DOI:
10.1039/D1RA07061D
(Paper)
RSC Adv., 2021,
11, 39666-39671
A novel biosensor based on Blu-ray disc coating film for determination of total amino acid content in tea leaves†
Received
21st September 2021
, Accepted 6th December 2021
First published on 13th December 2021
Abstract
Biosensor substrate materials are a key research focus in the field of sensors. Blu-ray discs (BDs) as universal sensor substrates are advantageous in comparison with other substrates (conventional paper and polycarbonate) in terms of easier activity treatment and higher density of reactive groups on the film surface. In this study, a novel and simple microfluidic biosensor based on BD coating film was developed by treating with sodium hydroxide solution and a piece of filter paper at slightly elevated temperatures. There are no significant physical damages to the substrate morphology, and the aging effect is minimal. The unique wetting, optical, and self-cleaning properties of the modified surfaces can be demonstrated in the paper. We have tested this new type of biosensor substrates for assay applications (the determination of total amino acids in tea leaves), which showed excellent performance in terms of sensitivity and reproducibility. The novel biosensor substrate material based on a simple BD coating film displayed preferable merits with easy making, low cost, easy using, and extensive application prospect.
1. Introduction
With the development of automated, inexpensive, miniaturized and portable testing devices with real-time response, high sensitivity and selectivity, biosensors are widely used in the fields of food safety, medical diagnostics, process control, environmental monitoring, and so on.1 In the past 50 years, research on biosensors has made considerable progress, which benefits mainly from the achievements of material science, microfluidics technology, nanoscience, and biotechnology.2,3 Biosensors have excellent predispositions and are a significant promise to become a powerful tool used in prevention and monitoring of diseases, rationalization of way of medical treatment and improving the patient quality of life.1,4 Attractively, biosensors can convert the concentration of biological analytes into an electrical signal or other signals for detection,5 which expands the scope of feasible application. Because biosensor substrate material is of a key research focus in the field of sensors, the novel substrates of stable biosensor interfaces in recent years have attracted much attention. The substrate material selection and interface activity are vital parts of biosensor development, since its stability can be directly related to the quality of sensing performance such as sensitivity, stability, and linearity.
Recently, microfluidic biosensors and biochips are emerging as a highly attractive platform for performing micro chemical and biological experiments.6 Microfluidic sensors based-chips have the advantages of low sample and reagent consumption, fast analysis speed, high degree of automation and broad application over conventional analytical systems.7,8 These biosensor-based microassays have been fabricated on a diverse range of substrates including glass,9 paper,10,11 plastics,12,13 and recent polymeric materials such as polymethyl methacrylate (PMMA),9,14 polydimethylsiloxane (PDMS)10,15 and polycarbonate (PC).16,17 However, it is travail and time-consuming to accomplish the surface activation of substrate materials between hydrophobicity and hydrophilicity which provides assays with reaction region.9 Many of these plastic substrates can be activated and patterned at the same time via direct and controlled UV exposure,12,18 which is unhealthy and environmentally unfriendly. Researchers are committed to obtaining a novel substrate material for biosensors, and a simple, well defined and reproducible surface modification method is essential for the development of microfluidic sensor in polymer-based substrates.
Blu-ray discs (BD) are advanced from other optical discs (CD, DVD) in terms of not only the storage capacity, but also the fabrication materials. We have recently discovered that the “Hard Coat™” film of Verbatim Blu-ray discs is a unique type of substrate materials (lactone composite) that can be activated and applied for multiplex biosensor applications. Herein we explore a kind of novel substrate material for microfluidics biosensors, and it is of great interest to us because of modifying hydrophobicity into hydrophilicity for material surface that can be applied to sensor manufacture. In detail we study how to convert Blu-ray discs with a special “hard-coat” film as universal substrates for microfluidic biosensors and carry out the characterization of surface activity. As detailed in the following sections, we will show how to activate and pattern the “Hard Coat™” film of Verbatim BDs (a unique type of substrate materials, lactone composite), and then take an example of determination of total amino acid content in tea leaves.
2. Experimental section
2.1 Materials and instruments
All reagents were of analytical grade. Ninhydrin and L-isoleucine were purchased from Nanjing Chemical Reagent Co., Ltd (Beijing, China). Blank BD-Rs (Verbatim 25 GB) were purchased from Millennium Compact Disc Industries Inc. (Vancouver, BC). Filter paper packs (Whatman, Grade 1) were purchased from local distributors of GE Healthcare Life Sciences. AST's VCA digital contact angle goniometer (AST Products, Inc., Billerica, MA) and atomic force microscopy (AFM, Dimension Edge, Bruker Corporation) were utilized to characterize the film surface. A digital camera (Huawei P20 with the resolution of 2244 × 1080 pixels, China) was used to capture the images of colorimetric assay performed on BD coating film-based microfluidic sensor.
2.2 Surface activation and characterization
We activated the surface of BD coating film with 1.0 M NaOH solution. The water contact angles of modified BD coating film were measured with AST's VCA digital contact angle goniometer. To confirm the generation of carboxylic acid groups (–COOH) on the BD coating film resulting from hydrolyzed treatment, the crystal violet, a cationic dye, was utilized. The vivid photographs of butterfly and panda obtained.
It is also important that the vital activity factors were optimized, such as the activity time of BD coating film, storage time of activated surface, the pH range of the wettability switch on the activated surface, and the surface roughness. The activity time of BD coating film were optimized by detecting the water contact angles in 15 min, 30 min, 45 min, 60 min, 75 min, 90 min, 105 min, and 120 min. The storage time of activated surface was studied by detecting the water contact angles after activity at 0 h (0 d), 24 h (1 d), 48 h (2 d), 96 h (4 d), 120 h (5 d), 144 h (6 d), 168 h (7 d), 192 h (8 d), 216 h (9 d), 240 h (10 d), 288 h (12 d), 336 h (14 d), and 432 h (18 d). The pH range of the wettability switch on the activated surface was researched by the contact angles of the hydrolyzed BD-surface treated with buffer solutions of different pH. The surface topographical changes of the original and hydrolyzed BD film and surface roughness were examined by AFM with a silicon tip (Dimension Edge, Bruker Corporation, resonance frequency 70 kHz, force constant 0.4 N m−1) in the ScanAsyst mode.
2.3 Creation of assay region in microfluidic biosensors
The BD coating film-based microfluidic sensor was fabricated with a piece of film from BD coating and a piece of NaOH-soaked filter paper mask. The BD coating film was prepared by removing the reflective layer via scoring and vigorous rinsing with deionized water and peeling off slightly, then rinsing the dye layer of BD-R film with a rapid methanol, and a final rinse with deionized water, last dried under N2.12,19 The filter paper mask was prepared by cutting six distribution channels consisting of six circle zones with a diameter of 6.0 mm on the ends of the channels and one circle zone with a diameter of 8.0 mm in the center. Notably, the film was patterned with microchannels by putting the filter paper mask soaked by 1.0 M NaOH solution onto the film, and incubated for 1 h at 55 °C in sealed chamber, which result in changing surface hydrophobicity into hydrophilicity.20 After removal of the NaOH paper masker, these hydrophilic microchannels were created and allowed the liquid to flow inside assay region. The creation demonstration of assay region in the microfluidic sensor is displayed in Fig. 1.
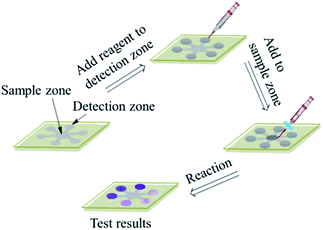 |
| Fig. 1 Creation demonstration of assay region in the microfluidic sensor. | |
2.4 Sample preparation
2.0% of ninhydrin solution was prepared by mixing 1.0 g of ninhydrin and 40.0 mg of SnCl2·2H2O in 25 mL of water. Then the solution was covered to protect from light and keep 12 h. The solution was filtrated by gravity and the filtrate was transferred to a volumetric flask and diluted to 50 mL with water. The phosphate buffer solution was prepared by mixed 11.2822 g of Na2HPO4·2H2O and 0.4539 g of KH2PO4 in 1.0 L of H2O and the pH was adjusted to 8.04. The L-isoleucine was used to prepare the standard solution of total amino acids because L-isoleucine is abundant in a tea leaf extract. A L-isoleucine stock standard solution of 0.2 mg mL−1 was prepared by dissolving 20 mg of L-isoleucine with water and diluted to 100 mL. The L-isoleucine working standard solutions were prepared by appropriate dilution of the stock solution with phosphate buffer solution.
1.0 g of tea leaves was accurately weighed into a beaker with 300 mL of boiling water, then boiled the water for 40 min to extract the amino acids from tea leaves. After the solution was cooled to room temperature naturally, the filtrate was transferred into a volumetric flask and diluted to 500 mL with the distilled water.
2.5 Procedures of determination of amino acids
In this experimental procedure, the circle zone in the center was used to spot ninhydrin solution, and the six circle zones were used as detection zones. To detect the amino acids in the samples, 15 μL of 2.0% ninhydrin was first spotted on the circle zone in the center of the BD film-based sensor, and then the ninhydrin solution automatically flowed into the detected zones by capillary force during 2 min. Due to the same diameter, length and width of the six distribution channels, the solution spotted onto the circle zone can flow equally along the channels and into the detected zones. That is to say, the six detected zones contain equal amounts of ninhydrin solution after the solution in the sensor was allowed to dry for 9 min. Then, 1.0 μL of five standard solutions with different concentrations of isoleucine and 1.0 μL of the prepared tea leave solution were spotted onto the detected zones. The sensor was heated in water bath at 80 °C for 15 min so that the isoleucine was reacted with the ninhydrin to form a purple-colored complex in detected zones. These images of the detected zones were taken and the gray values were calculated with the Adobe Photoshop CS4 software program for the quantitative analysis of the total amino acid content.
3. Results and discussion
3.1 Contact angles
The water contact angles of modified BD coating film were measured with AST's VCA digital contact angle goniometer by determining the contact line of the water droplet with the surface and measuring the inside angle of the drop with its tangent. Visual differences between the contact angles should be evident, but a setup using an optical lens and camera to magnify the image is also appropriate.21 The measured contact angles indicate that the hydrophilic groups produced after the surface activation with NaOH solution and the wettability of film surface changed from hydrophobicity to hydrophilicity. Whereas both slides become hydrophobic upon modification, a clear difference can be observed between the two slides between untreated and treated film, as shown in Fig. 2, and the water contact angle dropped from 141 ± 3° to 36 ± 2° after hydrolyzed treatment. For the wet hydrophilicity, the BD-R surface activated with alkali hydrolysis has the similar effect to PC activated with UV-ozone and PMMA activated with UV.16 A simple, well defined and reproducible surface modification method is come true in this study for the development of microfluidic sensor in polymer-based substrates.
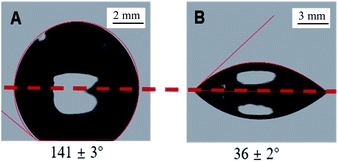 |
| Fig. 2 Side-view images of water droplets on BD coating film: (A) untreated film, and (B) film treated with 1.0 M NaOH aqueous solution for 1 h at 55 °C. The dashed horizontal lines indicate the contact lines between the water droplet and the BD film surface; the portion above the line is the water droplet and the portion below the line is a reflection of the droplet. The contact angles, measured by an AST VCA system, are (A) 141 ± 3° and (B) 36 ± 2°. | |
3.2 Pattern of surface activation
We activated the surface of BD coating film with 1.0 M NaOH solution. To confirm the generation of carboxylic acid groups (–COOH) on the BD coating film resulting from hydrolyzed treatment, the crystal violet, a cationic dye, was utilized. This method relies on the electrostatic interactions between crystal violet molecules and carboxylate groups. The ESI† displayed how to determine the surface density of –COOH on activated BD film. As shown in Fig. 3, the vivid photographs of butterfly and panda obtained by dropping aqueous violet solution onto the film surface activated, which indicate the wet hydrophilicity and –COOH on the film surface were generated. The major advantage of this procedure is its safety, convenience, easy operation and without equipment over conventional UV-ozone irradiation for polymer activation.
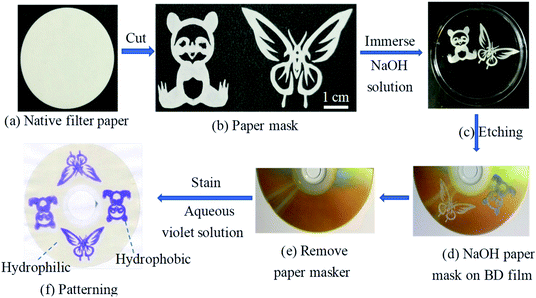 |
| Fig. 3 Schematic diagram of fabricating patterning by wet etching. (a) Native filter paper, (b) paper mask produced by cutting on the filter paper, (c) paper mask penetrated with NaOH solution, (d) NaOH paper mask on BD surface, (f) remove paper masker, and (g) patterning with hydrophilic–hydrophobic contrast. | |
3.3 Optimization of surface activation
A simple, well defined and reproducible surface modification method is essential for the development of biosensors in polymer-based substrates. The hard coating material on BDs is typically an UV-curable resin with dispersed silica nanoparticles,22 which is different from the CD/DVD surface (polycarbonate substrate). Therefore, we need to explore a new surface activation method.
We have used a common base, NaOH (1.0 M), to activate the BD film at 55 °C. As shown in Fig. 4, the water contact angle dropped from 92 ± 2° to 33 ± 2° after less than 45 min of hydrolyzed treatment and then remained constant. For the wet hydrophilicity, the activation of BD surface with alkaline hydrolysis had the similar effect to PC activation with UV/ozone, whose water contact angle dropped from 88 ± 2° to 20 ± 2°.16 Compared with the conventional UV/ozone PC activation, this hydrolysis activation had advantages of safety, convenience, easy operation and without equipment.
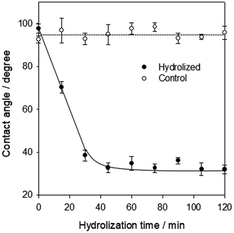 |
| Fig. 4 Dependence of water contact angle at the BD-R film surface on activation time during the treatment with 1.0 M NaOH at 55 °C (solid circles) and an untreated BD surface (open circles). The experimental uncertainties were determined from at least three sets of independent measurements. | |
A major problem for potential applications upon surface activation is the so-called aging effect, i.e., the increase of hydrophobicity during sample storage. It is believed that reorganization of the polymer chains on the surface induces the hydrophilic groups to move into the bulk. As shown in Fig. 5, the water contact angle increased ∼10° initially during 120 hours (5 days) and then remained stable, indicating the surface remained reactivity after several days, while UV/ozone treated PC samples increased 20° during 48 h (2 days).16 Therefore, the aging effect of BD surfaces treated with NaOH solution displayed a preferable stability.
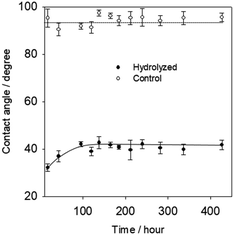 |
| Fig. 5 BD-R surface on storage time (aging) after initial activation by dependence of water contact angle at the BD-R film surface on storage time (aging) after initial activation by NaOH solution (1.0 M) for 45 min at 55 °C. The dashed and solid lines are to direct the eyes only. The experimental uncertainties were determined from three sets of independent measurement. | |
To confirm the formation of reactive COOH groups besides other polar functionalities (e.g., alcoholic OH), which also reduce the surface hydrophobicity, contact angle titrations were carried out. As Fig. 6 shown, the contact angles of the hydrolyzed BD-surface treated with buffer solutions of different pH went through a smooth transition upon raising the pH from 3 to 10 shown, due to the ionization of carboxylic acid groups. The formation of carboxylate anions (COO–) made the surface more hydrophilic. In contrast, the constant angle remains at 98 ± 2° on the untreated surfaces throughout. The pH range of the wettability switch is broad, from 3 to 10, which may be due to the generation of different types of carboxylic acids upon surface activation. It is remarkable that the difference between the two “plateaus” is as high as 25°, indicating a high surface density of reactive –COOH groups. The surface density of reactive carboxylic acid groups generated, 6.6 ± 0.7 × 10−9 mol cm−2, is much higher than that on polycarbonate upon UV/ozone irradiation (4.8 ± 0.2 × 10−10 mol cm−2).16
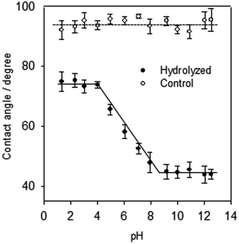 |
| Fig. 6 Contact angles of buffer solutions on a BD-R film surface treated with 1.0 M NaOH for 45 min at 55 °C (solid circles) and an untreated BD surface (open circles). Each data point represents the average reading of at least three replicated data, and the error bars show the corresponding standard deviation. | |
To ensure the topography of the BD-R film substrates is not significantly altered upon hydrolyzation treatment, the surfaces roughness was examined by tapping-mode atomic force microscopy (AFM). Fig. 7 shows the AFM images of (a) an untreated and (b) a treated BD-R film surface. The surface roughness did not increase significantly as indicated by the z scales (2.2 nm and 5.1 nm) and similar rms roughness factors (0.9–3 nm). Although, the hydrolyzation-treated samples appear a little rougher and more porous; the rms roughness factor just increased from 0.89 to 3.05 nm. This indicates that the basic hydrolyzation and subsequent washing steps don't almost alter the surface morphology and it is just non-destructive except slightly rougher, which would be beneficial for the preparation of assays on BD film substrates. The fact that the surface morphology of BD film was not physically damaged guarantees the feasible application of hydrolyzation activation protocol and potential application of multiplex assay as universal biosensor substrates.
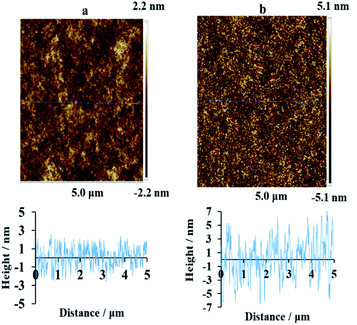 |
| Fig. 7 AFM images and corresponding cross sections of the BD-R surfaces (a) before and (b) after hydrolyzation with NaOH solution (1.0 M) for 45 min at 55 °C. | |
3.4 Total amino acid concentration in sample
The total amino acids in the tea leaf extract sample were determined via the procedure described above, and the images of colorimetric assays were taken by a camera and stored in JPEG format, as Fig. 8(A) shown. These JPEG images were opened with the Adobe Photoshop CS4 software program under the RGB colour format. Notice that the image was inverted and then the mean gray value in detection zones was obtained with subtracting the blank value. Lastly, the linear correlation between mean gray value that is gray intensity and the concentration of total amino acid was obtained by these data imported into the SigmaPlot 10.0. The linear eqn (1) between the GI and C was obtained with a correlation coefficient of 0.993, as Fig. 8(B) shown.where the GI means grayscale intensity, and the C means the concentration of total amino acid in the sample with the unit of μg mL−1.
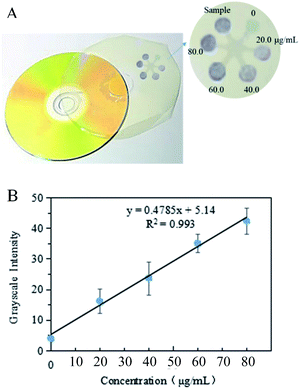 |
| Fig. 8 (A) Images showing the results of amino acid assay on the simple microfluidic sensor based on BD coating film with varied concentrations. (B) Mean grayscale intensity value varies as a function of isoleucine concentration obtained from data of (A). The grayscale intensity values were provided by the Adobe Photoshop CS4 software after subtraction of the blank value. | |
According to the gray value in the sample detection zone and the linear eqn (1), the concentration of the total amino acids in the tea leaf extract was calculated as 69.3 μg mL−1. The relative standard deviation (RSD) was 8.3% by determining 60.0 μg mL−1 isoleucine solution five times. Thus, the content (μg g−1) of the total amino acid in tea leaves was calculated according to the following eqn (2)
|
 | (2) |
where
w (μg g
−1) means the concentration of the total amino acids in tea leaves,
C (μg mL
−1) is the concentration of the total amino acid in tea leaf extract,
V (mL) is the volume of tea leaf extract, and
m (g) is the weighed mass of tea leaves. The content of the total amino acids in tea leaves,
w (μg g
−1), was calculated as 3.46 × 10
4 μg g
−1 according to the
eqn (2). This result compared well with that measured by a Chinese national standard method,
23 3.24 × 10
4 μg g
−1, which demonstrated that the analytical method based BD film in this study could be utilized to determine the total amino acids in tea leaves, and displayed easy, fast, economic and non-equipment merits.
4. Conclusions
In conclusion, BD substrates can be readily converted to a novel biosensor platform for the fabrication of chip-based biosensing devices (the determination of total amino acids in tea leaves as an example) by brief hydrolysis with NaOH solution. The new substrate material with the activity method is without equipment (just keeping on 55 °C), simple (immersing in alkaline solution), efficient (yielding a high surface density of –COOH), and nondestructive (surface morphology is not altered). The fabrication procedure (activation, patterning, and applying) is easy and effective, and the immobilization assay is highly sensitive. The surface chemistry of novel biosensor material based on BD substrate described herein may find preferable applications well outside the determination of total amino acids in tea leaves: it is not overemphasized that the value of this method extends far beyond the simple examples presented in this paper; i.e., it is potentially useful for the development of disposable biochips and the fabrication of biomedical devices that are readable with conventional disc drives and smartphones.
Conflicts of interest
There are no conflicts to declare.
Acknowledgements
The authors gratefully acknowledge the financial from the National Natural Science Foundation of China (82003507), the Open Fund of State Key Laboratory of Tea Plant Biology and Utilization (SKLTOF20190123), University Science Research Project of Jiangsu Province (19KJB330001), Postdoctoral Science Foundation of China (2019M661699), Jiangsu province Planned Projects for Postdoctoral Research Funds of China (2019K297). We would like to thank Lingling Zhang, Clayton, Yihan Zhang, and Yuanyuan Tao for their help and participation in the experiments.
References
- A. Kowalczy, Curr. Opin. Electrochem., 2020, 23, 36–41 CrossRef.
- M. Song, X. G. Lin and Z. J. Peng, et al., Front. Mater., 2020, 7, 583739 CrossRef.
- B. Schuster, Biosensors, 2018, 8, 40 CrossRef PubMed.
- S. Vigneshvar and B. Senthilkumaran, Med. Devices Sens., 2018, 1, e10011 CrossRef.
- D. R. Thevenot, K. Toth and R. A. Durst, et al., Biosens. Bioelectron., 2001, 16, 121–131 CrossRef CAS PubMed.
- E. Verpoorte, Electrophoresis, 2015, 23, 677–712 CrossRef.
- Y. Sun, T. L. Quyen and T. Q. Hung, et al., Lab Chip, 2015, 15, 1898–1904 RSC.
- J. Kim, H. Cho and J. Kim, et al., Biosens. Bioelectron., 2021, 176, 112897 CrossRef CAS PubMed.
- L. Wang, C. H. Li and H. Z. Yu, et al., Anal. Chim. Acta, 2008, 610, 97–104 CrossRef CAS PubMed.
- L. Cai, W. Yong and Y. Wu, et al., Analyst, 2014, 139, 4593–4598 RSC.
- X. Li, C. Zhao and X. Y. Liu, Microsyst. Nanoeng., 2015, 1, 15014 CrossRef.
- P. Zuo, X. J. Li and D. C. Dominguez, Lab Chip, 2013, 13, 3921–3928 RSC.
- D. M. Ivnitskii, I. N. Kurochkin and S. D. Varfolomeev, et al., J. Anal. Chem., 1991, 46, 725–728 Search PubMed.
- C. Situma, Y. Wang and M. Huperta, et al., Anal. Biochem., 2005, 340, 123–135 CrossRef CAS PubMed.
- H. H. Chen, C. H. Wu and M. L. Tsai, et al., Anal. Chem., 2012, 84, 8635–8641 CrossRef CAS PubMed.
- Y. C. Li, Z. Wang and M. L. Ou, et al., Anal. Chem., 2007, 79, 426–433 CrossRef CAS PubMed.
- C. I. Kammann, H. P. Schmidt and N. Messerschmidt, et al., Sci. Rep., 2015, 5, 11080 CrossRef PubMed.
- A. Krasiński, Z. Radić and R. Manetsch, et al., J. Am. Chem. Soc., 2005, 127, 6686–6692 CrossRef PubMed.
- S. Weng, X. C. Li and M. Niu, et al., Anal. Chem., 2016, 88, 6889–6896 CrossRef CAS PubMed.
- L. L. Chu, L. L. Zhang and Z. Z. Gu, et al., ACS Appl. Mater. Interfaces, 2019, 11, 37330–37337 CrossRef CAS PubMed.
- L. Guillaume, H. Ahmed and B. Andrii, et al., J. Chem. Educ., 2010, 87, 1403–1407 CrossRef.
- V. Gubala, L. F. Harris and A. J. Ricco, et al., Anal. Chem., 2012, 84, 487–515 CrossRef CAS PubMed.
- J. F. Xu, W. L. Zhou and X. L. Lu, et al., Chinese National Standards, 2013, GB/T 8314-2013 Search PubMed.
Footnote |
† Electronic supplementary information (ESI) available. See DOI: 10.1039/d1ra07061d |
|
This journal is © The Royal Society of Chemistry 2021 |
Click here to see how this site uses Cookies. View our privacy policy here.