DOI:
10.1039/D1RA06745A
(Paper)
RSC Adv., 2021,
11, 36689-36697
Investigation on the upconversion luminescence and ratiometric thermal sensing of SrWO4:Yb3+/RE3+ (RE = Ho/Er) phosphors
Received
8th September 2021
, Accepted 8th November 2021
First published on 15th November 2021
Abstract
SrWO4 phosphors doped with Ho3+(Er3+)/Yb3+ are successfully prepared by a high temperature solid-state reaction method. The upconversion (UC) luminescence properties of all the samples have been investigated under 980 nm excitation. Strong green emissions are obtained in the SrWO4:Yb3+/Ho3+ and SrWO4:Yb3+/Er3+ samples with the naked eyes. In a temperature range going from 303 K to 573 K, the UC emission spectra of the phosphors have been measured. Then the temperature sensing properties also have been discussed via fluorescence intensity ratio (FIR) technology. For the SrWO4:Yb3+/Ho3+ phosphor, the FIR technologies based on thermal coupling levels (TCLs)(5F4,5F5) and non-thermal coupling levels (non-TCLs)(5S2, 5F4/5F5) are used for investigating the sensitivity. The results show that the maximum absolute sensitivity reaches 0.0158 K−1 with non-TCLs. As for Yb3+/Er3+ codoped SrWO4 phosphor, the maximum absolute sensitivity reaches 0.013 K−1 with TCLs (2H11/2,4S5/2) at a temperature of 513 K. These significant results demonstrate that the SrWO4:Ho3+(Er3+)/Yb3+ phosphors are robust for optical temperature sensors.
1. Introduction
As the basic parameter of thermodynamics, temperature measurement plays a vital role in scientific research, industrial production and medicine.1 In recent years, much attention has been paid to non-contact temperature sensors based on rare earth (RE) ion activated luminescent materials.2 Some UC luminescent materials with doping of RE3+ ions have been studied for temperature sensors.3–7 The FIR technology based on the measurement of the temperature-dependent FIR from two excited state energy levels of activators represents an accurate temperature measuring method, because the FIR from the thermally coupled levels (TCLs) of RE ions is independent of the excitation intensity fluctuations, external disturbance and spectrum losses. Thus, reasonable measurement accuracy and sensitivity could be obtained with this method. The variation of FIR is generally caused by a thermally induced population re-distribution among the TCLs. And some RE ions have already been explored for designing FIR thermometry, such as Er3+, Tm3+, Ho3+ et al.8–13 The sensitivity is an important role of a temperature sensing device. The absolute sensitivity is defined as the rate of change of FIR with change in temperature relative to FIR. Basically, a larger energy gap between the TCLs benefits the enhancement of sensitivity. However, the largest energy mismatches between those TCLs cannot exceed 2000 cm−1, so it is difficult for the further improvement of sensitivity. There is an urgent need to design a new temperature measurement method to obtain high detecting sensitivity. Recently, a new kind of strategy has been proposed to solve the above drawback, which is based on FIR between the non-TCLs of the activators. The FIR derived from non-TCLs is also related to temperature. Compared with the FIR technique based on the TCLs, the FIR technique based on the non-TCLs is no longer limited by the energy gap. The Er3+ and Ho3+ ions also have non-TCLs pairs in addition to TCLs. So further investigation on temperature sensing properties of Er3+ and Ho3+ ions based on the FIR technology (TCLs and non-TCLs) is necessary.
Besides activators, temperature sensing properties of UC luminescent materials are also decided by host material. Among various hosts, tungstates have obtained much attention because of their brilliant physical and chemical stability, low phonon threshold energy, and high density.14 The Ln3+ ions also have high doped concentration in tungstates host. So the activators can emit intense and stable fluoresce even at high temperature.15 SrWO4 belongs to a body-centered tetragonal system with scheelite crystal structure where WO42− molecular ions are loosely bound to Sr2+ cations. It has been reported that SrWO4 is an ideal host for optical temperature sensing materials. Recently, the temperature sensing properties of Ln3+ doped tungstates also have been studied. Pandey et al. report that the Er3+–Yb3+ co-doped SrWO4 phosphor is a good optical temperature sensing material, and its maximum absolute sensitivity reaches 0.01498 K−1.16 The high sensitivity of Tm3+/Yb3+ co-doped SrWO4 phosphor for optical thermometry is synthesized by Song et al., and when the temperature is 323 K, the absolute sensitivity reaches a maximum of 0.00617 K−1.17 For Sm3+ doped SrWO4 phosphor and Nd3+/Yb3+ co-doped SrWO4 phosphor, they also have good temperature sensing performance.18,19 Note that, the SrWO4 material is more suitable to be a UC fluorescence host for designing optical temperature sensor. However, as far as we know, the SrWO4:Yb3+/Ho3+ phosphor for optical thermometry has not been investigated. There are even fewer reports on its optical thermometry using FIR technique based on the non-TCLs.
In this paper, the SrWO4:Yb3+/RE3+(RE = Er, Ho) phosphors are synthesized by a high temperature solid state reaction. The UC luminescence performances and temperature sensing properties are investigated under 980 nm excitation. The experimental results illustrate that the temperature sensitivity of our phosphors are better than the most reported Ho3+(Er3+) doped materials. The SrWO4 doping Ho3+(Er3+) ions materials are optical temperature sensing material with good application value.
2. Experimental
2.1 Synthesis of the phosphor
The samples are designed according to the molar composition of Sr1–x−0.01YbxHo0.01WO4 (x = 0, 1%, 2%, 3%, 4%, 6%, 10%) and Sr1−y−0.01YbyEr0.01WO4 (y = 0, 1%, 2%, 3%, 4%), and synthesized by solid-state reaction method. High-purity SrCO3 (99%), WO3 (99.8%), Yb2O3 (99.99%), Ho2O3 (99.99%) and Er2O3 (99.99%) are purchased from Aladdin Chemical Reagent Co. Ltd (China). The raw materials are weighed by molar ratio and mixed together. The mixtures thoroughly are ground in a mortar of agate for 60 minutes. The ground mixture is transferred to a crucible ceramics and then heated it in a muffle furnace. First, the temperature rises to 900 °C by 10 °C min−1. The samples are kept at 900 °C for 4 h. Next, temperature is increased by 5 °C min−1 to 1100 °C and kept for 6 h. Finally the SrWO4:Ho3+/Yb3+ and SrWO4:Er3+/Yb3+ phosphors are obtained.
2.2 Measurement and characterization
The structural formation of the phosphors has been measured using an X-ray diffraction (Rigaku D/Max-2500) in the range of 15° to 80° (2θ), and its radiation source is Cu Kα ray of λ = 0.15406 nm. For morphology and size of the phosphors, the field emission scanning electron microscope (FE-SEM, JEOL JEM-6700F) is used to perform. The UC emission spectra are recorded using the Zolix Omni-λ500 spectrometer under a 980 nm laser (MDL-III-980-2W, China) excitation. The samples are heated using an Orient KOJI TAP-02 high temperature thermometer, among a temperature range of 303 to 573 K, with a temperature control accuracy of 0.1 °C.
3. Result and discussion
3.1 Structural characterization
Fig. 1(a) shows the XRD patterns of the SrWO4:Ho3+/Yb3+ phosphors. It can be seen that the diffraction peaks of the samples are very consistent with the standard card of SrWO4 (JCPDS no. 08-0490). The sharp and strong diffraction peaks show that the synthesized samples are crystallized well. It also means that Sr2+ in the SrWO4 lattice is substituted by Ho3+ and Yb3+ ions. The XRD patterns of the SrWO4:Er3+/Yb3+ samples are shown in Fig. 1(b). The main diffraction peaks of samples are also consistent with the standard card of SrWO4 (JCPDS no. 08-0490). But an extra weak peak at 29.3° appears in SrWO4:1%Er3+, x%Yb3+(x = 2, 3, 4) samples, which may be induced by the minor unreacted component of Yb2O3. Once the concentration of the Yb3+ ions is over 2%, a part of the Yb3+ ions will not be added in the lattice. In addition, the peak at 30°moves to a high angle direction with the concentration of Yb3+ ions increasing (see the illustration in Fig. 1(b)), which is attributed to the shrinkage of the crystal lattice caused by the doped Er3+ and Yb3+ ions substituting the Sr2+ sites. The ionic radius of Er3+, Yb3+ and Sr2+(Er3+: 0.89 Å, Yb3+: 0.86 Å and Sr2+: 1.12 Å)are different, so the crystal lattice will produce deformation. The SEM images of SrWO4:Ho3+(Er3+)/Yb3+ phosphor are shown in Fig. 2. It indicates that the size of the prepared phosphor is almost uniform.
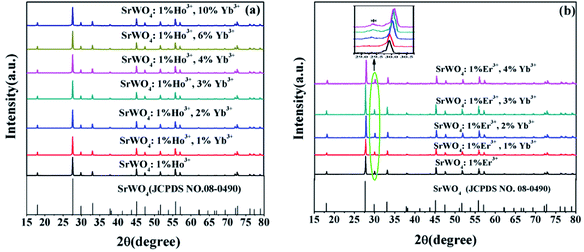 |
| Fig. 1 XRD patterns of SrWO4: (a) 1%Ho3+/x%Yb3+(x = 0, 1, 2, 3, 4, 6, 10) phosphors. (b) 1%Er3+/x%Yb3+(x = 0, 1, 2, 3, 4) phosphors. | |
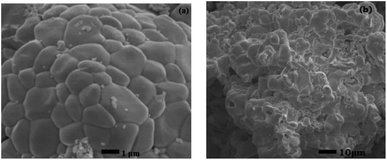 |
| Fig. 2 SEM images of SrWO4: (a) 1%Ho3+/2%Yb3+ phosphor. (b) 1%Er3+/1%Yb3+ phosphor. | |
3.2 Upconversion emission properties
Fig. 3(a) shows the UC emission spectrum of SrWO4:1%Ho3+/2%Yb3+ phosphor from 500 to 800 nm under 980 nm excitation. Three characteristic emission bands are presented that the strong green emission (∼540 nm) belongs to the radiative transition from the 5S2/5F4 to 5I8, the red emission (∼663 nm) belongs to the radiative transition from 5F5 to 5I8 and the weak near infrared emission (∼756 nm) corresponds to 5F4 → 5I7 energy level transition of Ho3+ ions. Fig. 3(b) shows the UC emission spectra of SrWO4:1%Ho3+/x%Yb3+ (x = 0, 1, 2, 3, 4, 6, 10). It is seen that, the intensities of all the UC emission peaks reach maximum values at x = 2. But they decrease if the Yb3+ concentration is further increased up to 2%. The Yb3+ ion is a high-efficiency sensitizer for Ho3+ ion because it has lager absorption cross-sectional area at the near infrared. With increasing the Yb3+ ions concentration to 2%, the energy transfer process from Yb3+ to Ho3+ can be enhanced, resulting in higher UC emission intensity. However, with the further enhancement of the Yb3+ concentrations, the distance between the doping ions decreases with the increase of Yb3+ concentration. It induces more intense interaction among adjacent Yb3+ ions, which cause concentration quenching.20
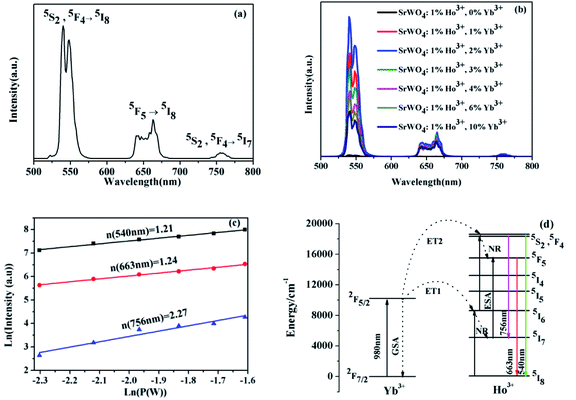 |
| Fig. 3 (a). The emission spectrum of SrWO4:1%Ho3+/2%Yb3+ phosphor from 500 to 800 nm under exciting at 980 nm. (b). The UC emission spectra of SrWO4:1%Ho3+/x%Yb3+ (x = 0, 1, 2, 3, 4, 6, 10). (c). Log–log diagram of excitation power density and UC emission intensity. (d). The energy level diagrams of Ho3+/Yb3+ ions and possible UC emission processes. | |
In order to explicate the possible UC emission process of the SrWO4:Ho3+/Yb3+ sample, the dependence relation of the UC emission intensity via pump power is measured. The relationship between UC emission intensity and pumping power can be described by the following eqn (1):21
where
I is the UC emission intensity,
P is the pumping power and
n is the photon numbers which correspond to populate the upper emitting levels.
Fig. 3(c) shows the log–log plot of intensity
versus pump power and the slopes of the fitted lines of SrWO
4:1%Ho
3+/2%Yb
3+ sample. It can be seen that
n values are 1.21, 1.24 and 2.27 for green (∼540 nm), red (∼663 nm), and near-infrared (∼756 nm) emissions. It indicates that these UC emission processes are related to the two-photon processes. The energy level diagrams of Ho
3+/Yb
3+ ions and possible UC emission processes are shown in
Fig. 3(d).
Under 980 nm excitation, the Yb3+ ion are excited from the ground state 2F7/2 to the excited state 2F5/2 by ground state absorption (GSA) process. Ho3+ ions are excited to the excited state levels mainly through the energy transfer (ET) process from Yb3+ to Ho3+. Through ET1 (2F5/2 (Yb3+) + 5I8 (Ho3+) → 2F7/2 (Yb3+) + 5I6 (Ho3+)), the Ho3+ ions are excited from the 5I8 to the 5I6 state.22 Some Ho3+ ions at 5I6 state can nonradiatively (NR) relax to 5I7 state, and then populating the 5F5 state by excited state absorption (ESA) from 5I7 to 5F5. Ho3+ ions at 5I6 state can continue to be excited into the 5S2, 5F4 coupling state by ET2 (2F5/2 (Yb3+) + 5I6 (Ho3+) → 2F7/2 (Yb3+) + 5S2, 5F4 (Ho3+)). Subsequently, the Ho3+ ions at the 5S2, 5F4 coupling state will relax to the 5F5 state by NR relaxation, and then a part of the Ho3+ ions at the 5F5 state are transferred to the 5I8 state, concurrently red light of 663 nm is emitted. The 5S2, 5F4 coupling state relax to the 5I8 and 5I7 states, resulting in high intensity green light (∼540 nm) and low intensity red light (∼756 nm), respectively.
The UC emission spectrum of SrWO4:1%Er3+/1%Yb3+ phosphor is shown in Fig. 4(a). Two UC emission bands are exhibited, the strong green and the weak red emission, which can be attributed to the radiative transitions from 2H11/2/4S3/2 to 4I15/2 and the 4I9/2 → 4I15/2 of the Er3+ ions, respectively. Fig. 4(b) shows the UC emission spectra of SrWO4:1%Er3+/x%Yb3+ (x = 0, 1, 2, 3, 4). It can be found that the intensities of two emission bands first increase and then decrease with increasing Yb3+ concentrations, and reach a maximum at x = 1. Once the concentration of Yb3+ is over 1%, the UC emission intensities rapidly decline. This is mainly attributed to that the energy transfer efficiency from Yb3+ to Er3+ ion decrease because the limit of the Yb3+ that can be stabilized into the matrix. As demonstrated by the extra peaks in the XRD patterns, when the concentration of the Yb3+ ions is over 2%, a part of the Yb3+ ions will not be added in the lattice.
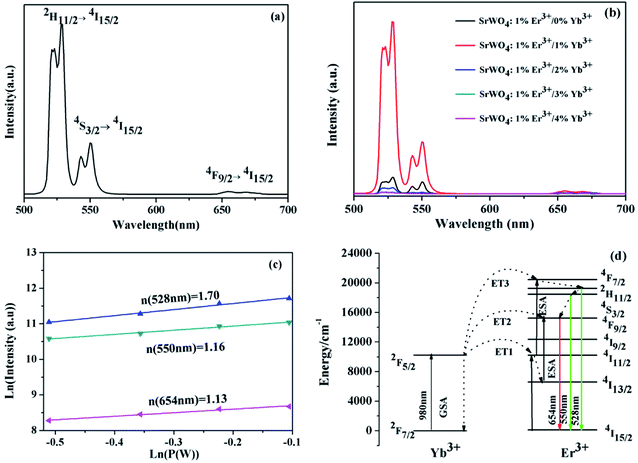 |
| Fig. 4 (a). The UC emission spectrum of SrWO4:1%Er3+/1%Yb3+ phosphor. (b). UC emission spectra of SrWO4:1%Er3+/x%Yb3+ (x = 0, 1, 2, 3, 4). (c). Log–log relationship between pump power and UC emission intensity of SrWO4:1%Er3+/1%Yb3+ phosphor. (d). The energy level diagrams of Er3+/Yb3+ ions and possible UC emission processes. | |
The dependence relationship of the UC emission intensities of SrWO4:Er3+/Yb3+ on pump power are shown in Fig. 4(c). For the emission ∼528 nm, 550 nm and 654 nm, the slopes of the fitting experiment data are 1.70, 1.16 and 1.13, which is near 2. It indicates that all emission processes are related to the two-photon processes. Fig. 4(d) shows the energy level diagrams and possible UC emission processes of Er3+ and Yb3+ ion. Under 980 nm laser excitation, Yb3+ ions are excited from the ground state 2F7/2 to the excited state 2F5/2 by GSA process. The Er3+ ions are excited to the 4I11/2 state from the ground state through the ET1 (2F5/2 (Yb3+) + 4I15/2 (Er3+) → 2F7/2 (Yb3+) + 4I11/2 (Er3+)). After that, the Er3+ ions at 4F7/2 state can be populated from the 4I11/2 state by the ET3 (2F5/2 (Yb3+) + 4I11/2 (Er3+) → 2F7/2 (Yb3+) + 4F7/2 (Er3+)). The 2H11/2 and 4S3/2 states are populated from the 4F7/2 state owing to the NR relaxation, and then the Er3+ ions at 2H11/2 and 4S3/2 states relax to the 4I15/2 state, leading to the green UC emissions (528 nm and 550 nm). The Er3+ ions at 4I11/2 state can relax to the 4I13/2 state through the multiphonon relaxation (MPR) process, and then the Er3+ ions at 4I13/2 state are excited to the 4F9/2 by ET2 (2F5/2 (Yb3+) + 4I13/2 (Er3+) → 2F7/2 (Yb3+) + 4F9/2 (Er3+)). Meanwhile, the 4F9/2 state can be also populated from the 4S3/2 state through the NR relaxation. The Er3+ at the 4F9/2 state will transit to the 4I15/2 state and thus emitted the red light (∼654 nm).
3.3 Optical temperature-sensing properties
For investigating the optical temperature sensing characteristics of SrWO4:Ho3+/Yb3+ phosphor, the emission spectra of the SrWO4:Ho3+/Yb3+ sample are measured at different temperature. As shown in Fig. 5(a), it can be seen that the UC emission intensities of all emission band decrease with the temperature increasing. The possible reason is thermal quenching effect.23 But the decreasing rate of the different emission band is different. Fig. 5(b) shows the UC emission intensity variation of 540 nm (5S2, 5F4 → 5I8) and 756 nm (5S2, 5F4 → 5I7) with temperature increasing. It is clearly observed that the UC emission intensity at 756 nm drops much more slowly than that at 540 nm with the temperature increasing. By considering the thermalization between 5S2 and 5F4 levels, the FIR of the UC emission from (5F4, 5S2) → 5I8 and (5F4, 5S2) → 5I7 transitions can be analyzed by using a four-level system, which was introduced by González-Pérez et al.24 as shown in Fig. 5(c). The 5S2, 5F4, 5I7 and 5I8 levels form a four-level system. The emission intensity is proportional to the population of each energy level and the population of the excited state relates to the temperature. So the FIR can be expressed by the following eqn (2).25 |
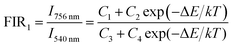 | (2) |
where C1, C2, C3 and C4 are constants about spontaneous emission rate, energy level degeneracy and emission energy. ΔE is the energy gap of the 5S2 and 5F4, k is the Boltzmann constant, and T is the absolute temperature. It can be seen in Fig. 5(d), the FIR of the I756 nm/I540 nm regularly changes with the temperature increasing. It shows a nonlinear variation of FIR value at the temperature range 303 to 573 K. The eqn (2) is used to well fit the data, the C1, C2, C3, C4 and ΔE are 0.15, −0.3, 2.98, −6.1 and 429.15 cm−1, respectively.
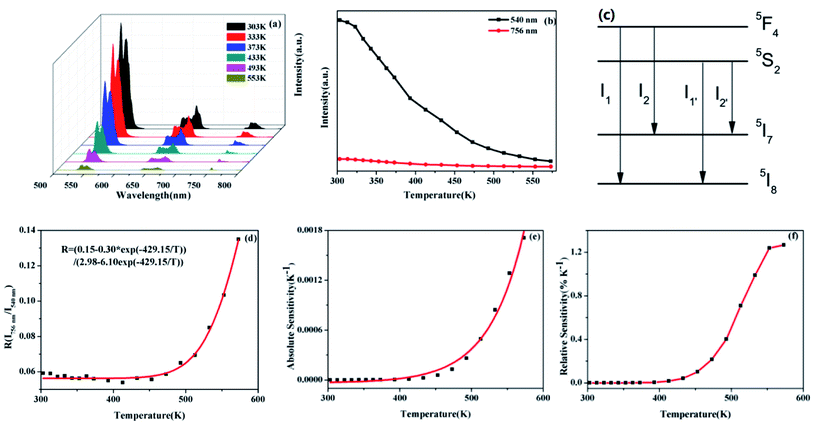 |
| Fig. 5 (a). Temperature-dependent on UC emission spectra of SrWO4:1%Ho3+/2%Yb3+ phosphor at 303–573 K. (b). The emission intensity of SrWO4:1%Ho3+/2%Yb3+ phosphor center at 540 nm and 756 nm change in the range 303–573 K. (c) Four-level simplified diagram of transitions from adjacent excited levels to two lower levels. (d) The FIR (I756 nm/I540 nm), (e) the absolute sensitivity based on the I756 nm/I540 nm (f) the relative sensitivity based on the I756 nm/I540 nm. | |
In the experiment, absolute sensitivity (Sa) is a non-negligible evaluation index of temperature, which indicates the absolute change of FIR in unit temperature, as shown in eqn (3).
|
 | (3) |
Relative sensitivity (Sr) is also an important parameter that can be described as:
|
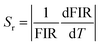 | (4) |
Sa and
Sr of the sample are shown in
Fig. 5(e) and (f), which are fitted by
eqn (3) and
(4). The
Sa increases with the temperature enhancement, reaching a maximum of 0.0017 K
−1 at 573 K. While the maximum value of
Sr is 1.27% K
−1 at 573 K.
Fig. 6(a) shows that the emission intensities of the green and red band vary with temperature increasing. It can see that the intensity of red emission decreases more slowly than that of green emission. The energy levels 5S2,5F4 and 5F5 energy levels are far apart, and the particle population at the two energy levels do not follow the Boltzmann-type distribution. The 5S2, 5F4 and 5F5 belong to non-TCLs. So the traditional FIR analysis method based on TCLs is not appropriate for the non-TCLs system. In the previous report, the relation of the non-TCLs FIR and temperature can be fitted by eqn (5).26
|
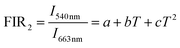 | (5) |
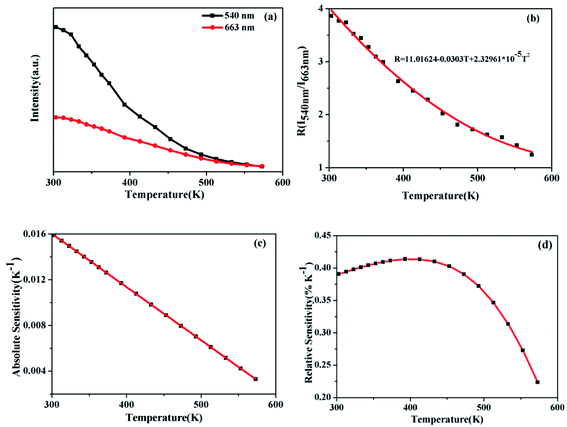 |
| Fig. 6 (a) The emission intensity of SrWO4:1%Ho3+/2%Yb3+ phosphor center at red (663 nm) and green (540 nm) changes with temperature. (b) The FIR (I540 nm/I663 nm), (c) the absolute sensitivity and (d) the relative sensitivity of SrWO4:Ho3+/Yb3+ phosphor as a function of temperature. | |
Fig. 6(b) shows that the original data for the I540 nm/I663 nm as a function of the temperature fitting with the eqn (5). Consequently the FIR based on 5S2, 5F4/5F5 (Ho3+) shows the significant temperature dependence and well fits the eqn (5). The curve of Sa and Sr by calculation and fitting are shown in Fig. 6(c) and (d). The Sa goes down with the increasing of temperature. The maximum Sa is 0.0158 K−1 at 303 K. While the Sr reaches a maximum of 0.41% K−1 at 393 K. The above analysis of the sensitivity of SrWO4:Ho3+/Yb3+ based on the TCLs and the non-TCLs shows that the sample has high sensitivity and it has certain application value in optical temperature measurement.
Besides, the Er3+–Yb3+ co-doped SrWO4 phosphor has also been studied for its sensing properties. As shown in Fig. 7(a), the UC emission spectra of green light in the different temperature are recorded. The UC luminescence intensities of 528 nm and 550 nm (generated by 2H11/2 → 4I15/2 and 4S3/2 → 4I15/2) vary differently with the change of temperature. At 313 K, the intensity of the two emission bands is almost equal, with the temperature increasing, the luminescence intensity of the 528 nm emission band is gradually increased within 313–433 K, and that of the 550 nm emission band gradually decreases. Then, in the range of 433–573 K, as the temperature increasing, the intensities of two bands also decrease because of the non-radiative relaxation enhancement. But the luminescence intensity of the 550 nm emission band decreases faster than that of 528 nm emission band. When the temperature increases, the electrons at 4S3/2 state are excited. The transition from 4S3/2 to 2H11/2 occurs. It indicates that the 2H11/2 and 4S3/2 states of Er3+ can be regarded as TCLs, and the electrons population at 2H11/2 and the 4S3/2 states follows the Boltzmann distribution. The FIR of TCLs can be expressed by the eqn (6):27
|
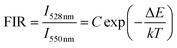 | (6) |
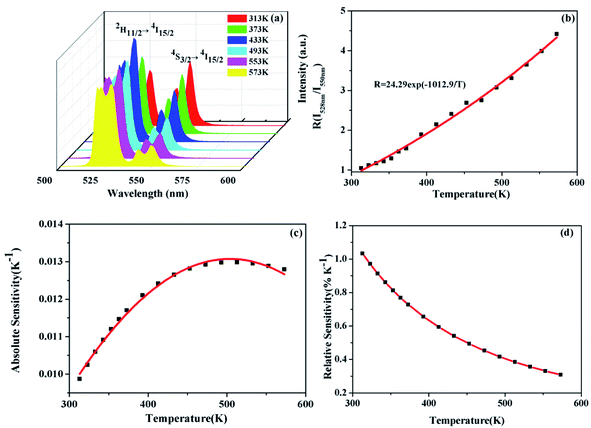 |
| Fig. 7 (a) Temperature-dependent green UC emission spectra of 1%Er3+/1%Yb3+ co-doped SrWO4 phosphor at 313–573 K. (b) dependence of FIR for I528 nm/I550 nm on absolute temperature. (c and d) The variation of absolute sensitivity and the relative sensitivity for SrWO4:1%Er3+/1% Yb3+ phosphor with temperature between 313 K and 573 K. | |
The changing of FIR of I528 nm/I550 nm with temperature is displayed in Fig. 7(b), which is fitted by eqn (6). According to the fitting curve of the experimental data, the calculated C value is 24.29 and ΔE/k is 1012.9, so the ΔE between 2H11/2 and 4S3/2 is 703.7 cm−1, which is very close to the experiment value of 758 cm−1, the latter is obtained from the UC emission spectrum. Fig. 7(c) shows the Sa of SrWO4:1%Er3+/1%Yb3+ phosphor. The Sa of the phosphor increases at first and then decreases, reaching a maximum of 0.013 K−1 at 513 K. In Fig. 7(d) the Sr also is calculated, and the Sr reaches a maximum of 1.03% K−1 at 313 K.
In addition, thermal resolution (ΔT) is also a key parameter, which can be defined as:
|
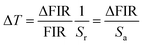 | (7) |
where ΔFIR is the uncertainty of FIR,
Sr is the relative sensitivity, and
Sa is the absolute sensitivity. The ΔFIR can be calculated by the following equation.
|
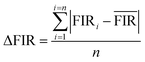 | (8) |
where

and the corresponding uncertainty ΔFIR are the average and the standard deviation values from 20 measurements at each temperature, FIR
i is the
i-th FIR measured at the same temperature and
n is the number of measurements.
For SrWO4:Ho3+/Yb3+ phosphor, the thermal resolution of temperature measurement based on the non-TCLs (5S2, 5F4/5F5) FIR has been investigated. While the thermal resolution of temperature measurement based on the TCLs (2H11/2/4S3/2) also has been discussed for SrWO4:Er3+/Yb3+ phosphor. The values of ΔFIR and ΔT are presented among the range of 303–573 K in Fig. 8(a) and (b), respectively. It can be seen that the ΔFIR of SrWO4:Ho3+/Yb3+ is lower than that of SrWO4:Er3+/Yb3+. At 303 K, the ΔT of SrWO4:Ho3+/Yb3+ and SrWO4:Er3+/Yb3+ have the minimum values of 0.72 K and 1.14 K, respectively. The values of ΔT become increasingly with the temperature enhancement. Therefore, the samples have better accuracy at lower temperature.
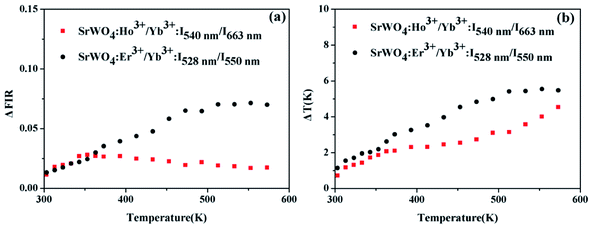 |
| Fig. 8 (a) ΔFIR and (b) ΔT of SrWO4:Ho3+/Yb3+ based on the non-TCLs (5S2, 5F4/5F5) and SrWO4:Er3+/Yb3+ based on the TCLs (2H11/2/4S3/2) pair at 303–573 K. | |
In order to correctly evaluate experimental results, the other materials doped with different RE ions are compared in Table 1. It can clearly conclude that the sensitivity of our sample is higher than that of the most of samples in the table.
Table 1 Comparison of the maximum sensitivity of Ho3+(Er3+)/Yb3+ doped in different material
Materials |
Energy level |
Sr (K−1) |
Sa (K−1) |
Reference |
Bi2SiO5:Yb3+,Tm3+ |
3F3,/2/1G4 |
1.95% (300 K) |
0.0168 (300 K) |
7 |
NaGdF4:Er3+/Yb3+ |
2H11/2/4S3/2 |
1.29% (303 K) |
0.0382 (363 K) |
28 |
LaF3:Er3+/Yb3+ |
2H11/2/4S3/2 |
844/T−2 (150 K) |
0.0025 (400 K) |
29 |
Y2O3:Ho3+/Yb3+/Zn3+ |
5F3/3K8 |
1067.76/T−2 (299 K) |
0.003 (673 K) |
30 |
NaLa(MnO4)2:Sm3+/Tb3+ |
4G5/2/5D4 |
1.93 (498 K) |
0.119 (265 K) |
31 |
BaY2Si3O10:Ho3+/Yb3+ |
5F5/5S2,5F4 |
0.49% (298 K) |
0.023 (298–448 K) |
32 |
BaY2Si3O10:Er3+/Yb3+ |
4F9/2/2H11/2 |
0.78% (298 K) |
0.091 (298 K) |
32 |
LaAlO3:Er3+/Yb3+ |
2H11/2/4S3/2 |
575.27/T−2 (281 K) |
0.0032 (281 K) |
33 |
NaY(WO4)2:Er3+/Yb3+ |
2H11/2/4S3/2 |
1043.12/T−2 (133 K) |
0.0112 (515 K) |
34 |
SrWO4:Er3+/Yb3+ |
2H11/2/4S3/2 |
1.03% (313 K) |
0.013 (513 K) |
This work |
SrWO4:Ho3+/Yb3+ |
5S2/5F4 |
1.27% (573 K) |
0.0017 (573 K) |
This work |
SrWO4:Ho3+/Yb3+ |
5S2, 5F4/5F5 |
0.41% (393 K) |
0.0158 (303 K) |
This work |
4. Conclusion
In conclusion, the SrWO4:Ho3+(Er3+)/Yb3+ phosphors are successfully prepared by solid state reaction method. The UC luminescence properties of SrWO4:Ho3+(Er3+)/Yb3+ samples are investigated under 980 nm excitation. Furthermore, the temperature sensing properties of the Ho3+:5S2/5F4 (TCLs), Er3+:2H11/2/4S3/2 (TCLs) and the Ho3+:5S2, 5F4/5F5 (non-TCLs) are investigated in the temperature range of 303–573 K. Specifically, the absolute sensitivity value reaches 0.0158 K−1 at 303 K for Ho3+:5S2, 5F4/5F5 (non-TCLs) and the relative sensitivity value reaches 0.41% at 393 K, which is superior to most optical temperature sensing materials doped with RE3+ ions. It means that the SrWO4:Ho3+/Yb3+ can achieve higher absolute sensitivity by FIR of non-TCLs, as well as it has potential application in optical temperature sensors.
Conflicts of interest
There are no conflicts to declare.
Acknowledgements
This work was supported by Natural Science Foundation of China (Grant No: 61705077); Science Foundation of Jilin Province Education Department (JJKH20190853KJ); Project of Jilin Provincial Science and Technology Department (No: 20190303064SF, 20200403072SF); Project of Jilin Province Development and Reform Commission (2019C048-4, 2020C021-5).
References
- X. F. Wang, Q. Liu, Y. Y. Bu, C. S. Liu, T. Liu and X. H. Yan, Optical temperature sensing of rare-earth ion doped phosphors, RSC Adv., 2015, 5(105), 86219–86236 RSC.
- M. Back, E. Casagrande, E. Trave, D. Cristofori, E. Ambrosi, F. Dallo, M. Roman, J. Ueda, J. Xu, S. Tananbe, A. Benedetti and P. Riello, Confined-Melting-Assisted Synthesis of Bismuth Silicate Glass-Ceramic Nanoparticles: Formation and Optical Thermometry Investigation, ACS Appl. Mater. Inter., 2020, 12, 55195–55204 CrossRef CAS PubMed.
- S. Balabhadra, M. L. Debasu, C. D. S. Brites, R. A. S. Ferreira and L. D. Carlos, Upconverting Nanoparticles Working as Primary Thermometers in Different Media, J. Phys. Chem. C., 2017, 121(25), 13962–13968 CrossRef CAS.
- M. Back, J. Ueda, M. G. Brik and S. Tananbe, Pushing the Limit of Boltzmann Distribution in Cr3+-Doped CaHfO3 for Cryogenic Thermometry, ACS Appl. Mater. Inter., 2020, 12(34), 38325–38332 CrossRef CAS PubMed.
- A. Ćirić, T. Gavrilović and M. D. Dramićanin, Luminescence Intensity Ratio Thermometry With Er3+: Performance Overview, Crystals, 2021, 11(2), 189 CrossRef.
- M. Back, E. Casagrande, C. A. Brondin, E. Ambrosi, D. Cristofori, J. Ueda, S. Tanabe, E. Trave and P. Riello, Lanthanide-Doped Bi2SiO5@SiO2 Core–Shell Upconverting Nanoparticles for Stable Ratiometric Optical Thermometry, ACS Appl. Nano Mater., 2020, 3, 2594–2604 CrossRef CAS.
- E. Casagrande, M. Back, D. Cristofori, J. Ueda, S. Tanabe, S. Palazzolo, F. Rizzolio, V. Canzonieri, E. Trave and P. Riello, Upconversion-mediated Boltzmann thermometry in double-layered Bi2SiO5:Yb3+,Tm3+@SiO2 hollow nanoparticles, J. Mater. Chem. C, 2020, 8, 7828–7836 RSC.
- S. S. Zhou, S. Jiang, X. T. Wei, Y. H. Chen, C. K. Duan and M. Yin, Optical thermometry based on upconversion luminescence in Yb3+/Ho3+ co-doped NaLuF4, J. Alloys Compd., 2014, 588, 654–657 CrossRef CAS.
- M. Back, J. Ueda, J. Xu, D. Murata, M. G. Brik and S. Tanabe, Ratiometric Luminescent Thermometers with a Customized Phase-Transition-Driven Fingerprint in Perovskite Oxides, ACS Appl. Mater. Inter., 2019, 11, 38937–38945 CrossRef CAS PubMed.
- S. A. Wade, S. F. Collins and G. W. Baxter, Fluorescence intensity ratio technique for optical fiber point temperature sensing, J. Appl. Phys., 2003, 94(8), 4743–4756 CrossRef CAS.
- M. Back, J. Ueda, H. Nambu, M. Fujita, A. Yamamoto, H. Yoshida, H. Tanaka, M. G. Brik and S. Tanabe, Boltzmann Thermometry in Cr3+-Doped Ga2O3 Polymorphs: The Structure Matters, Adv. Optical Mater., 2021, 9, 2100033 CrossRef CAS.
- Y. Zhao, X. Wang, Y. Zhang, Y. Li and X. Yao, Optical temperature sensing of up-conversion luminescent materials: Fundamentals and progress, J. Alloy Cod., 2020, 817, 152691 CrossRef CAS.
- M. Back, J. Ueda, J. Xu, K. Asami, M. G. Brik and S. Tananbe, Effective Ratiometric Luminescent Thermal Sensor by Cr3+-Doped Mullite Bi2Al4O9 with Robust and Reliable Performances, Adv. Optical Mater., 2020, 8(11), 2000124 CrossRef CAS.
- C. Shivakumara, R. Saraf, S. Behera, N. Dhananjaya and H. Nagabhushana, Scheelite-type MWO4(M = Ca, Sr, and Ba) nanophosphors: Facile synthesis, structural characterization, photoluminescence, and photocatalytic properties, Mater, Res Bull, 2015, 61, 422–432 CrossRef CAS.
- A. Y. Lan, B. Li, H. Shen and J. L. Zhang, SrWO4:Ho3+, Yb3+, Tm3+ microspheres with white-light emission:synthesis and luminescence, J. Mater. Sci.: Mater. Electron., 2015, 26(3), 1695–1699 CrossRef CAS.
- A. Pandey, V. K. Rai, V. Kumar, V. Kumar and H. C. Swart, Upconversion based temperature sensing ability of Er3+–Yb3+codoped SrWO4: An optical heating phosphor, Sens. Actuators, B, 2015, 209, 352–358 CrossRef CAS.
- H. L. Song, C. Wang, Q. Han, X. Y. Tang, W. C. Yan, Y. F. Chen, J. F. Jiang and T. G. Liu, Highly sensitive Tm3+/Yb3+codoped SrWO4 for optical thermometry, Sens. Actuators, A, 2018, 271, 278–282 CrossRef CAS.
- H. L. Song, Q. Han, C. Wang, X. Y. Tang, W. C. Yan, Y. F. Chen, X. R. Zhao, J. F. Jiang and T. G. Liu, Optical temperature sensing properties of Sm3+ doped SrWO4 phosphor, Opt. Mater., 2018, 78, 402–406 CrossRef CAS.
- H. L. Song, Q. Han, X. Y. Tang, X. R. Zhao, K. Ren and T. G. Liu, Nd3+/Yb3+ codoped SrWO4 for highly sensitive optical thermometry based on the near infrared emission, Opt. Mater., 2018, 84, 263–267 CrossRef CAS.
- F. Huang, Y. Gao, J. C. Zhou, J. Xu and Y. S. Wang, Yb3+/Er3+ co-doped CaMoO4: a promising green upconversion phosphor for optical temperature sensing, J. Alloys Compd., 2015, 639, 325–329 CrossRef CAS.
- J. Zhou, Y. Q. Chen, R. S. Lei, H. P. Wang, Q. G. Zhu, X. M. Wang, Y. Q. Wu, Q. H. Yang and S. Q. Xu, Excellent photoluminescence and temperature sensing properties in Ho3+/Yb3+ codoped (Y0.88La0.09Zr0.03)2O3 transparent ceramics, Ceram. Int., 2019, 45(6), 7696–7702 CrossRef CAS.
- X. N. Chai, J. Li and X. S. Wang, Upconversion Luminescence and Temperature Sensing Properties of Ho3+/Yb3+-Codoped ZnWO4 Phosphors Based on Fluorescence Intensity Ratios, RSC Adv., 2017, 64, 40046–40052 RSC.
- L. Lei, D. Q. Chen, C. Li, F. Huang, J. J. Zhang and S. Q. Xu, Inverse thermal quenching effect in lanthanide-doped upconversion nanocrystals for anti-counterfeiting, J. Mater. Chem. C, 2018, 6(20), 5427–5433 RSC.
- P. Haro-González, S. F. León-Luis, S. González-Pérez and I. R. Martín, Analysis of Er3+ and Ho3+ codoped fluoroindate glasses as wide range temperature sensor, Mater. Res. Bull., 2011, 46, 1051–1054 CrossRef.
- P. Du, L. H. Luo and J. S. Yu, Low-temperature thermometry based on upconversion emission of Ho/Yb-codoped Ba0.77Ca0.23TiO3 ceramics, J. Alloys Compd., 2015, 632, 73–77 CrossRef CAS.
- H. Y. Lu, H. Y. Hao, G. Shi, Y. C. Gao, R. X. Wang, Y. L. Song, Y. X. Wang and X. R. Zhang, Optical temperature sensing in β-NaLuF4:Yb3+/Er3+/Tm3+ based on thermal, quasi-thermal and non-thermal coupling levels, RSC Adv., 2016, 6, 55307–55311 RSC.
- X. N. Chai, J. Li, X. S. Wang, Y. X. Li and X. Yao, Color-tunable upconversion photoluminescence and highly performed optical temperature sensing in Er3+/Yb3+ co-doped ZnWO4, Opt. Express, 2016, 24(20), 22438–22447 CrossRef CAS PubMed.
- J. M. Wang, H. Lin, Y. Cheng, X. S. Cui, Y. Gao, Z. L. Ji, J. Xu and Y. S. Wang, A novel high-sensitive upconversion thermometry strategy: Utilizing synergistic effect of dual-wavelength lasers excitation to manipulate electron thermal distribution, Sens. Actuators, B, 2019, 278, 165–171 CrossRef CAS.
- H. J. Zhang, X. B. Dong, L. Y. Jiang, Y. Yang, X. R. Cheng and H. M. Zhao, Comparative analysis of upconversion emission of LaF3:Er/Yb and LaOF:Er/Yb for temperature sensing, J. Mol. Struct., 2020, 1206, 127665 CrossRef CAS.
- A. Pandey and V. K. Ray, Improved Luminescence and Temperature Sensing Performance of Ho3+, Yb3+, Zn2+: Y2O3 Phosphor, Dalton Trans., 2013, 42, 11005–11011 RSC.
- Y. Zhu, Q. Meng, W. Sun and S. Lü, Sm3+, Tb3+ co-doped NaLa(MoO4)2 temperature sensing materials based on the fluorescence intensity ratio, J. Alloys Compd., 2019, 784, 456–462 CrossRef CAS.
- H. Q. Ge and J. Zhang, Investigation on luminescence properties of BaY2Si3O10:Er3+/Ho3+-Yb3+ for optical temperature sensing, J. Mater. Sci.: Mater. Electron., 2018, 29, 20033–20039 CrossRef CAS.
- G. F. Liu, L. L. Fu, Z. Y. Gao, X. X. Yang, Z. L. Fu, Z. Y. Wang and Y. M. Yang, Investigation on the Temperature Sensing Behavior in Yb3+ Sensitized Er3+ doped Y2O3, YAG and LaAlO3 Phosphors, RSC Adv., 2015, 5(64), 51820–51827 RSC.
- P. Du, L. H. Luo and J. S. Yu, Upconversion emission, cathodoluminescence and temperature sensing behaviors of Yb3+ ions sensitized NaY(WO4)2:Er3+ phosphors, Ceram. Int., 2015, 42(5), 5635–5641 CrossRef.
|
This journal is © The Royal Society of Chemistry 2021 |
Click here to see how this site uses Cookies. View our privacy policy here.