DOI:
10.1039/D1RA02515E
(Paper)
RSC Adv., 2021,
11, 25995-26007
Novel nano-architectured carbon quantum dots (CQDs) with phosphorous acid tags as an efficient catalyst for the synthesis of multisubstituted 4H-pyran with indole moieties under mild conditions†
Received
30th March 2021
, Accepted 28th June 2021
First published on 27th July 2021
Abstract
In this work, a new nano-structured catalyst with phosphorus acid moieties, synthesized by the reaction of carbon quantum dots (CQDs) and phosphorus acid under refluxing EtOH. The structure and morphology of CQDs–N(CH2PO3H2)2 were fully characterized using various techniques such as Fourier transform infrared (FT-IR) spectroscopy, transmission electron microscopy (TEM), scanning electron microscopy (SEM), energy dispersive X-ray (EDX) spectroscopy, thermogravimetric (TG) analysis, fluorescence and X-ray diffraction (XRD) measurements. The new CQDs–N(CH2PO3H2)2 catalyst was successfully used for the synthesis of 2-amino-6-(2-methyl-1H-indol-3-yl)-4-phenyl-4H-pyran-3,5-dicarbonitriles by the one-pot reaction of various aromatic aldehydes, 3-(1H-indol-3-yl)-3-oxopropanenitrile derivatives and malononitrile in refluxing EtOH and/or ultrasonic irradiation conditions.
1. Introduction
Carbon quantum dots (CQDs) have recently attracted comprehensive research interest due to their various physicochemical properties and favorable features such as biocompatibility, distinctive optical properties and low cost.1–6 In 2004, Xu et al. developed carbon quantum dots (CQDs) during the purification of single-walled carbon nanotubes via a preparative electrophoresis methodology.7 Carbon quantum dots (CQDs) have been used in many interesting fields of research such as bioimaging, biosensing, catalysis, heavy metal element sensing and biomolecule/drug delivery.8–13 Also, these nanomaterials show intense photoluminescence (PL), arising from quantum-confinement effects.14 Materials with phosphorous acid tags have been introduced as catalysts, adsorbents, inhibitors and extractants.15 Recently a wide variety of solid acid catalysts with phosphorous acid functional groups, such as glycoluril,16 SBA-15,17 a melamine-based nano catalyst,18 metal–organic frameworks (MOFs)19–21 and uric acid,22 have been reported for the synthesis of organic compounds.
Organic compounds with indole scaffolds have diverse biological and pharmacological applications such as antifungal, optimal inhibitory, anticholinergic, antihypertensive, antibacterial, antiviral, cardiovascular, anticonvulsant and antiproliferative activities.23–30 Also, several natural products and pharmaceutically important compounds which act as antitumor, anticancer, anti-inflammatory, hypoglycemic, antipyretic or analgesic reagents have indole scaffolds in their structures.31 Additionally, 4H-pyran derivative structures are an important category of heterocyclic compounds due to their biological properties, such as their anticoagulant, anticancer, antioxidant, spasmolytic, diuretic and anti-anaphylactic activities (Fig. 1).32–36 2-Amino-4H-pyran derivatives have also been applied as photoactive materials, cosmetics and pigments.37 Ultrasonic irradiation has been widely applied for the preparation of organic compounds with biological activity. On the other hand, ultrasonic irradiation as an efficient strategy for the preparation of materials in chemical synthesis has received great attention in chemical processes.38,39 One of the major advantages of ultrasonic irradiation is the controllability of the time and energy power.
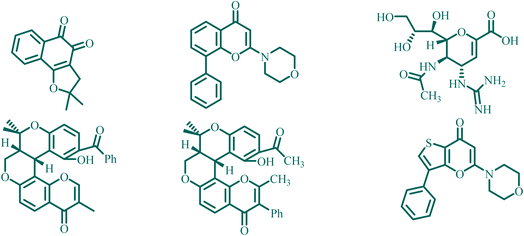 |
| Fig. 1 Structure of pyran compounds with biological properties. | |
On the basis of the above-mentioned facts, the synthesis of 4H-pyran-3,5-dicarbonitrile and pyridines with indole moieties in the presence of reusable solid acid catalysts is our main research interest. With this aim, CQDs-N(CH2PO3H2)2, as a novel carbon quantum dot (CQD) nano-catalyst structure with phosphorus acid tags, was synthesized, characterized and used in the synthesis of 4H-pyran-3,5-dicarbonitrile with indole moieties, both in refluxing EtOH and ultrasonic irradiation in EtOH as a solvent (Scheme 1).
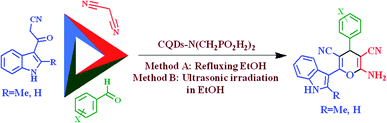 |
| Scheme 1 Synthesis of 4H-pyran-3,5-dicarbonitrile with indole moieties using CQDs-N(CH2PO3H2)2. | |
2. Experimental
2.1. General procedure for the preparation of CQDs–N(CH2PO3H2)2
Initially, carbon quantum dots (CQDs) were synthesized by adding citric acid (1.05 g, 5.5 mmol), ethane-1,2-diamine (5 mmol, 0.33 mL) and 10 mL H2O under ultrasonic irritation for 30 min.40 Then, this mixture was kept in a Teflon-lined stainless-steel autoclave at 200 °C for 6 h. After the reaction was completed, a dark precipitate appeared, which was filtered by centrifugation (1000 rpm, 20 min). The carbon quantum dots (CQDs) were dried under vacuum. Then, in a 25 mL round-bottomed flask connected to a reflux condenser, carbon quantum dots (CQDs) (0.5 g), paraformaldehyde (4 mmol, 0.12 g), phosphorous acid (2 mmol, 0.164 g), p-TSA (0.01 g) and ethanol (10 mL) were added and refluxed for 8 hours. A white solid appeared, which was filtered by centrifugation (1000 rpm, 10 min). The obtained CQDs–N(CH2PO3H2)2 was dried under vacuum (Scheme 2).
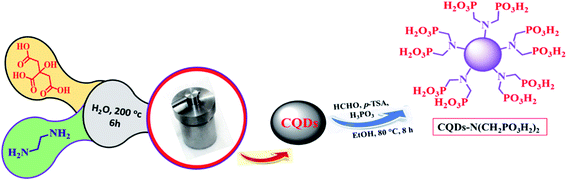 |
| Scheme 2 Preparation of CQDs–N(CH2PO3H2)2 as the desired catalyst. | |
2.2. General procedure for the synthesis of 2-amino-6-(2-methyl-1H-indol-3-yl)-4-phenyl-4H-pyran-3,5-dicarbonitrile using CQDs–N(CH2PO3H2)2 as a catalyst
In a 20 mL round-bottomed flask, a mixture of aldehyde (1 mmol), 3-(1H-indol-3-yl)-3-oxopropanenitrile derivatives (1 mmol), malononitrile (1.1 mmol, 0.073 g), CQDs–N(CH2PO3H2)2(10 mg) as a catalyst and EtOH (10 mL) was stirred under reflux conditions (method A) or under ultrasonic irradiation (method B). After the completion of the reaction (monitored by TLC n-hexane/ethyl acetate; 7
:
3), PEG (10 mL) was added to the mixture and the catalyst was separated by centrifugation (1000 rpm, 10 min). Finally, the mixture was poured into H2O and the precipitate was filtered off. The obtained residue was washed with warm ethanol and dried at 100 °C (Scheme 1).
3. Result and discussion
3.1. Synthesis and characterization of the catalyst with phosphorous acid tags
Since molecules with indole moieties have been considered as candidates with biological interest41 and we have conducted a literature survey for publishing a comprehensive review on the subject of bis and tris indolyl methanes,42 we decided to report a catalytic methodology for the synthesis of multisubstituted 4H-pyran with indole moieties (Schemes 1 and 2). Here, 2-amino-6-(2-methyl-1H-indol-3-yl)-4-phenyl-4H-pyran-3,5-dicarbonitriles were successfully synthesized in the presence of CQDs–N(CH2PO3H2)2, various aromatic aldehydes, 3-(1H-indol-3-yl)-3-oxopropanenitrile derivatives and malononitrile both in refluxing EtOH and/or ultrasonic irradiation conditions.
At first, the desired catalyst CQDs–N(CH2PO3H2)2 was prepared according to Scheme 2. This novel nano-structure catalyst was fully characterized by applying FT-IR spectroscopy, XRD spectroscopy, FE-SEM, energy dispersive X-ray spectroscopy (EDS), transmission electron microscopy (TEM), TG and fluorescence analysis.
The FT-IR spectra of carbon quantum dots (CQDs) and CQDs–N(CH2PO3H2)2 are compared in Fig. 2. The broad peak at 2600–3500 cm−1 is related to the OH of the PO3H2 functional groups. The absorption bands at 1015 and 1050 cm−1 are related to P–O bond stretching and the band at 1128 cm−1 is related to P
O. The differences between the FT-IR spectra of the carbon quantum dots (CQDs) and CQDs-N(CH2PO3H2)2 verified the structure of the catalyst.
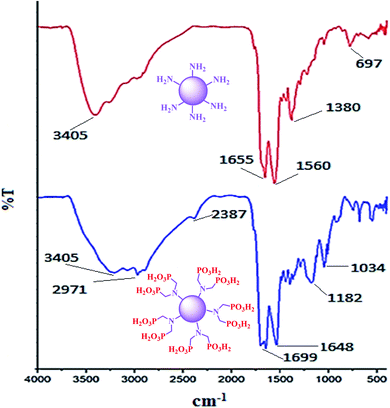 |
| Fig. 2 Comparison of the FT-IR spectra of the CQDs and CQDs–N(CH2PO3H2)2. | |
Following this, the fluorescence features of the initial CQDs and functionalized CQDs were investigated for the evaluation of the synthesized CQDs and the effect of post-functionalization on the surface of the final catalyst. As can be seen in Fig. 3, the initial synthesized CQDs display a high-intensity emission peak at 450 nm (peak a). But the fluorescence intensity of the functionalized CQDs with PO3H2 groups is decreased and shifted towards longer wavelengths (the red shift effect). Many factors are effective for amplifying or attenuating the fluorescence intensity by affecting the resonance system, including structural rigidity, steric effect interactions, temperature, solvent, pH, the presence of para-magnetite species, heavy atoms and electron donor/acceptor groups. It can be proposed that the presence of larger PO3H2 groups instead of the smaller proton groups (Scheme 1) can have a negative effect on the fluorescence intensity due to the steric effects. In the other words, the presence of the large PO3H2 groups, along with the possible rotation of these groups, reduces the symmetry of the molecule. So, increasing the mobility of the molecule will reduce the fluorescence intensity of the functionalized CQDs due to the decreased rigidity and disturbance of the conjugated system. The decreasing fluorescence intensity of the functionalized CQDs compared to that of the initial CQDs can be a reason for the stabilization of PO3H2 functional groups on the initial CQD surface. Fig. 3 shows photographic images of the decreased fluorescence intensity of the initial CQDs after post-modification with the PO3H2 groups.
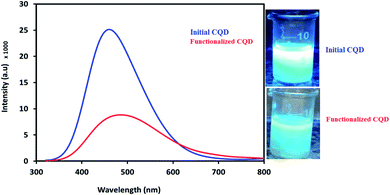 |
| Fig. 3 Fluorescence spectra of the initial CQDs and functionalized CQDs. | |
The XRD pattern for CQDs–N(CH2PO3H2)2 is shown in the region of 2θ = 5–80° (Fig. 4). The broad peak of CQDs-N(CH2PO3H2)2 corresponds to the diffraction lines in previously reported literature.43 Therefore, the structure and morphology of the carbon quantum dots (CQDs) is stable after functionalization with phosphorous acid groups.
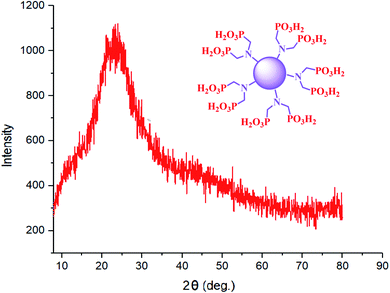 |
| Fig. 4 X-ray diffraction (XRD) pattern of CQDs functionalized with PO3H2 groups. | |
The elements that CQDs–N(CH2PO3H2)2 was composed of were also studied with energy dispersive X-ray (EDX) analysis (Fig. 5). The structure of the catalyst was verified by the existence of N, C, O and P atoms.
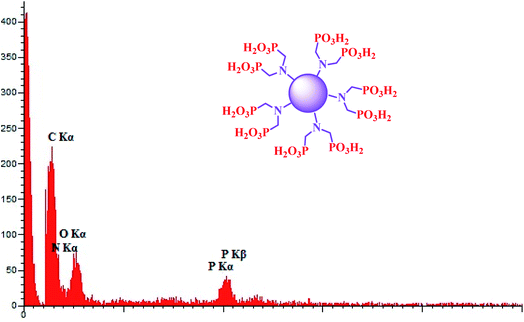 |
| Fig. 5 Energy dispersive X-ray (EDX) analysis of CQDs–N(CH2PO3H2)2. | |
The morphology and particle size of CQDs–N(CH2PO3H2)2 were also studied from the scanning electron microscopy (SEM) (Fig. 6a and b) and transmission electron microscopy (TEM) images (Fig. 7). The scanning electron microscopy (SEM) of CQDs–NH2 (Fig. 6a) and CQDs–N(CH2PO3H2)2 (Fig. 6b) did not show different morphologies of these materials. As shown in the transmission electron microscopy images (Fig. 7), nanoparticles of CQDs-N(CH2PO3H2)2 are approximately 5–15 nm with a narrow size, which are regularly arranged and not completely stacked.
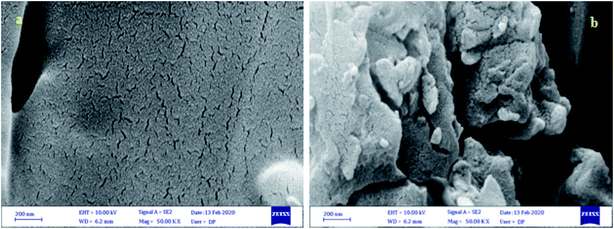 |
| Fig. 6 Scanning electron microscopy (SEM) images of CQDs–NH2 (a) and CQDs–N(CH2PO3H2)2 (b). | |
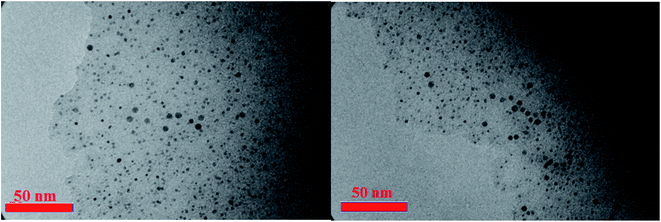 |
| Fig. 7 Transmission electron microcopy (TEM) images of CQDs–N(CH2PO3H2)2. | |
The thermal gravimetric (TG) analysis results for CQDs–N(CH2PO3H2)2 are shown in Fig. 8. Two declining stages were observed for CQDs–N(CH2PO3H2)2 in Fig. 8. The first weight loss (which includes about 5% weight loss) was related to the evaporation of the solvents (organic and water). The second weight loss is at 400 °C (includes about 40% weight loss), which is linked to the breaking of the bonds of N–C–PO3H2 of the structure of CQDs–N(CH2PO3H2)2.
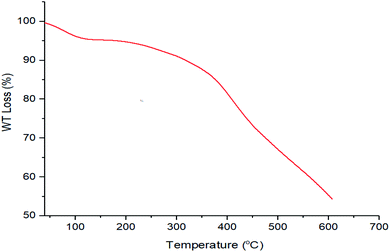 |
| Fig. 8 Thermal gravimetric (TG) analysis of CQDs–N(CH2PO3H2)2. | |
3.2. Catalytic properties of the catalyst with phosphorous acid tags
After the synthesis and characterization of CQDs–N(CH2PO3H2)2, it was applied for the synthesis of 2-amino-6-(2-methyl-1H-indol-3-yl)-4-phenyl-4H-pyran-3,5-dicarbonitrile derivatives with indole and pyran moieties. The above-mentioned products were obtained by the reaction of 4-chloro-benzaldehyde (1 mmol, 0.14 g), 3-(1H-indol-3-yl)-3-oxopropanenitrile (1 mmol, 0.184 g) and malononitrile (1.1 mmol, 0.073 g) as a model for the optimization of the reaction conditions. The optimization data is listed in Table 1. As shown in Table 1, the best synthesis of 2-amino-4-(4-chlorophenyl)-6-(1H-indol-3-yl)-4H-pyran-3,5-dicarbonitrile was achieved in the presence of 10 mg CQDs–N(CH2PO3H2)2 in EtOH (5 mL) as the solvent (entry 1, Table 1). The model reaction was also studied using several solvents such as H2O, CH3CN, n-hexane, CHCl3, toluene, MeOH, DMF, EtOH, CH2Cl2 and EtOAc (5 mL), as well as a solvent-free condition, in the presence of 10 mg of CQDs–N(CH2PO3H2)2. The results of the reaction show that the yield and time were not improved when using other amounts of catalyst (Table 1, entries 16–18).
Table 1 Effects of different amounts of catalyst, temperature and solvent (5 mL) on the synthesis of 2-amino-4-(4-chlorophenyl)-6-(1H-indol-3-yl)-4H-pyran-3,5-dicarbonitrile
Entry |
Solvent |
Catalyst (mg) |
Temp. (°C) |
Time (min) |
Yield (%) |
1 |
EtOH |
10 |
Reflux |
25 |
89 |
2 |
EtOH |
10 |
50 |
45 |
55 |
3 |
EtOH |
10 |
25 |
80 |
30 |
4 |
EtOH |
5 |
Reflux |
35 |
70 |
5 |
EtOH |
20 |
Reflux |
30 |
75 |
6 |
EtOH |
— |
Reflux |
120 |
25 |
7 |
DMF |
10 |
100 |
90 |
60 |
8 |
H2O |
10 |
Reflux |
120 |
— |
9 |
CH3CN |
10 |
Reflux |
120 |
Trace |
10 |
n-hexane |
10 |
Reflux |
120 |
— |
11 |
CHCl3 |
10 |
Reflux |
100 |
50 |
12 |
Toluene |
10 |
Reflux |
120 |
— |
13 |
MeOH |
10 |
Reflux |
50 |
60 |
14 |
CH2Cl2 |
10 |
Reflux |
50 |
35 |
15 |
EtOAc |
10 |
Reflux |
120 |
— |
16 |
— |
10 |
100 |
30 |
60 |
17 |
— |
10 |
25 |
120 |
35 |
18 |
— |
10 |
50 |
50 |
45 |
After optimizing the reaction conditions, CQDs–N(CH2PO3H2)2(10 mg) was applied to synthesise a good range of desired compounds using various aromatic aldehydes bearing electron-donating groups, electron-withdrawing groups and heterocycles, as well as malononitrile and 3-(1H-indol-3-yl)-3-oxopropanenitrile derivatives, both in refluxing EtOH or ultrasonic irradiation conditions (methods A and B, respectively). As shown in Table 2 the obtained results indicated that CQDs–N(CH2PO3H2)2 is appropriate for the preparation of target molecules in high to excellent yields with short reaction times (methods A and B).
Table 2 Synthesis of 2-amino-4-(4-chlorophenyl)-6-(1H-indol-3-yl)-4H-pyran-3,5-dicarbonitrile derivatives using CQDs–N(CH2PO3H2)2 under refluxing EtOH (method A) and ultrasonic irradiation (method B)
In the proposed mechanism, the aldehyde is activated with a proton of the acidic functional groups of CQDs–N(CH2PO3H2)2 and intermediate (I) is prepared by the reaction of malononitrile with the loss of one molecule of H2O. In the second step, 3-(1H-indol-3-yl)-3-oxopropanenitrile reacts with intermediate (I) to give intermediate (II) after tautomerization. Then, intermediate (II) gives the desired product after intramolecular cyclization and the loss of another molecule of H2O (Scheme 3).
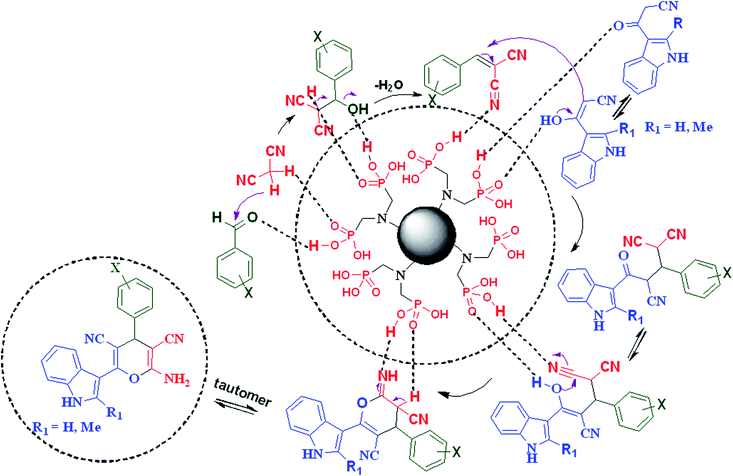 |
| Scheme 3 Proposed mechanism for the synthesis of 2-amino-4-(4-chlorophenyl)-6-(1H-indol-3-yl)-4H-pyran-3,5-dicarbonitrile. | |
To compare the efficiency of the described CQDs–N(CH2PO3H2)2 for the synthesis of 2-amino-6-(2-methyl-1H-indol-3-yl)-4-phenyl-4H-pyran-3,5-dicarbonitrile that was achieved in the presence of 10 mg of the catalyst by the reaction of 4-chloro-benzaldehyde (1 mmol, 0.14 g), 3-(1H-indol-3-yl)-3-oxopropanenitrile (1 mmol, 0.184 g) and malononitrile (1.1 mmol, 0.073 g) under the above-mentioned optimized reaction conditions, various organic and inorganic solid acid catalysts for the above reaction were tested (Table 3). As Table 3 indicates, CQDs–N(CH2PO3H2)2 is the best choice for the synthesis of 2-amino-6-(2-methyl-1H-indol-3-yl)-4-phenyl-4H-pyran-3,5-dicarbonitrile derivatives; due to the shorter reaction times, higher yields and amount of applied catalyst. Also, the reusability of CQDs–N(CH2PO3H2)2 as a catalyst for the preparation of 2-amino-6-(2-methyl-1H-indol-3-yl)-4-phenyl-4H-pyran-3,5-dicarbonitrile derivatives was examined in the above reaction. The results show that the catalyst has the potential to be recycled and reused up to 7 times without a significant decrease in its catalytic activity (Fig. 9).CQDs–N(CH2PO3H2)2 was also characterized by FT-IR and energy dispersive X-ray analysis (EDX) analysis after its application in the reaction. These spectra were same as those of the fresh catalyst (Fig. 10 and 11).
Table 3 Synthesis of 2-amino-4-(4-chlorophenyl)-6-(1H-indol-3-yl)-4H-pyran-3,5-dicarbonitrile the presence of various catalysts using method A
Entry |
Catalyst |
(mol %) |
Time (min) |
Yield (%) |
1 |
FeCl3 |
10 |
80 |
25 |
2 |
H2SO4 |
10 |
120 |
20 |
3 |
Fe3O4 |
10 mg |
120 |
Trace |
4 |
NH4NO3 |
10 |
90 |
25 |
5 |
CF3SO3H |
10 |
70 |
35 |
6 |
GTBSA47 |
10 |
120 |
15 |
7 |
MIL-100(Cr)/NHEtN(CH2PO3H2)2 (ref. 21) |
10 |
80 |
60 |
8 |
H3[p(W3O10)4]·XH2O |
10 |
120 |
Trace |
9 |
SBA-15/(CH2)3N(CH2PO3H2) (CH2)2–N(CH2PO3H2)2 (ref. 17b) |
10 |
90 |
62 |
10 |
[PVI-SO3H]FeCl4 (ref. 48) |
10 |
100 |
48 |
11 |
p-TSA |
10 |
120 |
48 |
12 |
SSA49 |
10 mg |
110 |
50 |
13 |
Et3N |
10 |
120 |
— |
14 |
MHMHPA18 |
10 |
90 |
50 |
15 |
Nano-SB-[PSIM]Cl50 |
— |
— |
— |
16 |
[Py-SO3H]Cl51 |
10 |
120 |
35 |
17 |
APVPB52 |
10 mg |
80 |
60 |
18 |
Fe3O4@Co(BDC)-NH2 (ref. 53) |
10 mg |
70 |
65 |
19 |
H3PO3 |
10 |
60 |
63 |
20 |
CQDs |
10 mg |
45 |
70 |
21 |
CQDs-N(CH2PO3H2)2 (this work) |
10 mg |
25 |
89 |
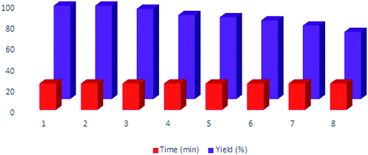 |
| Fig. 9 Recyclability of CQDs–N(CH2PO3H2)2 for the synthesis of 4H-pyran-3,5-dicarbonitrile derivatives. | |
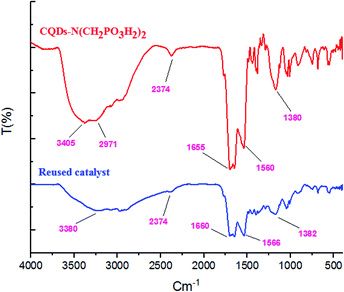 |
| Fig. 10 The characterization of the reused catalysts after seven runs using FT-IR spectroscopy. | |
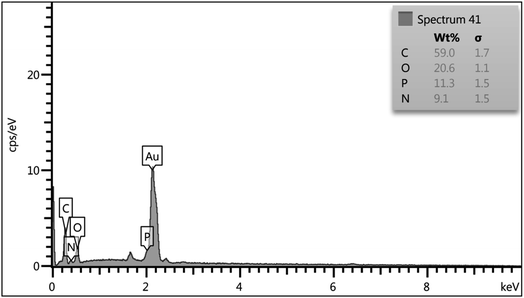 |
| Fig. 11 The characterization of the reused catalysts after seven runs using energy dispersive X-ray analysis (EDX). | |
4. Conclusion
In conclusion, we have designed and introduced CQDs–N(CH2PO3H2)2 as a novel heterogeneous nano-catalyst. It was identified using various techniques. CQDs–N(CH2PO3H2)2, as an efficient catalyst, was applied for the synthesis of multi substituted 4H-pyran-3,5-dicarbonitrile with indole moieties as candidates with biological interest. The presented methodology is not suitable for synthesis of pyridines with indole moieties. A short reaction time, clean and mild reaction conditions and the recycling of the catalyst are the major advantages of the presented work.
Conflicts of interest
There are no conflicts to declare.
Acknowledgements
We thank the Bu-Ali Sina University and Iran National Science Foundation (INSF) (grant number: 98020070) for financial support.
References
- M. J. Molaei, Anal. Methods, 2020, 12, 1266 RSC
. - M. J. Molaei, Sol. Energy, 2020, 196, 549 CrossRef CAS
. - X. Kou, S. Jiang, S. J. Park and L. Y. Meng, Dalton Trans., 2020, 49, 6915–6928 RSC
. - J. M. Katzen, C. Tserkezis, Q. Cai, L. H. Li, J. M. Kim, G. Lee, G. Yi, W. R. Hendren, E. J. G. Santos, R. M. Bowman and F. Huang, ACS Appl. Mater. Interfaces, 2020, 12, 19866 CrossRef CAS PubMed
. - G. Kalaiyarasan, J. Joseph and P. Kumar, ACS Omega, 2020, 5, 22278 CrossRef PubMed
. - F. Li, T. Li, C. Sun, J. Xia, Y. Jiao and H. Xu, Angew. Chem., Int. Ed., 2017, 56, 9910 CrossRef CAS PubMed
. - X. Xu, R. Ray, Y. Gu, H. J. Ploehn, L. Gearheart, K. Raker and W. A. Scrivens, J. Am. Chem. Soc., 2004, 126, 12736 CrossRef CAS PubMed
. - H. Li, X. He, Z. Kang, H. Huang, Y. Liu, J. Liu, S. Lian, C. H. A. Tsang, X. Yang and S. T. Lee, Angew. Chem., 2010, 122, 4532 CrossRef
. - W. Su, R. Guo, F. Yuan, Y. Li, X. Li, Y. Zhang, S. Zhou and L. Fan, J. Phys. Chem. Lett., 2020, 11, 1357 CrossRef CAS PubMed
. - B. C. Martindale, G. A. Hutton, C. A. Caputo and E. Reisner, J. Am. Chem. Soc., 2015, 137, 6018 CrossRef CAS PubMed
. - B. B. Karakoçak, A. Laradji, T. Primeau, M. Y. Berezin, S. Li and N. Revi, ACS Appl. Mater. Interfaces, 2021, 13, 277 CrossRef PubMed
. - K. M. Chan, W. Xu, H. Kwon, A. M. Kietrys and E. T. Kool, J. Am. Chem. Soc., 2017, 139, 13147 CrossRef CAS PubMed
. - X. Xiao, Z. Shao and L. Yu, Chin. Chem. Lett., 2021, 23, 4647 CAS
. - X. Wang, Y. Feng, P. Dong and J. Huang, Front. Chem., 2019, 7, 671 CrossRef CAS PubMed
. -
(a) C. Fujun, Y. Ping, L. Xiguang, L. Chunping and Q. Rongjun, Renewable Energy, 2014, 71, 61 CrossRef
;
(b) M. F. Mady and M. A. Kelland, Energy Fuels, 2017, 31, 4603 CrossRef CAS
;
(c) A. Kadous, M. Didi and D. Villemin, J. Radioanal. Nucl. Chem., 2010, 284, 431 CrossRef CAS
. - S. Moradi, M. A. Zolfigol, M. Zarei, D. A. Alonso and A. Khoshnood, ChemistrySelect, 2018, 3, 3042 CrossRef CAS
. -
(a) M. Zarei, M. A. Zolfigol, A. R. Moosavi-Zare, E. Noroozizadeh and S. Rostamnia, ChemistrySelect, 2018, 3, 12144 CrossRef CAS
;
(b) F. Jalili, M. Zarei, M. A. Zolfigol, S. Rostamnia and A. R. Moosavi-Zare, Microporous Mesoporous Mater., 2020, 294, 109865 CrossRef CAS
. - J. Afsar, M. A. Zolfigol, A. Khazaei, M. Zarei, Y. Gu, D. A. Alonso and A. Khoshnood, Mol. Catal., 2020, 482, 110666 CrossRef CAS
. - S. Babaee, M. Zarei, H. Sepehrmansourie, M. A. Zolfigol and S. Rostamnia, ACS Omega, 2020, 5, 6240 CrossRef CAS PubMed
. - S. Babaee, M. Zarei, M. A. Zolfigol, S. Khazalpour, M. Hasani, U. Rinner, R. Schirhagl, N. Norouzi and S. Rostamnia, RSC Adv., 2021, 11, 2141 RSC
. - H. Sepehrmansouri, M. Zarei, M. A. Zolfigol, A. R. Moosavi-Zare, S. Rostamnia and S. Moradi, Mol. Catal., 2020, 481, 110303 CrossRef CAS
. - S. Kalhor, M. Zarei, H. Sepehrmansourie, M. A. Zolfigol, H. Shi, J. Wang, J. Arjomandi, M. Hasanie and R. Schirhagl, Mol. Catal., 2021, 507, 111549 CrossRef CAS
. -
(a) A. Varvaresou, A. Tsantili-Kakoulidou, T. Siatra-Papastaikoudi and E. Tiligada, Arzneimittelforschung, 2000, 50, 48 CAS
;
(b) J. Slaett, I. Romero and J. Bergman, Synth., 2004, 16, 2760 Search PubMed
;
(c) F. Palluotto, A. Carotti, G. Casini, M. Ferappi, A. Rosato, C. Vitali and F. Campagna, Il Farmaco, 1999, 54, 191 CrossRef CAS PubMed
;
(d) M. Shiri, Chem. Rev., 2012, 112, 3508 CrossRef CAS PubMed
;
(e) M. Shiri, M. A. Zolfigol, H. G. Kruger and Z. Tanbakouchian, Chem. Rev., 2010, 110, 2250 CrossRef CAS PubMed
. -
(a) P. Kutsky, T. M. Dzurilla and A. Sabova, Collect. Czech. Chem. Commun., 1999, 64, 348 CrossRef
;
(b) P. Kutschy, M. Dzurilla, M. Takasugi, M. Török, I. Achbergerová, R. Homzová and M. Rácová, Tetrahedron, 1998, 54, 3549 CrossRef CAS
. - S. A. Zotova, T. M. Korneeva, V. I. Shvedov, N. I. Fadeeva, I. A. Leneva, I. T. Fedyakina, M. L. Khristova, I. S. Nikolaeva, V. V. Peters and T. A. Gus’kova, Pharm. Chem. J., 1995, 29, 57 CrossRef
. - N. V. Lakshmi, P. Thirumurugan, K. M. Noorulla and P. T. Perumal, Bioorg. Med. Chem. Lett., 2010, 20, 5054 CrossRef CAS PubMed
. - R. D. Dillard, N. J. Bach, S. E. Draheim, D. R. Berry, D. G. Carlson, N. Y. Chirgadze and J. P. Wery, J. Med. Chem., 1996, 39, 5119 CrossRef CAS PubMed
. - S. M. Gomha and H. A. Abdel-Aziz, Bull. Korean Chem. Soc., 2012, 33, 2985 CrossRef CAS
. - S. Battagba, E. Boldrini, F. D. Settimo, G. Dondio, C. L. Motta, A. M. Marini and G. Primofiore, Eur. J. Med. Chem., 1999, 34, 93 CrossRef
. - L. Garuti, M. Roberti, T. Rossi, M. Castelli and M. Malagoli, Eur. J. Med. Chem., 1998, 33, 43 CrossRef CAS
. -
(a) M. A. Radwan, E. A. Ragab, N. M. Sabry and S. M. El-Shenawy, Bioorg. Med. Chem., 2007, 15, 3832 CrossRef CAS PubMed
;
(b) S. L. Zhu, S. J. Ji, X. M. Su, C. Sun and Y. Liu, Tetrahedron Lett., 2008, 49, 1777 CrossRef CAS
;
(c) A. A. Fadda, A. El-Mekabaty, I. A. Mousa and K. M. Elattar, Synth. Commun., 2014, 44, 1579 CrossRef CAS
;
(d) P. Thirumurugan, A. Nandakumar, D. Muralidharan and P. T. Paramasivan, J. Comb. Chem., 2010, 12, 161 CrossRef CAS PubMed
. - D. Kumar, P. Sharma, H. Singh, K. Nepali, G. K. Gupta, S. K. Jain and F. Ntie-Kang, RSC Adv., 2017, 7, 36977 RSC
. - Saigal, M. Irfan, P. Khan, M. Abid and M. M. Khan, ACS Omega, 2019, 4, 16794 CrossRef CAS PubMed
. - P. K. Singh and O. Silakari, ChemMedChem, 2018, 13, 1071 CrossRef CAS PubMed
. - S. Gupta, S. Adhikary, R. K. Modukuri, D. Choudhary, R. Trivedi and K. V. Sashidhara, Bioorg. Med. Chem. Lett., 2018, 28, 1719 CrossRef CAS PubMed
. - S. P. Khare, T. R. Deshmukh, S. V. Akolkar, J. N. Sangshetti, V. M. Khedkar and B. B. Shingate, Res. Chem. Intermed., 2019, 45, 5159 CrossRef CAS
. - Z. Tashrifi, M. Mohammadi-Khanaposhtani, H. Hamedifar, B. Larijani, S. Ansari and M. Mahdavi, Mol. Diversity, 2020, 1 Search PubMed
. -
(a) P. N. Amaniampong and F. Jerome, Curr. Opin. Green Sustain. Chem., 2020, 22, 7 CrossRef
;
(b) J. Amaro-Gahete, R. Klee, D. Esquivel, J. R. Ruiz, C. Jiménez-Sanchidrián and F. J. Romero-Salguero, Ultrason. Sonochem., 2019, 50, 59 CrossRef CAS PubMed
. - F. Bigdeli, F. Rouhani, A. Morsali and A. Ramazani, Ultrason. Sonochem., 2020, 62, 104862 CrossRef CAS PubMed
. - J. Schneider, C. J. Reckmeier, Y. Xiong, M. von Seckendorff, A. S. Susha, P. Kasák and A. L. Rogach, J. Phys. Chem. C, 2017, 121, 2014 CrossRef CAS
. - M. Shiri, Chem. Rev., 2012, 112, 3508 CrossRef CAS PubMed
. - M. Shiri, M. A. Zolfigol, H. G. Kruger and Z. Tanbakouchian, Chem. Rev., 2010, 110, 2250 CrossRef CAS PubMed
. - H. Kooshki, A. Sobhani-Nasab, M. Eghbali-Arani, F. Ahmadi, V. Ameri and M. Rahimi-Nasrabadi, Sep. Purif. Technol., 2019, 211, 873 CrossRef CAS
. - T. Chen, X. P. Xu and S. J. Ji, J. Heterocycl. Chem., 2013, 50, 244 CrossRef CAS
. - N. V. Lakshmi, P. Thirumurugan, K. M. Noorulla and P. T. Perumal, Bioorg. Med. Chem. Lett., 2010, 20, 5054 CrossRef CAS PubMed
. - L. Kheirkhah, M. Mamaghani, A. Yahyazadeh and N. O. Mahmoodi, Appl. Organomet. Chem., 2018, 32, 4072 CrossRef
. - M. Zarei, H. Sepehrmansourie, M. A. Zolfigol, R. Karamian and S. H. Moazzami Farida, New J. Chem., 2018, 42, 14308 RSC
. - H. Sepehrmansourie, M. Zarei, R. Taghavi and M. A. Zolfigol, ACS Omega, 2019, 4, 17379 CrossRef CAS PubMed
. -
(a) M. A. Zolfigol, Tetrahedron, 2001, 57, 9509 CrossRef CAS
;
(b) H. Sepehrmansourie, Iran. J. Catal., 2020, 10, 175 CAS
. - A. Zare, M. Merajoddin, A. R. Moosavi-Zare, M. Zarei, M. H. Beyzavi and M. A. Zolfigol, Res. Chem. Intermed., 2016, 42, 2365 CrossRef CAS
. - A. R. Moosavi-Zare, M. A. Zolfigol, M. Zarei, V. Khakyzadeh and A. Hasaninejad, Appl. Catal., A, 2013, 467, 61 CrossRef CAS
. - E. Noroozizadeh, A. R. Moosavi-Zare, M. A. Zolfigol, M. Zarei, R. Karamian, M. Asadbegy, S. Yari and S. H. M. Farida, J. Iran. Chem. Soc., 2018, 15, 471 CrossRef CAS
. - H. Sepehrmansourie, M. Zarei, M. A. Zolfigol, S. Babaee and S. Rostamnia, Sci. Rep., 2021, 11, 5279 CrossRef CAS PubMed
.
Footnote |
† Electronic supplementary information (ESI) available. See DOI: 10.1039/d1ra02515e |
|
This journal is © The Royal Society of Chemistry 2021 |