DOI:
10.1039/D1RA02553H
(Paper)
RSC Adv., 2021,
11, 19587-19597
First total synthesis of chromanone A, preparation of related compounds and evaluation of their antifungal activity against Candida albicans, a biofilm forming agent†
Received
31st March 2021
, Accepted 24th May 2021
First published on 1st June 2021
Abstract
A straightforward and convenient approach for the first total syntheses of chromanone A and a related 7-OMe substituted natural product is reported. These unique C-3 substituted 2-hydroxymethyl chromones were recently isolated as fungal metabolites. Chromanone A was synthesized in 25.3% overall yield from the readily available pyrocatechol, whereas the second natural product was prepared in 39.7% global yield. A small library of chromones, including both natural products and some of their synthetic heterocyclic precursors, was evaluated against Candida albicans ATCC 10231, a biofilm forming agent. It was found that 8-methoxy-3-methyl-4-oxo-4H-chromene-2-carbaldehyde, a partially oxidized form of chromanone A, exhibited a minimum inhibitory concentration of 7.8 μg mL−1 and significantly inhibited the yeast's virulence factors, including the adherence to buccal epithelial cells and the secretion of phospholipases, as well as the formation of germ tubes and the generation of the hyphal pseudomycelium. In addition, despite the heterocycle exhibiting non-significant inhibition of the formation of the Candida biofilm, it completely inhibited the growth of C. albicans in preformed biofilms at 62.5 μg mL−1.
1. Introduction
Biofilms are structured microbial communities, embedded in a self-produced polymeric matrix of protein and polysaccharides. They are frequently found on interfaces or attached to surfaces, and are the scenario of complex interactions. These enduring structures are elusive to the defense system of the host, reluctant to undergo phagocytosis, and offer the producing microorganism a markedly increased resistance to antibiotics and chemical disinfectants.1a Therefore, biofilms are an important form of microbial resistance and transmission of chronic infections.1b
The biofilms are ubiquitous, and their presence on surfaces such as biomedical implants, may put at risk complex surgical interventions and ultimately compromise the life of the patient. In this context, there is a need for antimicrobial agents capable of attacking microorganisms within the biofilms. Hence, the search for new compounds endowed with this property is currently relevant.
The chromone skeleton is a “privileged structure”,2a meaning a promising motif for drug development. Not surprisingly, substituted chromones are known to display a variety of useful biological properties, including antifungal activity.2b,c
In 2009, Gamal-Eldeen and coworkers reported the isolation of chromanone A (A, Fig. 1),3 from an algicolous marine Penicillium species, cultivated on a solid biomalt medium. In turn, this fungus was isolated as an endophyte of the Egyptian green alga Ulva sp. The natural product inhibits the activity of CYP1A at a level of 4 μg mL−1, being a potential cancer chemopreventive agent.3
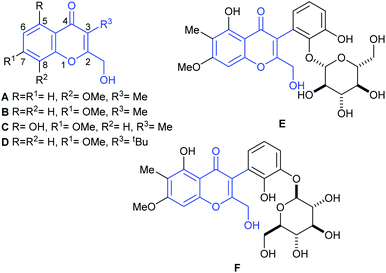 |
| Fig. 1 Chromanone A (A) and related naturally occurring 3-substituted-2-hydroxymethyl chromone derivatives. | |
The structure of the natural product was assigned based on an analysis of 1D and 2D (HSQC, HMBC) NMR spectra. Being a member of the very small family of the naturally occurring 2-hydroxymethylchromones which bear a C-3 functionalization, chromanone A is a structurally unique chromone. Its special structural characteristics are shared only by a handful of natural products, such as its isomer B, recently isolated from Rhinocladiella sp., a fungus obtained from the marine sponge Ircinia oros.4a These features are also found in heterocycles C4b and D,4c as well as in boeravinone Q (E)5a and its congener mirabijalone C (F).5b
Our work is focused on the synthesis of structurally unique heterocyclic natural products,6 as well as in their evaluation.7a,b Further, among chromone derivatives, we have developed the total synthesis of the structure assigned to aspergillitine,7c a 2,3-dimethyl chromone derivative isolated from a marine Aspergillus species.
In pursuit of these interests, here we report an efficient approach to the first total syntheses of chromanone A (A) and of its isomer, the related natural product B. The results of the evaluation of both natural products and their heterocyclic synthetic intermediates, as antifungal agents against the biofilm forming yeast Candida albicans ATCC 12031, are also discussed.
2. Results and discussion
2.1 Chemistry
The initial step toward chromanone A as the first target (1), was a retrosynthetic analysis of the product (Scheme 1). Initially, focus was made on the oxygen bearing functionality of the 2-hydroxymethyl feature; a functional group interconversion was proposed, conjecturing that the proper oxidation stage of the C-2 substituent could be set either through reduction (aldehyde, ester, 2a) or by means of a selective oxidation.7a The latter possibility revealed the 2,3-dimethylchromone 2b as a suitable precursor.
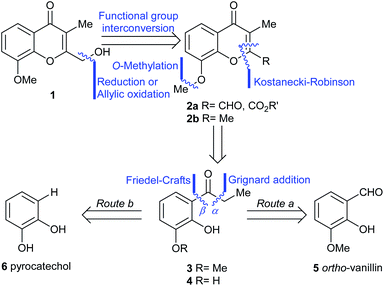 |
| Scheme 1 Retrosynthetic analyses of chromanone A (1). | |
Then, two different alternatives were considered. In one of them (Route a), the heterocyclic ring of 2a,b was disassembled as shown, to unveil a propiophenone derivative (3), which was further submitted to a C–O disconnection on the aliphatic side of the carbonyl moiety (bond α), to uncover ortho-vanillin (5) as a suitable starting material.
In the second case (Route b), an additional methyl ether C–O disconnection was considered on the chromone 2, which suggested the catechol derivative 4 as the most appropriate synthetic intermediate. In turn, a C–O disconnection on the aromatic side of the carbonyl motif of propiophenone 4 (bond β) set aside the three-carbon side chain and determined that the logical starting material for this approach was the commercially available pyrocatechol (6).
For simplicity, the Route a was explored first. Thus, ortho-vanillin (5) was subjected to a 1,2-addition to the carbonyl with excess ethyl Grignard reagent (Scheme 2), freshly prepared from iodoethane and activated magnesium. The reaction was executed at room temperature (RT), giving alcohol 7 in 97% yield.8
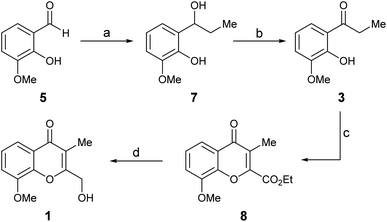 |
| Scheme 2 Reagents and conditions (a) CH3CH2I, Mg0, Et2O, RT (97%); (b) IBX, EtOAc, RT, 1 h (81%); (c) EtO2C–COCl, Et3N, CH2Cl2, MW (100 °C), 1 h (42%); (d) NaBH4, CaCl2, EtOH, 0 °C, 24 h (41%). | |
Subsequently, 7 was submitted to a selective oxidation of its secondary alcohol moiety. Different conditions [PDC, CH2Cl2; H2N–NH2·xH2O, DMSO/I2, MeCN/H2O (5
:
1, v/v); DMSO/Ac2O (2.3 equiv.); IBX (1.5 equiv.), EtOAc]9 were tested; however, the reaction proved most successful with IBX in EtOAc, furnishing ketone 3 in up to 81% yield in just one hour at room temperature.10 Unfortunately, this transformation proved to lack robustness, being highly dependent on the experimental conditions, including the source of the oxidizing reagent. This characteristic frequently resulted in an exacerbated concomitant oxidation of the ortho-phenol, ultimately causing tarry materials and low yields.
Next, a one-pot Kostanecki–Robinson cyclization protocol was practiced on the propiophenone 3 in order build the chromone system. The reaction of aromatic ketones with a glycolate ether was considered as an alternative, to access a 2-alkoxymethylene chromone; however, it generally proved to proceed in low yield11a and was soon discarded due to inability of the product to withstand the harsh conditions required to break the ether bond and the lack of selectivity of the reaction. In addition, model experiments with the scarcely precedented use of glyoxal11b as a two-carbon synthon, which would have delivered a 2-hydroxymethyl chromone directly, met with failure; no reaction was observed, despite different basic conditions (KOH, KtBuO and NH4OH in EtOH) were explored.
Therefore, the ketone 3 was exposed to ethyl chlorooxoacetate,12 which can be regarded as an oxidized form of glyoxal or glycolate ethers. Luckily, the reaction took place in CH2Cl2, in the presence of triethylamine at 100 °C under microwaves irradiation (MW), giving chromone 8 in a reproducible but rather moderate (42%) yield.
Finally, the required adjustment of the oxidation state of the C-2 substituent was carried out by means of a selective reduction of 8; however, since the use of NaBH4 as reducing agent13 did not perform as expected, this challenging transformation was carried out with Ca(BH4)2.14 The reagent was prepared in situ by adding a stoichiometric amount of CaCl2 to NaBH4 in EtOH.14c Under these conditions, chromanone A (1, compound A of Fig. 1) was obtained in 41% yield. Its spectroscopic data in CDCl3 confirmed its structure, whereas the NMR spectra taken in MeOH-d4 were in full agreement with those reported for the natural product.3 Signal enhancement (NOE) of the CH2OH moiety (0.8%) was observed upon irradiation of the hydrogen atoms of the 3-Me group, as well as between the hydrogen atoms of the 8-OMe group and H-7 (1.7%). Notably, the spectra taken in both solvents were alike, and differences exceeding 10 ppm were found between them, particularly for the carbon atoms of the isocyclic ring attached to oxygen.
Despite this first approach to 1 afforded the natural product in just four steps and 13.5% overall yield, the lack of robustness of the selective oxidation of 7, coupled to the moderate yields of the cyclization toward 8 and its selective ester moiety reduction stages, suggested the need to devise an improved alternative.
Therefore, we resorted to the second synthetic plan toward 1, commencing with the selective Friedel–Crafts ortho-acylation of pyrocatechol (6) under BF3·OEt2 promotion.15 However, since in our hands the reported procedure proved hard to be reproduced, a series of optimization experiments were run in order to find the proper reaction conditions.
In the process, it was soon found that ZnCl2 and AlCl3 are not suitable promoters, and that the use of 1,2-dichlorobenzene as solvent negatively affects the reaction performance. It was also discovered that under conventional thermal conditions (Table 1), the transformation hardly proceeded after 3 days at 110 °C (entry 1), whereas only small amounts of product were recovered when the reaction was performed at 180 °C for 5 h (entry 2).
Table 1 Optimization of the synthesis of propiophenone 4
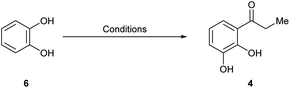
|
Entry no. |
EtCO2H (equiv.) |
BF3·Et2O (equiv.) |
Temp. (°C) |
Power MW (W) |
Time (min) |
Yield of 4 (%) |
Propionic anhydride was employed, in the presence of BHT (0.05 equiv.). |
1 |
9 |
1 |
110 |
— |
3 days |
7 |
2 |
13 |
1 |
180 |
— |
300 |
23 |
3 |
9.5 |
1 |
180 |
200 |
10 |
0 |
4 |
10 |
1.5 |
170 |
100 |
5 |
33 |
5 |
12 |
1 |
170 |
100 |
5 |
61 |
6 |
20 |
1 |
170 |
100 |
5 |
27 |
7 |
12 |
1 |
160 |
100 |
10 |
50 |
8 |
6.4a |
1 |
170 |
100 |
5 |
29 |
On the other hand, employing microwaves radiation, no product was isolated after 10 min at 180 °C (entry 3), whereas slightly milder conditions (170 °C, 5 min) resulted in 33% yield of product (entry 4), when 10 equiv. EtCO2H were used. Increasing the amount of acid caused a further increase in the yield to 61% (entry 5), while unexpectedly, additional amounts of acid produced a drastic yield reduction (entry 6). In addition, performing the reaction at 160 °C for 10 min (entry 7) or in the presence of propionic anhydride (entry 8) did not improve the results.
Therefore, the reaction was best performed as in entry 5, in a solventless condition and under microwaves irradiation, affording consistently over 60% yield of the expected product 4.16 The use of 6 instead of guaiacol for this approach was based on literature precedents, which suggested that the latter should not be a suitable starting material because it would afford the unwanted propiophenone isomer.17a,b
Next, 4 was cyclocondensed and further cyclized with Ac2O under Kostanecki-Robinson conditions, uneventfully affording the expected 2,3-dimethylchromone 9 (ref. 7c) in 76% yield (Scheme 3). A subsequent Williamson O-methylation of 9 with MeI/K2CO3 in refluxing acetone gave 94% yield of 2.17c This was followed by a selective oxidation with the versatile I2/DMSO reagent system, which conveniently furnished aldehyde 10 in 83% yield. The transformation, which was carried out aerobically, required the addition of TsOH.18a
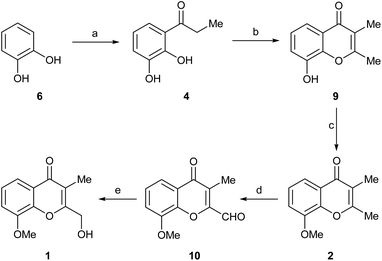 |
| Scheme 3 Reagents and conditions: (a) MeCH2CO2H, BF3·OEt2, MW (160 °C), 5 min (61%); (b) 1. Ac2O, NaOAc, reflux, 3 h; 2. Et3N, 115 °C, 14 h; 3. 1 M HCl, 40 °C, 3 h (76% overall); (c) K2CO3, MeI, Me2CO, reflux, 24 h (94%); (d) I2, TsOH, DMSO, O2, 130 °C, 2 h (83%); (e) NaBH4, EtOH, 0 °C → RT, 1 h (70%). | |
Finally, the reduction of the formyl moiety with NaBH4 in EtOH uneventfully provided the expected product 1 in 70% yield. Interestingly, unlike the reduction of compound 8, in this case the use of Ca(BH4)2 proved to be unnecessary, since the formyl group attached to C-2 is much more reactive than the C-4 ketone moiety.
This optimized approach gave 1 in 25.3% overall yield, after five synthetic steps. The NMR spectroscopic data of the synthetic compound (in MeOH-d4) were in excellent agreement with those of the literature,3 and the heterocycle obtained through Route a, confirming the structure of the natural product.
The intimate details of the mechanism of the key I2/DMSO-mediated oxidation toward 10 remain unclear; however, based on some previous literature precedents, a polar rather than a free-radical reaction mechanism can be proposed. Further, considering that the 2-methylchromone moiety may be regarded as a vinylogous α-methylketone,18 it can be conjectured that a Kornblum-like oxidation is at the heart of the mechanistic sequence (Scheme 4). In this scenario, at first the carbonyl group of the substrate (2) would be activated by the added TsOH, giving rise to intermediate I. In turn, this intermediate would be subjected to deprotonation to provide the dienol II, being followed by an iodination with I2, to generate the reactive iodide III.
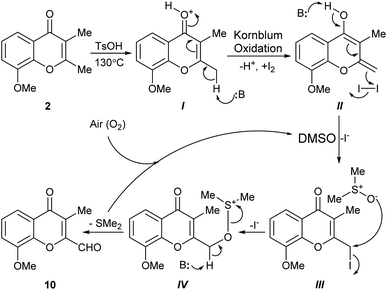 |
| Scheme 4 The Kornblum oxidation-based mechanistic proposal for the conversion of 2,3-dimethylchromone 2 into 10 with the I2/DMSO reagent system. | |
Next, in the presence of DMSO which plays the dual role of solvent and oxidant, the iodide III would undergo a SN2-type reaction with the nucleophilic oxygen atom of the reagent, losing iodide and forming the alkoxysulfonium salt intermediate IV. The latter would undergo a proton abstraction, resulting in the aldehyde product 10. Interestingly, it has been reported that reaction of a primary iodide like III with KO2 in DMSO afforded a hydroxymethyl chromone, albeit in rather low yield (27%).19
During the reaction two molecules of HI and one of SMe2 are produced. Although DMSO can oxidize iodide ions to iodine, generating SMe2 (DMSO + 2I− → SMe2 + I2 + H2O), it is assumed that the presence of oxygen (air) under the strenuous reaction conditions (130 °C) would serve to reoxidize all the SMe2 formed in the transformation and/or to regenerate the iodine in the presence of DMSO.
The general guidelines provided by the retrosynthetic analysis of Scheme 1 were used to synthesize compound 16 (Fig. 1, compound B) and to access additional heterocyclic intermediates for bioactivity testing.
The synthetic sequence commenced with the commercially available propiophenone 12 (Scheme 5) which was sequentially exposed to Ac2O/NaOAc,20 Et3N and HCl. Under these conditions, it experienced an exhaustive acetylation, followed by a Baker–Venkataraman rearrangement and cyclization, and a final dehydration and deacetylation to afford 13 in 77% overall yield.
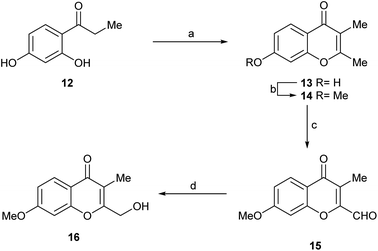 |
| Scheme 5 Reagents and conditions: (a) 1. Ac2O, NaOAc, reflux, 3 h; 2. Et3N, 115 °C, 14 h; 3. 1 M HCl, 40 °C, 6 h (77% overall); (b) MeI, K2CO3, Me2CO, reflux, 24 h (93%); (c) I2, TsOH, DMSO, O2, 130 °C, 2 h (78%); (d) NaBH4, EtOH, 0 °C → RT, 1 h (71%). | |
The phenol was methylated under conventional conditions, with MeI in refluxing acetone, using K2CO3 as base to afford 14 (93%). This is a natural product, which has been recently isolated from Rhinocladiella sp.4b and from the co-culture of a marine-derived Actinomycete (Streptomyces rochei MB037) and the fungus Rhinocladiella similis 35. Compound 14 proved to display weak antibacterial activity against Staphylococcus aureus and Pseudomonas aeruginosa.21
Next, 14 was selectively oxidized with the DMSO–I2 reagent system, providing aldehyde 15 in 78% yield.22 Finally, the aldehyde was selectively reduced with the aid of the NaBH4, affording the alcohol 16 (Fig. 1, compound B) in 71% yield. This synthetic route provided the natural product 16 with an overall yield of 39.7% in just four steps.
2.2 Evaluation of bioactivity
The methods employed are fully described in the ESI.† Initially, antifungal susceptibility tests were carried out, with the determination of the minimum inhibitory concentration (MIC) and the minimum fungicidal concentration (MFC). The antifungal activity of the compounds was assessed in the concentration range 0.49–250 μg mL−1 with the standardized CLSI microbroth dilution method for yeasts23 against C. albicans ATCC 10231. Initially, the aldehyde 10 and the natural product (1) were screened; however, in order to obtain a better insight into the structural factors implied in the bioactivity, their precursors 2 and 9 as well as the related 7-substituted 2,3-dimethylchromones 13 and 14 were also tested, along with the aldehyde 15 and the natural product 16.
The results are collected in Fig. 2, which depicts the percentage of growth inhibition of a standardized inoculum of C. albicans (1 × 103 CFU per mL) plotted against the logarithm of the corresponding concentration of each compound.
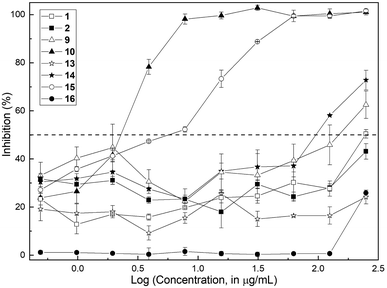 |
| Fig. 2 Semi-logarithmic plot of the growth inhibition of Candida albicans ATCC 10231 in the presence of different concentrations of the chromone test compounds. The dashed line indicates the 50% inhibition level. | |
The assay revealed that the natural product (1) is a very weak inhibitor, being considered inactive. It barely caused 50% inhibition at a concentration of 250 μg mL−1 (MIC > 250 μg mL−1); quite a similar profile was exhibited by its phenolic precursor 9 (63% inhibition at 250 μg mL−1). On the contrary, the aldehyde 10 proved to be a very good inhibitor with MIC = 7.8 μg mL−1.
Regarding the 7-substituted chromones, compounds 13, 14 and 16 demonstrated to be inactive. Compound 14 exhibited 72.8% inhibition at 250 μg mL−1 (MIC > 250 μg mL−1), whereas aldehyde 15 was moderately active, with a MIC value of 62.5 μg mL−1. The MFC values for the isomeric active aldehydes 10 and 15 were also determined according to the established protocol,23 being 125 μg mL−1 in both cases.
The results suggested that the presence of a formyl moiety is relevant for the observed activity, and that the latter can be modulated by the position of the oxygenated substituent in the homocyclic ring.
Next, the effect of the heterocycles on the virulence factors of C. albicans were examined. The tests included inhibition of the adherence to buccal epithelial cells, inhibition of the formation of the germ-tube, morphogenesis of C. albicans on solid media and the inhibition of lytic enzymes.
The first step by which a microorganism can initiate an infection is through adherence to an epithelial surface; this ability enables it to exist in biofilms and is in clear association with its virulence.24 In order to evaluate whether the active compounds are able to affect this process, at sub-lethal concentrations, compounds 10 and 15 were submitted to the assay of inhibition of the adherence to buccal epithelial cells (BEC).
The results (Fig. 3A) showed that the number of yeasts adhered to 100 BEC decreased from 2972 ± 233 in the untreated control cells to 177 ± 60, in the presence of compound 10 at MFC/2; interestingly, the compound was still active at MFC/32 (number of yeasts adhered to 100 BEC = 1724 ± 399). Further, as seen in Fig. 3B, treatment with sub-lethal concentrations of compound 15 also caused a remarkable decrease in yeast adherence to BEC at levels ranging from MFC/2 (482 ± 187) to MFC/8 (1334 ± 233).
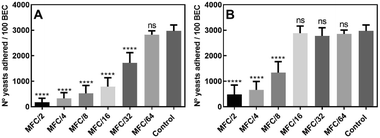 |
| Fig. 3 Adherence of Candida albicans ATCC 10231 to human buccal epithelial cells (BEC) after incubation in media containing sub-lethal concentrations of 10 (A) and 15 (B). The adherence to BEC is expressed as the number of adhered yeasts/100 BEC. Wilcoxon test = ****(p < 0.0001), ns: not significant. | |
These results clearly indicated that the amount of adhered fungal cells to BEC was significantly lower (Wilcoxon test, p < 0.0001) in yeasts treated with both aldehydes than in the untreated cells, suggesting that the presence of these heterocycles causes some degree of resistance to the colonization of BEC by C. albicans.
In the germ-tube inhibition assay, the effect of different geometrically distributed sub-lethal concentrations (MFC/64–MFC/2) of compounds 10 and 15 on the formation of germ tubes (GT) in C. albicans was assessed.
The results (Fig. 4A) showed that the presence of compound 10 at concentrations between MFC/2 and MFC/32, inhibited the formation of hyphae (GT% = 48.5 ± 2.1% at MFC/32) in a dose-dependent way with respect to the control (GT% = 92 ± 1.4%). The maximum degree of inhibition was recorded at MFC/2 (GT% = 12.0 ± 1.4%).
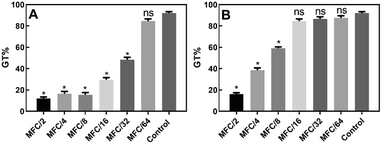 |
| Fig. 4 Candida albicans ATCC 10231 germ tube formation (% cells) either without (control) or with exposure to sub-lethal concentrations of compounds 10 (A) and 15 (B). Holm–Sidak test = *(p < 0.05), ns: not significant. | |
Compound 15 proved to be a less powerful inhibitor (Fig. 4B), which inhibited hyphae formation up to a concentration of MFC/8 (GT% = 59 ± 1.4%). At MFC/2, the observed GT% was 16.0 ± 1.4%. The results were all statistically significant according to the Holm–Sidak test (p < 0.05).
The morphogenesis of C. albicans on solid media was studied by examining the effects of compounds 10 and 15 on the formation of pseudomycelium in C. albicans in the nutrient-poor Spider medium, that induces pseudohyphal morphogenesis. It was observed that the aldehyde 10 effectively reduced formation of the hyphal pseudomycelium up to a concentration of MFC/4. This effect was apparent by the smoother aspect of the colonies and the notable reduction of hyphae at the edges. On the other hand, in the presence of compound 15, the C. albicans colonies showed their typical filamentation at the edges, suggesting that this heterocycle is not an effective inhibitor of pseudomycelium formation.
Finally, in the study of lytic enzyme inhibition, it was detected a statistically significant inhibition of phospholipases secretion in the presence of compound 10 at MFC/2 (Pz = 0.92 ± 0.01) and MFC/4 (Pz = 0.82 ± 0.02) with respect to the control (Pz = 0.74 ± 0.02). Contrarily, however, no significant changes in the Pz index were observed between the untreated (control) cells and those exposed to compound 15. This signaled that the secretion of phospholipases was not inhibited.
On the other hand, it was also observed that at sub-lethal concentrations, compounds 10 and 15 did not inhibit secretion of C. albicans esterases, since their values of the Pz index (Pz = 0.82 ± 0.02) were the same as the control.
In view of these promising observations, the interaction of the chromone derivatives on formation of the C. albicans biofilm and on preformed biofilms was examined. In the first case, an inoculum prepared according to Pierce et al. was used, employing yeast extract–peptone–dextrose (YPD) medium.25
Fig. 5A displays the data related to the inhibition of the formation of the C. albicans biofilm in the presence of different concentrations of compounds 10 and 15. A compound was considered active if it significantly inhibited biofilm formation at concentrations below the MIC (sub-inhibitory). However, it was detected that none of the heterocycles managed to completely avoid formation of the biofilm.
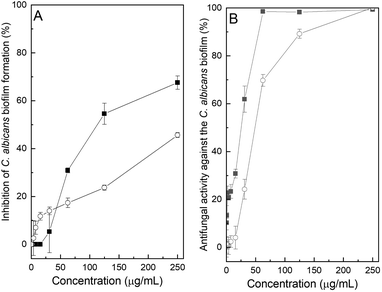 |
| Fig. 5 Activity of compounds 10 (■) and 15 (○) against the C. albicans ATCC 10231 biofilm. (A) Inhibition of the biofilm formation. (B) Antifungal activity against the pre-formed biofilm. | |
In the presence of 10 at a level of 250 μg mL−1, the inhibition was 67.6% (54.5% at 125 μg mL−1), while 15 exhibited meager values of 45.6% and 23.7% inhibition at the same concentrations, respectively. Not unexpectedly, at the corresponding MIC values, their degree of inhibition lacked statistical significance; therefore, based on these results, none of the heterocycles inhibited biofilm formation.
On the other hand, when the antifungal activity against preformed C. albicans biofilms was evaluated, it was observed that compounds 10 and 15 displayed almost complete inhibition at concentrations of 62.5 and 250 μg mL−1, respectively. Further, compound 10 caused over 60% inhibition when the concentration was halved. The effect was concentration dependent, as reflected in the progressive increase in cell viability with reducing concentrations of both compounds.
The results indicate that, while the MIC of compound 10 against planktonic cells of C. albicans is 7.8 μg mL−1, the aldehyde can completely inhibit the growth of the biofilm yeast colonies at a concentration of 62.5 μg mL−1, suggesting that it may be a useful and promising advantage in the development of more effective anti-biofilm agents.
3. Conclusions
Two facile and expedient approaches to the first total synthesis of chromanone A, a unique chromone isolated from an algicolous fungus Penicillium sp., endophyte of the Egyptian green alga Ulva sp., were developed. In the most efficient sequence, the heterocycle was accessed in only five steps and 25.3% overall yield from pyrocatechol, a commercial and easily available starting material.
The key stages of the synthesis included a selective Friedel–Crafts acylation of the starting catechol under BF3·Et2O promotion and the aerobic oxidation of the C-2 methyl group of a chromone intermediate by the I2/DMSO reagent system. To the best of our knowledge, this is the first report of the use of this approach and this reagent system for the synthesis of 2-formyl- and 2-hydroxymethyl chromones. A 7-methoxy isomer of the natural product, which is also a fungal natural product, was prepared following the same strategy. Neither of the synthetic sequences required the use of protecting groups, favouring the efficiency of the syntheses.
The tests of antifungal activity against C. albicans revealed that 8-methoxy-3-methyl-4-oxo-4H-chromene-2-carbaldehyde, the 2-formyl analogue of chromanone A, exhibited a MIC = 7.8 μg mL−1 (MFC = 125 μg mL−1). In addition, at sub-lethal concentration levels, this compound significantly inhibited various C. albicans virulence factors, including the secretion of phospholipases and the yeast adherence to buccal epithelial cells, as well as the formation of the hyphal pseudomycelium and germ tubes. On the other hand, the formyl derivative completely inhibited the growth of C. albicans in preformed biofilms at 62.5 μg mL−1.
Taken together, the results indicate that the 2-formyl analogue of chromanone A not only kills C. albicans, but also inhibits its virulence factors, suggesting that it is able to target both, the cell growth and the pathogenic process. These features turn the aldehyde into a potentially useful lead to develop more effective agents to fight this yeast in biofilm scenarios. These findings also support the hypothesis that marine fungi may be important sources of inspiration for the development of new agents to combat biofilm-forming microorganisms, and perhaps help to overcome difficulties or failure of current therapy for fungal infections.
4. Experimental section
4.1 General information
The chemical transformations were carried out under dry argon atmospheres, employing freshly distilled anhydrous solvents and oven-dried glassware. Anhydrous CH2Cl2 was obtained from an MBraun solvent purifier and dispenser system. Absolute EtOH was prepared by refluxing the commercial solvent over clean Mg/I2 and distilling from the resulting magnesium alkoxide. Anhydrous Et3N was prepared by distillation of the commercial product from CaH2. The anhydrous solvents were stored in dry Young ampoules. The rest of the solvents and reagents were used as received.
The reactions were monitored by TLC, employing aluminium supported silica gel 60 GF254 plates run in different hexanes:EtOAc mixtures. The chromatographic spots were revealed by exposure to UV light (254 and 365 nm), followed by spraying with the ethanolic p-anisaldehyde/sulfuric acid reagent and gentle heating to improve selectivity.
The flash column chromatographies were developed under positive pressure with slurry-packed silica gel 60 H for thin layer chromatography (particle size < 55 μm), employing gradient of solvent polarity techniques with hexanes:EtOAc.
4.2 Equipment
The melting points were determined on an Ernst Leitz Wetzlar model 350 hot-stage microscope and are informed uncorrected. The FT-IR spectra were acquired on a Shimadzu Prestige 21 spectrophotometer, as thin films held between two NaCl disks or as solid dispersions in KBr (solid samples). The NMR spectra were recorded in CDCl3 with a Bruker Avance 300 NMR spectrometer (300.13 MHz for 1H and 75.48 MHz for 13C). The chemical shifts are reported in the δ scale, in ppm downfield from TMS (δ = 0.0 ppm). The residual solvent peaks of CDCl3 (δH = 7.26 ppm, δC = 77.16 ppm), were used as internal references. The scalar coupling constant (J) and width and half-height (w1/2) values are given in Hertz. NOE and 2D NMR experiments (HSQC and HMBC) were also acquired in order to ensure unambiguous signal assignment. The HRMS were obtained with a Bruker MicroTOF-Q II instrument from UMyMFOR (Buenos Aires, Argentina). The microwave-assisted reactions were carried out in a CEM Discover microwave reactor. The light microscopy observations and determinations were performed with an Eclipse E100 (Nikon Corp., Tokyo, Japan) instrument. The microplates were read in a VERSA Max microplate reader (Molecular Devices, Sunnyvale, CA, USA).
4.3 2-(1-Hydroxypropyl)-6-methoxyphenol (7)
A stirred solution of ortho-vanillin (5, 1500 mg, 9.86 mmol) in anhydrous Et2O (10 mL) was purged with nitrogen, cooled to 0 °C in an ice bath and treated dropwise with a solution of EtMgI (2.5 M, 24.6 mmol) in Et2O, prepared in situ from ethyl iodide and magnesium turnings. The mixture was allowed to warm to room temperature and then additionally stirred for 1 hour. At the end of the reaction (assessed by TLC), a saturated solution of NH4Cl was added (10 mL) and the organic products were extracted with CH2Cl2 (3 × 20 mL). The combined organic extracts were washed with brine (10 mL), dried over anhydrous MgSO4, and concentrated under reduced pressure. Chromatography of the residue provided 7 (1742 mg, 97%) as a yellowish oil.8b IR (film,
): 3443, 2965, 1620, 1593, 1487 and 1454 cm−1. 1H NMR (300 MHz, CDCl3): δ = 6.85–6.75 (3H, m, H-3, H-4, H-5), 6.31 (1H, s, OH), 4.79 (1H, q, J = 6.3, H-1′), 3.90 (3H, s, OMe), 2.55 (1H, d, J = 5.6, OH) 1.97–1.78 (2H, m, H-2′) and 0.96 (3H, t, J = 7.4, Me). 13C NMR (75 MHz, CDCl3): δ = 146.8 (C-6), 143.1 (C-1), 129.5 (C-2), 119.6 (C-3)*, 119.3 (C-4)*, 109.8 (C-5), 73.4 (C-1′), 56.1 (OMe), 30.1 (C-2′) and 10.3 (C-Me).
4.4 1-(2-Hydroxy-3-methoxyphenyl)propan-1-one (3)
A stirred solution of 7 (34 mg, 0.19 mmol) in EtOAc (1 mL) was treated with 2-iodoxybenzoic acid (78 mg, 0.28 mmol) and the mixture was warmed to 80 °C for 1 h. Once the reaction was completed (after TLC monitoring), the solvent was removed under reduced pressure and the resulting crude was chromatographed, affording 3 (27.4 mg, 81%) as a yellowish solid,26 mp: 69–71 °C (Lit.: 72–73 °C).10 IR (KBr,
): 3420, 2918, 1636, 1458, 1435, 1368, 1317, 1273, 1252, 1227, 1088, 1022, 986, 822, 806, 770, 739, 719 and 625 cm−1. 1H NMR (300 MHz, CDCl3): δ = 7.37 (1H, dd, J = 1.5, 8.0, H-6′), 7.05 (1H, d, J = 8.0, H-4′), 6.84 (1H, t, J = 8.0, H-5′), 3.91 (3H, s, OMe), 3.05 (2H, q, J = 7.4, H-2) and 1.24 (3H, t, J = 7.4, Me). 13C NMR (75 MHz, CDCl3): δ = 207.5 (C-1), 152.8 (C-2′), 149.0 (C-3′), 121.0 (6′), 119.3 (C-4′), 118.2 (C-5′), 116.7 (C-1′), 56.2 (OMe), 31.9 (C-2) and 8.2 (Me). HRMS (ESI+) calcd for C10H13O3 [M+]: 181.0859, found 181.0859.
4.5 Ethyl 8-methoxy-3-methyl-4-oxo-4H-chromene-2-carboxylate (8)
Under an argon atmosphere, anhydrous triethylamine (198 mg, 1.95 mmol) and ethyl chlorooxoacetate (67 mg, 0.49 mmol) were successively added to a stirred solution of 3 (44 mg, 0.24 mmol) in anhydrous CH2Cl2 (1 mL), placed in a microwave vial. The mixture was heated at 100 °C in a microwave oven for 1 h; after its completion was confirmed by TLC, it was cooled to room temperature and diluted with brine (10 mL). The reaction products were extracted with EtOAc (3 × 20 mL). The combined organic extracts were washed with brine (5 mL), dried over MgSO4 and concentrated under reduced pressure. The resulting residue was subjected to chromatography, giving 8 (26 mg, 42%) as a yellowish oil. 1H NMR (300 MHz, CDCl3): δ = 7.75 (1H, dd, J = 1.5, 8.0, H-5), 7.32 (1H, t, J = 8.0, H-6), 7.18 (1H, dd, J = 1.5, 8.0, H-7), 4.49 (2H, q, J = 7.2, CH2Me), 4.01 (3H, s, OMe), 2.37 (3H, s, Me) and 1.46 (3H, t, J = 7.2, CH2Me). 13C NMR (75 MHz, CDCl3): δ = 178.8 (C-4), 161.8 (CO2Et), 148.9 (C-2), 148.7 (C-8), 146.0 (C-8a), 125.1 (C-6), 123.9(C-3), 123.5 (C-4a), 116.7 (C-5), 114.7 (C-7), 62.6 (CH2Me), 56.5 (OMe), 14.1 (CH2Me) and 10.3 (Me-3). HRMS (ESI+) calcd for C14H15O5 [M+]: 263.0914, found 263.0911.
4.6 2-(Hydroxymethyl)-8-methoxy-3-methyl-4H-chromen-4-one (1)
A cold (5 °C) mixture of CaCl2 (13.0 mg, 0.11 mmol) and NaBH4 (3.5 mg, 0.09 mmol) in an EtOH
:
THF (1
:
1 v/v, 500 μL) solvent mixture was added to a stirred solution of 8 (20 mg, 0.08 mmol) in EtOH
:
THF (1
:
1 v/v, 500 μL), placed in an ice-water bath. After 24 h, no starting material was observed by TLC and the reaction was quenched with acetone (1 mL), the solvents were removed under reduced pressure and the crude product was chromatographed, yielding 1 (6.8 mg, 41%) as a yellow solid, mp: 152–154 °C. IR (KBr,
): 3397, 2955, 2916, 2849, 1734, 1717, 1684, 1636, 1609, 1570, 1497, 1458, 1398, 1375, 1362, 1285, 1265, 1225, 1179, 1163, 1115, 1072 and 1020 cm−1. 1H NMR (300 MHz, CDCl3): δ = 7.76 (1H, dd, J = 1.4, 8.0, H-5), 7.29 (1H, t, J = 8.0, H-6), 7.12 (1H, dd, J = 1.4, 8.0, H-7), 4.75 (2H, s, H-1′), 3.98 (3H, s, OMe), 2.65 (1H, bs, w1/2 = 13, OH), and 2.12 (3H, s, Me-3). 13C NMR (75 MHz, CDCl3): δ = 178.3 (C-4), 160.5 (C-2), 148.5 (C-8), 146.2 (C-8a), 124.5 (C-6), 123.6 (C-4a), 117.4 (C-3), 116.8 (C-5), 113.8 (C-7), 60.4 (CH2), 56.2 (OMe) and 9.2 (Me-3).
1H NMR (300 MHz, MeOH-d4): δ = 8.00 (1H, dd, J = 1.5, 7.8, H-5), 7.03 (1H, bs, H-6), 7.02 (1H, dd, J = 1.5, 7.8, H-7), 4.62 (2H, s, H-1′), 3.98 (3H, s, OMe) and 2.07 (3H, s, Me). 13C NMR (75 MHz, MeOH-d4): δ = 180.1 (C-4), 166.0 (C-8a), 164.2 (C-8), 159.4 (C-2), 127.7 (C-6), 118.1 (C-4), 117.3 (C-4a), 116.0 (C-7), 101.0 (C-3), 60.7 (CH2), 56.5 (OMe) and 9.2 (Me-3). The NMR signals of the synthetic compound in MeOH-d4 were in agreement with those of the natural product (compound A).3
4.7 1-(2,3-Dihydroxyphenyl)propan-1-one (4)
A mixture of pyrocatechol (6, 100 mg, 0.91 mmol), BF3OEt (128.9 mg, 0.91 mmol) and propionic acid (807 mg, 10.9 mmol) reacted together under microwave irradiation at 160 °C, without any solvent for a short time. After cooling to room temperature, the reaction mixture was dissolved in dichloromethane (10 mL) and brine (about 20 mL). After extraction of the organic phase, it was washed with aqueous NaHCO3 (20 mL), dried with MgSO4, filtered and evaporated to give a crude product. The crude product was chromatographed to afford 11 (92 mg, 61%), as a yellow solid, mp: 54–56 °C (Lit.: 56–57 °C).16a IR (KBr,
): 3566, 3491, 2978, 2940, 1643, 1636, 1597, 1456, 1452, 1369, 1271, 1109, 1076, 1045, 880, 820 and 731 cm−1. 1H NMR (300 MHz, CDCl3): δ = 7.31 (1H, dd, J = 1.4, 8.2, H-6′), 7.12 (1H, dd, J = 1.4, 7.9, H-4′), 6.81 (1H, t, J = 8.0, H-5′), 5.75 (2H, bs, w1/2 = 76, 2 × OH). 3.04 (2H, q, J = 7.3, CH2) and 1.24 (3H, t, J = 7.3, Me). 13C NMR (75 MHz, CDCl3): δ = 207.7 (C-1), 149.5 (C-2′), 145.5 (C-3′), 120.5 (C-6′), 120.0 (C-4′), 119.1 (C-1′), 118.9 (C-5′), 31.7 (CH2) and 8.2 (Me).
4.8 8-Hydroxy-2,3-dimethyl-4H-chromen-4-one (9)
A solution of 4 (110 mg, 0.66 mmol) in Ac2O (2973 mg, 29.13 mmol) was treated with anhydrous NaOAc (271 mg, 3.31 mmol) and the mixture was heated under reflux for 3 h. The excess Ac2O was distilled under reduced pressure (6 mm Hg), the residue was suspended with AcOEt and the remainder of NaOAc was filtered off under reduced pressure, supplying a mixture of acetates. The acetates were treated with anhydrous Et3N (0.5 mL) and the mixture was heated for 14 h at 115 °C. After that period, the reaction was cooled to room temperature, the solvent was removed under reduced pressure and the residue was treated with 1 M HCl solution at 5 °C (5 mL). The resulting suspension was stirred for 6 h at 40 °C and the white precipitate formed was filtered under reduced pressure and washed with water at 5 °C. Chromatography of the product provided 9 (96.1 mg, 76% overall), as a yellowish solid, mp: 195–197 °C (Lit.: 203–204 °C).27 IR (KBr,
): 3462, 3447, 3416, 1636, 1616, 1578, 1558, 1362, 1269, 1159, 1134, 762 and 619 cm−1. 1H NMR (300 MHz, (CD3)2CO): δ = 9.05 (1H, s, OH), 7.59–7.51 (1H, m H-5), 7.26–7.19 (2H, m, H-6, H-7), 2.45 (3H, s, Me-3) and 2.00 (3H, s, Me-2). 13C NMR (75 MHz, (CD3)2CO3): δ = 177.5 (C-4), 162.3 (C-2), 147.0 (C-8), 146.2 (C-8a), 125.2 (C-6), 124.5 (C-4a), 118.9 (C-7), 117.1 (C-3), 116.1 (C-5), 18.3 (Me-2) and 10.0 (Me-3). HRMS (ESI+) calcd for C11H11O3 [M+]: 191.0703, found 191.0705.
4.9 8-Methoxy-2,3-dimethyl-4H-chromen-4-one (2)
A solution of 9 (69 mg, 0.36 mmol) and K2CO3 (70,2 mg, 0.51 mmol) in anhydrous acetone (1 mL) was heated under reflux and treated with MeI (66.9 mg, 0.47 mmol) for 24 h. Then the solvent was evaporated, brine (10 mL) added, and the product was extracted with EtOAc (3 × 20 mL). The combined extracts were concentrated under reduced pressure and the residue was chromatographed to afford 2 (70 mg, 94%), as a white solid, mp: 149–151 °C (Lit.: 154 °C).17c 1H NMR (300 MHz, CDCl3): δ = 7.72 (1H, dd, J = 1.4, 8.1, H-5), 7.22 (1H, t, J = 8.0, H-6), 7.07 (1H, dd, J = 1.4, 7.9, H-7), 3.95 (3H, s, OMe), 2.43 (3H, s, Me-3) and 2.04 (3H, s, Me-2). 13C NMR (75 MHz, CDCl3): δ = 177.7 (C-4), 161.6 (C-2), 148.3 (C-8a), 146.3 (C-8), 124.1 (C-6), 123.6 (C-4a), 117.0 (C-3), 116.8 (C-5), 113.4 (C-7), 56.2 (OMe), 18.6 (Me-2) and 10.1 (Me-3). HRMS (ESI+) calcd for C12H13O3 [M+]: 205.0859, found 205.0859.
4.10 8-Methoxy-3-methyl-4-oxo-4H-chromene-2-carbaldehyde (10)
A mixture of 2 (52.9 mg, 0.26 mmol), I2 (13.15 mg, 0.10 mmol), TsOH (44.6 mg, 0.26 mmol) in DMSO (1 mL) was heated at 130 °C under oxygen atmosphere for 2 h. After this time, the reaction solution was washed with saturated NH4Cl (5 mL) and extracted with CH2Cl2 (3 × 5 mL), the organic phase was dried with MgSO4, filtered and the remaining DMSO was distilled under reduced pressure evaporated to give a crude product. The crude product was chromatographed to afford 10 (47 mg, 83%), as a yellow solid, mp: 150–152 °C. IR (KBr,
): 3539, 3420, 3233, 1724, 1636, 1622, 1605, 1582, 1493, 1458, 1441, 1406, 1377, 1356, 1271, 1227, 1194, 1159, 1121, 1076, 1001, 918, 824 and 752 cm−1. 1H NMR (300 MHz, CDCl3): δ = 10.20 (1H, s, CHO), 7.73 (1H, dd, J = 1.2, 8.4, H-5), 7.33 (1H, t, J = 8.1, H-6), 7.20 (1H, dd, J = 1.4, 8.0, H-7), 4.01 (3H, s, OMe) and 2.43 (3H, s, Me-2). 13C NMR (75 MHz, CDCl3): δ = 186.5 (C-1′), 179.2 (C-4), 150.5 (C-2), 149.1 (C-8), 145.9 (C-8a), 125.3 (C-6, C-3), 123.6 (C-4a), 116.6 (C-5), 114.9 (C-7), 56.4 (OMe) and 8.2 (Me-2). HRMS (ESI+) calcd for C12H11O4 [M+]: 219.0652, found 219.0652.
4.11 2-(Hydroxymethyl)-8-methoxy-3-methyl-4H-chromen-4-one (1)
To a solution of 13 (24 mg, 0.11 mmol) in EtOH (1 mL) was added NaBH4 (5.0 mg, 0.13 mmol) under an argon atmosphere at 0 °C and stirred the reaction mixture at RT for 1 h. After completion of the reaction, the reaction mixture was quenched with acetone (1 mL). Then, acetone was distilled under reduced pressure evaporated and the crude product was chromatographed to afford chromanone A (1, 17 mg, 70%), as a yellow solid. The NMR spectroscopic data of this compound exhibited concordance with those recorded for compound A3 (Fig. 1) and for 1 when obtained from 5.
4.12 7-Hydroxy-2,3-dimethyl-4H-chromen-4-one (13)
A solution of 2,4-dihydroxypropiophenone (12, 1000 mg, 6.0 mmol) in Ac2O (25 mL, 265 mmol) was treated with anhydrous NaOAc (2468 mg, 30.1 mmol) and the mixture was heated under reflux for 3 h. The excess Ac2O was distilled under reduced pressure (6 mm Hg), the residue was suspended with AcOEt and the remainder of NaOAc was filtered off under reduced pressure, supplying a mixture of acetates. The acetates were treated with anhydrous Et3N (1 mL) and the mixture was heated for 14 h at 115 °C. After that period, the reaction was cooled to room temperature, the solvent was removed under reduced pressure and the residue was treated with 1 M HCl solution at 5 °C (40 mL). The resulting suspension was stirred for 6 h at 40 °C and the precipitate formed was filtered under reduced pressure and washed with water at 5 °C. Chromatography of the product provided 13 (880 mg, 77% overall) as a yellowish solid, mp: 280–282 °C (Lit.: 281 °C).28 IR (KBr,
): 3993, 2924, 2853, 1653, 1558, 1541, 1458, 1246, 1101, 860 and 689 cm−1. 1H NMR (300 MHz, acetone-d6): δ = 9.7 (1H, bs, w1/2 = 42, OH), 7.94 (1H, d, J = 8.7, H-5), 6.81 (1H, dd, J = 2.2, 8.7, H-6), 6.71 (1H, d, J = 2.2, H-8), 2.31 (3H, d, J = 0.6, Me-2) and 1.96 (3H, d, J = 0.6, Me-3). 13C NMR (75 MHz, acetone-d6): δ = 177.4 (C-4), 163.2 (C-7), 162.3 (C-2), 158.5 (C-8a), 127.9 (C-5), 116.6 (C-3), 116.4 (C-4a), 115.3 (C-6), 102.7 (C-8), 18.4 (Me-2) and 9.9 (Me-3).7c
4.13 7-Methoxy-2,3-dimethyl-4H-chromen-4-one (14)
A solution of 13 (800 mg, 4.21 mmol) and K2CO3 (813.8 mg, 5.89 mmol) in anhydrous acetone (20 mL) was heated under reflux and treated with MeI (776 mg, 5.47 mmol) for 24 h. Then the solvent was evaporated, brine added, and the product was extracted with EtOAc. The combined extracts were concentrated under reduced pressure and the residue was chromatographed to afford 14 (795 mg, 93%) as a as a yellowish solid,21 mp: 125–127 (Lit.: 127 °C).29 IR (KBr,
): 3420, 2924, 1636, 1605, 1443, 1404, 1352, 1244, 1204, 1111, 1030, 856, 824 and 689 cm−1. 1H NMR (300 MHz, CDCl3): δ = 8.09 (1H, d, J = 8.8, H-5), 6.92 (1H, dd, J = 2.5, 8.8, H-6), 6.77 (1H, d, J = 2.5, H-8), 3.88 (3H, s, OMe), 2.38 (3H, d, J = 0.5, Me-3) and 2.04 (3H, d, J = 0.5, Me-2). 13C NMR (75 MHz, CDCl3): δ = 177.4 (C-4), 163.5 (C-7), 161.2 (C-2), 157.6 (C-8a), 127.2 (C-5), 116.6 (C-3), 116.6 (C-4a), 113.9 (C-6), 99.7 (C-8), 55.7 (OMe), 18.4 (Me-2) and 10.0 (Me-3).
4.14 7-Methoxy-3-methyl-4-oxo-4H-chromene-2-carbaldehyde (15)
A mixture of 14 (20.4 mg, 0.10 mmol), I2 (5.07 mg, 0.04 mmol) and TsOH (17.2 mg, 0.10 mmol) was heated at 130 °C under an oxygen atmosphere for 3 h in DMSO (1 mL). After this time, the reaction was diluted with saturated NH4Cl solution (10 mL) and the product was extracted with CH2Cl2 (3 × 10 mL). The combined organic phases were dried with MgSO4, and concentrated under reduced pressure. The remaining DMSO was distilled under vacuum and the crude product was chromatographed to afford 15 (17 mg, 78%) as a yellowish solid,22 mp: 118–121 °C. IR (KBr,
): 2920, 2849, 1699, 1624, 1443, 1281, 1261, 1206, 1159, 1111, 1020, 851, 768 and 623 cm−1. 1H NMR (300 MHz, CDCl3): δ = 10.20 (1H, s, CHO), 8.11 (1H, d, J = 8.9, H-5), 6.99 (1H, dd, J = 2.4, 8.9, H-6), 6.93 (1H, d, J = 2.4, H-8), 3.92 (3H, s, OMe) and 2.44 (3H, s, Me-3). 13C NMR (75 MHz, CDCl3): δ = 185.7 (C-1′), 178.3 (C-4), 165.1 (C-7), 157.2 (C-8a), 150.6 (C-2), 127.4 (C-5), 126.6 (C-3), 116.8 (C-4a), 115.7 (C-6), 99.9 (C-8), 55.9 (OMe) and 8.0 (Me-3).
4.15 2-(Hydroxymethyl)-7-methoxy-3-methyl-4H-chromen-4-one (16)
A stirred solution of 13 (10.5 mg, 0.05 mmol) in EtOH (1 mL) under an argon atmosphere was cooled to 0 °C and treated with NaBH4 (2.73 mg, 0.07 mmol). The reaction mixture was further stirred at RT for 1 h, when it was quenched with acetone (1 mL). The volatiles were removed under reduced pressure and the crude product was chromatographed to afford 13 (7.5 mg, 71%), as a white solid, mp: 122–125 °C. IR (KBr,
): 3323, 2924, 2853, 1638, 1597, 1449, 1244, 1177, 1042, 1024 and 827 cm−1. 1H NMR (300 MHz, CDCl3): δ = 8.06 (1H, d, J = 8.9, H-5), 6.92 (1H, dd, J = 2.4, 8.9, H-6), 6.74 (1H, d, J = 2.4, H-8), 4.67 (2H, s, H-1′), 3.87 (3H, s, OMe), 2.75 (1H, bs, w1/2 = 16, OH) and 2.07 (3H, s, Me-3). 13C NMR (75 MHz, CDCl3): δ = 178.0 (C-4), 163.9 (C-7), 160.5 (C-2), 157.5 (C-8a), 127.2 (C-5), 117.3 (C-3), 116.4 (C-4a), 114.5 (C-6), 99.6 (C-8), 60.5 (C-1′), 55.7 (OMe) and 9.2 (Me-3). The spectroscopic data of this compound were in full agreement with those recorded for the natural product (compound C).4b
Author contributions
All the authors were involved in the manuscript, performing conceptualization (IC, TSK, ABJB, LAS), data curation (IC, MAS, LAS), formal analysis (IC, EC, TSK, ABJB), funding acquisition (TSK, ABJB, MAS, LAS), investigation (IC, EC, TSK, ABJB), methodology (IC, EC, TSK, LAS, MAS, ABJB), project administration (TSK, ABJB, LAS, MAS), resources (TSK, ABJB, LAS, MAS), use of software (IC, TSK, ABJB), supervision (ABJB, TSK, MAS, LAS), results validation (TSK), results visualization (IC, EC, TSK), writing – original draft (IC, EC, TSK, ABJB) and writing – review & editing (IC, EC, TSK, LAS, MAS, ABJB).
Conflicts of interest
There are no conflicts to declare.
Acknowledgements
The authors gratefully acknowledge Consejo Nacional de Investigaciones Científicas y Técnicas (CONICET, PUE IQUIR 2016) and Agencia Nacional de Promoción Científica y Tecnológica (ANPCyT, PICT 2017-0149 and PICT 2016-1833) for financial support. IC and EC also thank CONICET for their Doctoral fellowships.
References
-
(a) P. S. Stewart and M. J. Franklin, Nat. Rev. Microbiol., 2008, 6, 199–210 CrossRef CAS PubMed
;
(b) J. W. Costerton, P. S. Stewart and E. P. Greenberg, Science, 1999, 284, 1318–1322 CrossRef CAS PubMed
. -
(a) L. Yet, Privileged Structures in Drug Discovery, Wiley, Hoboken, USA, 2018 Search PubMed
;
(b) D. S. Gül, H. Ogutcu and Z. Hayvali, J. Mol. Struct., 2020, 1204, 127569 CrossRef
;
(c) A. Mendieta-Moctezuma, C. Rugerio-Escalona, N. Villa-Ruano, R. U. Gutierrez, F. E. Jiménez-Montejo, M. J. Fragoso-Vázquez, J. Correa-Basurto, M. C. Cruz-López, F. Delgado and J. Tamariz, Med. Chem. Res., 2019, 28, 831–848 CrossRef CAS
. - A. M. Gamal-Eldeen, A. Abdel-Lateff and T. Okino, Environ. Toxicol. Pharmacol., 2009, 28, 317–322 CrossRef CAS PubMed
. -
(a) T. Tanahashi, Y. Takenaka and N. Hamada, Heterocycles, 2011, 83, 2157–2164 CrossRef
;
(b) M.-F. Li, G.-H. Li and K.-Q. Zhang, Metabolites, 2019, 9, 58 CrossRef CAS PubMed
;
(c) L.-H. Menga, H.-Q. Chen, I. Form, B. Konuklugil, P. Proksch and B.-G. Wang, Nat. Prod. Commun., 2016, 11, 1293–1296 Search PubMed
. -
(a) T. M. L. Do, A. V. Truong, T. N. Vo, T. G. Pinnock, L. M. Pratt, D. Guillaume and K. P. P. Nguyen, Phytochem. Lett., 2013, 6, 544–551 CrossRef CAS
;
(b) W. Yi-Fen, C. Ji-Jun, Y. Yan, Z. Yong-Tang, T. Shao-Zong and L. Shi-De, Helv. Chim. Acta, 2002, 85, 2342–2348 CrossRef
. -
(a) A. A. Arroyo Aguilar, S. J. Bolívar Avila, T. S. Kaufman and E. L. Larghi, Org. Lett., 2018, 20, 5058–5061 CrossRef CAS PubMed
;
(b) J. L. Pergomet, A. B. J. Bracca and T. S. Kaufman, Org. Biomol. Chem., 2017, 15, 7040–7049 RSC
;
(c) M. V. Méndez, D. A. Heredia, E. L. Larghi, A. B. J. Bracca and T. S. Kaufman, RSC Adv., 2017, 7, 28298–28307 RSC
;
(d) M. V. Méndez, S. O. Simonetti, T. S. Kaufman and A. B. J. Bracca, New J. Chem., 2019, 43, 10803–10813 RSC
. -
(a) I. Cortés, C. Borini Etichetti, J. Girardini, T. S. Kaufman and A. B. J. Bracca, Synthesis, 2019, 51, 433–440 CrossRef
;
(b) J. L. Pergomet, M. G. Di Liberto, M. G. Derita, A. B. J. Bracca and T. S. Kaufman, Fitoterapia, 2018, 125, 98–105 CrossRef CAS PubMed
;
(c) S. O. Simonetti, E. L. Larghi, A. B. J. Bracca and T. S. Kaufman, Org. Biomol. Chem., 2012, 10, 4124–4134 RSC
. -
(a) F. Saito, K. Kuramochi, A. Nakazaki, Y. Mizushina, F. Sugawara and S. Kobayashi, Eur. J. Org. Chem., 2006, 4796–4799 CrossRef CAS
;
(b) W. A. König, H. Faasch, H. Heitsch, C. Colberg and B. M. Hausen, Z. Naturforsch., B: J. Chem. Sci., 1993, 48, 387–393 Search PubMed
;
(c) L. A. Hasvold, L. Hexamer, G. Li, N.-H. Lin, H. Sham, T. Sowin, G. M. Sullivan, L. Wang and P. X. Xia, WO2004/076424A1, 2004
;
(d) J. Halbrook, E. A. Kesicki, L. E. Burgess, S. T. Schlachter, C. T. Eary, J. G. Schiro, H. Huang, M. Evans and Y. Han, US Pat., US2002/165218A1, 2002 Search PubMed
. -
(a) R. Frenette, M. Monette, M. A. Bernstein, R. N. Young and T. R. Verhoeven, J. Org. Chem., 1991, 56, 3083–3089 CrossRef CAS
;
(b) S. Ducki, R. Forrest, J. A. Hadfield, A. Kendall, N. J. Lawrence, A. T. McGown and D. Rennison, Bioorg. Med. Chem. Lett., 1998, 8, 1051–1056 CrossRef CAS PubMed
;
(c) P. Gogoi, G. K. Sarmah and D. Konwar, J. Org. Chem., 2004, 69, 5153–5154 CrossRef CAS PubMed
. - K. A. Parker and J. J. Petraitis, Tetrahedron Lett., 1981, 22, 397–400 CrossRef CAS
. -
(a) S. Gobbi, Q. Hu, C. Zimmer, M. Engel, F. Belluti, A. Rampa, R. W. Hartmann and A. Bisi, J. Med. Chem., 2016, 59, 2468–2477 CrossRef CAS PubMed
;
(b) M. Kumar, P. Rawat, J. Kureel, A. K. Singh, D. Singh and R. Maurya, Bioorg. Med. Chem. Lett., 2011, 21(2011), 1706–1709 CrossRef CAS PubMed
. -
(a) K. S. Lee, S. H. Seo, Y. H. Lee, H. D. Kim, M. H. Son, B. Y. Chung, J. Y. Lee, C. Jin and Y. S. Lee, Bioorg. Med. Chem. Lett., 2005, 15, 2857–2860 CrossRef CAS PubMed
;
(b) S. H. Kim, Y. H. Lee, S. Y. Jung, H. J. Kim, C. Jin and Y. S. Lee, Eur. J. Med. Chem., 2011, 46, 1721–1728 CrossRef CAS PubMed
;
(c) F. M. Hauser and W. A. Dorsch, Org. Lett., 2003, 5, 3753–3754 CrossRef CAS PubMed
. -
(a) A. M. Helguera, A. Pérez-Garrido, A. Gaspar, J. Reis, F. Cagide, D. Vina, M. N. D. S. Cordeiro and F. Borges, Eur. J. Med. Chem., 2013, 59, 75–90 CrossRef CAS PubMed
;
(b) M. Payard, G. Mouysset, P. Tronche and P. Bastide, Eur. J. Med. Chem., 1985, 20, 117–120 CAS
;
(c) G. E. Daia, C. D. Gabbutt, J. D. Hepworth, B. M. Heron, D. E. Hibbs and M. B. Hursthouse, Tetrahedron Lett., 2002, 43, 4507–4510 CrossRef CAS
. -
(a) J. Bolós, L. Anglada, S. Gubert, J. M. Planas, J. Agut, M. Príncep, A. de la Fuente, A. Sacristán and J. A. Ortiz, J. Med. Chem., 1998, 41, 5402–5409 CrossRef PubMed
;
(b) J. H. Hutchinson and M. W. Rowbottom, WO2017/15221A1, 2017
;
(c) S. Narasimhan, K. G. Prasad and S. Madhavan, Synth. Commun., 1995, 25, 1689–1697 CrossRef CAS
. -
(a) H. Naeimi and L. Moradi, Bull. Chem. Soc. Jpn., 2005, 78, 284–287 CrossRef CAS
;
(b) N. Zhang, Z. Yu, X. Yang, Y. Zhou, J. Wang, S.-L. Zhang, M.-W. Wang and Y. He, Eur. J. Med. Chem., 2018, 158, 707–719 CrossRef CAS PubMed
;
(c) K. Wahala and T. A. Hase, J. Chem. Soc., Perkin Trans. 1, 1991, 3005–3008 RSC
;
(d) S.-C. Kuo, K.-H. Lee, L.-J. Huang, L.-C. Chou, T.-S. Wu, T.-D. Way, J.-G. Chung, J.-S. Yang, C.-H. Huang and M.-T. Tsai, US Pat., US2012/15908A1, 2012 Search PubMed
. -
(a) M. Albrecht, S. Mirtschin, M. de Groot, I. Janser, J. Runsink, G. Raabe, M. Kogej, C. A. Schalley and R. Fröhlich, J. Am. Chem. Soc., 2005, 127, 10371–10387 CrossRef CAS PubMed
;
(b) A. C. N. Kwamen, J. Jenniches, I. M. Oppel and M. Albrecht, Chem.–Eur. J., 2020, 26, 10550–10554 CrossRef CAS PubMed
. -
(a) X. Wang, T. Zhao, B. Yang, Z. Li, J. Cui, Y. Dai, Q. Qiu, H. Qiang, W. Huang and H. Qian, Bioorg. Med. Chem., 2015, 23, 132–140 CrossRef PubMed
;
(b) J. E. Immoos, J. Labelled Compd. Radiopharm., 2015, 58, 419–424 CrossRef CAS PubMed
;
(c) I. M. Helibron, H. Barnes and R. A. Morton, J. Chem. Soc., Trans., 1923, 123, 2559–2570 RSC
. -
(a) R. Ye, Y. Cao, X. Xi, L. Liu and T. Chen, Org. Biomol. Chem., 2019, 17, 4220–4224 RSC
;
(b) M. Raghavender Reddy, N. Nageswara Rao, K. Ramakrishna and H. M. Meshram, Tetrahedron Lett., 2014, 55, 1898–1901 CrossRef CAS
;
(c) Q. Cai, S. Zhuang, M. Yang, N. Peng, Y. Liu and A. Wu, Tetrahedron, 2019, 75, 130756 CrossRef
. - R. B. Gammill, J. Org. Chem., 1984, 49, 5035–5041 CrossRef CAS
. -
(a) G. Höfle, B. Kunze, C. Zorzin and H. Reichenbach, Liebigs Ann. Chem., 1984, 1883–1904 CrossRef
;
(b) N. R. Ayyangar, R. A. Khan and V. H. Deshpande, Tetrahedron Lett., 1988, 29, 2347–2348 CrossRef CAS
. - M. Yu, Y. Li, S. P. Banakar, L. Liu, C. Shao, Z. Li and C. Wang, Front. Microbiol., 2019, 10, 915 CrossRef PubMed
. - A. Srinivas, Y. Hemasri and R. Y. Jayaprakash, Heterocycl. Lett., 2018, 8, 303–311 CAS
. - Reference method for broth dilution antifungal susceptibility testing of yeasts, 4th edn, approved standard CLSI M27-Ed4, Clinical and Laboratory Standard Institute, Wayne, PA, USA, 2017 Search PubMed.
- J. C. O. Sardi, L. Scorzoni, T. Bernardi, A. M. Fusco-Almeida and M. J. S. Mendes Giannini, J. Med. Microbiol., 2013, 62, 10–24 CrossRef CAS PubMed
. - C. G. Pierce, P. Uppuluri, A. R. Tristan, F. L. Wormley, E. Mowat, G. Ramage and J. L. Lopez-Ribot, Nat. Protoc., 2008, 3, 1494–1500 CrossRef CAS PubMed
. - T. T. Kung, Y. Crawley, B. Luo, S. Young, W. Kreutner and R. W. Chapman, Br. J. Pharmacol., 2000, 130, 457–463 CrossRef CAS PubMed
. - P. Da Re and L. Cimatoribus, Chem. Ber., 1962, 95, 2912–2920 CrossRef CAS
. - Y. J. Rao and G. L. D. Krupadanam, Bull. Chem. Soc. Jpn., 1994, 67, 1972–1975 CrossRef CAS
. - F. W. Canter, F. H. Curd and A. Robertson, J. Chem. Soc. C, 1931, 1255–1265 RSC
.
Footnote |
† Electronic supplementary information (ESI) available: NMR spectra of the intermediates and final products, bioactivity testing methodology and results of inhibition assays. See DOI: 10.1039/d1ra02553h |
|
This journal is © The Royal Society of Chemistry 2021 |
Click here to see how this site uses Cookies. View our privacy policy here.