DOI:
10.1039/D1RA06472J
(Paper)
RSC Adv., 2021,
11, 36958-36964
Magnetic sulfonated polysaccharides as efficient catalysts for synthesis of isoxazole-5-one derivatives possessing a substituted pyrrole ring, as anti-cancer agents†
Received
27th August 2021
, Accepted 4th November 2021
First published on 17th November 2021
Abstract
Four polysaccharides (chitosan, cellulose, starch, and pectin) were magnetized with magnetic iron oxide (Fe3O4) and then sulfonated (except pectin) with chlorosulfonic acid. The obtained solid acids were used as a catalyst in three-component reactions between N-substituted-2-formylpyrrole, hydroxylamine-hydrochloride, and β-keto esters for the synthesis of 4-(2-pyrrolyl) methylene-isoxazole-5-ones. The optimal catalyst system was selected and studied by IR, SEM, TEM and XRD methods. The diverse isoxazoline derivatives (obtained via a mild and simple approach) were also fully characterized by spectroscopic methods and screened for anti-cancer activities against HT-29 and MCF-7 colon and breast cancer and HEK 293 normal cells. The results revealed interesting anti-cancer activities.
Introduction
Structurally modified carbohydrates are of great interest due to their wide applications in physiological, industrial, and catalytic processes.1,2 Meanwhile, functionally improved polysaccharides such as sulfonated backbones play important roles as heterogeneous catalysts in a variety of organic syntheses.3–5 These –SO3H appended biopolymers with high acid site density as well as recyclability and biodegradability, could be efficient solid acid catalysts in many attractive multicomponent reactions.6–8 Considering that magnetized substrates facilitate the separation process and reusability of the catalytic systems, their use has received special attention in improving the catalyst structure.9 Among the known multicomponent reactions, those leading to heterocyclic ring formation are particularly interesting. Isoxazole is a heterocycle ring with nitrogen and oxygen heteroatoms at the adjacent position whose derivatives are of great biological and medicinal importance10–13 as well as optical and photochromic applications.14,15 Isoxazol-5-ones are also one of the valuable synthetic targets because of their broad spectrum of pharmaceutical properties such as tyrosine inhibitory16 and antitumor activity.17,18 A catalytic one-pot reaction of aldehydes, β-ketoesters, and hydroxylamine-hydrochloride is the known method for the synthesis of these compounds.19,20 The development of several synthetic methodologies by utilizing diverse catalytic substrates exhibits the growing interests into the synthesis of many isoxazole-5-one derivatives.21–24 Various magnetic functionalized polysaccharides have been recently prepared by our group whose copper complexes showed high catalytic activities for the synthesis of diverse N-sulfonylamidine derivatives.25–28 In this work we report the preparation and fully characterization of Fe3O4 magnetized sulfonated polysaccharides such as chitosan, cellulose and starch and evaluation of their catalytic activities in three-component reactions between N-substituted-pyrrole-2-carboxaldehyde, hydroxylamine-hydrochloride, and β-ketoesters to afford pyrrole containing isoxazole-5-ones. Since the hybrid molecules containing a pyrrole ring with other heterocyclic moiety, have shown biological activities such as antibacterial, anti-tumor, and antifungal (Fig. 1),29–31 we used N-substituted-2-formylpyrrole as aldehyde partner at the mentioned reactions. The synthesized compounds were screened for anti-cancer activity against HT-29 and MCF-7 of colon and breast cancer and HEK 293 normal cells which the results are presented.
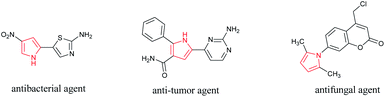 |
| Fig. 1 Structure of pyrrole based bioactive compounds. | |
Results and discussion
Synthesis and characterization of magnetic sulfonated polysaccharides
In this work, we first prepared the magnetic polysaccharides based on chitosan, cellulose, starch and pectin.
Commercially available polysaccharide (Fig. 2) was treated with FeCl3·6H2O and FeCl2·4H2O (in a molar ratio 2
:
1) in the aqueous alkaline mixture at 80 °C to prepare magnetic solid, which was separated using an external magnetic field.25,32–34 Magnetic product (Fe3O4@polymer) was then reacted with chlorosulfonic acid to form the sulfonated magnetic polymer (Fe3O4@polymer–SO3H).6,35,36 The typical route to prepare the chitosan based product is illustrated in Scheme 1. Pectin was not sulfonated in order to evaluate its ability to release of H+ from the carboxylic acid groups and its catalytic proficiency without sulfonic acid groups.
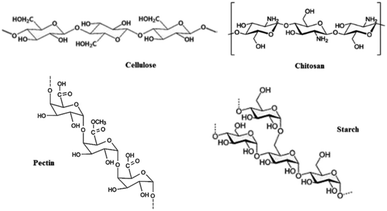 |
| Fig. 2 Structures of studied polysaccharides in this work. | |
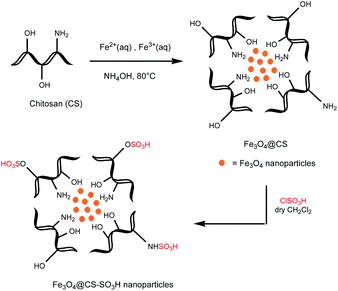 |
| Scheme 1 Synthesis of Fe3O4@CS–SO3H nanoparticles. | |
We then used potentiometric titration to determine the acidity of the obtained solid acids. A mixture of solid acid (100 mg) in distilled water (25 mL) was stirred at room temperature for 24 hours and then dispersed with ultrasonic probe for 1 hour (frequency of 70 Hz). The mixture was then titrated with NaOH solution (0.08 N) and potentiometric curve was obtained (Fig. 3). According to data of the equivalence point, the acidity was calculated as shown in Table 1. As seen, the chitosan and cellulose based acids have a similar state in terms of initial pH and H+ release power. The starch containing composite showed the lower acidity and the pectin based acid was not be titrated due to its higher primary pH.
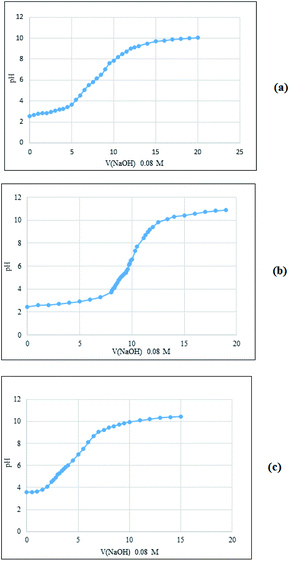 |
| Fig. 3 The potentiometric curves of Fe3O4@chitosan–SO3H (a), Fe3O4@cellulose–SO3H (b), Fe3O4@starch–SO3H (c). | |
Table 1 Potentiometric data of the functionalized polysaccharides
Solid acids |
Primary pH |
Volume of used NaOH solution up to the equivalence point (mL) |
pH at the equivalence point |
Acidity (mmol of H+ per 100 mg solid acid) |
Fe3O4@chitosan–SO3H |
2.58 |
8.5 |
6.49 |
0.7 |
Fe3O4@cellulose–SO3H |
2.45 |
9.9 |
6.47 |
0.8 |
Fe3O4@starch–SO3H |
3.57 |
4 |
6.03 |
0.3 |
Fe3O4@pectin |
7.54 |
— |
— |
— |
These solid acids were then used as heterogeneous catalysts in multi-component synthesis of pyrrole containing isoxazole-5-one derivatives. As will be seen, the catalyst containing chitosan showed higher catalytic activity than the others. For this reason, we report herein full characterization of this catalyst (Fe3O4@chitosan–SO3H) by IR, SEM, EDX, VSM, TEM, and XRD methods. Some information about the other three catalysts is given in the ESI.† Fig. 4 illustrates the FT-IR spectra of the generated species within the synthesis of the nanocatalyst. As shown in the FT-IR spectrum of chitosan (a), the strong and broad absorption band at around 3430 cm−1, overlapped with the N–H stretching band, is related to the OH group. The bands at 2873 cm−1 and 1384 cm−1 are imputed to the C–H stretching vibrations of the CH2 groups and the C–H bending vibrations, respectively. The particular signals for the amine (N–H) bending vibration arise at 1606 cm−1 for chitosan. In the FT-IR spectrum of magnetic chitosan (b), absorption at 578 cm−1 assigned to the vibration of Fe–O in Fe3O4. The spectrum (c) also appears the absorption bands at 1249 and 1027 cm−1 which are ascribed to –S
O stretching vibration of –SO3H groups.
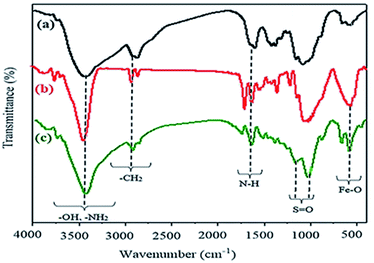 |
| Fig. 4 FT-IR spectra for chitosan (a), Fe3O4@CS (b), and Fe3O4@CS–SO3H (c). | |
The XRD patterns of the synthesized nanocatalysts were indicated in Fig. 5(I). The specific peaks of the catalyst at 2θ = 32.81, 35.68, 43.65, 53.38, 57.37, and 63.55 degrees are related to inverse cubic spinel magnetite (Fe3O4) crystal structure. No improbity in the XRD patterns suggests the foundation of pure Fe3O4 nanoparticles. The weak and small broad bands (curve b) in the range of 2θ = 25–28 represent the entity of amorphous sulfonated chitosan. The quantity of magnetization saturation of the Fe3O4@CS and Fe3O4@CS–SO3H nanocomposites was obtained using a vibrating sample magnetometer at room temperature with values 30.25 and 20.03 emu g−1 respectively (Fig. 5(II)). These results demonstrate that the catalyst is paramagnetic and consequently can be easily separated from the reaction medium through an external magnetic field and reused. Fig. 5(III) also shows the surface morphology and particle size distribution of the catalyst that were studied by SEM analysis. The SEM images illustrate the uniform dispersity of spherical particles and also the attendance of Fe3O4 on chitosan support with average particle size of 9–15 nm. The EDX spectroscopy was carried out to study the incidence of elemental signals of prepared Fe3O4@CS–SO3H nanocomposite (Fig. 5(IV)). The results illustrate the presence of N, O, C, Fe, and S elements in the nanocatalyst and confirm that chitosan polymer is covered on the surface of iron oxide nanoparticles.
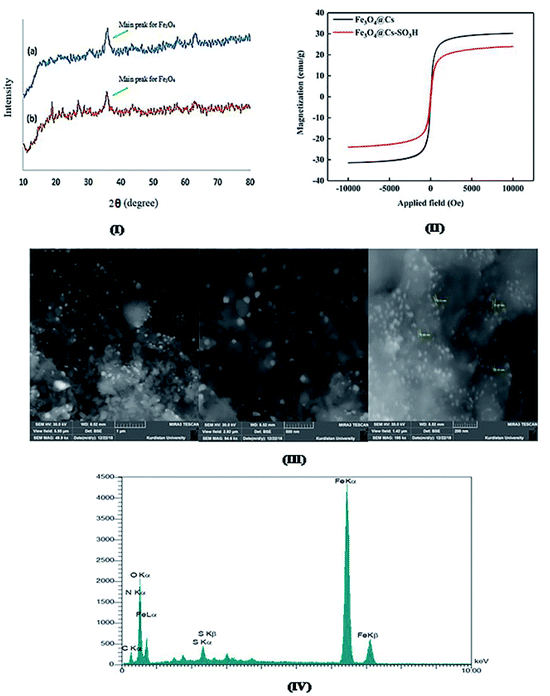 |
| Fig. 5 (I) XRD patterns of Fe3O4@CS (a) and Fe3O4@CS–SO3H (b); (II) magnetic hysteresis curves for prepared nanocomposites; (III) SEM images of the Fe3O4@chitosan–SO3H: 1 μm (left), 500 nm (middle), 200 nm (right); (IV) EDX pattern of the Fe3O4@CS–SO3H. | |
The TEM micrograph of the Fe3O4@chitosan–SO3H which is shown in Fig. 6, reveals the distribution of Fe3O4 particles on functionalized chitosan. The size of nanoparticles was calculated using image J software to be approximately 22 nm.
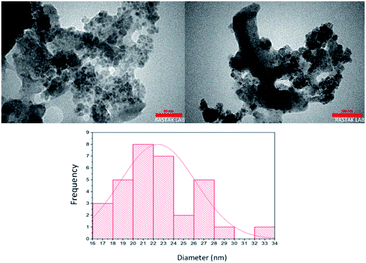 |
| Fig. 6 TEM images of Fe3O4@chitosan–SO3H. | |
Application of the catalysts towards one-pot synthesis of the novel isoxazole-5-one derivatives
We used commercial pyrrole-2-carbaldehyde 1a and its N-methyl derivative 1b as well as the prepared N-substituted derivatives 1c–e (Scheme 2), as aldehyde partner in the desired three-component reactions. As shown, substitution reactions of 1a with benzyl chloride or propargyl bromide in the presence of K2CO3 in DMF gave the compounds 1c
37 and 1d
38 respectively. CuI catalyzed 1,3-dipolar cycloaddition of 1d with benzyl azide,39 resulted in the new triazole derivative 1e. Three-component reaction between 1-benzylpyrrole-2-carboxaldehyde 1c, hydroxylamine-hydrochloride and methyl acetoacetate to obtain product 3e, was selected as a model to optimize reaction conditions (Table 2). Initially, four kinds of magnetic catalysts containing Fe3O4@chitosan–SO3H, Fe3O4@cellulose–SO3H, Fe3O4@starch–SO3H, and Fe3O4@pectin were examined in equal amounts (0.04 g) per 1 mmol of aldehyde component (entries 1–4). As shown, the chitosan containing catalyst resulted in higher yield of 3e (89%) at shorter time (2 hours). Changing the amount of this catalyst did not improve the result (entries 5 and 6). Although solvent-free conditions reduced the yield of the product (entry 8), the use of ethanol had a good effect (entry 7) and even was better when using ethyl benzoyl acetate as β-ketoester. The use of other solvent such as THF and CH2Cl2 increased the purification steps and reduced the yield of 3e (entries 9 and 10). Three-component condensation of N-substituted-2-formylpyrrole 1a–e, hydroxylamine hydrochloride, and β-keto ester 2a,b in the presence of Fe3O4@chitosan–SO3H in EtOH or H2O at room temperature, gave a series of 4-(2-pyrrolyl)methylene-isoxazole-5-ones 3a–j in good to excellent yields (Table 3). 5-Methylfuran-2-carboxaldehyde was also applied as aldehyde partner in this reaction and product 3k was obtained as yellow solid.
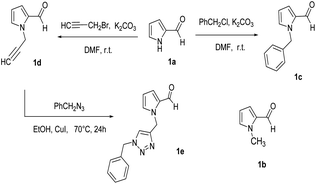 |
| Scheme 2 The preparation of aldehyde precursors. | |
Table 2 Optimization of the reaction conditionsa
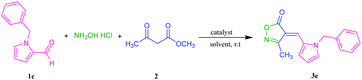
|
Entry |
Catalyst (mg) |
Solvent |
Time (h) |
Yieldb (%) |
Reaction conditions: aldehyde (1 mmol), hydroxylamine hydrochloride (1.5 mmol), β-keto ester (1.5 mmol), room temperature, solvent (10 mL). Isolated yields. |
1 |
Fe3O4@pectin (40) |
H2O |
5 |
58 |
2 |
Fe3O4@starch–SO3H (40) |
H2O |
3 |
65 |
3 |
Fe3O4@cellulose–SO3H (40) |
H2O |
2 |
82 |
4 |
Fe3O4@chitosan–SO3H (40) |
H2O |
2 |
89 |
5 |
Fe3O4@chitosan–SO3H (50) |
H2O |
2 |
89 |
6 |
Fe3O4@chitosan–SO3H (30) |
H2O |
3 |
75 |
7 |
Fe3O4@chitosan–SO3H (40) |
EtOH |
2 |
88 |
8 |
Fe3O4@chitosan–SO3H (40) |
Solvent-free |
2 |
30 |
9 |
Fe3O4@chitosan–SO3H (40) |
THF |
2 |
63 |
10 |
Fe3O4@chitosan–SO3H (40) |
CH2Cl2 |
2 |
59 |
Table 3 Synthesis of isoxazole-5-(4H)-one derivatives 3a–k in the presence of Fe3O4@chitosan–SO3Ha,b
Isolated yield. The reactions were carried out with 1a–c (1 mmol), β-keto ester 2 (1.5 mmol), hydroxylamine hydrochloride (1.5 mmol) in ethanol or water (10 mL) at room temperature. |
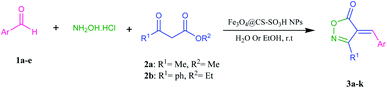 |
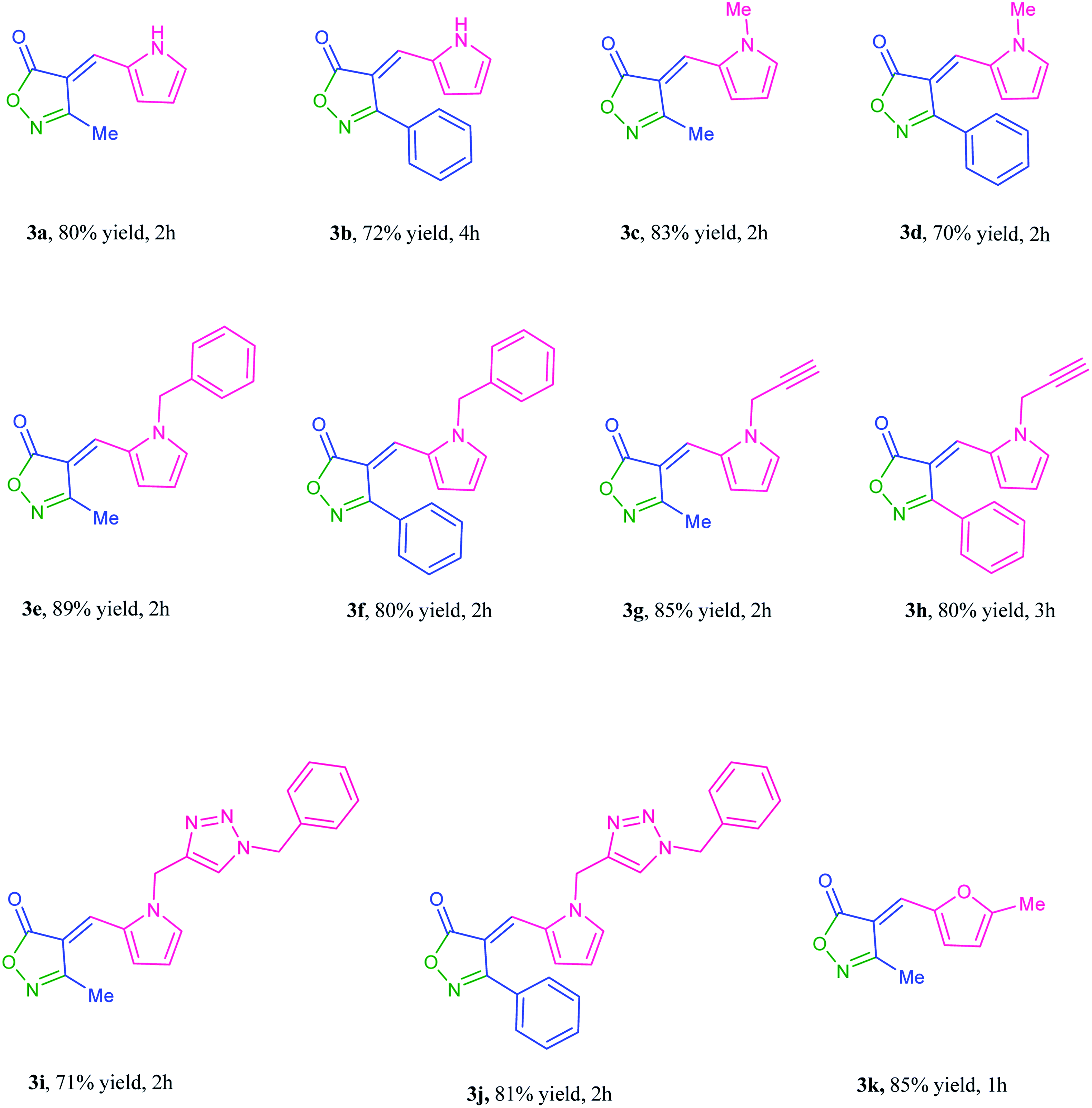 |
It is undeniable that the recovery and reuse of catalysts are important for a catalytic procedure. In this regard, the recyclability of the Fe3O4@chitosan–SO3H was explored using the model reaction of N-benzyl-2-formylpyrrole 1c, hydroxylamine hydrochloride, and methyl acetoacetate under identical reaction conditions. Once the reaction is complete, the recovered magnetic nanocatalyst was washed with ethanol, dried at room temperature, and reused for more runs. Heterogeneous and magnetic property of the Fe3O4@chitosan–SO3H which facilitates the recovery of the nanocatalyst from the reaction mixture by an external magnet, allowed recyclization and four times reuse the catalyst. The obtained yields in these 4 uses were: 89%, 85%, 81% and 78% for product 3e. A possible mechanism for the synthesis of isoxazole-5-(4H)-one, in the presence of Fe3O4@CS–SO3H, is shown in Fig. 7. In the first step, acid catalyst protonates the carbonyl group of β-ketoester and activates it for condensation with hydroxylamine to give oxime (I). In the next step, activated aromatic aldehyde reacts with the oxime intermediate through Knoevenagel condensation. Finally, the desired product 3a–k was obtained by intramolecular cyclization and elimination of alcohol.
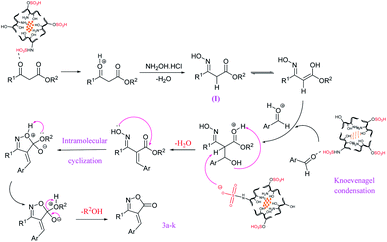 |
| Fig. 7 The proposed mechanism for the formation of isoxazole-5-(4H)-one derivatives. | |
Biological studies
The synthesized 4-arylmethylene-isoxazol-5(4H)-ones 3a–k were examined for cell cytotoxicity analyses against three different cancer cell lines: normal cell (HEK 293), human colon adenocarcinoma (HT-29) and human breast adenocarcinoma (MCF-7) using the MTT assay.40–44 Afterward, doxorubicin was utilized as a prominent anticancer medicine for positive control of this research. The antiproliferative efficacy values are used as IC50 which are regarded as the levels of the synthesized materials that cause about 50% cell proliferation (Table 4). The IC50 values of at least three separated experiments were averaged. Cell viability was measured through MTT assay as described in the methods subsection. The examination of cytotoxicity properties of the compounds 3a–k showed that all of eleven desired compounds possessed cytotoxicity activity against MCF-7 and HT-29 cancer cell lines. The compounds 3b, 3e, 3f, 3h, 3j and 3k exhibited good cytotoxicity activities against MCF-7 cell line and the compounds 3b, 3f, 3h and 3k against HT-29 cell line. It has been obvious that the derivative 3k containing furan ring indicated remarkable cytotoxic activity against both HT-29 and MCF-7 cell lines (Table 4). Moreover, the cytotoxicity activities of desired compounds 3a–k against HEK 293 normal cells were also investigated and the results showed poor cytotoxic effects. The cytotoxic activities of all derivatives have been graphically exhibited in Fig. 8.
Table 4 IC50 (μM) of the synthesized compounds against different cancer cell lines
Product |
MCF-7 |
HT-29 |
HEK 293 |
3a |
10 |
12 |
102 |
3b |
3 |
10 |
98 |
3c |
7 |
15 |
189 |
3d |
5 |
13 |
201 |
3e |
4 |
15 |
167 |
3f |
3 |
10 |
79 |
3g |
5 |
15 |
94 |
3h |
4 |
10 |
109 |
3i |
7 |
18 |
171 |
3j |
4 |
15 |
128 |
3k |
2 |
10 |
101 |
Doxorubicin |
2.4 ± 0.12 |
0.49 |
1.30 |
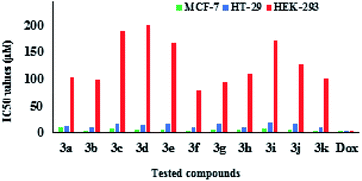 |
| Fig. 8 Graphical presentation of the cytotoxic activity of the 4-arylmethylene-isoxazole-5(4H)-ones. | |
Conclusions
We report in this research, the use of Fe3O4 incorporated sulfonated polysaccharides as solid acid catalysts for the synthesis of novel isoxazole-5-one derivatives comprising substituted pyrrole ring. The three-component reactions of N-substituted-2-formylpyrrole, hydroxylamine-hydrochloride, and β-ketoesters under optimized conditions (in the presence of chitosan based catalyst) resulted in the desired products in good yields. The obtained products 3a–k were also examined for cell cytotoxicity analyses against human colon adenocarcinoma (HT-29) and human breast adenocarcinoma (MCF-7) using the MTT assay. The results showed good cytotoxicity for compounds 3b, 3f, 3h and 3k and relatively poor cytotoxicity against HEK 293 normal cells.
Conflicts of interest
There are no conflicts to declare.
Acknowledgements
This project has been supported by a research grant from the University of Tabriz (number: 793). The authors are grateful for the research interests of the University of Tabriz for this grant.
Notes and references
- H. E. Caputo, J. E. Straub and M. W. Grinstaff, Chem. Soc. Rev., 2019, 48, 2338–2365 RSC.
- R. Mahajan, R. Mahajan, A. Selim, K. M. Neethu, S. Sharma, V. Shanmugam and G. Jayamurugan, Nanotechnol., 2021, 32, 475704–475716 CrossRef PubMed.
- A. Pourjavadi, F. Seidi, S. S. Afjeh, N. Nikoseresht, H. Salimi and N. Nemati, Starch/Staerke, 2011, 63, 780–791 CrossRef CAS.
- A. Shaabani, A. Rahmati and Z. Badri, Catal. Commun., 2008, 9, 13–16 CrossRef CAS.
- I. M. Lokman, U. Rashid and Y. H. Taufiq-Yap, Arabian J. Chem., 2016, 9, 179–189 CrossRef CAS.
- A. Maleki, E. Akhlaghi and R. Paydar, Appl. Organomet. Chem., 2016, 30, 382–386 CrossRef CAS.
- M. Mamaghani, K. Tabatabaeian, M. Mohammadi and A. Khorshidi, J. Chem., 2013, 2013, 1–6 Search PubMed.
- A. Shaabani, A. Maleki, J. M. Rad and E. Soleimani, Chem. Pharm. Bull., 2007, 55, 957–958 CrossRef CAS PubMed.
- K. Hasan, I. A. Shehadi, N. D. Al-Bab and A. Elgamouz, Catal, 2019, 9, 839–856 CrossRef CAS.
- C. Altug, H. Güneş, A. Nocentini, S. M. Monti, M. Buonanno and C. T. Supuran, Bioorg. Med. Chem., 2017, 25, 1456–1464 CrossRef CAS PubMed.
- C. Changtam, P. Hongmanee and A. Suksamrarn, Eur. J. Med. Chem., 2010, 45, 4446–4457 CrossRef CAS PubMed.
- P. Diana, A. Carbone, P. Barraja, G. Kelter, H.-H. Fiebig and G. Cirrincione, Bioorg. Med. Chem., 2010, 18, 4524–4529 CrossRef CAS PubMed.
- S. Suryawanshi, A. Tiwari, N. Chandra and S. Gupta, Bioorg. Med. Chem. Lett., 2012, 22, 6559–6562 CrossRef CAS PubMed.
- G. Liu, M. Liu, S. Pu, C. Fan and S. Cui, Tetrahedron, 2012, 68, 2267–2275 CrossRef CAS.
- X.-H. Zhang, Y.-H. Zhan, D. Chen, F. Wang and L.-Y. Wang, Dyes Pigm., 2012, 93, 1408–1415 CrossRef CAS.
- S. J. Kim, J. Yang, S. Lee, C. Park, D. Kang, J. Akter, S. Ullah, Y.-J. Kim, P. Chun and H. R. Moon, Bioorg. Med. Chem., 2018, 26, 3882–3889 CrossRef CAS PubMed.
- S. K. Laughlin, M. P. Clark, J. F. Djung, A. Golebiowski, T. A. Brugel, M. Sabat, R. G. Bookland, M. J. Laufersweiler, J. C. VanRens and J. A. Townes, Bioorg. Med. Chem. Lett., 2005, 15, 2399–2403 CrossRef CAS PubMed.
- S. Rollas, Ş. Kokyan, B. K. Kaymakçıoğlu, S. Ö. Turan and J. Akbuğa, Marmara Pharm. J., 2011, 15, 94–99 CrossRef CAS.
- F. Saikh, J. Das and S. Ghosh, Tetrahedron Lett., 2013, 54, 4679–4682 CrossRef CAS.
- M. Parveen, A. Aslam, A. Ahmad, M. Alam, M. R. Silva and P. P. Silva, J. Mol. Struct., 2020, 1200, 127067 CrossRef.
- M. Ahmadzadeh, Z. Zarnegar and J. Safari, Green Chem. Lett. Rev., 2018, 11, 78–85 CrossRef CAS.
- H. Kiyani and F. Ghorbani, J. Saudi Chem. Soc., 2017, 21, S112–S119 CrossRef CAS.
- S. Fozooni, N. G. Hosseinzadeh, H. Hamidian and M. R. Akhgar, J. Braz. Chem. Soc., 2013, 24, 1649–1655 CAS.
- Q. Liu and R.-T. Wu, J. Chem. Res., 2011, 35, 598–599 CrossRef CAS.
- Z. Ghasemi, S. Shojaei and A. Shahrisa, RSC Adv., 2016, 6, 56213–56224 RSC.
- S. Shojaei, Z. Ghasemi and A. Shahrisa, Appl. Organomet. Chem., 2017, 31, 3788–3807 Search PubMed.
- S. Shojaei, Z. Ghasemi and A. Shahrisa, Tetrahedron Lett., 2017, 58, 3957–3965 CrossRef CAS.
- S. Valizadeh, Z. Ghasemi, A. Shahrisa, B. Notash, M. Pirouzmand and R. Kabiri, Carbohydr. Polym., 2019, 226, 115310–115320 CrossRef PubMed.
- S.-G. Zhang, C.-G. Liang, Y.-Q. Sun, P. Teng, J.-Q. Wang and W.-H. Zhang, Mol. Diversity, 2019, 23, 915–925 CrossRef CAS PubMed.
- V. Bhardwaj, D. Gumber, V. Abbot, S. Dhiman and P. Sharma, RSC Adv., 2015, 5, 15233–15266 RSC.
- R. A. Rane, P. Bangalore, S. D. Borhade and P. K. Khandare, Eur. J. Med. Chem., 2013, 70, 49–58 CrossRef CAS PubMed.
- H. Naeimi and S. Lahouti, J. Iran. Chem. Soc., 2018, 15, 2017–2031 CrossRef CAS.
- C. P. Okoli, E. B. Naidoo and A. E. Ofomaja, Environ. Nanotechnol. Monit. Manag., 2018, 9, 141–153 Search PubMed.
- O. A. Attallah, M. A. Al-Ghobashy, M. Nebsen, R. El-Kholy and M. Y. Salem, Environ. Sci. Pollut. Res., 2018, 25, 18476–18483 CrossRef CAS PubMed.
- J. Safari, P. Aftabi, M. Ahmadzadeh, M. Sadeghi and Z. Zarnegar, J. Mol. Struct., 2017, 1142, 33–39 CrossRef CAS.
- R. Mohammadi and M. Z. Kassaee, J. Mol. Catal. A: Chem., 2013, 380, 152–158 CrossRef CAS.
- R. Di Santo, R. Costi, M. Artico, R. Ragno, G. Greco, E. Novellino, C. Marchand and Y. Pommier, Il Farmaco, 2005, 60, 409–417 CrossRef CAS PubMed.
- P. R. Loaiza, S. Löber, H. Hübner and P. Gmeiner, Bioorg. Med. Chem., 2007, 15, 7248–7257 CrossRef PubMed.
- For preparation of benzyl azide from reaction of benzyl chloride and sodium azide, see: T. Miao and L. Wang, Synthesis, 2008, 2008, 363–368 CrossRef.
- S. Azizi, J. Soleymani and M. Hasanzadeh, Appl. Organomet. Chem., 2020, 34, e5440 CAS.
- Z. Ghasemi, S. Azizi, R. Salehi and H. S. Kafil, Monatsh. Chem., 2018, 149, 149–157 CrossRef CAS.
- S. Azizi, J. Soleymani and N. Shadjou, J. Mol. Recognit., 2020, 33, e2871 CrossRef CAS PubMed.
- M. Pirouzmand, P. S. Sani, Z. Ghasemi and S. Azizi, J. Biol. Inorg Chem., 2020, 25, 411–417 CrossRef CAS PubMed.
- E. F. Oskuie, S. Azizi, Z. Ghasemi, M. Pirouzmand, B. N. Kojanag and J. Soleymani, Monatsh. Chem., 2020, 151, 243–249 CrossRef CAS.
Footnote |
† Electronic supplementary information (ESI) available. See DOI: 10.1039/d1ra06472j |
|
This journal is © The Royal Society of Chemistry 2021 |