene occurred when there was no suitable π-acceptor.7i We also performed controlled experiments of the low-cost CoBr2 salt with magnesium in the absence of diketimine ligand (Table 1, entry 13), but were failed to get hydrogenated anthracene. Additionally, we also checked the Jacobi von Wagelin's arene–M(0) complexes,7i using anthracene instead of diketimine as ligand for the catalytic hydrogenation of olifens but were unable to get hydrogenated products.
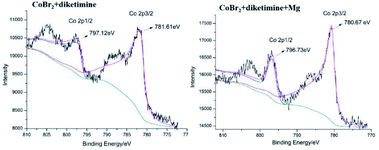 |
| Fig. 1 XPS analysis of stoichiometric reaction mixtures of CoBr2 with diketimine ligand in the absence or presence of metallic magnesium. | |
Reaction profiles for the Co-catalyzed hydrogenation of anthracene were investigated. The formation of two-side-aromatic-reduced product 3a was observed in Co catalysis within the first 10 hours (Fig. 2), and the hydrogenation rate for the conversion of 2a to 3a increased.
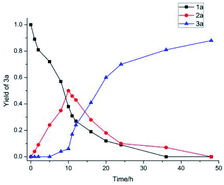 |
| Fig. 2 Reaction profiles for Co-catalyzed hydrogenation of anthracene. | |
The scalability of the hydrogenation of anthracene was examined on gram scale. It was found that performing the Co-catalyzed reactions on the scale of 10 mmol did not affect the efficiency of site-selective hydrogenation, hence permitting the scalable preparation of 3a and 5p (Scheme 6).
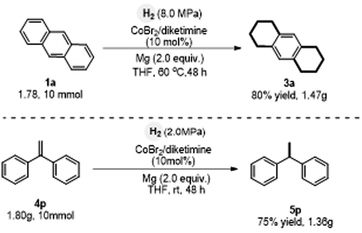 |
| Scheme 6 Gram-scale hydrogenation of 1a and 4p. | |
Conclusions
In summary, we have developed an economic and site-selective hydrogenation of challenging PAHs with earth-abundant Co catalysis.20 arene–Co and arene–Fe catalysts for hydrogenations of olefins, alkenes, ketones, and imines have been previously reported by Jacobi von Wangelin et al.7i in this study, we used low-cost and commercially available CoBr2 salts as precatalysts combined with diketimine ligand and air-stable magnesium as the reductant for site-selective hydrogenation of two side aromatic hydrocarbons of PAHs and olefins. A wide range of PAHs such as tetracene, tetraphene, perylene, pentacene, and bianthracene were amenable to the hydrogenation, providing a cost-effective and clean strategy for the synthesis of two-side-aromatic-reduced polycyclic hydrocarbon motifs in high site selectivity. The high reactivity of Co catalysis with diketimine ligand indicates that the methodology outlined herein will have potential applications in the hydrogenation of challenging substrates.
Conflicts of interest
The authors declare no competing financial interests.
Acknowledgements
We acknowledge financial support from the National Natural Science Foundation of China (22061041), National Natural Science Foundation of Shaanxi Province (2020JQ-789), Open Sharing Platform for Scientific and Technological Resources of Shaanxi Province (2021PT-004), Doctoral Research Foundation of Yan'an University (YDBK2019-60), the Shaanxi Province Training Program of Innovation and Entrepreneurship for Undergraduates (S202010719114) and Research Program of Yan'an University (YDY2020-61).
Notes and references
- Catalysis without precious metals, ed. R. M. Bullock, Wiley-VCH, Hoboken, NJ, 2010 Search PubMed.
- Handbook of Homogeneous Hydrogenation, ed. J. G. de Vries and C. J. Elsevier, Wiley-VCH, Weinheim, 2007 Search PubMed.
-
(a) F. Glorius, Asymmetric Hydrogenation of aromatic compounds, Org. Biomol. Chem., 2005, 3, 4171–4175 RSC;
(b) S. Werkmeister, J. Neumann, K. Junge and M. Beller, Pincer-Type Complexes for Catalytic (De)Hydrogenation and Transfer (De)Hydrogenation Reactions: Recent Progress, Chem.–Eur. J., 2015, 21, 12226–12250 CrossRef CAS PubMed;
(c) T. Zell and D. Milstein, Hydrogenation and Dehydrogenation Iron Pincer Catalysts Capable of Metal–Ligand Cooperation by Aromatization/Dearomatization, Acc. Chem. Res., 2015, 48, 1979–1994 CrossRef CAS PubMed;
(d) D. Zhao, L. Candish, D. Paul and F. Glorius, N-Heterocyclic Carbenes in Asymmetric Hydrogenation, ACS Catal., 2016, 6, 5978–5988 CrossRef CAS;
(e) J.-H. Xie, S.-F. Zhu and Q.-L. Zhou, Transition Metal-Catalyzed Enantioselective Hydrogenation of Enamines and Imines, Chem. Rev., 2011, 111, 1713–1760 CrossRef CAS PubMed;
(f) Y.-G. Zhou, Asymmetric Hydrogenation of Heteroaromatic Compounds, Acc. Chem. Res., 2007, 40, 1357–1366 CrossRef CAS PubMed;
(g) W. Tang and X. Zhang, New Chiral Phosphorus Ligands for Enantioselective Hydrogenation, Chem. Rev., 2003, 103, 3029–3070 CrossRef CAS;
(h) R. H. Morris, Exploiting Metal–Ligand Bifunctional Reactions in the Design of Iron Asymmetric Hydrogenation Catalysts, Acc. Chem. Res., 2015, 48, 1494–1502 CrossRef CAS;
(i) D. Zhao and F. Glorius, Angew. Enantioselective Hydrogenation of Isoquinolines, Angew. Chem. Int. Ed., 2013, 52, 9616–9618 CrossRef CAS PubMed;
(j) S. Werkmeister, K. Junge and M. Beller, Catalytic Hydrogenation of Carboxylic Acid Esters, Amides, and Nitriles with Homogeneous Catalysts, Org. Process Res. Dev., 2014, 18, 289–302 CrossRef CAS;
(k) R. Noyori, Asymmetric Catalysis: Science and Opportunities (Nobel Lecture), Angew. Chem., Int. Ed., 2002, 41, 2008–2022 CrossRef CAS.
- P. J. Chirik, Iron- and Cobalt-Catalyzed Alkene Hydrogenation: Catalysis with Both Redox-Active and Strong Field Ligands, Acc. Chem. Res., 2015, 48, 1687–1695 CrossRef CAS.
-
(a) R. V. Jagadeesh, A.-E. Surkus, H. Junge, M.-M. Pohl, J. Radnik, J. Rabeah, H. Huan, V. Schünemann, A. Brückner and M. Beller, Nanoscale Fe2O3-Based Catalysts for Selective Hydrogenation of Nitroarenes to Anilines, Science, 2013, 342, 1073–1076 CrossRef CAS PubMed;
(b) J. A. Garg, S. Chakraborty, Y. Ben-David and D. Milstein, Unprecedented Iron-catalyzed Selective Hydrogenation of Activated Amides to Amines and Alcohols, Chem. Commun., 2016, 52, 5285–5288 RSC;
(c) S. C. Bart, E. Lobkovsky and P. J. Chirik, Preparation and Molecular and Electronic Structures of Iron(0) Dinitrogen and Silane Complexes and Their Application to Catalytic Hydrogenation and Hydrosilation, J. Am. Chem. Soc., 2004, 126, 13794–13807 CrossRef CAS PubMed;
(d) T. N. Gieshoff, U. Chakraborty, M. Villa and A. Jacobi von Wangelin, Alkene Hydrogenations by Soluble Iron Nanocluster Catalysts, Angew. Chem., Int. Ed., 2017, 56, 3585–3589 CrossRef CAS PubMed;
(e) S. Fleischer, S. Zhou, K. Junge and M. Beller, General and Highly Efficient Iron-Catalyzed Hydrogenation of Aldehydes, Ketones, and α,β-Unsaturated Aldehydes, Angew. Chem., Int. Ed., 2013, 52, 5120–5124 CrossRef CAS PubMed.
-
(a) N. G. Léonard and P. J. Chirik, Air-Stable α-Diimine Nickel Precatalysts for the Hydrogenation of Hindered, Unactivated Alkenes, ACS Catal., 2018, 8, 342–348 CrossRef;
(b) X. Li, C. You, S. Li, H. Lv and X. Zhang, Nickel-Catalyzed Enantioselective Hydrogenation of β-(Acylamino)acrylates: Synthesis of Chiral β-Amino Acid Derivatives, Org. Lett., 2017, 19, 5130–5133 CrossRef CAS.
- Selected examples of Co-catalyzed hydrogenation:
(a) D. Gärtner, A. Welther, B. R. Rad, R. Wolf and A. Jacobi von Wangelin, Heteroatom-Free Arene-Cobalt and Arene-Iron Catalysts for Hydrogenations, Angew. Chem., Int. Ed., 2014, 53, 3722–3726 CrossRef PubMed;
(b) G. Zhang, B. L. Scott and S. K. Hanson, Mild and Homogeneous Cobalt-Catalyzed Hydrogenation of C=C, C=O, and C=N Bonds, Angew. Chem., Int. Ed., 2012, 51, 12102–12106 CrossRef CAS PubMed;
(c) D. Srimani, A. Mukherjee, A. F. G. Goldberg, G. Leitus, Y. Diskin-Posner, L. J. W. Shimon, Y. B. David and D. Milstein, Cobalt-Catalyzed Hydrogenation of Esters to Alcohols: Unexpected Reactivity Trend Indicates Ester Enolate Intermediacy, Angew. Chem., Int. Ed., 2015, 54, 12357–12360 CrossRef CAS PubMed;
(d) R. Adam, J. R. Cabrero-Antonino, A. Spannenberg, K. Junge and R. Jackstell, A General and Highly Selective Cobalt-Catalyzed Hydrogenation of N-Heteroarenes under Mild Reaction Conditions, Angew. Chem., Int. Ed., 2017, 56, 3216–3220 CrossRef CAS PubMed;
(e) M. R. Friedfeld, M. Shevlin, G. W. Margulieux, L.-C. Campeau and P. J. Chirik, Cobalt-Catalyzed Enantioselective Hydrogenation of Minimally Functionalized Alkenes: Isotopic Labeling Provides Insight into the Origin of Stereoselectivity and Alkene Insertion Preferences, J. Am. Chem. Soc., 2016, 138, 3314–3324 CrossRef CAS PubMed;
(f) A. Mukherjee, D. Srimani, S. Chakraborty, Y. Ben-David and D. Milstein, Selective Hydrogenation of Nitriles to Primary Amines Catalyzed by a Cobalt Pincer Complex, J. Am. Chem. Soc., 2015, 137, 8888–8891 CrossRef CAS PubMed;
(g) R. Adam, C. B. Bheeter, J. R. Cabrero-Antonino, K. Junge, R. Jackstell and M. Beller, Selective Hydrogenation of Nitriles to Primary Amines by using a Cobalt Phosphine Catalyst, ChemSusChem, 2017, 10, 842–846 CrossRef CAS PubMed;
(h) M. R. Friedfeld, G. W. Margulieux, B. A. Schaefer and P. J. Chirik, Bis(phosphine)cobalt Dialkyl Complexes for Directed Catalytic Alkene Hydrogenation, J. Am. Chem. Soc., 2014, 136, 13178–13181 CrossRef CAS PubMed;
(i) P. Büschelberger, D. Gärtner, E. Reyes-Rodriguez, F. Kreyenschmidt, K. Koszinowski, A. Jacobi von Wangelin and R. Wolf, Chem.–Eur. J., 2017, 23, 3139–3151 CrossRef PubMed.
-
(a) Y.-Q. Zou, S. Chakraborty, A. Nerush, D. Oren, Y. Diskin-Posner, Y. Ben-David and D. Milstein, Highly Selective, Efficient Deoxygenative Hydrogenation of Amides Catalyzed by a Manganese Pincer Complex via Metal–Ligand Cooperation, ACS Catal., 2018, 8, 8014–8019 CrossRef CAS PubMed;
(b) F. Kallmeier, T. Irrgang, T. Dietel and R. Kempe, Highly Active and Selective Manganese C=O Bond Hydrogenation Catalysts: The Importance of the Multidentate Ligand, the Ancillary Ligands, and the Oxidation State, Angew. Chem., Int. Ed., 2016, 55, 11806–11809 CrossRef CAS PubMed;
(c) R. van Putten, E. A. Uslamin, M. Garbe, C. Liu, A. Gonzalez-de-Castro, M. Lutz, K. Junge, E. J. M. Hensen, M. Beller, L. Lefort and E. A. Pidko, Non-Pincer-Type Manganese Complexes as Efficient Catalysts for the Hydrogenation of Esters, Angew. Chem., Int. Ed., 2017, 56, 7531–7534 CrossRef CAS PubMed.
-
(a) W. Liu, B. Sahoo, K. Junge and M. Beller, Cobalt Complexes as an Emerging Class of Catalysts for Homogeneous Hydrogenations, Acc. Chem. Res., 2018, 51, 1858–1869 CrossRef CAS PubMed;
(b) M. D. Greenhalgh, A. S. Jones and S. P. Thomas, Iron-Catalysed Hydrofunctionalisation of Alkenes and Alkynes, ChemCatChem, 2015, 7, 190–222 CrossRef CAS.
- Selected examples of hydrogenation of arenes:
(a) M. P. Wiesenfeldt, Z. Nairoukh, W. Li and F. Glorius, Hydrogenation of fluoroarenes: Direct access to all-cis-(multi)fluorinated cycloalkanes, Science, 2017, 357, 908–912 CrossRef CAS;
(b) M. P. Wiesenfeldt, T. Knecht, C. Schlepphorst and F. Glorius, Silylarene Hydrogenation: A Strategic Approach that
Enables Direct Access to Versatile Silylated Saturated Carbo- and Heterocycles, Angew. Chem., Int. Ed., 2018, 57, 8297–8300 CrossRef CAS PubMed;
(c) Y. Wei, B. Rao, X. Cong and X. Zeng, Highly Selective Hydrogenation of Aromatic Ketones and Phenols Enabled by Cyclic (Amino)(alkyl)carbene Rhodium Complexes, J. Am. Chem. Soc., 2015, 137, 9250–9253 CrossRef CAS PubMed;
(d) R. Kuwano, R. Morioka, M. Kashiwabara and N. Kameyama, Catalytic Asymmetric Hydrogenation of Naphthalenes, Angew. Chem., Int. Ed., 2012, 51, 4136–4139 CrossRef CAS PubMed.
-
(a) S.-C. Qi, X.-Y. Wei, Z.-M. Zong and Y.-K. Wang, Application of Supported Metallic Catalysts in Catalytic Hydrogenation of Arenes, RSC Adv., 2013, 3, 14219–14232 RSC;
(b) A. Gual, C. Godard, S. Castilloń and C. Claver, Soluble Transition-Metal Nanoparticles-Catalysed Hydrogenation of Arenes, Dalton Trans., 2010, 39, 11499–11512 RSC.
-
(a) J.-i. Ito, K. Sugino, S. Matsushima, H. Sakaguchi, H. Iwata, T. Ishihara and H. Nishiyama, Synthesis of NHC-Oxazoline Pincer Complexes of Rh and Ru and Their Catalytic Activity for Hydrogenation and Conjugate Reduction, Organometallics, 2016, 35, 1885–1894 CrossRef CAS;
(b) H. B. Pan and C. M. Wai, Facile Sonochemical Synthesis of Carbon Nanotube-Supported Bimetallic Pt–Rh Nanoparticles for Room Temperature Hydrogenation of Arenes, New J. Chem., 2011, 35, 1649–1660 RSC;
(c) M. Fang and R. A. Sánchez-Delgado, Ruthenium Nanoparticles Supported on Magnesium Oxide: A Versatile and Recyclable Dual-site Catalyst for Hydrogenation of Mono- and Poly-cyclic Arenes, N-heteroaromatics, and S-heteroaromatics, J. Catal., 2014, 311, 357–368 CrossRef CAS;
(d) C. Hubert, E. G. Bilé, A. Denicourt-Nowicki and A. Roucoux, Rh(0) Colloids Supported on TiO2: A Highly Active and Pertinent Tandem in Neat Water for the Hydrogenation of Aromatics, Green Chem., 2011, 13, 1766–1771 RSC.
-
(a) J. H. Docherty, J. Peng, A. P. Dominey and S. P. Thomas, Activation and Discovery of Earth-Abundant Metal Catalysts Using Sodium tert-butoxide, Nat. Chem., 2017, 9, 595–600 CrossRef CAS PubMed;
(b) M. D. Greenhalgh, D. J. Frank and S. P. Thomas, Iron-Catalysed Chemo-, Regio-, and Stereoselective Hydrosilylation of Alkenes and Alkynes using a Bench-Stable Iron(II) Pre-Catalyst, Adv. Synth. Catal., 2014, 356, 584–590 CrossRef CAS.
- K. Gao and N. Yoshikai, Low-Valent Cobalt Catalysis: New Opportunities for C–H Functionalization, Acc. Chem. Res., 2014, 47, 1208–1219 CrossRef CAS PubMed.
- S. Friedman, S. Metlin, A. Svedi and I. Wender, Selective Hydrogenation of Polynuclear Aromatic Hydrocarbons, J. Org. Chem., 1959, 24, 1287–1289 CrossRef CAS.
-
(a) F. Nador, Y. Moglie, C. Vitale, M. Yus, F. Alonso and G. Radivoy, Reduction of Polycyclic Aromatic Hydrocarbons Promoted by Cobalt or Manganese Nanoparticles, Tetrahedron, 2010, 66, 4318–4325 CrossRef CAS;
(b) E. Nelkenbaum, I. Dror and B. Berkowitz, Reductive Hydrogenation of Polycyclic Aromatic Hydrocarbons Catalyzed by Metalloporphyrins, Chemosphere, 2007, 68, 210–217 CrossRef CAS.
- B. Han, P. Ma, X. Cong, H. Chen and X. Zeng, Chromium- and Cobalt-Catalyzed, Regiocontrolled Hydrogenation of Polycyclic Aromatic Hydrocarbons: A Combined Experimental and Theoretical Study, J. Am. Chem. Soc., 2019, 141, 9018–9026 CrossRef CAS PubMed.
- M. T. Mock, S. Chen, M. O'Hagan, R. Rousseau, W. G. Dougherty, W. S. Kassel and R. M. Bullock, Dinitrogen Reduction by a Chromium(0) Complex Supported by a 16-Membered Phosphorus Macrocycle, J. Am. Chem. Soc., 2013, 135, 11493–11496 CrossRef CAS PubMed.
- T. V. Laine, M. Klinga, A. Maaninen, E. Aitola and M. Leskelä, Effect of Metal on the Ethylene Polymerization Behavior of a Diimine-Based Homogeneous Late Transition Metal Catalyst System, Acta Chem. Scand., 1999, 53, 968–973 CrossRef CAS.
- J. Schneidewind, R. Adam, W. Baumann, R. Jackstell and M. Beller, Low-Temperature Hydrogenation of Carbon Dioxide to Methanol with a Homogeneous Cobalt Catalyst, Angew. Chem., Int. Ed., 2017, 56, 1890–1893 CrossRef CAS PubMed.
Footnote |
† Electronic supplementary information (ESI) available. CCDC 1840125. For ESI and crystallographic data in CIF or other electronic format see DOI: 10.1039/d1ra07266h |
|
This journal is © The Royal Society of Chemistry 2021 |