DOI:
10.1039/D1RA04700K
(Review Article)
RSC Adv., 2021,
11, 26354-26367
Recent progress of effect of crystal structure on luminescence properties of Ce3+–Eu2+ Co-doped phosphors
Received
17th June 2021
, Accepted 20th July 2021
First published on 2nd August 2021
Abstract
Currently, the mechanism of Ce3+–Eu2+ ET is frequently used to obtain color adjustable or white phosphors. Correspondingly, the ET efficiency from Ce3+ to Eu2+ becomes an important indication of the luminescent properties of phosphors. However, the ET efficiency calculated using the formula
does not always match the emission spectra; the transmission efficiency of Ce3+ is high, but the emission efficiency of Eu2+ is low, depending on our investigation results. In addition to this problem, here we mainly review, on the basis of substantial examples, how to boost the actual ET efficiency of Ce3+-to-Eu2+ and thus to improve the luminescent properties of phosphors through the rational design of layered crystal structure and the way of selective occupation of activator ions. Moreover, the possible physical mechanisms are proposed.
1. Introduction
In recent years, a broad spectrum of new lighting materials has emerged, and white LED (WLED) materials have gradually replaced the traditional fluorescent lamps and incandescent lamps because of their energy-saving, environmental protection, high efficiency, long life and small size.1 Due to these advantages, LEDs can be used in a wide range of areas, not only in solid state lighting, but also in other electronic devices such as displays. At present, researchers prefer to obtain color adjustable phosphors or white light phosphors by rational design of ET through rare earth (RE) ions co-doping such as Ce3+–Eu2+, Ce3+–Mn2+, Eu2+–Mn2+, Ce3+–Eu2+–Mn2+, Ce3+–Tb3+–Mn2+ and Ce3+–Tb3+–Eu2+ systems, and so forth.2–14 Among these systems, both Ce3+ and Eu2+ can emit 4f–5d transitions. Because their exposed 5d orbitals are susceptible to the crystal field environment, the luminescence range of Ce3+ can vary from the violet to red, and the spectral coverage of Eu2+ can even range from the ultraviolet to near-infrared, such as that in K3LuSi2O7.15 Therefore, rational design of Ce3+-to-Eu2+ ET has become a popular way to obtain white light phosphors.
Over the past decades, many phosphors emitting adjustable color have been prepared by doping RE ions, and we could draw a conclusion that there are two main factors affecting the luminescent properties of phosphors: the kind of activator ions and the type of hosts. Both are indispensable for phosphors to achieve good luminescent properties. First, even for the same host, different kinds of activator ions will show different phenomena.16–19 For example, solid state synthesis and tunable luminescence of Li2SrSiO4:Eu2+/Ce3+ phosphors have been reported by Wei et al. Under excitation at 365 nm, the Ce3+ exhibits a broad blue emission ranging from 380 to 500 nm centered at 425 nm, while the Eu2+ exhibits a broad green emission in the range of 480–680 nm with center at 556 nm.20 Synthesis, luminescence and ET of Ce3+-to-Eu2+ of SrSc2O4:Ce3+/Eu2+ phosphors have been reported by Zhao et al. Under excitation at 440 nm, SrSc2O4:Ce3+ shows a green emission peaking at ∼535 nm, and the spectral coverage ranges from 475 to 675 nm. Under excitation at 523 nm, SrSc2O4:Eu2+ shows a red emission ranging from 650 to 775 nm with center at ∼702 nm.21 Photoluminescence, ET and tunable color of Ce3+/Tb3+/Eu2+ activated oxynitride phosphor with high brightness has been reported by Lv et al. Ca3Al8Si4O17N4:Ce3+ shows a blue emission centering at 420 nm in the range of 340–525 nm, under excitation at 330 nm. And Ca3Al8Si4O17N4:Eu2+ shows a blue emission in the range of 400–550 nm with center at 450 nm under excitation at 350 nm.22 It can be seen that in the same host, different kinds of activator ions lead to different luminescence phenomenon.
In addition to the type of activator ions, the choice of host also has an impact on the luminescent properties of phosphors.23–40 Eu2+ is a significant activator for luminescent materials because of its unique emission properties, so it has been researched in many hosts, as proved by the abundant examples such as K3LuSi2O7:Eu2+, NaAlSiO4:Eu2+, Ca2Al3O6F:Eu2+, K2BaCa(PO4)2:Eu2+, Sr9Mg1.5(PO4)7:Eu2+, etc.15,41–44 First of all, with the variation of hosts, the emission wavelength of Eu2+ can change from the near-ultraviolet to near-infrared. Secondly, the thermal stability of phosphor materials is different in different hosts, which can be proved by the phosphors of KBa2(PO3)5 (ref. 45) and Ca2Al3O6F (ref. 42) reported by Zhao and Xia, respectively. According to these two papers, the PL intensity of Ca2Al3O6F:Eu2+ at 150 °C is only about 39% of its initial value (25 °C), but the PL intensity of KBa2(PO3)5:Eu2+ at 150 °C retains 97% of its initial value.42,45 In addition to the luminous color and the temperature stability of phosphors, host will also affect the quantum efficiency of phosphors. For example, in host CaAlSiN3, the quantum efficiency of Eu2+ is as high as 95%, but in host Ca7Mg(SiO4)4, the quantum efficiency of Eu2+ is only 30%.23,29 Therefore, the effect of crystal field environment on the luminescence properties of Eu2+ is significant, including the position and half-peak width of luminescence, as well as the temperature stability and quantum efficiency of phosphors.
From the above discussion, we can conclude that host composition and crystal structure have a great effect on the luminescence properties of Eu2+-singly doped phosphors, and in fact, for Ce3+–Eu2+ co-doped phosphors, these factors also play a key role. The luminescence properties and ET mechanism of Ce3+-to-Eu2+ have been analyzed by some research groups.46–93 For instance, the ET processes of Ce3+-to-Eu2+ in Ca2PO4Cl,92 Ba3Ca2(PO4)3F10 and Ba3Y(PO4)3 (ref. 93) were once studied by our group. The overlaps between the emission spectra of Ce3+ and the excitation spectra of Eu2+, the luminescence intensity and fluorescence lifetime of Ce3+ with changing the Eu2+ doping concentration were investigated in detail. After years of study in this field, we have a deeper understanding of the luminescence performance and ET mechanism of many Ce3+–Eu2+ co-doped systems, and therefore putting forward the guiding ideology of improving the application of WLED. Despite the progress, there are still some problems to be further explored. Generally, the ET efficiency of Ce3+-to-Eu2+ is calculated based on the luminescence intensity of Ce3+ together with the formula
. Sometimes, this calculated result is in accord with the emission spectra of Ce3+ and Eu2+. For example, in the hosts Ba2MgSi2O7,63 Ba3Si6O12N2 (ref. 49) and BaMg2(PO4)2,87 their ET efficiency was calculated to be 90%, corresponding well to the emission spectra that the luminous intensity of Ce3+ decreased significantly and the luminous intensity of Eu2+ increased obviously. However, in the hosts such as LiSr4(BO3)3,72 Ba3Y(PO4)3,93 Na5Ca2Al(PO4)4,56 although the ET efficiency of Ce3+-to-Eu2+ was calculated to be 80% according to the formula
, it does not agree with the emission spectra of Eu2+ which did not increase correspondingly. Therefore, it is worth considering and exploring whether this phenomenon is related to the crystal structure and composition of host. If this problem can be solved, it may provide some clues and ideas when designing and selecting new Ce3+–Eu2+ and even other RE ions co-doped materials.
2. Subject source
So far, there have some useful reviews summarizing on the Ce3+–Eu2+ doped pc-WLED phosphors for solid-state lighting and displays such as those by Li,94 Terraschke & Wickleder,95 Xia,96,97 Qiao,98,99 Qin,100 Wang,101 Lin,102 Li103 et al. Especially, several recent reviews and articles have systematically introduced the design, the optical properties and the application of pc-WLED phosphors with the emphasis on the dependence of luminescence properties on the crystal structure and composition of host.104–135 Different from these work, here we provide a new perspective to review the recent reports. In order to find out the reason why the ET efficiency calculated according to the formula
does not match the Eu2+'s emission spectra, we have carefully investigated the crystal structure, luminescence location, quenching concentration and other related information of many hosts, as exhibited in Tables 1 and 2. As the hosts with similar crystal structure and composition have similar luminescent phenomenon, so we focus on the crystal structure of these hosts. Moreover, it was found that the actual ET efficiency of Ce3+-to-Eu2+ in the hosts with layered crystal structure is higher than those with non-layered crystal structure. Based on these results, the crystal structures of all hosts involved in this paper are then divided into two categories: layered structure and non-layered structure, in the following discussion.
Table 1 Layer spacing, crystal structure, space group, and photoluminescence properties of Ce3+–Eu2+ co-doped layered structured luminescent materials
Layered structure |
Compound |
L (Å) |
Crystal structure |
Space group |
λem (Ce3+) |
λem (Eu2+) |
Xc (Eu2+) |
Rc (Å) |
Mechanism of ET |
Ref. |
BaMg2(PO4)2 |
9.691 |
Trigonal |
P![[1 with combining macron]](https://www.rsc.org/images/entities/char_0031_0304.gif) |
412 nm |
447 nm |
0.05 |
30.7 |
d–d |
87 |
Ba1.2Ca0.8SiO4 |
7.352 |
Trigonal |
P m2 |
385 nm |
450 nm |
0.02 |
17.11 |
|
80 |
SrSi2O2N2 |
7.231 |
Trigonal |
P![[1 with combining macron]](https://www.rsc.org/images/entities/char_0031_0304.gif) |
395 nm |
530 nm |
0.02 |
26.05 |
d–d |
17 |
Ba3Si6O12N2 |
6.784 |
Trigonal |
P3 |
393 nm |
526 nm |
0.01 |
25.11 |
d–d |
49 |
Li2SrSiO4 |
5.522 |
Trigonal |
P3 |
420 nm |
570 nm |
0.01 |
27.62 |
|
20 |
Ba3Si6O9N4 |
6.784 |
Trigonal |
P3 |
420 nm |
520 nm |
0.005 |
24.86 |
d–d |
59 |
Ba2MgSi2O7 |
5.406 |
Tetragonal |
P 21m |
|
560 nm |
0.04 |
|
|
63 |
Sr2Al2SiO7 |
5.264 |
Tetragonal |
P 21m |
415 nm |
510 nm |
0.03 |
18.3 |
d–d |
57 |
Ca2Mg0.25Al1.5Si1.25O7 |
5.05 |
Tetragonal |
P 21m |
409 nm |
520 nm |
0.025 |
|
d–d |
46 |
Ca2Mg0.5AlSi1.5O7 |
5.036 |
Tetragonal |
P 21m |
410 nm |
525 nm |
0.015 |
26.71 |
d–d |
4 |
Ca8Mg3Al2Si7O28 |
5.029 |
Tetragonal |
P 21m |
|
535 nm |
0.01 |
|
|
40 |
BaSi2O2N2 |
7.592 |
Orthorhombic |
Pbcn |
390 nm |
495 nm |
0.05 |
24.87 |
d–d |
79 |
Ba2SiO4 |
7.513 |
Orthorhombic |
Pmcn |
|
505 nm |
0.03 |
|
|
39 |
Sr2SiO4 |
6.148 |
Orthorhombic |
Pmnb |
406 nm |
469 nm |
0.01 |
|
|
24 |
CaAlSiN3 |
5.636 |
Orthorhombic |
Cmc21 |
580 nm |
650 nm |
0.008 |
22.75 |
d–d |
85 |
Ba2ZnS3 |
4.21 |
Orthorhombic |
Pnam |
498 nm |
660 nm |
0.008 |
32.7 |
d–d |
77 |
Ba4Si6O16 |
6.983 |
Monoclinic |
P1211 |
442 nm |
497 nm |
≥0.025 |
|
|
66 |
LiBaBO3 |
6.416 |
Monoclinic |
P121/c1 |
430 nm |
530 nm |
0.02 |
25.06 |
|
70 |
Ca2Si5N8 |
6.991 |
Monoclinic |
C1c1 |
465 nm |
595 nm |
0.0035 |
22.27 |
d–d |
50 |
Table 2 Crystal structure, space group, and photoluminescence properties of Ce3+–Eu2+ co-doped non-layered structured luminescent materials
Non-layered structure |
Compound |
Crystal structure |
Space group |
λem (Ce3+) |
λem (Eu2+) |
Xc (Eu2+) |
Rc (Å) |
Mechanism of ET |
Lattice site of Eu2+ |
Ref. |
Ca2PO4Cl |
Orthorhombic |
Pbcm |
370 nm |
460 nm |
0.07 |
14.7 |
d–d |
Ca1 |
92 |
SrSc2O4 |
Orthorhombic |
Pnam |
535 nm |
702 nm |
0.04 |
14.498 |
d–d |
Sr |
21 |
BaSiO3 |
Orthorhombic |
P212121 |
404 nm |
570 nm |
0.02 |
|
|
Ba |
48 |
SrB2Si2O8 |
Orthorhombic |
Pnma |
373 nm |
440 nm |
0.01 |
25.82 |
d–d |
Sr |
51 |
Ca1.65Sr0.35SiO4 |
Orthorhombic |
P21cn |
465 nm |
538 nm |
|
17 |
d–d |
Sr1 to Sr6 |
88 |
Ca3MgSi2O8 |
Monoclinic |
P21/a |
400 nm |
470 nm |
0.03 |
18.64 |
d–d |
Ca |
60 |
Ca3Si2O7 |
Monoclinic |
P121/a1 |
490 nm |
625 nm |
0.0125 |
13.7 |
d–d |
Ca1 to Ca3 |
75 |
Ca4(PO4)2O |
Monoclinic |
P1121 |
460 nm |
356 nm |
0.01 |
23.8 |
d–d |
Ca1 to Ca8 |
83 |
Sr2LiSiO4F |
Monoclinic |
P21/m11 |
400 nm |
465/520 nm |
0.0075 |
|
|
Sr1 and Sr2 |
74 |
Ca2BO3Cl |
Monoclinic |
P21/c |
410 nm |
573 nm |
0.015 |
|
d–d |
Ca1 and Ca2 |
55 |
Ca3SiO4Cl2 |
Monoclinic |
P21/c |
400 nm |
505 nm |
0.006 |
|
|
Ca |
67 |
Sr5(PO4)3Cl |
Hexagonal |
P63/m |
370 nm |
443 nm |
0.1 |
21 |
d–d |
Sr2 |
65 |
La5Si2BO13 |
Hexagonal |
P63/m |
380 nm |
519 nm |
0.05 |
10.66 |
|
La1 and La2 |
54 |
Ba3Ca2(PO3)3F |
Hexagonal |
P63/m |
387 nm |
508 nm |
0.05 |
17.506 |
d–d |
M1 and M2 |
10 |
Ca4Y6O(SiO4)6 |
Hexagonal |
P63/m |
426 nm |
527 nm |
0.1 |
18.7 |
d–d |
— |
69 |
Ca9Al(PO4)7 |
Trigonal |
R3c |
356 nm |
445 nm |
0.01 |
12.64 |
d–d |
Ca |
78 |
Sr3B2O6 |
Trigonal |
R ch |
434 nm |
574 nm |
0.002 |
30.7 |
d–d |
Sr1 and Sr2 |
53 |
Ca9Y(PO4)7 |
Trigonal |
R3c |
342 nm |
493 nm |
0.005 |
30.644 |
d–d |
Ca1 to Ca3, Y |
52 |
LiSr4(BO3)3 |
Cubic |
Ia d |
423 nm |
612 nm |
0.004 |
29.14 |
d–d |
Sr1 and Sr2 |
72 |
Sr3Gd(PO4)3 |
Cubic |
I4 d |
374 nm |
518 nm |
0.01 |
32.02 |
d–d |
Sr/Gd |
62 |
Ba3Y(PO4)3 |
Cubic |
I4 d |
398 nm |
520 nm |
0.01 |
19.5 |
d–d |
Ba/La |
93 |
As shown in Fig. 1, for the hosts with layered crystal structure, high, medium and low actual ET efficiency of Ce3+-to-Eu2+ separately make up 65%, 20% and 15% of the whole cases. In contrast, high, medium and low actual ET efficiency of Ce3+-to-Eu2+ make up 31%, 24% and 45% of all the hosts with non-layered crystal structure, respectively. It is obvious that the actual ET efficiency of Ce3+-to-Eu2+ in the hosts with layered crystal structure is significantly higher than that with non-layered crystal structure.
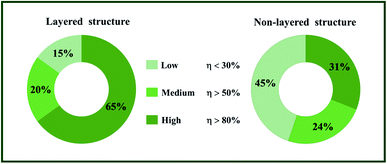 |
| Fig. 1 The proportion of the host with high, medium and low ET efficiency in the hosts with layered and non-layered crystal structure. | |
According to previous reports, in the host with layered crystal structure, the quenching concentration is relatively large, which indicates that this structure can inhibit the quenching effect of activated ions to a certain extent. For the hosts with layered crystal structure, the cation layer is separated by other anion groups or other cationic polyhedrons, thus widening the distance between the same cationic sites. According to the diagram of concentration quenching process (Fig. 2), we found that when the doping concentration of activator ions is relatively low, the average distance of activators is relatively large. Under such conditions, it is hardly any interaction between activators, so less ions forms one luminescence center that is shown in the left part of Fig. 2. With the increase of activator ions concentration, the distance between activator ions is shortened, which thus increases the probability of ET, causing the energy loss of activator ions through ET step by step, thus multiple ions will form one equivalent luminescence center that is shown in the right part of Fig. 2.6 Due to the particularity of crystal structure, the distance between the same cations in the hosts with layered crystal structure is far, therefore reducing the probability of ET of activator ions and make the emission efficiency of Eu2+ is higher.
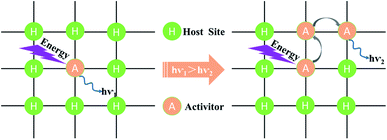 |
| Fig. 2 Diagram of concentration quenching process. | |
In order to find out the further explanation, the hosts with layered crystal structure listed above are investigated in detail. Owing to the special layered crystal structure, the same cations are far apart from each other, which effectively inhibits the concentration quenching effect of activator ions. So for the layered structure, the larger the spacing between layers (L) is, the more obvious the effect of inhibiting the concentration quenching of activator ions is. And then, the higher the doping concentration of activator ions is, the greater the luminous intensity is. Since the activator ion Eu2+ is sensitive to the changes of surrounding crystal field environment, the quenching concentration (QC) of activator ion is expected to change when changing the spacing between layers (L). The hosts with layered crystal structure can be divided into three categories according to crystal system: tetragonal, trigonal and orthorhombic (Fig. 3a–c). All the hosts listed in Fig. 3a are tetragonal with space group P
21m, those listed in Fig. 3b are trigonal with space group P
or P
, and the hosts listed in Fig. 3c are all orthorhombic. The insets in Fig. 3a and b are the crystal structure diagrams of hosts seen from different directions. In Fig. 3c, although the hosts are all layered structures, they can be divided into the layered structures with and without connecting bonds due to different space groups. It can be seen from Fig. 3 that the QC of Eu2+ increases with the increase of spacing between layers (L) in the same crystal system. It is further proved that with the increase of distance between the cationic sites occupied by activator ions, the effect of inhibiting the concentration quenching of activator ion becomes greater. Therefore, the doping concentration of activator ions becomes higher and the luminescence property of phosphors becomes better.
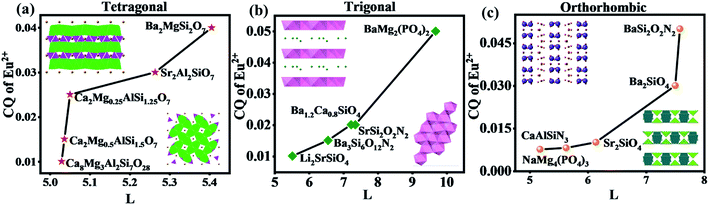 |
| Fig. 3 The relationship between the spacing between layers (L) and the QC of Eu2+ in (a) tetragonal, (b) trigonal and (c) orthorhombic host. | |
It should be noticed, however, that the luminescence properties of some hosts with non-layered structure are also excellent, which can be explained as follows. Some of the hosts with non-layered structure are separated by the surrounding anionic groups, while others have gaps between the same cations by means of the selective occupancy of activator ions, which is called the interstitial structure. This structure can also indirectly increase the distance between the same cationic sites, followed by the inhabitation of the quenching effect of activator ions and the increase of doping concentration of activator ions. As shown in Fig. 4, the 21 hosts listed can be divided into five categories according to their crystal systems: orthorhombic, hexagonal, cubic, monoclinic and trigonal. And the proportions of these five crystal systems are 23.81%, 19.05%, 14.29%, 28.57% and 14.29%, respectively. In Fig. 4, different colors represent different crystal systems. For the hosts within the same crystal system, the unshadowed portion represents the host with interstitial structure, while the shadowed portion represents the host whose crystal lattice is occupied by activator ions that is tightly connected (we call it the cluster structure). It can be seen from the diagram that the QC of activator ions in the interstitial structure is higher than that in the cluster structure for all crystal systems. This further demonstrates that the larger the distance between the cationic sites occupied by the activator ions is, the greater the effect of inhibiting the concentration quenching of activator ions is. And then, the greater the doping concentration of activator ions is, the better the luminescence property of phosphors is.
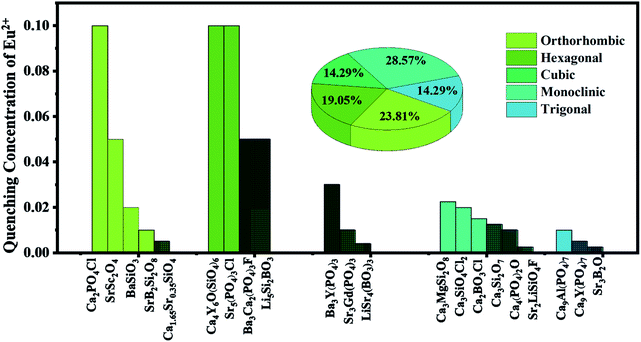 |
| Fig. 4 Effect of selective occupancy of activator ions on the QC of Eu2+ in non-layered hosts. | |
As is well known, the emitting intensity of activator ions will be weak if the doping concentration is quite small. Therefore, inhibiting the concentration quenching of activator ions can increase the doping concentration of activator ions, thus enhancing the intensity of luminescence. Based on this conclusion, we found that the design of layered crystal structure and the selective occupation of activator ions can inhibit the concentration quenching of activator ions and improve the luminescent properties of Ce3+–Eu2+ co-doped phosphors to some extent. Next, we will take some reported hosts as an example for specific analysis.
3. Effects of crystal structure on luminescence properties of Ce3+–Eu2+ co-doped phosphors
3.1 Improving luminescence performance of Ce3+–Eu2+ co-doped phosphors by designing selective occupancy of activator ions
A large amount of Ce3+, Eu2+ singly-doped and Ce3+–Eu2+ co-doped phosphors have been reported. According to these reports, Ce3+ and Eu2+ are sensitive to the changes of surrounding crystal field environment. Specifically, their luminescence location, spectral range, quenching concentration and thermal stability will be different with the variations of crystal field environment. Therefore, it is a common way to adjust the luminescent properties of phosphors by changing the crystal field environment around activator ions. In particular, the method of improving the luminescent properties of phosphors by designing the occupation sites of activator ions has been intensively studied.127–135
Since the luminescent properties of Ce3+ or Eu2+-singly doped phosphors can be improved by the method of activator ion selective occupation, the luminescence properties of Ce3+–Eu2+ co-doped phosphors is also expected to be improved using the same way. Currently, a large number of Ce3+–Eu2+ co-activated halogenated phosphate hosts have been found. Ba3Ca2(PO4)3F10 and Sr5(PO4)3Cl65 are two representatives, and both of them belong to the apatite structure system. As shown in Fig. 5a and d, the crystal structure of them belongs to the hexagonal system with P63/m space group. There are two kinds of cationic sites in Ba3Ca2(PO4)3F, which are signed as M1(Ca1/Ba1) and M2(Ca2/Ba2) to facilitate the following discussion. The M1 sites are surrounded by nine oxygen anions with local symmetry C3, forming a tricapped trigonal prism. The M2 sites are seven-fold coordinate surrounded by six oxygen anions and one fluorine anion with Cs point group symmetry. While in Sr5(PO4)3Cl, the cationic sites are signed as Sr1 and Sr2. From Fig. 5a and d we can see that each Sr1/M1 sites is surrounded by anionic polyhedron and Sr2/M2, every three Sr2/M2 polyhedrons form a cluster with F− ion through the vertex angle, and each cluster is separated by Sr1/M1 polyhedron and anionic polyhedron.
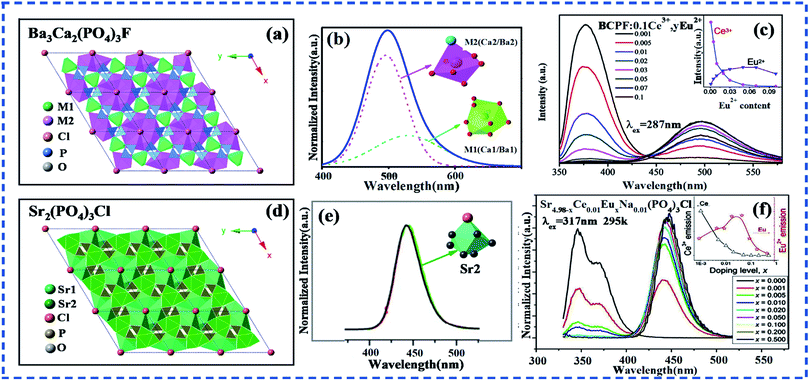 |
| Fig. 5 (a) The crystal structure of Ba3Ca2(PO4)3F. (b) The PL spectra of Ba3Ca2(PO4)3F:Eu2+ phosphors (c) the PL spectra of a series of Ba3Ca2(PO4)3F:0.1Ce3+,xEu2+ phosphors with different Eu2+ concentrations excited at 287 nm. The inset shows the emission intensity of Ce3+ and Eu2+ with the increase of Eu2+ concentration in Ba3Ca2(PO4)3F phosphors. (d) The crystal structure of Sr5(PO4)3Cl. (e) The PL spectra of Sr5(PO4)3Cl:Eu2+ phosphors. (f) The PL spectra of a series of Sr5(PO4)3Cl:0.01Ce3+,xEu2+ phosphors with different Eu2+ concentrations excited at 317 nm. The inset shows the emission intensity of Ce3+ and Eu2+ with the increase of Eu2+ concentration in Sr5(PO4)3Cl phosphors. (Reproduced with permission from ref. 10 and 65, copyright 2013, 2017, J. Mater. Chem. C, J. Alloys Compd.) | |
It can be seen from the structure diagram that if the doped RE ions occupy both lattice sites, the distance between activator ions is likely to be smaller, which increases the possibility of concentration quenching as discussed above. As shown in Fig. 5b and e, the emission spectrum of Ba3Ca2(PO4)3F:Eu2+ contains asymmetrically broad green-emission band from 400 to 650 nm, which is ascribed to the two different sites of Eu2+.10 According to the previous analysis of crystal structure, this spectrum can be decomposed well into two Gaussian bands with peaks centering at 498 and 534 nm, respectively. The Eu2(M2) site will lead to a shorter wavelength band due to the presence of F− anion, so the 498 nm emission comes from the Eu2(M2) site and the 534 nm emission comes from the Eu1(M1) site.10 In contrast, in the host Sr5(PO4)3Cl, the emission spectrum of Eu2+ shows a symmetrically narrow emission band from 400 to 500 nm, which indicates that Eu2+ is likely to enter only one of the cation sites and therefore indirectly pull the distance between the same lattices, making the same cationic lattices farther apart and reducing the probability of ET between activator ions.65
According to the above inference, the doping concentration of Eu2+ in Sr5(PO4)3Cl is higher than that in Ba3Ca2(PO4)3F. It can be seen from Fig. 5c and f that in Sr5(PO4)3Cl, with the increase of Eu2+ doping concentration, the luminescence intensity of Ce3+ decreases greatly, while the luminescence intensity of Eu2+ increases sharply. When the doping concentration of Eu2+ reaches x = 0.5, the emission intensity of Eu2+ still does not show a downward trend.65 In contrast, the emission intensity of Ce3+ in Ba3Ca2(PO4)3F decreases with the increase of Eu2+ doping concentration, and the emission intensity of Eu2+ increases first and then decreases with the maximum at x = 0.05.10 Therefore, no matter in terms of the QC of Eu2+ or the actual ET efficiency of Ce3+-to-Eu2+, Sr5(PO4)3Cl is superior to Ba3Ca2(PO4)3F. This result proves that the previous inference is correct. The distance between cation lattices does affect the quenching concentration of Eu2+ and the ET efficiency of Ce3+-to-Eu2+. When the cation lattices are far apart, the ET efficiency of Ce3+-to-Eu2+ is relatively high. It is because the probability of ET between the same activator ions is reduced and the concentration quenching effect between activator ions is restricted to some degree.
The layered structure sometimes can be formed by selective occupation of activator ions in several hosts, which thus improves the luminescent properties of phosphors to a certain extent. It will be shown using the crystal structures of Ca2PO4Cl92 and Ca2BO3Cl55 as given in Fig. 6a and d. The Ca2PO4Cl crystallizes in the orthorhombic system with space group of Pbcm, and the crystal structure of Ca2BO3Cl belongs to the monoclinic system with space group of P21/c. There are two kinds of cationic sites (Ca1 and Ca2 sites) in Ca2PO4Cl and Ca2BO3Cl, as shown in Fig. 6a and d. The Ca1 and Ca2 sites of Ca2PO4Cl are arranged in layers, while in Ca2BO3Cl, the Ca1 and Ca2 sites are staggered. If the doped Eu2+ enter only one of the lattice sites, the far distance between the lattice sites will lower the probability of ET between activator ions, so the concentration quenching of Eu2+ does not occur easily when the doped concentration is low.
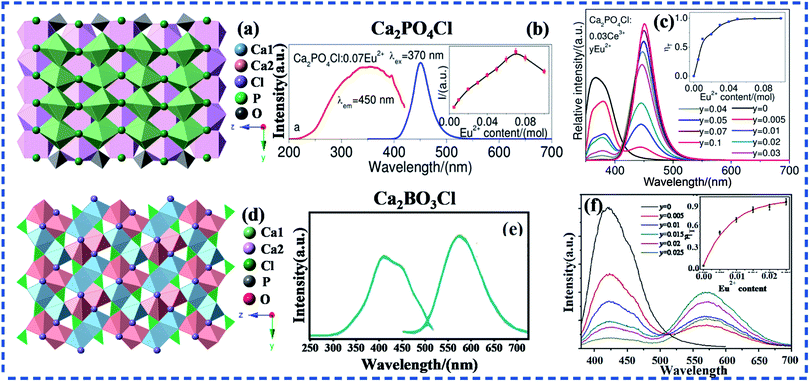 |
| Fig. 6 (a) The crystal structure of Ca2PO4Cl. (b) The PL spectra of Ca2PO4Cl:Eu2+ phosphors. (c) The PL spectra of a series of Ca2PO4Cl:0.03Ce3+,yEu2+ phosphors with different Eu2+ concentrations excited at 370 nm. The inset shows the ET efficiency as a function of Eu2+ concentration in Ca2PO4Cl phosphors. (d) The crystal structure of Ca2BO3Cl. (e) The PL spectra of Ca2BO3Cl:Eu2+ phosphors. (f) The PL spectra of a series of Ca2BO3Cl:0.006Ce3+,yEu2+ phosphors with different Eu2+ concentrations excited at 320 nm. (Reproduced with permission from ref. 92 and 55, copyright 2014, 2019, RSC Adv., J. Mater. Sci.: Mater. Electron.) | |
It can be seen from Fig. 6b that the emission spectrum of Ca2PO4Cl:Eu2+ ranges from 400 to 530 nm under excitation at 370 nm, and the spectral profile of this emission are symmetrical with a relatively narrow full width at half maximum.92 Thus, it is very likely that Eu2+ occupies only one of the cationic lattices after entering the host, and the luminescence intensity of Eu2+ does not quench when the doping concentration is y = 0.1, as can be seen from Fig. 6c.92 This further confirms that Ce3+–Eu2+ system has relatively high ET efficiency due to the relatively high QC of Eu2+ caused by the large distance between cationic lattices. However, the emission spectrum of Ca2BO3Cl:Eu2+ contains asymmetrically broad emission band from 450 to 700 nm, which is ascribed to the two different sites of Eu2+.55 It can be seen from the structure diagram (Fig. 6d) that if the doped RE ions occupy both lattice sites, the distance between activator ions will be closer when the concentration of activator ions doped becomes larger. The energy will gradually dissipate due to the ET between different lattice sites and the same lattice sites, so the activator ion quenches when the doping concentration is low. In Ca2BO3Cl, the luminous intensity of Ce3+ decreased with the increase of Eu2+, and the luminous intensity of Eu2+ increased first and then decreased with its maximum at x = 0.015.55 Therefore, no matter in terms of the QC of Eu2+ or the actual ET efficiency from Ce3+ to Eu2+, Ca2PO4Cl is superior to Ca2BO3Cl. This further shows the luminescence properties of phosphors can be improved by selective occupancy of activator ions.
3.2 Improving the luminescence properties of Ce3+–Eu2+ co-doped phosphors by designing layered crystal structure
In addition to the above mentioned way of elective occupation of activator ions, choosing the host with layered crystal structure is also a method to improve the luminescence performance of phosphors. In recent years, many Ce3+–Eu2+ co-doped hosts with layered structure have been reported, among which BaMg2(PO4)2 (ref. 87) and Ba2Mg(BO3)2 (ref. 86) are two representatives. Although the two hosts are both layered structure, the crystal structures, the composition of cationic layers, and the distances between layers are all different. As can be seen from Fig. 7c and d, the luminous intensity of Ce3+ decreased with increasing Eu2+ doping concentration, while the luminous intensity of Eu2+ increased first and then decreased. Moreover, the actual ET efficiency from Ce3+ to Eu2+ in BaMg2(PO4)2 is significantly higher than that in Ba2Mg(BO3)2.86,87 Therefore, it is reasonable to speculate that different distances between cationic layers cause different inhibition effects on the quenching of activator ions and thus different actual ET efficiency from Ce3+ to Eu2+.
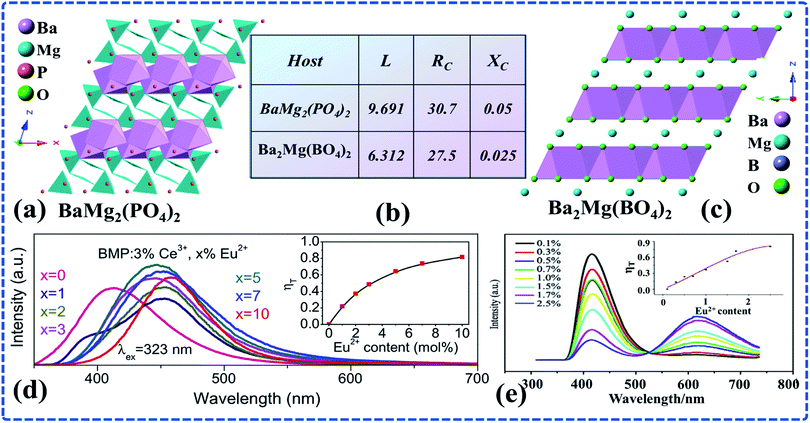 |
| Fig. 7 (a) The crystal structure of BaMg2(PO4)2. (b) The spacing between layers (L), critical distance (Rc) and quenching concentration (Xc) of BaMg2(PO4)2 and Ba2Mg(BO3)2. (c) The crystal structure of Ba2Mg(BO3)2. (d) The photoluminescence emission (PL) spectra of a series of BaMg2(PO4)2:3%Ce3+,x%Eu2+ phosphors with different Eu2+ concentrations excited at 323 nm. The inset shows the ET efficiency as a function of Eu2+ concentration in BaMg2(PO4)2 phosphors. (e) PL spectra of a series of Ba2Mg(BO3)2:2%Ce3+,x%Eu2+,2%Na+ phosphors with different Eu2+ concentrations excited at 296 nm. (Reproduced with permission from ref. 87 and 86, copyright 2014, 2012, J. Alloys Compd., J. Rare Earths.) | |
In fact, in addition to the above reasons, the critical distance between different hosts also affects QC effect. As shown in Fig. 7b, the distance between the cationic layers (Ba ions and Ba ions) in BaMg2(PO4)2 is 9.691 Å, and the critical distance of ET from Ce3+ to Eu2+ system is 30.7 Å. When the doping concentration of Eu2+ is x = 0.05, the luminescence intensity of Eu2+ reaches its maximum.87 In contrast, the distance between the cationic layers (Ba ions and Ba ions) in Ba2Mg(BO3)2 is 6.312 Å, and the critical distance between Ce3+ and Eu2+ is 27.5 Å. When the doping concentration of Eu2+ is x = 0.025, the luminescence intensity of Eu2+ reaches its maximum.86 It is obvious that BaMg2(PO4)2 is better than Ba2Mg(BO3) in terms of the distance between cationic layers, the critical distance between Ce3+ and Eu2+ and the QC of Eu2+. In addition, the actual ET efficiency from Ce3+ to Eu2+ in BaMg2(PO4)2 is significantly higher than that in Ba2Mg(BO3)2. It further indicates that for the hosts with similar composition and crystal structure, a greater distance between layers can lower the ET probability caused by the close distance between the layers of the same activated ions, thus inhibiting the concentration quenching of activator ions and leading to higher doping concentration of activator ions. In addition, large critical distance between Ce3+ and Eu2+ ensures that the doping concentration of activator ions can be effectively increased in the range where the ET from Ce3+ to Eu2+ can happen, thus making the actual ET efficiency from Ce3+ to Eu2+ of BaMg2(PO4)2 relatively high.
Although it has been mentioned above that the actual ET efficiency from Ce3+ to Eu2+ is relatively high in the hosts with layered crystal structure, it does not mean the hosts with layered structure always ensure a high actual ET efficiency of Ce3+-to-Eu2+. For example, the crystal structures of Ca2Mg0.25Al1.5Si1.25O7 (ref. 46) and Ca2Mg0.5Al1.5Si1.5O7 (ref. 4) as shown in Fig. 8a and b are all layered structures. It can be seen from the emission spectra presented in Fig. 8c and d that the luminous intensity of Ce3+ decreased greatly with the increase of Eu2+ doping concentration, whereas the Eu2+ emission only showed a slight increase, and the total emission intensity decreased. Obviously, the ET efficiency calculated according to the formula
does not match the spectra.
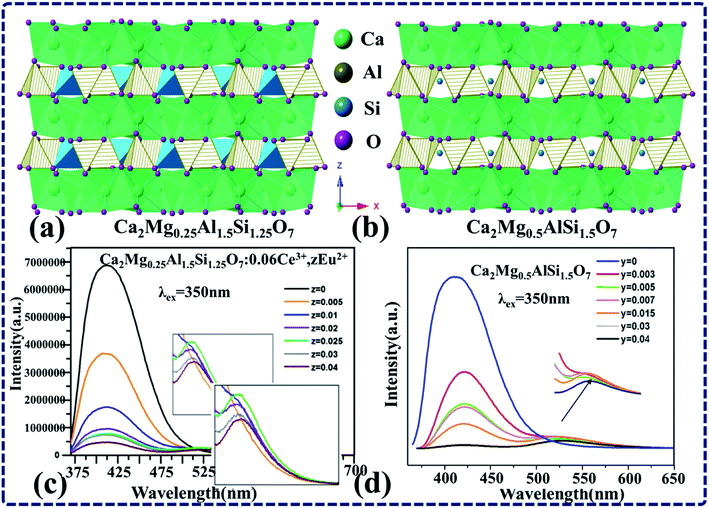 |
| Fig. 8 (a) and (b) The crystal structure of Ca2Mg0.25Al1.5Si1.25O7 and Ca2Mg0.5Al1.5Si1.5O7. (c) The PL spectra of a series of Ca2Mg0.25Al1.5Si1.25O7:0.06Ce3+,yEu2+ with different Eu2+ concentrations excited at 350 nm. (d) PL spectra of a series of Ca2Mg0.5Al1.5Si1.5O7:0.06Ce3+,zEu2+ phosphors with different Eu2+ concentrations excited at 350 nm. (Reproduced with permission from ref. 46 and 4, copyright 2014, 2016, Powder Technol. Lumin.) | |
In order to clarify this problem, we analyzed the crystal structure and the information obtained from the reported literatures, concluding that the crystal structure of these two hosts is layered structure. Moreover, the distances between each two layers of Ca2Mg0.25Al1.5Si1.25O7 (ref. 46) and Ca2Mg0.5Al1.5Si1.5O7 (ref. 4) are separately 5.05 Å and 5.036 Å, the smallest distances of the layered structure hosts with connective bonds listed in this paper. Based on the previous discussion, this may be due to the fact that the distance between cationic layers is smaller and the distance between the same cation lattices is closer, thus increasing the probability of ET between activator ions. Therefore, the emission of Eu2+ is quenched at low doping concentration. As shown in Fig. 8c and d, the luminescence intensity of Eu2+ decreased with increasing doping concentration, which further verifies that even if the crystal structure of a host with high calculated ET efficiency from Ce3+ to Eu2+ is layered, its actual ET efficiency might be relatively low due to the small distance between layers. Therefore, not every host with layered crystal structure has good luminescence performance. When the spacing between layers is small, the distance between the same cations will be closer, thus increasing the probability of concentration quenching of activator ions. Therefore, the hosts with layered crystal structure and large spacing between layers are highly recommended when designing new phosphors in the future.
4. Summary and outlook
To sum up, the effect of crystal structure on the luminescence properties of phosphors is reviewed in this paper. It is found that the actual ET efficiency from Ce3+ to Eu2+ in the hosts with layered structure is higher than that with non-layered structure. It is because in these hosts with layered structure, the same cation lattice is far apart due to the special crystal structure, thus reducing the probability of ET caused by the close distance between activator ions and making the quenching concentration of activator ions relatively high. Therefore, the actual ET efficiency from Ce3+ to Eu2+ in these hosts is relatively high. Although the luminescence performance of the hosts with layered crystal structure is excellent, the ET efficiency from Ce3+ to Eu2+ in some hosts such as Ca2Mg0.25Al1.5Si1.25O7 (ref. 46) and Ca2Mg0.5Al1.5Si1.5O7 (ref. 4) is, however, not so ideal. It is because the spacing between layers is small, and the distance between the cation sites is close, which increases the probability of ET of activator ions and leads to concentration quenching when the doping concentration is small. In addition, it is also found that for some non-layered matrices, the luminescent properties can also be improved by the method of selective occupation of activator ions. By using this method, the crystal structure of hosts can be changed from cluster structure to layered or interstitial structure, which indirectly increases the distance between the same cation sites, restrains the activator ion concentration quenching, and make the emission efficiency of Eu2+ is higher, the luminous performance of phosphor material is better.
Over the past decades, great progress has been made in the field of WLED, especially the single-phase WLED excited by the near-ultraviolet radiation. Since the positions of the excited 4f65d1 state relative to the ground state are strongly influenced by host lattice, the position of emission band depends strongly on the environmental conditions of Eu2+ and Ce3+ such as coordination number, bond length, crystal field splitting, the nephelauxetic effect, the Stokes shift, the distortion of host lattice and so forth. It thus varies between the near ultraviolet and near infrared spectral range. These works have been reviewed a lot, but there is no general rule applicable to all phosphor systems at present. Exploring the structure-related luminescence mechanism is still the focus of future research.
Although a particular emphasis is placed on Ce3+–Eu2+ co-doped phosphors, we believe these theoretical approaches should be readily expandable to other lanthanide-doped systems with the characteristics of 4f–5d optical transitions, such as Ce3+–Mn2+ and Eu2+–Mn2+ co-doped systems. In addition, in some Ce3+–Eu2+ co-doped phosphor, the crystal structure of host not only affects the QC of activator ions and the ET efficiency from Ce3+ to Eu2+, but also affects the luminous color, thermal stability and quantum efficiency of phosphors. Therefore, these issues are also worthy of exploration, in order to meet different market demand for different performance LED.
It is worth noting that although the design of layered crystal structure and the selective occupation of activator ions can improve the luminescent properties of Ce3+–Eu2+ co-doped phosphors, these rules do not apply to all Ce3+–Eu2+ co-doped materials. The luminescence properties of phosphors are related not only to the crystal structure of host, but also to the overlapping degree of excitation spectrum of activator and emission spectra of sensitizer, synthesizing environment of materials, doping concentration of sensitizer and the critical distance between Ce3+ and Eu2+. In conclusion, despite the many contributions to the development of Ce3+–Eu2+ co-doped phosphors in recent years, there are still some open questions that need to make further study in the future.
Conflicts of interest
The authors declare no competing financial interest.
Acknowledgements
The work is supported by the National Natural Science Foundation of China (Nos 51672066, 51902080), the Funds for Distinguished Young Scientists of Hebei Province, China (No. A2018201101), and the Natural Science Foundation of Hebei Province, China (No. E2019201223), the personnel training project of Hebei Province, China (No. A201902005).
References
-
(a) J. H. Oh, S. J. Yang and Y. R. Do, Light: Sci. Appl., 2014, 3, e141 CrossRef CAS;
(b) H. Zhu, C. C. Lin, W. Luo, S. Shu, Z. Liu, Y. Liu, J. Kong, E. Ma, Y. Cao, R.-S. Liu and X. Chen, Nat. Commun., 2014, 5, 4312 CrossRef CAS PubMed;
(c) T. Pulli, T. Donsberg, T. Poikonen, F. Manoocheri, P. Karha and E. Ikonen, Light: Sci. Appl., 2015, 4, e332 CrossRef CAS.
- P. Li, Z. Wang, Z. Yang and Q. Guo, A novel, warm, white light-emitting phosphor Ca2PO4Cl: Eu2+, Mn2+ for white LEDs, J. Mater. Chem. C, 2014, 2, 7823–7829 RSC.
- S. Liang, P. Dang, G. Li, M. S. Molokeev, Y. Wei, Y. Wei, H. Lian, M. Shang, A. A. Al Kheraiff and J. Lin, Controllable two-dimensional luminescence tuning in Eu2+, Mn2+ doped (Ca,Sr)9Sc(PO4)7 based on crystal field regulation and energy transfer, J. Mater. Chem. C, 2018, 6, 6714–6725 RSC.
- B. Yuan, Y. Song, Y. Sheng, K. Zheng, Q. Huo, X. Xu and H. Zou, Luminescence and energy transfer properties of color-tunable Ca2Mg0.25Al1.5Si1.25O7: Ce3+/Eu2+/Tb3+ phosphors for ultraviolet light-emitting diodes, Luminescence, 2016, 31(2), 453–461 CrossRef CAS PubMed.
- C. Guo, X. Ding, L. Luan and Y. Xu, Two-color emitting of Eu2+ and Mn2+ co-doped Sr2Mg3P4O15 for UV LEDs, Sens. Actuators, B, 2010, 143(2), 712–715 CrossRef CAS.
- C. Wang, P. Li, Z. Wang, Y. Sun, J. Cheng, Z. Li, M. Tian and Z. Yang, Crystal structure, luminescence properties, energy transfer and thermal properties of a novel color-tunable, white light-emitting phosphor Ca9−x−yCe(PO4)7: xEu2+, yMn2+, Phys. Chem. Chem. Phys., 2016, 18, 28661–28673 RSC.
- C. Guo, X. Ding, H. Seo, Z. Ren and J. Bai, Double emitting phosphor NaSr4(BO3)3: Ce3+, Tb3+ for near-UV light-emitting diodes, Opt. Laser Technol., 2011, 43(7), 1351–1354 CrossRef CAS.
- N. Zhang, C. Guo, H. Jing and J. H. Jeong, Color tunable emission in Ce3+ and Tb3+ co-doped Ba2Ln(BO3)2Cl (Ln = Gd and Y) phosphors for white light-emitting diodes, Spectrochim. Acta, Part A, 2013, 116, 556–561 CrossRef CAS.
- W. Tang and C. Ding, Luminescence Tuning of Sr8MgCe(PO4)7: Eu2+, Mn2+ Phosphors: Structure Refinement, Site Occupancy, and Energy Transfer, Zeitschrift für anorganische und allgemeine Chemie, 2018, 644(16), 893–900 CrossRef CAS.
- X. Meng, K. Qiu, Z. Tian, X. Shi, J. You, Z. Wang, P. Li and Z. Yang, Tunable-emission single-phase phosphors Ba3Ca2(PO4)3F:M (M = Ce3+, Eu2+, Mn2+): crystal structure, luminescence and energy transfer, J. Alloys Compd., 2017, 719, 322–330 CrossRef CAS.
- J. Yu, C. Guo, Z. Ren and J. Bai, Photoluminescence of double-color-emitting phosphor Ca5(PO4)3Cl: Eu2+, Mn2+ for near-UV LED, Opt. Laser Technol., 2011, 43(4), 762–766 CrossRef CAS.
- C. Wang, Z. Wang, P. Li, J. Cheng, Z. Li, M. Tian, Y. Sun and Z. Yang, Relationships between luminescence properties and polyhedron distortion in Ca9−x−y−zMgxSryBazCe(PO4)7: Eu2+, Mn2+, J. Mater. Chem. C, 2017, 5, 10839–10846 RSC.
- L. Zhang, Z. Wang, C. Liu, G. Dong, D. Dai, Z. Xing, X. Li, Z. Yang and P. Li, Tunable emitting phosphor Ca6Ce2Na2(PO4)6F2: Mn2+/Tb3+ for white LEDs: luminescence, energy transfer and high thermal property, J. Lumin., 2020, 217, 116817 CrossRef CAS.
- Q. Bao, Z. Wang, J. Sun, Z. Wang, X. Meng, K. Qiu, Y. Chen, Z. Yang and P. Li, Crystal structure, luminescence properties, energy transfer, tunable occupation and thermal properties of a novel color-tunable phosphor NaBa1−zSrzB9O15: xCe3+, yMn2+, Dalton Trans., 2018, 47, 13913–13925 RSC.
- J. Qiao, G. Zhou, Y. Zhou, Q. Zhang and Z. Xia, Divalent europium-doped near-infrared-emitting phosphor for light-emitting diodes, Nat. Commun., 2019, 10(1), 5267 CrossRef PubMed.
- M. Zhang, Y. Liang, R. Tang, D. Yu, M. Tong, Q. Wang, Y. Zhu, X. Wu and G. Li, Highly Efficient Sr3Y2(Si3O9)2: Ce3+, Tb3+/Mn2+/Eu2+ Phosphors for White LEDs: Structure Refinement, Color Tuning and Energy Transfer, RSC Adv., 2014, 4(76), 40626–40637 RSC.
- R. Liu, Y. Liu, N. C. Bagkar and S. Hu, Enhanced luminescence of SrSi2O2N2: Eu2+ phosphors by co-doping with Ce3+, Mn2+, and Dy3+ ions, Appl. Phys. Lett., 2007, 91(6), 061119–061119-3 CrossRef.
- X. Jiang, Y. Zhang and J. Zhang, White-emission in single-phase Ba2Gd2Si4O13: Ce3+, Eu2+, Sm3+ phosphor for white-LEDs, Spectrochim. Acta, Part A, 2018, 192, 194–201 CrossRef CAS PubMed.
- B. Cui, Z. Chen, Q. Zhang, H. Wang and Y. Li, A single-composition CaSi2O2N2:RE (RE = Ce3+/Tb3+, Eu2+, Mn2+) phosphor nanofiber mat: energy transfer, luminescence and tunable color properties, J. Solid State Chem., 2017, 253, 263–269 CrossRef CAS.
- Z. Wei, Y. Wang, X. Zhu, J. Guan, W. Mao and J. Song, Solid state synthesis and tunable luminescence of Li2SrSiO4: Eu2+/Ce3+ phosphors, Chem. Phys. Lett., 2016, 648, 8–12 CrossRef CAS.
- J. Zhao, X. Sun and Z. Wang, Ce3+/Eu2+ doped SrSc2O4 phosphors: synthesis, luminescence and energy transfer from Ce3+ to Eu2+, Chem. Phys. Lett., 2018, 691, 68–72 CrossRef CAS.
- W. Lv, J. Huo, Y. Feng, S. Zhao and H. You, Photoluminescence, energy transfer and tunable color of Ce3+, Tb3+ and Eu2+ activated oxynitrides phosphor with high brightness, Dalton Trans., 2016, 45(23), 9676–9683 RSC.
- J. Ha, Z. Wang, E. Novitskaya, G. A. Hirata, O. A. Graeve, S. P. Ong and J. McKittrick, An Integrated First Principles and Experimental Investigation of the Relationship between Structural Rigidity and Quantum Efficiency in Phosphors for Solid State Lighting, J. Lumin., 2016, 179, 297–305 CrossRef CAS.
- C. Guo, Y. Xu, F. Lv and X. Ding, Luminescent properties of Sr2SiO4: Eu2+ nanorods for near-UV white LED, J. Alloys Compd., 2010, 497(1–2), L21–L24 CAS.
- G. Li, Y. Fan, H. Guo and Y. Wang, Synthesis, structure and photoluminescence properties of Ce3+-doped SrSc2O4: a new scandate green-emitting phosphor with blue excitation, New J. Chem., 2017, 41, 5565–5571 RSC.
- C. Guo, M. Li, Y. Xu, T. Li, Z. Ren and J. Bai, A potential green-emitting phosphor Ca8Mg(SiO4)4Cl2: Eu2+ for white light emitting diodes prepared by sol–gel method, Appl. Surf. Sci., 2011, 257(21), 8836–8839 CrossRef CAS.
- G. Li, Y. Zhao, Y. Wei, Y. Tian, Z. Quan and J. Lin, Novel yellowish-green light-emitting Ca10(PO4)6O: Ce3+ phosphor: structural refinement, preferential site occupancy and color tuning, Chem. Commun., 2016, 52, 3376–3379 RSC.
- J. Xiang, J. Zheng, Z. Zhou, H. Suo, X. Zhao, X. Zhou, N. Zhang, M. S. Molokeev and C. Guo, Enhancement of red emission and site analysis in Eu2+ doped new-type structure Ba3CaK(PO4)3 for plant growth white LEDs, Chem. Eng. J., 2019, 356, 236–244 CrossRef CAS.
- K. Uheda, N. Hirosaki, Y. Yamamoto, A. Naito, T. Nakajima and H. Yamamoto, Luminescence Properties of a Red Phosphor, CaAlSiN3: Eu2+, for White Light-Emitting Diodes, Electrochem. Solid-State Lett., 2006, 9, H22–H25 CrossRef CAS.
- P. Li, Z. Wang, Z. Yang and Q. Guo, Ba2B2O5: Ce3+: a novel blue emitting phosphor for white LEDs, Mater. Res. Bull., 2014, 60, 679–681 CrossRef CAS.
- Q. Wu, Z. Yang, Z. Zhao, M. Que, X. Wang and Y. Wang, Synthesis, crystal structure and luminescence properties of a Y4Si2O7N2: Ce3+ phosphor for near-UV white LEDs, J. Mater. Chem. C, 2014, 2, 4967–4973 RSC.
- R. Yu, C. Guo, T. Li and Y. Xu, Preparation and luminescence of blue-emitting phosphor Ca2PO4Cl: Eu2+ for n-UV white LEDs, Curr. Appl. Phys., 2013, 13(5), 880–884 CrossRef.
- W. Geng, X. Zhou, J. Ding, G. Li and Y. Wang, K7Ca9[Si2O7]4F: Ce3+: a novel blue-emitting phosphor with good thermal stability for ultraviolet-excited light emitting diodes, J. Mater. Chem. C, 2017, 5, 11605–11613 RSC.
- X. Ding and Y. Wang, Structure and photoluminescence properties of a novel apatite green phosphor Ba5(PO4)2SiO4: Eu2+ excited by NUV light, Phys. Chem. Chem. Phys., 2017, 19, 2449–2458 RSC.
- X. Wang, Z. Zhao, Q. Wu, C. Wang, Q. Wang, Y. Li and Y. Wang, Structure, photoluminescence and abnormal thermal quenching behavior of Eu2+-doped Na3Sc2(PO4)3: a novel blue-emitting phosphor for n-UV LEDs, J. Mater. Chem. C, 2016, 4, 8795–8801 RSC.
- X. Wang, Z. Zhao, Q. Wu, Y. Li and Y. Wang, Synthesis, structure and photoluminescence properties of Ca2LuHf2(AlO4)3: Ce3+, a novel garnet-based cyan light-emitting phosphor, J. Mater. Chem. C, 2016, 4, 11396–11403 RSC.
- Y. Liu, J. Zhang, C. Zhang, J. Xu, G. Liu, J. Jiang and H. Jiang, Ba9Lu2Si6O24: Ce3+: An Efficient Green Phosphor with High Thermal and Radiation Stability for Solid-State Lighting, Adv. Opt. Mater., 2015, 3(8), 1096–1101 CrossRef CAS.
- Z. Wang, B. Yang, P. Li, Z. Yang and Q. Guo, Energy transfer between activators at different crystallographic sites in Sr3SiO5: Eu2+, Phys. B, 2012, 407(8), 1282–1286 CrossRef CAS.
- M. Zhang, J. Wang, Q. Zhang, W. Ding and Q. Su, Optical properties of Ba2SiO4: Eu2+ phosphor for green light-emitting diode (LED), Mater. Res. Bull., 2007, 42, 33–39 CrossRef CAS.
- W. Lv, Y. Jia, Q. Zhao, M. Jiao, B. Shao, W. Lü and H. You, Crystal Structure and Luminescence Properties of Ca8Mg3Al2Si7O28: Eu2+ for WLEDs, Adv. Opt. Mater., 2014, 2, 183–188 CrossRef.
- M. Zhao, Z. Xia, M. S. Molokeev, L. Ning and Q. Liu, Temperature and Eu2+ Doping Induced Phase Selection in NaAlSiO4 Polymorphs and the Controlled Yellow/Blue Emission, Chem. Mater., 2017, 29, 6552–6559 CrossRef CAS.
- Z. Xia, R. Liu, K. Huang and V. Drozd, Ca2Al3O6F: Eu2+: a green-emitting oxyfluoride phosphor for white light-emitting diodes, J. Mater. Chem., 2012, 22, 15183–15189 RSC.
- J. Qiao, L. Ning, M. S. Molokeev, Y. Chuang, Q. Liu and Z. Xia, Eu2+ Site Preferences in the Mixed Cation K2BaCa(PO4)2 and Thermally Stable Luminescence, J. Am. Chem. Soc., 2018, 140(30), 9730–9736 CrossRef CAS PubMed.
- W. Sun, Y. Jia, R. Pang, H. Li, T. Ma, D. Li, J. Fu, S. Zhang, L. Jiang and C. Li, Sr9Mg1.5(PO4)7: Eu2+: a novel broadband orange-yellow-emitting phosphor for blue light-excited warm white LEDs, ACS Appl. Mater. Interfaces, 2015, 7(45), 25219–25226 CrossRef CAS PubMed.
- D. Zhao, S.-R. Zhang, Y.-P. Fan, B.-Z. Liu and R.-J. Zhang, Thermally Stable Phosphor KBa2(PO3)5: Eu2+ with Broad-Band Cyan Emission Caused by Multisite Occupancy of Eu2+, Inorg. Chem., 2020, 59, 8789–8799 CrossRef CAS PubMed.
- B. Yuan, Y. Song, Y. Sheng, K. Zheng, Q. Huo, X. Xu and H. Zou, Luminescence properties and energy transfer of Ca2Mg0.5AlSi1.5O7:Ce3+, Eu2+ phosphors for UV-excited white LEDs, Powder Technol., 2014, 253, 803–808 CrossRef CAS.
- C. Ding and W. Tang, Crystal structure, energy transfer and tunable luminescence properties of Ca8ZnCe(PO4)7: Eu2+, Mn2+ phosphor, Opt. Mater., 2018, 76, 56–62 CrossRef CAS.
- C. Guo, Y. Xu, Z. Ren and J. Bai, Blue-White-Yellow Tunable Emission from Ce3+ and Eu2+ Co-Doped BaSiO3 Phosphors, J. Electrochem. Soc., 2011, 158(12), J373–J376 CrossRef CAS.
- C. Li, H. Chen, Y. Hua, L. Yu, Q. Jiang, D. Deng, S. Zhao, H. Ma and S. Xu, Photoluminescence and energy transfer studies on Ce3+/Eu2+ co-doped Ba3Si6O12N2 phosphor for white light-emitting-diodes, Opt. Commun., 2013, 295, 129–133 CrossRef CAS.
- C. Li, H. Zheng, H. Wei, S. Qiu, L. Xu, X. Wang and H. Jiao, A color tunable and white light emitting Ca2Si5N8:Ce3+, Eu2+ phosphor via efficient energy transfer for near-UV white LEDs, Dalton Trans., 2018, 47(19), 6860–6867 RSC.
- C. Yang, Q. Liu, D. Huang, X. Li, X. Zhang, Z. Bai and X. Wang, Synthesis and luminescence properties of Eu2+/Ce3+ co-doped SrB2Si2O8 phosphor, J. Mater. Sci.: Mater. Electron., 2019, 30(6), 5544–5554 CrossRef CAS.
- C.-H. Huang, L. Luo and T.-M. Chen, An Investigation on the Luminescence and Ce3+→Eu2+ Energy Transfer in Ca9Y(PO4)7:Ce3+,Eu2+ Phosphor, J. Electrochem. Soc., 2011, 158(11), J341–J344 CrossRef CAS.
- C.-K. Chang and T.-M. Chen, Sr3B2O6:Ce3+,Eu2+: a potential single-phased white-emitting borate phosphor for ultraviolet light-emitting diodes, Appl. Phys. Lett., 2007, 91(8), 81902–81902-3 CrossRef.
- F. Liu, Y. Tian, D. Deng, M. Wu, B. Chen, L. Zhou and S. Xu, An optical thermometer with high sensitivity and superior signal discriminability based on dual-emitting Ce3+/Eu2+ co-doped La5Si2BO13 thermochromic phosphor, Journal of Rare Earths, 2020, 10, 3 Search PubMed.
- F. Xiao, Y. N. Xue and Q. Y. Zhang, Ca2BO3Cl:Ce3+,Eu2+: a potential tunable yellow–white–blue-emitting phosphors for white light-emitting diodes, Phys. B, 2009, 404(20), 3743–3747 CrossRef CAS.
- F. Zhang and W. Tang, Luminescence properties of Eu2+ and Ce3+ in Na5Ca2Al(PO4)4 produced by the combustion-assisted synthesis method, J. Non-Cryst. Solids, 2014, 389, 46–49 CrossRef CAS.
- G. Li, M. Li, L. Li, H. Yu, H. Zou, L. Zou, S. Gan and X. Xu, Luminescent properties of Sr2Al2SiO7:Ce3+,Eu2+ phosphors for near UV-excited white light-emitting diodes, Mater. Lett., 2011, 65(23–24), 3418–3420 CrossRef CAS.
- G. Li and Y. Wang, Photoluminescence properties of novel BaGd2Si3O10:RE2+/3+ (RE = Eu or Ce) phosphors with trichromatic emission for white LEDs, New J. Chem., 2017, 41, 9178–9183 RSC.
- H. Chen, C. Li, Y. Hua, L. Yu, Q. Jiang, D. Deng, S. Zhao, H. Ma and S. Xu, Influence of energy transfer from Ce3+ to Eu2+ on luminescence properties of Ba3Si6O9N4:Ce3+, Eu2+ phosphors, Ceram. Int., 2014, 40(1), 1979–1983 CrossRef CAS.
- P. Thiyagarajan and M. S. Ramachandra Rao, Cool white light emission on Ca3−(l+n)MgSi2O8:Ce3+, Eu2+ phosphors and analysis of energy transfer mechanism, Appl. Phys. A, 2010, 99, 947–953 CrossRef CAS.
- J. Hou, W. Jiang, Y. Fang and F. Huang, Red, green and blue emissions coexistence in white-light-emitting Ca11(SiO4)4(BO3)2: Ce3+, Eu2+, Eu3+ phosphor, J. Mater. Chem. C, 2013, 1, 5892–5898 RSC.
- J. Sun, J. Zeng, Y. Sun and H. Du, Photoluminescence properties and energy transfer of Ce3+, Eu2+ co-doped Sr3Gd(PO4)3 phosphor, J. Alloys Compd., 2012, 540, 81–84 CrossRef CAS.
- J. Yan, C. Liu, W. Zhou, Y. Huang, Y. Tao and H. Liang, VUV-UV-vis photoluminescence of Ce3+ and Ce3+-Eu2+ energy transfer in Ba2MgSi2O7, J. Lumin., 2017, 185, 251–257 CrossRef CAS.
- L. Chen, R. Liu, W. Zhuang, Y. Liu, Y. Hu, X. Zhou and X. Ma, A study on photoluminescence and energy transfer of SrAlSi4N7:Eu2+, Ce3+ phosphors for application in white-light LED, J. Alloys Compd., 2015, 627, 218–221 CrossRef CAS.
- L. Zhou, H. Liang, P. A. Tanner, S. Zhang, D. Hou, C. Liu, Y. Tao, Y. Huang and L. Li, Luminescence, cathodoluminescence and Ce3+→Eu2+ energy transfer and emission enhancement in the Sr5(PO4)3Cl:Ce3+,Eu2+ phosphor, J. Mater. Chem. C, 2013, 1(43), 7155–7165 RSC.
- M. Chen, Z. Xia, M. S. Molokeev and Q. Liu, Insights into Ba4Si6O16 structure and photoluminescence tuning of Ba4Si6O16:Ce3+,Eu2+ phosphors, J. Mater. Chem. C, 2015, 3(48), 12477–12483 RSC.
- W. Shen, Y. Zhu and Z. Wang, Luminescent properties of Ca3SiO4Cl2 co-doped with Ce3+ and Eu2+ for near-ultraviolet light-emitting diodes, Luminescence, 2015, 30, 1409–1412 CrossRef CAS PubMed.
- N. Lakshminarasimhan and U. V. Varadaraju, White-Light Generation in Sr2SiO4: Eu2+, Ce3+ under Near-UV Excitation, J. Electrochem. Soc., 2005, 152(9), H152–H156 CrossRef CAS.
- P. Li, Z. Wang, Q. Guo and Z. Yang, Tunable Emission Phosphor Ca4Y6O(SiO4)6:Ce3+, Eu2+: Luminescence and Energy Transfer, J. Am. Ceram. Soc., 2015, 98(2), 495–500 CrossRef CAS.
- P. Li, Z. Wang, Z. Yang and Q. Guo, A potential single-phased white-emitting LiBaBO3: Ce3+, Eu2+ phosphor for white LEDs, J. Rare Earths, 2010, 28(4), 523–525 CrossRef CAS.
- P. Thiyagarajan and M. S. Ramachandra Rao, Cool white light emission on Ca3−(l+n)MgSi2O8: Cel3+, Eun2+ phosphors and analysis of energy transfer mechanism, Appl. Phys. A: Mater. Sci. Process., 2010, 99(4), 947–953 CrossRef CAS.
- Q. Wang, D. Deng, Y. Hua, L. Huang, H. Wang, S. Zhao, G. Jia, C. Xia and S. Xu, Potential tunable white-emitting phosphor LiSr4(BO3)3:Ce3+, Eu2+ for ultraviolet light-emitting diodes, J. Lumin., 2012, 132(2), 434–438 CrossRef CAS.
- T. Li, P. Li, Z. Wang, S. Xu, Q. Bai and Z. Yang, Coexistence phenomenon of Ce3+–Ce4+ and Eu2+–Eu3+ in Ce/Eu co-doped LiBaB9O15 phosphor: luminescence and energy transfer, Phys. Chem. Chem. Phys., 2017, 19, 4131–4138 RSC.
- V. Sivakumar and U. V. Varadaraju, Eu2+, Ce3+ Luminescence and Ce3+\Eu2+ Energy-Transfer Studies on Sr2LiSiO4F: A White Light-Emitting Phosphor, J. Electrochem. Soc., 2009, 156(7), J179–J184 CrossRef CAS.
- W. Lv, N. Guo, Y. Jia, Q. Zhao and H. You, A potential single-phased emission-tunable silicate phosphor Ca3Si2O7: Ce3+, Eu2+ excited by ultraviolet light for white light emitting diodes, Opt. Mater., 2013, 35(5), 1013–1018 CrossRef CAS.
- W. Wang, Y. Yang, Y. Liu, D. Pan, X. Luo, S. Yan, G. Xiang and Y. Jin, Photoluminescence properties and efficient energy transfer of Ce3+/Eu2+ activated K2Ba7Si16O40 phosphors, Mater. Res. Bull., 2018, 101, 232–239 CrossRef CAS.
- W.-J. Yang and T.-M. Chen, Ce3+/Eu2+ codoped Ba2ZnS3: a blue radiation-converting phosphor for white light-emitting diodes, Appl. Phys. Lett., 2007, 90(17), 171908–171908-3 CrossRef.
- X. Meng, X. Jie, Y. Zhang, D. Wang, Y. Wang, P. Li and Z. Wang, Luminescence and energy transfer of Ce3+-Eu2+ in Ca9Al(PO4)7, Optoelectronics Letters, 2015, 11(1), 45–48 CrossRef.
- X. Song, R. Fu, S. Agathopoulos, H. He, X. Zhao and X. Yu, Synthesis of BaSi2O2N2:Ce3+,Eu2+ Phosphors and Determination of their Luminescence Properties, J. Am. Ceram. Soc., 2011, 94(2), 501–507 CrossRef CAS.
- X. Tong, J. Han, Z. Cheng, P. Wu and X. Zhang, Efficient energy transfer induced tunable emission in Ce3+ and Eu2+ co-doped Ba1.2Ca0.8SiO4 phosphor, J. Lumin., 2019, 215, 116670 CrossRef CAS.
- Y. Fang, L. Li, Y. Chen, H. Wang and R. Zeng, Photoluminescence properties of Ce3+ and Ce3+–Eu2+ energy transfer in Ba1.3Ca0.7SiO4 phosphors, J. Lumin., 2013, 144, 13–17 CrossRef CAS.
- Y. Jia, H. Qiao, Y. Zheng, N. Guo and H. You, Synthesis and photoluminescence properties of Ce3+ and Eu2+-activated Ca7Mg(SiO4)4 phosphors for solid state lighting, Phys. Chem. Chem. Phys., 2012, 14, 3537–3542 RSC.
- Y. Jia, R. Pang, H. Li, W. Sun, J. Fu, L. Jiang, S. Zhang, Q. Su, C. Li and R.-S. Liu, Single-phased white-light-emitting Ca4(PO4)2O:Ce3+, Eu2+ phosphors based on energy transfer, Dalton Trans., 2015, 44, 11399–11407 RSC.
- Y. Wang, J. Ding, Y. Wang, X. Zhou, Y. Cao, B. Ma, J. Li, X. Wang, T. Setoa and Z. Zhao, Structural design of new Ce3+/Eu2+-doped or co-doped phosphors with excellent thermal stabilities for WLEDs, J. Mater. Chem. C, 2019, 7, 1792–1820 RSC.
- Z. Hu, Z. Cheng, P. Dong, H. Zhang and Y. Zhang, Enhanced photoluminescence
property of single-component CaAlSiN3: Ce3+, Eu2+ multicolor phosphor through Ce3+-Eu2+ energy transfer, J. Alloys Compd., 2017, 727, 633–641 CrossRef CAS.
- Z. Pan, J. Xu, C. Zhu, W. Liu and L. Wang, Ba2Mg(BO3)2:Ce3+,Eu2+,Na+: a potential single-phased two colour borate phosphor for white light-emitting diodes, J. Rare Earths, 2012, 30(11), 1088–1091 CrossRef CAS.
- Z. Wang, X. Teng, P. Li, J. S. Lee, S. Unithrattil, W. B. Im, S. Ye, F. Xiao, Y. X. Pan and Y. Y. Ma, Luminescence and energy transfer of Ce3+–Eu2+ in BaMg2(PO4)2, J. Alloys Compd., 2014, 589, 549–552 CrossRef CAS.
- Z. Xia, S. Miao, M. Chen, M. S. Molokeev and Q. Liu, Structure, Crystallographic Sites, and Tunable Luminescence Properties of Eu2+ and Ce3+/Li+-Activated Ca1.65Sr0.35SiO4 Phosphors, Inorg. Chem., 2015, 54, 7684–7691 CrossRef CAS PubMed.
- Z. Zhang and W. Tang, Efficient sensitization of Eu2+/Mn2+ emissions by Ce3+ doping in NaMgPO4 host under UV excitation, Appl. Phys. A: Mater. Sci. Process., 2016, 122(3), 229–237 CrossRef.
- Z. Zhang and W. Tang, Tunable luminescence and energy transfer of Ce3+/Eu2+/Mn2+-tridoped Sr8MgLa(PO4)7 phosphor for white light LEDs, J. Alloys Compd., 2016, 663, 731–737 CrossRef CAS.
- H. He, R. Fu a, Y. Cao, X. Song, Z. Pan, X. Zhao, Q. Xiao and R. Li, Ce3+, Eu2+ energy transfer mechanism in the Li2SrSiO4: Eu2+, Ce3+ phosphor, Opt. Mater., 2010, 32, 632–636 CrossRef CAS.
- P. Li, Z. Wang, Z. Yang and Q. Guo, Incorporating Ce3+ into a high efficiency phosphor Ca2PO4Cl: Eu2+ and its luminescent properties, RSC Adv., 2014, 4, 27708–27713 RSC.
- Y. Chen, Z. Wang, W. Ding, X. Li, Q. Bao, J. Liu, K. Qiu, X. Meng, Z. Yang and P. Li, A single-phase white light emitting phosphor Ba3Y(PO4)3: Ce3+/Eu2+/Mn2+: luminescence, energy transfer and thermal stability, J. Lumin., 2019, 210, 322–334 CrossRef CAS.
- G. Li, Y. Tian, Y. Zhao and J. Lin, Recent progress in luminescence tuning of Ce3+ and Eu2+ activated phosphors for pc-WLEDs, Chem. Soc. Rev., 2015, 44(23), 8688–8713 RSC.
- H. Terraschke and C. Wickleder, UV, Blue, Green, Yellow, Red, and Small: Newest Developments on Eu2+-Doped Nanophosphors, Chem. Rev., 2015, 115(20), 11352–11378 CrossRef CAS PubMed.
- Z. Xia and Q. Liu, Progress in discovery and structural design of color conversion phosphors for LEDs, Prog. Mater. Sci., 2016, 84, 59–117 CrossRef CAS.
- Z. Xia and K. R. Poeppelmeier, Chemistry-Inspired Adaptable Framework Structures, Acc. Chem. Res., 2017, 50, 1222–1230 CrossRef CAS PubMed.
- J. Qiao, J. Zhao, Q. Liu and Z. Xia, Recent advances in solid-state LED phosphors with thermally stable luminescence, J. Rare Earths, 2019, 37, 565–572 CrossRef CAS.
- J. Qiao, J. Zhao and Z. Xia, (INVITED) A review on the Eu2+ doped β-Ca3(PO4)2-type phosphors and the sites occupancy for photoluminescence tuning, Opt. Mater., 2019, 1, 100019 CrossRef CAS.
- X. Qin, X. Liu, W. Huang, M. Bettinelli and X. Liu, Lanthanide-Activated Phosphors Based on 4f-5d Optical Transitions: Theoretical and Experimental Aspects, Chem. Rev., 2017, 117(5), 4488–4527 CrossRef CAS PubMed.
- L. Wang, R.-J. Xie, T. Suehiro, T. Takeda and N. Hirosaki, Down-Conversion Nitride Materials for Solid State Lighting: Recent Advances and Perspectives, Chem. Rev., 2018, 118(4), 1951–2009 CrossRef CAS PubMed.
- C. Lin, A. Meijerink and R. S. Liu, Critical Red Components for Next-Generation White LEDs, J. Phys. Chem. Lett., 2016, 7, 495–503 CrossRef CAS PubMed.
- K. Li, M. Shang, H. Lian and J. Lin, Recent development in phosphors with different emitting colors via energy transfer for FEDs and UV/n-UV w-LEDs, J. Mater. Chem. C, 2016, 4, 5507–5530 RSC.
- M. Shang, C. Li and J. Lin, How to produce white light in a single-phase host, Chem. Soc. Rev., 2014, 43, 1372–1386 RSC.
- C. Guo, Y. Xu, X. Ding, M. Li, J. Yu, Z. Ren and J. Bai, Blue-emitting phosphor M2B5O9Cl: Eu2+ (M = Sr, Ca) for white LEDs, J. Alloys Compd., 2011, 509(4), L38–L41 CrossRef CAS.
- C. Tao, Z. Wang, Z. Li, N. Zhang, Z. Yang and P. Li, Blue emitting phosphor Sr0.8Ca0.2Al2+ySi2-yO8: Ce3+: substitution of Al-Si, structural modification, luminescence property and application, J. Alloys Compd., 2019, 788, 1000–1008 CrossRef CAS.
- J. Ding, H. You, Y. Wang, B. Ma, X. Zhou, X. Ding, Y. Cao, H. Chen, W. Geng and Y. Wang, Site occupation and energy transfer of Ce3+-activated lithium nitridosilicate Li2SrSi2N4 with broad-yellow-light-emitting property and excellent thermal stability, J. Mater. Chem. C, 2018, 6, 3435–3444 RSC.
- J. Liu, Z. Wang, X. Li, X. Meng, K. Qiu, D. Wang, J. Zhao, W. Lai, Z. Yang and P. Li, Achieving tunable emitting YAl3(BO3)4: Eu phosphors by substituting cations (Sr2+, Ca2+, Ga3+, In3+) and controlling BO3 group polarizability, CrystEngComm, 2020, 22, 5323–5337 RSC.
- J. Wang, S. Huang, M. Shang, P. Dang, H. Lian and J. Lin, The effect of local structure on the luminescence of Eu2+ in ternary phosphate solid solutions by cationic heterovalent substitution and their application in white LEDs, J. Mater. Chem. C, 2021, 9, 3 Search PubMed.
- K. V. Terebilenko, S. G. Nedilko, V. P. Chornii, V. M. Prokopets, M. S. Slobodyanika and V. V. Boykob, Structural and optical properties of langbeinite-related red-emitting K2Sc2(MoO4)(PO4)2: Eu phosphors, RSC Adv., 2020, 10, 25763–25772 RSC.
- M. Amin, P. Strobel, A. Qamar, T. Giftthaler, W. Schnick and A. Moewes, Understanding of Luminescence Properties Using Direct Measurements on Eu2+-Doped Wide Bandgap Phosphors, Adv. Opt. Mater., 2020, 8, 16 CrossRef.
- M. Tian, Z. Wang, W. Li, C. Wang, J. Cheng, Z. Li, Z. Yang and P. Li, Regulation defect and Eu2+ luminescence via cation substitution in Ca2BO3Cl: Eu2+, M2+ (M = Sr and Ba) for white LEDs, J. Alloys Compd., 2019, 787, 1004–1014 CrossRef CAS.
- M. Tian, Z. Wang, Z. Li, J. Cheng, C. Wang, X. Li, Z. Yang and P. Li, Excellent human eye sensitivity matching, high luminous efficacy, high quantum yield and thermal stability of narrow-band red-emitting SrLiAl3N4: Eu2+ phosphor, J. Lumin., 2019, 207, 545–552 CrossRef CAS.
- M. Zhao, Q. Zhang and Z. Xia, Structural Engineering of Eu2+ Doped Silicates Phosphors for LED Applications, Acc. Mater. Res., 2020, 1, 137–145 CrossRef CAS.
- P. Dang, G. Li, S. Liang, H. Lian and J. Lin, Multichannel photoluminescence tuning in Eu-doped apatite phosphors via coexisting cation substitution, energy transfer and valence mixing, J. Mater. Chem. C, 2019, 7, 5975–5987 RSC.
- P. Li, Z. Wang, Z. Li, J. Cheng, Y. Sun, C. Wang, X. Teng, Z. Yang and F. Teng, Controlling multi luminescent centers via anionic polyhedron substitution to achieve single Eu2+ activated high-color-rendering white light/tunable emissions in single-phased Ca2(BO3)1−x(PO4)xCl phosphors for ultraviolet converted LEDs, Chem. Eng. J., 2017, 326, 667–679 CrossRef.
- Q. Bai, P. Li, Z. Wang, S. Xu, T. Li and Z. Yang, Tuning of luminescence properties by controlling an aid-sintering additive and composition in Na(Ba/Sr/Ca)PO4: Eu2+ for white LEDs, RSC Adv., 2017, 7, 22301–22310 RSC.
- Q. Bao, Z. Wang, Z. Wang, X. Meng, K. Qiu, Y. Chen, Y. Li, J. Sun, Z. Yang and P. Li, Role of H3BO3 and Sr2+/K+ in the luminescent property of NaBaB9O15: Eu2+, J. Lumin., 2020, 218(30), 116840 CrossRef CAS.
- Q. Wei, X. Zhou, Z. Tang, X. Wang and Y. Wang, A novel blue-emitting Eu2+-doped chlorine silicate phosphor with a narrow band for illumination and displays: structure and luminescence properties, CrystEngComm, 2019, 21, 3660–3667 RSC.
- Q. Wu, X. Wang, Z. Zhao, C. Wang, Y. Li, A. Maoa and Y. Wang, Synthesis and luminescence characteristics of nitride Ca1.4Al2.8Si9.2N16: Ce3+, Li+ for light-emitting devices and field emission displays, J. Mater. Chem. C, 2014, 2, 7731–7738 RSC.
- Q. Zhang, X. Wang, Z. Tang and Y. Wang, A K3ScSi2O7: Eu2+ based phosphor with broad-band NIR emission and robust thermal stability for NIR pc-LEDs, Chem. Commun., 2020, 56, 4644–4647 RSC.
- X. Li, P. Li, C. Liu, L. Zhang, D. Dai, Z. Xing, Z. Yang and Z. Wang, Tuning the luminescence of Ca9La(PO4)7: Eu2+ via artificially inducing potential luminescence centers, J. Mater. Chem. C, 2019, 7, 14601–14611 RSC.
- Z. Li, T. Seto and Y. Wang, Enhanced crystallinity and thermal stability of Ba2+ and Al3+–O2− co-substituted Sr2Si5N8: Eu2+, J. Mater. Chem. C, 2020, 8, 9874–9884 RSC.
- Z. Li, Z. Wang, P. Li, J. Cheng, M. Tian, C. Wang and Z. Yang, Improvement of thermal stability and photoluminescence in Sr0.8Ca0.2Al2Si2O8: Eu2+ by the substitution of Si–Na # Al–Sr and Ca # Sr for structural modifications, Dalton Trans., 2017, 46, 14310–14317 RSC.
- Z. Xia, J. Zhuang, A. Meijerink and X. Jing, Host composition dependent tunable multicolor emission in the single-phase Ba2(Ln1−zTbz)(BO3)2Cl: Eu phosphors, Dalton Trans., 2013, 42, 6327–6336 RSC.
- Z. Zhou, N. Zhang, J. Chen, X. Zhou, M. S. Molokeev and C. Guo, The Vis-NIR multicolor emitting phosphor Ba4Gd3Na3(PO4)6F2: Eu2+, Pr3+ for LED towards plant growth, J. Ind. Eng. Chem., 2018, 65, 411–417 CrossRef CAS.
- H. Zhang, D. Yuan, X. Mi, X. Liu and J. Lin, Color tuning in Ca3−xMx(PO4)2: Eu2+ (M = Sr, Ba) phosphors via cation substitution, Dalton Trans., 2020, 49, 8949–8958 RSC.
- Z. Xia, H. Liu, X. Li and C. Liu, Identification of the crystallographic sites of Eu2+ in Ca9NaMg(PO4)7: structure and luminescence properties study, Dalton Trans., 2013, 42, 16588–16595 RSC.
- Z. Wang, Z. Xia, M. S. Molokeev, V. V. Atuchin and Q. Liu, Blue-shift of Eu2+ emission in (Ba,Sr)3Lu(PO4)3: Eu2+ eulytite solid-solution phosphors resulting from release of neighbouring-cation-induced stress, Dalton Trans., 2014, 43(44), 16800–16804 RSC.
- Z. Qiu, H. Lian, M. Shang, S. Lian and J. Lin, The structural evolution and spectral blue shift of solid solution phosphors Sr3−mCamB2O6: Eu2+, CrystEngComm, 2016, 18, 4597–4603 RSC.
- Y. Wei, X. Qi, H. Xiao, W. Luo, H. Yao, L. Lv, G. Li and J. Lin, Site-preferential occupancy induced photoluminescence tuning in (Ca,Ba)5(PO4)3Cl: Eu2+ phosphors, RSC Adv., 2016, 6, 43771–43779 RSC.
- Y. Wu, Q. Li, B. C. Chakoumakos, M. Zhuravleva, A. C. Lindsey, J. Johnson II, L. Stand, M. Koschan and C. L. Melcher, Quaternary Iodide K(Ca,Sr)I3: Eu2+ Single-Crystal Scintillators for Radiation Detection: Crystal Structure, Electronic Structure, and Optical and Scintillation Properties, Adv. Opt. Mater., 2016, 4(10), 1518–1532 CrossRef CAS.
- S. Xin, M. Gao, C. Wang, X. Wang, G. Zhu, F. Zhou, Z. Lia and Y. Wang, Efficient and controllable photoluminescence in novel solid solution Ca1−xSrxHf4(PO4)6: Eu2+ phosphors with high thermal stability for white light emitting diodes, CrystEngComm, 2018, 20, 4383–4394 RSC.
- W. Ji, Z. Xia, K. Liu, S. Alikhan, L. Hao, X. Xu, L. Yin, M. S. Molokeev, S. Agathopoulos, W. Yang, X. Ma, K. Sun and I. Silva, Crystal structure and luminescence properties of a novel green-yellow emitting Ca1.5Mg0.5Si1-xLixO4-δ: Ce3+ phosphor with high quantum efficiency and thermal stability, Dalton Trans., 2018, 47 Search PubMed.
- S. Liu, Z. Wang, Q. Bao, X. Li, Y. Chen, Z. Wang, X. Meng, Y. Li, Z. Yang and P. Li, Determination of luminescence and occupy sites of Ce3+ in Zn3(BO3)(PO4) by introducing Ca2+ and Mg2+ ions, Spectrochim. Acta, Part A, 2019, 213, 134–140 CrossRef CAS PubMed.
|
This journal is © The Royal Society of Chemistry 2021 |