DOI:
10.1039/D1RA02472H
(Paper)
RSC Adv., 2021,
11, 26160-26167
“Kill-release” antibacterial polysaccharides multilayer coating based therapeutic contact lens for effective bacterial keratitis treatment
Received
29th March 2021
, Accepted 24th July 2021
First published on 30th July 2021
Abstract
Contact lenses (CLs) are an important medical device for ophthalmic disease treatment. They can be used not only to correct refractive errors, such as myopia, but also can serve as a drug carrier for ocular surface disease treatment. In this study, a therapeutic CL was designed for bacterial keratitis treatment by constructing an antibacterial surface coating via a layer-by-layer (LbL) electrostatic self-assembly method. Vancomycin-incorporated chitosan nanoparticles were firstly prepared by ionic condensation of sodium tripolyphosphate (abbreviated as CTVNP). The positively charged CTVNP were then used for LbL deposition with negatively charged heparin (HEP), obtaining a (HEP/CTVNP)n polyelectrolyte multilayer on the CL surface. It is shown that such antibiotic incorporated surface coating doesn't influence the light transmittance of the CL, so it should not affect the patients' visual acuity when wearing them. The in vitro bacteriostatic effect evaluation was performed via live and dead bacteria staining and scanning electron microscope (SEM), which demonstrated the effective antibacterial property of such a surface. The fabricated therapeutic CL was then used to treat bacterial keratitis on a rabbit model. The results showed that such CL could effectively control the development of the bacteria-infected cornea and had a significant therapeutic effect.
Introduction
Corneal disease is one of the leading causes of blindness, with approximately 500
000 cases per year worldwide.1 Infectious keratitis is one of the main corneal diseases that is caused by direct infection by bacteria, fungi, yeasts, or amoebae, in which the bacterial infection stays at number one.1 Bacterial keratitis is often caused by infection of Gram-positive bacteria, which produces a variety of biologically active substances and toxins leading to corneal epithelial defects and corneal stroma necrosis in the defect area.2 It has the characteristics of high incidence, acute onset, and rapid progress. If the treatment is poorly controlled or not controlled in a timely manner, it can lead to progressive tissue destruction, corneal perforation, and even damage to the entire eye and serious vision loss. Clinical treatment of bacterial keratitis is mainly antibiotic eye drops administration. However, due to the limitation of the eye anatomy and physiological factors, the drug bioavailability in such administration mode is as low as less than 5%.3 As a result, high frequency administration is required. However, the patient compliance decreases with the increasing administration frequency. The side effects are increasing as well. As the cornea is a non-vascular tissue, the main route of its drug absorption is based on diffusion on the corneal surface. The corneal epithelium is a stratified layer presenting Ca2+-dependent membrane adherent regions (zonula occludens, zonula adherens, and desmosomes) that create tight junctions that are highly resistant to drug diffusion.4 So the drug delivery carriers those can increase the drug residence time and the corneal permeability may achieve higher bioavailability in actual application.
Contact lens (CL) is a kind of ocular device that commonly used in ophthalmic diseases treatment.5,6 Apart from vision correction, CL can be used therapeutically to treat dysfunctions of the eye, particularly corneal irregularities.7 They can also be used as drug carriers for the treatment of ocular diseases.8,9 Several techniques have been developed to construct drug eluting CL, including polymer coating,10 drug or drug contained nanoparticle soaking,11,12 hydrogel membranes coating,13 as well as supercritical fluid-assisted molecular imprinting.14 Polyelectrolyte multilayer that fabricated by layer-by-layer (LbL) self-assembly technology is proved to be an effective yet simple surface mediated drug eluting coating method.15 With advantages of water phase based preparation, good activity protection of the functional molecules, and ease fabrication on the sophisticated architecture, polyelectrolyte multilayer has been used to surface modification in several biomedical implants.16,17 In our previous investigations, polysaccharides multilayers, including chitosan (CHI)/hyaluronic acid,18 as well as antiproliferative drug loaded CHI nanoparticle/heparin (HEP),15 have been fabricated onto the intraocular lens to prevent the posterior capsular opacification postoperatively. Due to the good biocompatibility, the polysaccharides based polyelectrolyte multilayers were fabricated onto the CL surface for effectively antibiotic loading and thus to fabricate an antibacterial loaded therapeutic CL for effective bacterial keratitis treatment. The vancomycin (Van) was used due to its efficient antibacterial property on Staphylococcus aureus, Streptococcus pyogenes, and Streptococcus pneumonia. The Van incorporated CHI nanoparticle was firstly prepared via ionic condensation of CHI and tripolyphosphate (TPP), obtaining positively charged CHI–TPP–Van nanoparticle (CTVNP). The CTVNP was then used as a component for polyelectrolyte multilayer fabrication on CL surface via LbL deposition with negatively charged HEP (Fig. 1). The in vitro antibacterial effect as well as the in vivo treatment for bacterial keratitis was carried out for evaluation the fabricated therapeutic CL.
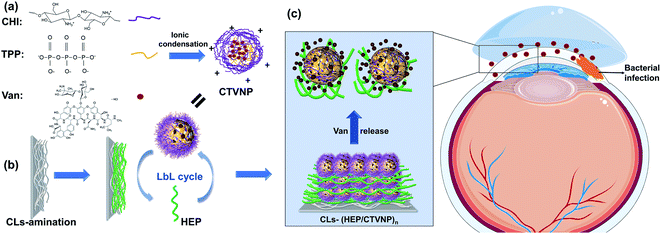 |
| Fig. 1 Schematic illustration of the therapeutic contact lens preparation and its treatment on bacterial keratitis. (a) Preparation of vancomycin loaded nanoparticle via ionic condensation of chitosan and tripolyphosphate (CTVNP); (b) CTVNP/HEP multilayer fabrication via LbL deposition. (c) CTVNP/HEP multilayer modified contact lens for bacterial keratitis treatment. | |
Materials and methods
Materials
The CHI (molecular weight (MW) approximately 179.17 kDa, high viscosity (>400 mPa s)), TPP, and HEP (185 USP units per mg) were purchased from Aladdin Biochemical Technology Co., Ltd. (Shanghai, China). The Van was purchased from Meilun Biotechnology Co., Ltd. (Dalian, China). The polyethyleneimine (PEI, MW = 25
000) was purchased from Sigma-Aldrich (St Louis, MO, USA). The LIVE/DEAD® Viability Kit, FBS, DMEM/F12 (1
:
1) cell culture media, and other cell culture-related reagents were purchased from Invitrogen (Waltham, MA, USA). The Luria–Bertani (LB) broth medium and agar powder were purchased from Solarbio Science & Technology Co., Ltd. (Beijing, China). Cell Counting Kit-8 (CCK-8) was purchased from Beyotime. All other chemicals were of analytical grade and used without further purification.
Drug-loaded CHI nanoparticle preparation
The Van incorporated CHI nanoparticle was prepared via ionic condensation of positively charged CHI and negatively charged TPP, obtaining CHI–TPP–Van nanoparticle (CTVNP). The fabrication process was according to our previous investigations.15,19 Briefly, 1 mg mL−1 CHI in 1% acetic acid solution, 2 mg mL−1 TPP solution were firstly prepared. A certain Van powder was then dissolved into CHI solution and the final Van concentration was 0.1 mg mL−1. Finally, 3 mL TPP was added dropwisely into 16 mL CHI–Van mixture solution under magnetic stirring at 800 rpm at room temperature and incubated for another 30 min and thus the CTVNP was obtained. The size and zeta potential of the nanoparticles were measured by dynamic light scattering method on a particle size analyzer (Autosizer 3000, Malvern Instruments Limited, UK).
Multilayer film fabrication and surface modification
Hydrophilic CL was used as substrate for the polyelectrolyte multilayer fabrication via electrostatic LbL assembly. CL was firstly immersed in 3 mg mL−1 PEI solution for 2 h, getting aminated surface to facilitate the subsequent polyelectrolyte multilayer fabrication.20 The aminated CL was then dipped into 1 mg mL−1 HEP acetate buffer solution (pH = 4) and freshly prepared CTVNP solution for 15 min alternately, with acetate buffer rinsing intervals, which obtains the HEP or CTVNP absorbed surface after each deposition step. One HEP and CTVNP deposition cycle was defined as (HEP/CTVNP)1 and the procedure was continued until the desired bilayer number was deposited. Thus the Van incorporated coating was fabricated onto the CL surface and the therapeutic CL was obtained. The multilayer fabrication on the surface was followed by water contact angle measurement by a contact angle analyzer (OCA20, Dataphysics, Germany). The surface morphology and the transmittance of the (HEP/CTVNP) multilayer modified CL materials were also investigated by using SEM (Phenom-World, the Netherlands) and ultraviolet-visible (UV-vis) spectrophotometer respectively (UV-1780, SHIMADZU, Japan), with pristine CL materials served as controls.
Cytotoxicity assays
1 × 1 cm2 PET sheets were used as the assembly substrate during the sample preparation, which were divided into four groups: pristine, PEI, (HEP/CTNP)4.5, (HEP/CTNP)5, each group had five parallel samples. After assembly, both sides were irradiated under UV for 4 hours to eliminate bacteria on the surface. Human corneal epithelial cells (HCEC) were selected and planted in a sterile 96 well plate with a density of 5000 per well. Each sample was placed in the corresponding well, 10 minutes later, and were co-cultured with cells for two days. Finally, the cytotoxicity was determined with the CCK-8 diluted with medium at the ratio of 10
:
1. 100 μL aforementioned CCK-8 solution was added to wells and incubated for 2 hours. The absorbance at 450 nm was determined and recorded using a microplate reader. The wells without cells in CCK-8 solution were set as blank group, the CCK-8 solution plus cells were set as control group. The remaining four groups above were treated as experimental groups. The cell viability was calculated by the formula: (A(experimental group) − A(blank group)) × (A(control group) − A(blank group)) × 100%.
Drug release profile investigation
The in vitro release behavior of the (HEP/CTVNP) multilayer modified CL materials was also conducted in this study to investigate the antibiotic release profile from the therapeutic CL. The materials were incubated in phosphate-buffered saline under gentle shaking at 37 °C. A total of 200 μL of the release buffer was collected at certain time point (15 min, 30 min, 1 h, 2 h, 4 h, 6 h, 8 h, 12 h, 24 h, 2 days, 4 days, 7 days, 2 weeks, and 1 month) and stored in a refrigerator at 4 °C. When all the samples were collected, the samples were tested by a microplate reader (SpectraMax M5, MD, USA). The optical density (OD) at 280 nm was read and the amount of the Van releasing at the certain time point was calculated according to the standard curve that measured simultaneously by a list of pure Van solution with different concentrations.
In vitro antibacterial properties assessment
In vitro antibacterial property of the Van incorporated CL materials were investigated by bacterial live/dead staining.21 The Staphylococcus aureus was used as model bacteria. The bacteria was cultured in an LB broth medium for 12 h, diluted to 105 CFU mL−1 and set aside for seeding. The materials were placed on 24 well cell culture plates and sterilized by ultraviolet irradiation for 2 h. After sterilization, 1 mL freshly propagated bacteria suspension (105 CFU mL−1) was seeded into the wells and incubated for 8 h. Then the culture supernatant was discarded, rinsed by PBS buffer for three times and the staining solution was subsequently added in the absence of light for 15 min, followed with PBS rinsing. The dead or live bacteria were observed by fluorescent microscopy (ECLIPSE-Ti, Nikon, Japan). The pristine or the no drug incorporated multilayer (HEP/CTNP, without Van adding when CHI nanoparticle preparation) modified CL materials were served as controls.
The in vitro antibacterial effect of the therapeutic CL was also evaluated by scanning electron microscopy (SEM).22,23 The bacteria culture was carried out in the similar way to the above bacterial live/dead staining experiment. The materials were soaked in the bacterial suspension for 12 h and washed with PBS. Then the adherent bacteria were fixed by 2% glutaraldehyde solution for 12 hours, dehydrated in 50%, 70%, 90%, and 100% alcohol for 2 h, 1 h, 1 h, and 2 h, respectively. Then the specimens were treated by spray-gold. SEM was used to study the bacterial distribution and morphology on the surfaces. The no drug loaded multilayer (HEP/CTNP) modified CL materials were served as controls.
Animal experiments
The animal experiments were approved by the local laboratory animal committee (Laboratory Animal Ethics Committee at Wenzhou Medical University). Japanese white rabbits with weight around 2.5 kg with random gender were obtained from the Wenzhou Medical University Laboratory Animal Center (Approval No. 2019-057). The experiment was carried out according to the Laboratory Animals – guidelines for ethical review of animal welfare (2018, China). Routine ocular examination was performed on all animals before the experiment to rule out eye diseases. Ofloxacin eye drops were used on the same batch of rabbits 3 times every day for 3 days. The animal model of bacterial keratitis was established according to the ref. 28. Briefly, the rabbits were anesthetized by injection of 2% pentobarbital (1 mL kg−1) and a muscle relaxant (SuMianXin, 0.2 mL kg−1). The cornea was circumcised with an 8.5 mm ring drill to reach the depth of the stroma, and 100 μL of Staphylococcus aureus (105 CFU mL−1) was injected into the stroma along the incision with a 1 mL disposable insulin syringe. As the liquid spread, the bacteria had entered the corneal stroma. After bacterial infection model setup, the prepared therapeutic CLs with Van incorporation in the surface coating were covered onto the cornea with 10-0 ophthalmic sutures fixation on the conjunctiva to keep them from falling off. The no drug loaded multilayer (HEP/CTNP) modified CL were served as controls. The anterior segment was photographed by slit lamp postoperatively 3 and 7 days after therapeutic CL wearing. The CLs were removed in the postoperatively 7 days and the ocular infection severity was evaluated by the morphology and integrity of the cornea observation. Finally, the rabbits were anesthetized and euthanized, the whole eyeballs of the rabbits were removed and fixed in 4% paraformaldehyde, and the tissues were separated for paraffin sections and HE staining.
Statistical analysis
The results were expressed as the mean ± standard error of the mean for each sample. Three parallel samples were used in each experimental group and each experiment was repeated three times independently.
Results and discussion
Nanoparticles and multilayer fabrication and coating onto cLs
CHI is a kind of natural polysaccharide with excellent biocompatibility, antibacterial activity, and good hydrophilicity.24 It is insoluble in neutral medium whereas became soluble in the acidic medium due to the protonation of the –NH2 group at the D-glucosamine unit. The protonation of the CHI makes it good positive charge. As a result, CHI nanoparticles were commonly investigated in the drug delivery fields via the method of the ionic condensation of the positively charged CHI with negatively charge molecules, such as tripolyphosphate (TPP).15,19 Herein, the CHI–TPP nanoparticle was used to load the antibiotic Van with the aim to fabricate therapeutic CL ultimately. The Van loaded CHI–TPP nanoparticle (CTVNP) was prepared under the optimized N/P ratio (the mole ratio of N element in CHI and P element in TPP) according to the previous investigations.15,19 As shown in Fig. 2(a), the prepared CTVNP rendered strong positively charge on their surface, with the zeta potential value of 38.7 ± 0.4 mV. The particle size was mainly concentrated at approximately 350 nm, as shown in Fig. 2(b). As expected, the results showed that the positively charged CTVNP was successfully prepared. And it is appropriate in the following surface modification via LbL electrostatic self-assemble technique.
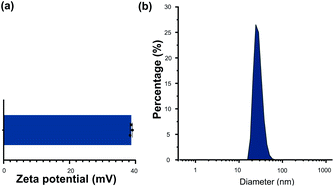 |
| Fig. 2 Zeta potential (a) and particle size (b) of the prepared CTVNP. | |
The fabricated CTVNP was then used to construct polyelectrolyte multilayer on the CL material surface via LbL deposition with negatively charged HEP. The multilayer deposition was followed by the surface wettability measurement by water contact angle measuring instrument in each step. As shown in Fig. 3, the water contact angle of the pristine material was approximately 75°. The surface aminolysis slightly decreased the contact angle. When the subsequent LbL deposition of the HEP and CTVNP carried out, the surface hydrophilicity greatly increased, which were indicated by the generally decreasing of the contact angle values. It is interesting that the contact angle values showed an alternating downward trend after one bilayer (HEP/CTVNP) deposition. The surfaces with HEP as the outmost layer (0.5, 1.5, 2.5, 3.5 and 4.5 bilayer) were more hydrophilic than that with CTVNP as the outmost layer (1, 2, 3, 4 and 5 bilayer). After the HEP/CTVNP multilayer deposition, the surface became more and more hydrophilicity, with water contact angle between 10° to 20° after 5 bilayer's modification. This result not only clearly indicates the successful alternate deposition of HEP and CTVNP onto the surface, but also indicates the excellent hydrophilicity of the modified surface.
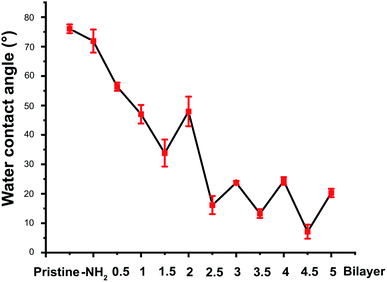 |
| Fig. 3 The water contact angle of the surface in each deposition step during the HEP/CTVNP multilayer fabrication. | |
As the prepared therapeutic CL not only a drug eluting system, but also a refractive correction device, the optical property of the HEP/CTVNP multilayer modified CL materials was investigated to evaluate the influence of the surface coating on the light transmittance. Fig. 4(a) shows that the light transmittance changes of the materials with or without HEP/CTVNP multilayer coating. It can be observed that the surface coating of HEP/CTVNP multilayer does not noticeably change the light transmittance of the substrate materials, no matter the deposition coating is 1 bilayer or 5 bilayer. Fig. 4(b) shows the surface morphology of the material with or without the HEP/CTVNP multilayer coating as observed via SEM. Compared with the pristine material, no significant change was found when the coating was established on the surface, which also a clue that such therapeutic coating modification does not affect the bulk property of the CL materials.
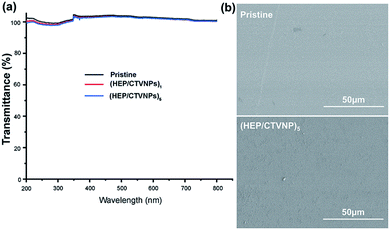 |
| Fig. 4 Light transmittance (a) and SEM images of surface morphology (b) of the CL materials with or without HEP/CTVNP multilayer modification. | |
Cytotoxicity evaluation
The purposes of wearing the coated CL on the damaged corneal surface were to protect the tissue and promote the self-repair of corneal epithelium; on the other hand, to control the spread of inflammation and treat infection. Different coatings were co-cultured with HCECs for 2 days, and the cytotoxicity was determined to evaluate the safety of the (HEP/CTNP)n on the ocular surface. As shown in Fig. 5, the cell viability of the unmodified group (pristine) was 88%, and that of the aminated group (PEI) was 86%. When heparin was used as the outermost layer, the value was 87%. It decreased in the (HEP/CTNP)5 group, because of the positive charge of chitosan nanoparticles. However, the viability was still above 80%, which was a normal range. The results demonstrated that the multilayer membranes were safe on ocular surface, and the application of (HEP/CTNP)n-CLs were feasible.
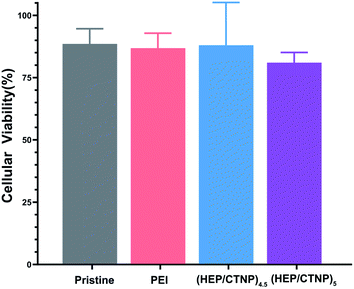 |
| Fig. 5 The toxicity of different coatings onto the CL cultured with HCECs. | |
Drug release investigation
Polysaccharides have an increasing number of applications in biomaterials fields such as tissue engineering, drug carriers, and drug controlled release.25,26 It is well known that owing to the rapid development of bacterial keratitis, the corneal structure would be rapidly destroyed if it is not treated in time or if the dose of antibiotics was not reached. Therefore, the drug release profiles of the therapeutic CL were investigated. The cumulative Van release was shown in Fig. 6. It can be observed that the drug underwent burst release within 24 h, and sustained release was followed which continued as long as one month. Therefore, enough therapeutic amount of the Van can be released in the early stage, which had great treating efficiency of infectious disease treatment. Also, the sustained release of the Van from the therapeutic CL could effectively prevent the biofilm formation when the antibiosis was not complete.27
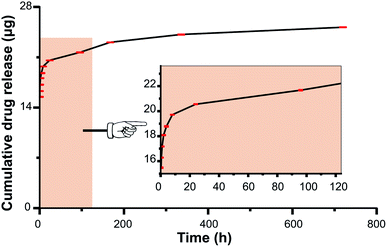 |
| Fig. 6 Cumulative Van release amounts from the HEP/CTVNP multilayer modified CL materials in one month. The insertion is the amplification in the early stage. | |
In vitro antibacterial effect evaluation
The antibacterial effect of the fabricated therapeutic CL was investigated by in vitro bacteria culture. To compared the effect of the loaded Van in the therapy, no Van loaded surface coatings (HEP/CTNP) were also investigated as controls, which were fabricated by the LbL deposition of the HEP with the CHI nanoparticles without Van loading. The bacteria were seeded onto the surface modified materials and the adherent bacteria on the surface were photographed under the condition of SEM photography by fixation and dehydration.28,29 It can be seen that there were plenty of bacteria found on the material surface of the no Van loading. The adherent bacteria presented intact morphology. However, after the Van loaded multilayer modification, almost no bacteria were found on the surface. A very few adherent bacteria found on the surface were rendering structural failure in morphology. This result indicated that the HEP/CTVNP surface modification on the CL can serve an effective antibacterial therapy (Fig. 7).
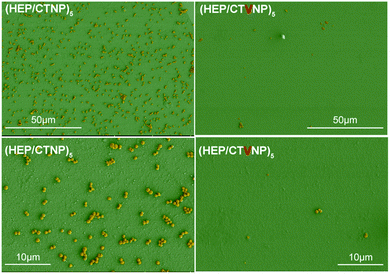 |
| Fig. 7 The Staphylococcus aureus adhesion on the CL material surfaces. HEP/CTNP and HEP/CTVNP referred to the CL surface coating without or with Van loading. | |
To further confirm the antibacterial property, bacterial live/dead staining was performed to observe the germicidal efficacy of the coating surfaces. It is a common yet effective method used to assess the bacterial activity by making use of the differences in physiological functions and properties of dead and live bacteria. The kit consisted of two stains, propidium iodide (PI) and SYTO 9. The green fluorescing SYTO 9 can enter intact cell membranes (live bacteria), whereas red fluorescing PI only permeates cells with damaged cytoplasmic membranes (dead bacteria).30,31 As shown in Fig. 8, numerous live bacteria were found on the pristine CL material surface, cell biofilm also began to formation on such surface in the investigated time period. When the surface was covered by the multilayer coating without Van loading, the surface bacterial adhesion were similar to that on the pristine, whereas no bacterial biofilm was detected on the surface as the adherent bacteria were uniformly dispersed. However, there was almost no bacterial adhesion found on the Van loaded HEP/CTVNP multilayer surface, no matter live or dead bacteria. There was no doubt about it that the Van loading could kill the bacteria on the surface, yet it was surprising and interesting that the killed bacterial corpses were not found on the surface. It is known that the bacterial corpse accumulation is also a key issue in biofilm formation. So the bacterial corpses releasing property after bacterial killing was also important for long term antibacterial therapy. Herein, no live and dead bacteria was found on the HEP/CTVNP multilayer coating surface, which suggested that such surface coating can not only kill bacteria effectively on the surface but also release the bacterial corpse from the surface. It is reported that the hydrophilic polysaccharide multilayer renders excellent anti-fouling properties.32 So, the release of the bacterial corpses may due to the excellent hydrophilicity of the (HEP/CTVNP) multilayer.
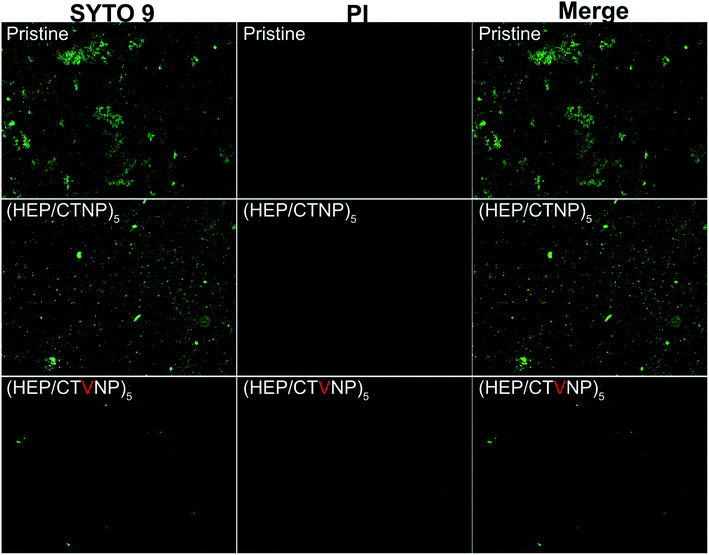 |
| Fig. 8 The fluorescent images of bacterial live/dead staining results on pristine, HEP/CTNP multilayer and HEP/CTVNP multilayer modified CL material surfaces. SYTO 9, PI, and merge response to living channel, dead channel, and merging channel respectively (100×). | |
In vivo antibacterial effect and biocompatibility evaluation
The fabricated therapeutic CLs were applied to the rabbit eyes with bacterial keratitis pre-established. To avoid the detachment of the therapeutic CLs from the ocular surface induced by the animal scratching, bulbar conjunctiva four points fixation was given via 10-0 ophthalmic suture. When the CLs were attached to the eye, they acted as a bandage, preventing the invasion of other external bacteria and promoting wound healing. As shown in Fig. 9a1 and b1, the bacterial keratitis was successfully established before the therapeutic CLs administration. The arrows represent the injected bacteria in the cornea stroma. The therapeutic CLs were then worn onto the ocular surface and the therapy lasted for one week. It showed that severe inflammation produced in the eyes which wore the HEP/CTNP multilayer modified CLs for 3 days (Fig. 9a2). Secretory liquid was found on the eyes and white blind spots were generated in the cornea. The white blind spots were observed worse growth in the postoperative 7 days. Almost all the cornea was blind due to the serious bacterial keratitis (Fig. 9a3). In the contrary, though there were still some secretions on the eye surface near the sutures, the eyes wore with the HEP/CTVNP multilayer coated CLs showed less inflammation and the cornea basically became clear in the postoperative 3 days (Fig. 9b2) and the cornea recovered to clear 7 days postoperatively (Fig. 9b3).
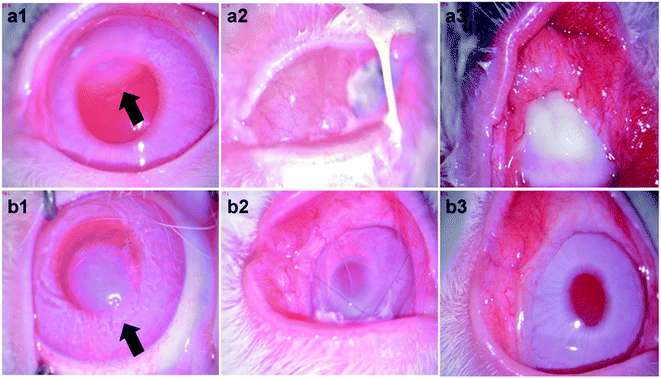 |
| Fig. 9 The slit lamp images of the bacterial keratitis established rabbit eyes with HEP/CTNP (a) or HEP/CTVNP (b) multilayer modified CLs treatment for 0 day (a1, b1), 3 day (a2, b2) and 7 day (a3, b3). | |
The histological sections of the anterior segment were investigated for further demonstration of the therapeutic CLs effectiveness in treating the bacterial keratitis, as shown in Fig. 10. As a transparent refractive medium without blood vessels, the cornea has a profound influence on vision. Its position in front of the eyeball enables the cornea to protect the visual organs. Therefore, the integrity of the structure and function of the cornea are of great significance. It could be seen that the ocular tissues in anterior segment kept normal structure when the bacterial infected eyes were treated with therapeutic HEP/CTVNP multilayer modified CLs. Typically, cornea consists of the epithelium, Bowman's layer, stroma, Descemet's membrane, and the endothelium.33 The cornea was intact when the infected eyes were treated the therapeutic HEP/CTVNP multilayer coated CLs. The corneal thickness and structure were not significantly changed in this situation. However, when no Van was incorporated in the coatings (HEP/CTNP), the corneal thickness was greatly increased. The chamber angle (as indicated θ in the figures), which is the angle between cornea and iris, also became narrower in this situation (θ1 < θ2). The thicker cornea and the narrower chamber angle might due to the serious inflammatory reaction of the bacterial infection when coatings on the CLs were no therapeutic effects. All these results revealed the excellent effectiveness of the therapeutic CL in treating the bacterial keratitis.
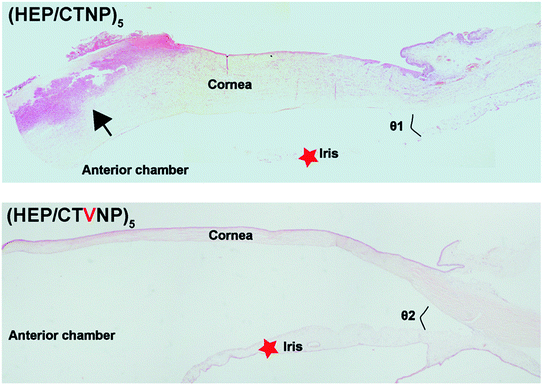 |
| Fig. 10 Histological images of the anterior section in the eyes treated with non-therapeutic coating (HEP/CTNP) and therapeutic coating (HEP/CTVNP) via hematoxylin–eosin staining. | |
Conclusions
In this investigation, therapeutic CLs were designed and prepared via the LbL self-assembly of the HEP and the Van incorporated CHI nanoparticle. The fabricated antibiotics incorporated polysaccharide (HEP/CTVNP) multilayer coating modification on the CL materials does not significantly affect its light transmittance whereas the incorporated antibiotics could be released from the coatings. It was interesting that the (HEP/CTVNP) multilayer coating could not only effectively kill the bacteria on the surface, but also release the bacterial corpse from the material surface. The Specific bacterial “kill-release” property may due to the excellent hydrophilicity of the therapeutic coating, which might have great potential in treating the infectious disease. The curative effect was confirmed by the bacterial keratitis in the rabbit models, where significant healing was found when the infected eyes were treated by the therapeutic coating modified CLs. All these results suggested that such “kill-release” antibacterial surface coating based therapeutic contact lens could serve an effective alternative in treating bacterial keratitis.
Conflicts of interest
There are no conflicts to declare.
Acknowledgements
The support from the National Key R&D Program (2017YFC1104602) was greatly acknowledged.
References
- N. Ustundag-Okur, E. H. Gokce, D. I. Bozbıyık, S. Egrilmez, O. Ozer and G. Ertan, Eur. J. Pharm. Sci., 2014, 63, 204–215 CrossRef CAS PubMed.
- M. E. Marquart and R. J. O'Callaghan, J. Ophthalmol., 2013, 2013, 369094 Search PubMed.
- S. K. Bharti and K. Kesavan, Ocul. Immunol. Inflamm., 2017, 25, 463–474 CrossRef CAS PubMed.
- P. W. Morrison and V. V. Khutoryanskiy, Int. J. Pharm., 2014, 472, 56–64 CrossRef CAS PubMed.
- L. Jones, N. A. Brennan, J. Gonzalez-Meijome, J. Lally, C. Maldonado-Codina, T. A. Schmidt, L. Subbaraman, G. Young, J. J. Nichols and Members of the TFOS International Workshop on Contact Lens Discomfort, Invest. Ophthalmol. Vis. Sci., 2013, 54, TFOS37–70 CrossRef PubMed.
- C. S. A. Musgrave and F. Fang, Materials, 2019, 12, 261 CrossRef CAS PubMed.
- R. Moreddu, D. Vigolo and A. K. Yetisen, Adv. Healthcare Mater., 2019, 8, 1900368 CrossRef PubMed.
- Z. Mutlu, S. Shams Es-Haghi and M. Cakmak, Adv. Healthcare Mater., 2019, 8, 1801390 CrossRef PubMed.
- U. Ubani-Ukoma, D. Gibson, G. Schultz, B. O. Silva and A. Chauhan, Int. J. Pharm., 2019, 565, 499–508 CrossRef CAS PubMed.
- X. Xie, X. Shao, W. Ma, D. Zhao, S. Shi, Q. Li and Y. Lin, Nanoscale, 2018, 10, 5457–5465 RSC.
- H. J. Jung, M. Abou-Jaoude, B. E. Carbia, C. Plummer and A. Chauhan, J. Controlled Release, 2013, 165, 82–89 CrossRef CAS PubMed.
- J. Kim, C. C. Peng and A. Chauhan, J. Controlled Release, 2010, 148, 110–116 CrossRef CAS PubMed.
- J. Xu, X. Li and F. Sun, Acta Biomater., 2010, 6, 486–493 CrossRef CAS PubMed.
- F. Yanez, L. Martikainen, M. E. Braga, C. Alvarez-Lorenzo, A. Concheiro, C. M. Duarte, M. H. Gil and H. C. de Sousa, Acta Biomater., 2011, 7, 1019–1030 CrossRef CAS PubMed.
- Y. Han, J. Tang, J. Xia, R. Wang, C. Qin, S. Liu, X. Zhao, H. Chen and Q. Lin, Int. J. Nanomed., 2019, 14, 9047–9061 CrossRef CAS PubMed.
- J. J. Richardson, M. Bjornmalm and F. Caruso, Science, 2015, 348, 2491 CrossRef PubMed.
- K. Ariga, J. P. Hill and Q. Ji, Phys. Chem. Chem. Phys., 2007, 9, 2319–2340 RSC.
- Q. Lin, X. Xu, Y. Wang, B. Wang and H. Chen, Int. J. Polym. Mater. Polym. Biomater., 2017, 66, 97–104 CrossRef CAS.
- Y. Han, X. Xu, Y. Wang, S. Liu, X. Zhao, H. Chen and Q. Lin, J. Colloid Interface Sci., 2018, 24, 40–44 CrossRef CAS.
- Q. Lin, X. Ding, F. Qiu, X. Song, G. Fu and J. Ji, Biomaterials, 2010, 31, 4017–4025 CrossRef CAS PubMed.
- C. Unoson and E. G. Wagner, Mol. Microbiol., 2008, 70, 258–270 CrossRef CAS PubMed.
- T. H. Z. Shaobin Liu, M. Hofmann, E. Burcombe, J. Wei, R. Jiang, J. Kong and Y. Chen, ACS Nano, 2011, 5, 6971–6980 CrossRef PubMed.
- C. Krishnaraj, E. G. Jagan, S. Rajasekar, P. Selvakumar, P. T. Kalaichelvan and N. Mohan, Colloids Surf., B, 2010, 76, 50–56 CrossRef CAS PubMed.
- M. Rinaudo, Prog. Polym. Sci., 2006, 31, 603–632 CrossRef CAS.
- S. Calamak, R. Shahbazi, I. Eroglu, M. Gultekinoglu and K. Ulubayram, Expert Opin. Drug Discovery, 2017, 12, 391–406 CrossRef CAS PubMed.
- M. Rinaudo, Polym. Int., 2008, 57, 397–430 CrossRef CAS.
- R. M. Donlan and J. W. Costerton, Clin. Microbiol. Rev., 2002, 15, 167–193 CrossRef CAS PubMed.
- L. Zhang, Y. Jiang, Y. Ding, M. Povey and D. York, J. Nanoparticle Res., 2006, 9, 479–489 CrossRef.
- P. T. Kumar, V. K. Lakshmanan, T. V. Anilkumar, C. Ramya, P. Reshmi, A. G. Unnikrishnan, S. V. Nair and R. Jayakumar, ACS Appl. Mater. Interfaces, 2012, 4, 2618–2629 CrossRef PubMed.
- B. Lina, B. Benoit, C. Josee and D. Raymond, J. Microbiol. Methods, 1999, 3, 77–86 Search PubMed.
- M. Berney, F. Hammes, F. Bosshard, H. U. Weilenmann and T. Egli, Appl. Environ. Microbiol., 2007, 73, 3283–3290 CrossRef CAS PubMed.
- Q. Lin, X. Xu, B. Wang, C. Shen, J. Tang, Y. Han and H. Chen, J. Mater. Chem. B, 2015, 3, 3695–3703 RSC.
- W. Roger and P. Lia, Microsc. Res. Tech., 1996, 33, 320–335 CrossRef.
|
This journal is © The Royal Society of Chemistry 2021 |
Click here to see how this site uses Cookies. View our privacy policy here.