DOI:
10.1039/D1RA02464G
(Paper)
RSC Adv., 2021,
11, 17630-17641
Betel (Piper betle L.) leaf ethanolic extracts dechlorophyllized using different methods: antioxidant and antibacterial activities, and application for shelf-life extension of Nile tilapia (Oreochromis niloticus) fillets
Received
29th March 2021
, Accepted 4th May 2021
First published on 13th May 2021
Abstract
Different methods for chlorophyll removal were used for betel leaf ethanolic extracts (BLEE). Chlorophyll content, color, and antioxidant and antibacterial activities of the resulting extracts were examined. Sedimentation process remarkably reduced the chlorophyll content and color of BLEE (p < 0.05), while antioxidant and antibacterial activities were enhanced (p < 0.05). Polyphenol content and bioactivities of the extracts dechlorophyllized using organic solvents varied (p < 0.05). Antibacterial efficacy of BLEE dechlorophyllized by the sedimentation method (BLEE-SED) depended on concentrations. Lower minimum inhibitory concentration (MIC) and minimum bactericidal concentration (MBC) of BLEE-SED toward 4 bacteria were obtained, compared to other extracts. Lower microbiological and chemical changes were achieved when Nile tilapia fillets were treated with BLEE-SED at 400 and 600 ppm after 12 days of storage at 4 °C. Therefore, sedimentation as a green process could be adopted for preparing a safe BLEE with augmented bioactivities and pale color, which could extend shelf-life of refrigerated Nile tilapia fillets.
1. Introduction
Fish are highly perishable because of their biological composition.1 Unfavorable enzymatic mediated reactions and protein decomposition induced by microbial proliferation contribute to undesirable changes e.g., off-flavor and unpalatable taste of fish during refrigerated storage.2 Fish spoilage not only contributes to a serious public health hazard, but also causes economic losses. Synthetic preservatives have been extensively used to extend the shelf-life of fish.3 Nevertheless, safety concerns regarding the excessive usage of such preservatives required adopting alternative methods based on application of natural compounds to fish products.3 In this context, plant extracts possessing both antioxidant and antibacterial properties have been employed.4,5 Nile tilapia (Oreochromis niloticus) has been consumed globally owing to its high nutritive value with high protein content (15–20%) and considerably low fats (1–4%).6 It was estimated that about 4.13 million tons were produced in 2017 globally with an expected rapid growth in the future.7
Betel (Piper betle L.), known commonly as “phlu” in Thailand, has several uses in traditional medicine. High content of secondary metabolites such as polyphenols and essential oils in betel leaves could be responsible for their antioxidant and antibacterial activities.8 Polyphenols are naturally occurring organic compounds of plant origin with different chemical structures. They include various sub-compounds such as flavonoids which exhibit bioactivities including antioxidant, antimicrobial, and anti-cancer activities. As a response to the adverse effects caused by the synthetic compounds used in food and pharmaceutical applications, polyphenols were proposed as perfect alternative candidates to be used in such applications including food preservation applications.9,10 This suggests the possible use of betel leaf ethanolic extract as an alternative to the synthetic preservatives for shelf-life extension of fish and fish products.
However, the presence of high chlorophyll content in betel leaf extract is a major obstacle for its use in foods since the extract rich in chlorophyll will inevitably change natural color of foods, leading to the undesirable color and rejection by consumers. Also, chlorophyll is unstable molecule when extracted due to substitution of its central magnesium ion with weak acids in food materials as well as its photo-oxidant characters. This could induce oxidation in foods, thus decreasing their storage stability and causing off-flavor, apart from discoloration.11,12 For these reasons, chlorophyll removal is a crucial step for maximized exploitation of plant extract, especially betel leaf extract for food applications.
Traditionally, different organic solvents were used for dechlorophyllization of different plant extracts. Nevertheless, the aforementioned process was not effective as the coloring pigments were still present in the extracts. Only 63% of the chlorophyll was removed from ethanolic guava leaf extract using some organic solvents as reported by Olatunde et al.,4 while 76% was removed from brown lead seed as observed by Benjakul et al.9 Additionally, different bioactivities were negatively affected by the process. Toxicity of these organic solvents used for chlorophyll removal was another matter of consideration,13 especially when the plant extract was used in foods. Other techniques were also reported for the dechlorophyllization. Those include the use of activated charcoal, liquid–liquid partitioning, and ChloroFiltr cartridges.14 Countercurrent separation (CCS) was used for chlorophyll removal from botanical crude extracts enriched in chlorophyll.15 However, the complexity, time consumed, cost, and less availability of required materials and instruments may be drawbacks for the use of those techniques. The practical need is focused on a simple, affordable, and green process to remove chlorophyll from plant extracts, particularly leaves, while maintaining their bioactivities. Sedimentation induced by water has been shown as a satisfying means and is proposed as a potential and green method for dechlorophyllization.13,16
The investigation aimed to comparatively study antioxidant and antibacterial activities of betel leaf ethanolic extracts (BLEE) when dechlorophyllized using different methods. Different organic solvents with various polarities were used as the traditional method for chlorophyll removal, while sedimentation method was implemented as a novel green method for chlorophyll removal. The selected dechlorophyllized extract showing the highest antioxidant and antibacterial activities was applied on Nile tilapia fillets as a preservative and quality changes were monitored during refrigerated storage (4 °C) for 12 days.
2. Materials and methods
2.1. Chemicals
All chemicals used were of analytical grade. Plate count agar was procured from Difco (Maryland, USA). Eosin methylene blue (EMP) agar, triple sugar iron agar, and Pseudomonas isolation agar were obtained from Himedia (Mumbai, India).
2.2. Preparation of betel leaf powder (BLP)
Betel (Piper betle L.) leaves were gathered from a plantation near Songkhla province, Thailand, between October and November 2020. Vines were approximately 3 to 5 years old and only mature and healthy leaves were used. Betel leaf powder was prepared as tailored by Tagrida and Benjakul.13 Leaves were washed thoroughly using the running tap water. Subsequently, leaves were dried using a hot air oven overnight at 50 °C until the moisture content of the leaves was less than 10%. Dried leaves were collected, blended using a high-speed blender (Panasonic, Model MX-898N, Berkshire, UK), and sieved using 80 mesh stainless-steel sieve to obtain the fine powder. The betel leaf powder (BLP) was kept in zip-lock bags and placed in a desiccator at room temperature until further processing.
2.3. Preparation of betel leaf ethanolic extract (BLEE) without dechlorophyllization
The extraction process was performed following the method outlined by Tagrida and Benjakul.13 Ethanol (70%) was used as extraction solvent at a leaf powder/solvent ratio of 1
:
15 (w/v). Ethanol was removed by a rotary evaporator before being lyophilized. Dried extract without removing the chlorophyll was used as the control termed ‘BLEE-CON’ and stored at −20 °C until use.
2.4. Preparation of BLEE with dechlorophyllization using different methods
2.4.1. Dechlorophyllization using different organic solvents. The procedure of Chotphruethipong et al.17 was adopted with minor modifications. Acetone, chloroform, and petroleum ether were selected as dechlorophyllizing solvents. Each solvent was mixed with BLP separately at a 1
:
10 powder/solvent ratio (w/v) and stirred for 30 min, followed by filtering using Whatman filter paper no. 1. The filtrate was discarded, and the retentate was subjected to dechlorophyllization in the same manner for another two times. The retentates were subsequently dried for 1 h at 105 °C in a hot air oven. Thereafter, the dried powders were subjected to ethanolic (70%) extraction and filtered following the procedure of Tagrida and Benjakul.13 The obtained extracts after lyophilization were termed BLEE-ACT, BLEE-CF, and BLEE-PET for BLP dechlorophyllized using acetone, chloroform, and petroleum ether, respectively. The resulting lyophilized powders were placed in vials, capped, and kept at −20 °C until use.
2.4.2. Dechlorophyllization using sedimentation. Sedimentation technique was used as tailored by Tagrida and Benjakul13 for the dechlorophyllization. Firstly, ethanolic extract was prepared from BLP as described by Tagrida and Benjakul.13 After removing the ethanol by a rotary evaporator at 40 °C, the distilled water was added to the concentrated extract at 1
:
1 ratio (v/v). Sedimentation was then allowed to occur at 4 °C for 24 h. After sedimentation and being centrifuged at 10
000×g and 4 °C for 30 min, the supernatant was collected and lyophilized. The dried extract termed “BLEE-SED” was placed in vial, capped, and kept at −20 °C before analysis.
2.5. Analyses
2.5.1. Extraction yield. The yield of the extracts was considered as the dry weight of the final extracts relative to the initial dry weight of their corresponding powder. Yield was calculated as per the method of Tagrida and Benjakul.13
2.5.2. Chlorophyll content and color. To determine chlorophyll content, dry extracts without and with dechlorophyllization were dissolved in 70% (v/v) ethanol to obtain a concentration of 2 mg ml−1. Chlorophyll content was measured as guided by Olatunde et al.4 The same solutions were used for the determination of color attributes (L*, a*, b*, chroma, and hue angle) with the aid of a colorimeter (Hunter Lab's Colorflex, Reston, USA).4
2.5.3. Total phenolic content (TPC). Folin–Ciocalteu's reagent (FCR) was employed for TPC determination of the extracts without and with chlorophyll removal using various methods. The assay outlined by Benjakul et al.9 was applied for determination of TPC. TPC was calculated and expressed as mg gallic acid equivalent (GAE) per g dry extract.
2.5.4. Total flavonoid content (TFC). The colorimetric assay tailored by Wonghirundecha et al.18 was used for the measurement of TFC. Absorbance of the solutions was read at 510 nm. TFC was calculated from standard curve of catechin (0–250 mg l−1) and expressed as mg catechin equivalent (CE) per g dry extract.
2.5.5. Determination of antioxidant activity. The procedure of Benjakul et al.9 was followed for estimating DPPH radical scavenging activity (DPPH-RA), ABTS radical scavenging activity (ABTS-RA), and ferric reducing antioxidant power (FRAP). Trolox standard curve was used for the calculation of activities and the results were expressed as μmol Trolox equivalents (TE) per g dry extract. Metal chelating activity (MC-A) was measured.9 Calculation was done using a standard curve of EDTA and expressed as μmol EDTA equivalents (EE) per g dry extract.
2.5.6. Determination of antibacterial activity.
2.5.6.1. Bacterial strains. Selected Gram-positive bacteria (G+-Bac) and Gram-negative bacteria (G−-Bac) were assessed for inhibition by the extracts without and with dechlorophyllization processes. The bacteria used included Escherichia coli DMST 4122 and Staphylococcus aureus DMST 4547, obtained from the Department of Medical Science, Ministry of Health, Thailand. Listeria monocytogenes PSU.SCB.16S.13 and Pseudomonas aeruginosa PSU.SCB.16S.12, were gifted from Food Safety Laboratory, Department of Food Technology, Prince of Songkhla University, Hat Yai, Thailand. Culture conditions and cell suspension were maintained as delineated by Olatunde et al.4
2.5.6.2. Minimum inhibitory concentration (MIC) and minimum bactericidal concentration (MBC). The method of Olatunde et al.16 was adopted to determine the MIC and MBC of BLEE without and with dechlorophyllization. Samples (100 μl) with diversified concentrations (50 to 0.097 mg ml−1) prepared in tryptic soy broth (TSB), were placed into a sterile 96 flat shaped multi wells, and then 100 μl of a 0.5 McFarland standard (108 colony forming unit (CFU) ml−1) bacterial suspension were inoculated into all the wells. Extracts at a concentration of 50 mg ml−1 without bacterial suspension were served as negative control, while bacterial suspensions devoid of extracts were considered as positive control. After 24 h incubation period at 37 °C, resazurin solution (0.2 mM, 50 μl) was added to all the wells. MIC was assessed visually for color change and it was defined as the lowest concentration, which completely inhibited bacterial growth. Before adding the resazurin solution, aliquots (15 μl) from wells showing no apparent bacterial growth were inoculated into tryptic soy agar (TSA) plates. Subsequently, the plates were incubated at 37 °C for 24 h. Least concentration with no bacterial growth was recorded as MBC.
2.5.6.3. Time-kill kinetics. BLEE with the lowest MIC and MBC was selected to study its killing rate against G+-Bac and G−-Bac. The experiment was conducted as tailored by Odedina et al.19 The selected BLEE solutions (1.5 ml) at 0.5, 1, 2, and 4 MIC were inoculated with a 1.5 ml of 0.5 McFarland bacterial suspension from each growing culture (108 CFU ml−1) prepared previously in TSB. All culture media were incubated for 24 h at 37 °C. Plate count method was used for enumerating the surviving cells at time 0, 2, 4, 6, 8, 12, and 24 h. Control culture with no extract was incubated and enumerated in the same manner. The experiment was performed in duplicate for three independent studies (detection limit: 102 CFU).
2.5.6.4. Scanning electron microscopy (SEM). The BLEE showing the lowest MIC level on G+-Bac and G−-Bac was selected for treatment of bacteria.20 All bacterial cells were visualized and photographed with the aid of scanning electron microscope (FEI Quanta 400-FEG SEM, Oregon, USA). Bacterial culture untreated with the selected BLEE was used as the control.
2.5.7. Influence of the selected BLEE on shelf-life extension of Nile tilapia fillets during refrigerated storage.
2.5.7.1. Preparation of tilapia fillets. Deceased Nile tilapia (Oreochromis niloticus) (around 4 kg; 1 ± 0.1 kg per fish) were purchased from the local market at Hat Yai, Songkhla, Thailand. Those fish (approximately 3 h after capture from fish farm in Songkhla) were kept in ice (ice/fish ratio of 2
:
1 (w/w)) and transported within 30 min to the International Center of Excellence in Seafood Science and Innovation (ICE-SSI), Prince of Songkhla University, where they were immediately washed upon arrival. Subsequently, fish were decapitated, skinned, deboned, and filleted using stainless-steel knife. Average weight of the fillets was approximately 100 ± 5 g.
2.5.7.2. Pretreatment of tilapia fillets with the selected BLEE extract. The selected extract BLEE-SED at known amount was dissolved in the minimum volume of distilled water. Tilapia fillets were mixed manually with the prepared extract to obtain the levels of 200, 400, and 600 ppm. For fillet treated with BLEE-SED (600 ppm), sensory evaluation was performed in comparison to the control (no treatment) by 50 untrained panelists. The fish fillets were steamed as tailored by Olatunde et al.20 before serving. There was no difference in all attributes (color, odor, flavor, texture, and overall likeness) between the control and that treated with BLEE-SED (p > 0.05).Untreated and treated fillets (100 ± 5 g) were separately placed on polystyrene trays, wrapped tightly with shrink film, and stored at 4 °C. Sampling for analyses was done at day 0, 3, 6, 9, and 12 of storage.
2.5.7.3. Microbiological analyses. Firstly, fillets (10 g) were added with 90 ml of sterilized saline solution (0.85%) in stomacher bag. Blending was done using a stomacher blender (M400, Seward Ltd., West Sussex, England) for 1 min at 220 rpm, followed by serial dilutions using 0.85% saline solution. Spread plate method with the appropriate dilutions was used for monitoring microbiological changes in tilapia fillets.5 Psychrophilic bacterial count and total viable count were enumerated on plate count agar after incubation at 4 °C for 10 days and 37 °C for 3 days, respectively. Triple sugar iron agar and Pseudomonas isolation agar were used for evaluating H2S producing bacteria and Pseudomonas sp. counts, respectively, after 3 days incubation period at 25 °C. Counts of Enterobacteriaceae sp. were evaluated after being incubated at 37 °C for 24 h using eosin methylene blue agar.
2.5.7.4. Chemical analyses. Total volatile base (TVB) content was determined as tailored by Shiekh et al.5 and reported as mg N per 100 g fish meat. Peroxide value (PV) expressed as mg cumene hydroperoxide per kg fish meat, was evaluated.21 Thiobarbituric acid reactive substances (TBARS) were examined as per the method of Olatunde et al.20 The values were expressed as mg MDA per kg fish meat. The pH of fish homogenate in distilled water (2
:
10, w/v) was measured using a pH meter (Lab 855, SI analytics, Weilheim, Germany).
2.6. Statistical analyses
Completely randomized design (CRD) was applied for the whole study. Experiments were carried out in triplicates (n = 3). One-way Analysis of Variance (ANOVA) and the Duncan's Multiple Range Test (DMRT) for mean comparison were performed for the analysis of data using SPSS package (SPSS 22.0 for Windows, SPSS Inc., Chicago, IL, USA) software.
3. Results and discussion
3.1. Effect of different dechlorophyllization methods on chlorophyll content of betel leaf ethanolic extracts (BLEEs)
Compared to the control, BLEE after dechlorophyllization showed varied chlorophyll contents (Table 1). BLEE dechlorophyllized using organic solvents showed the decreases in chlorophyll A, chlorophyll B and total chlorophyll contents (p < 0.05). No difference in total chlorophyll content was observed between BLEE-PET and BLEE-CF (p > 0.05). Among all the extracts with dechlorophyllization, BLEE-ACT had the highest total chlorophyll contents, indicating that petroleum ether and chloroform were more efficient for chlorophyll removal than acetone. Olatunde et al. also documented that non-polar solvents were more effective in chlorophyll removal than polar counterparts.4 Chlorophyll is an amphiphilic compound containing a hydrophilic head of porphyrin ring, where magnesium atom is located at the center, and a long lipophilic hydrocarbon tail is linked by an ester bond to this porphyrin ring.13 Polarity of the organic solvents used for dechlorophyllization may affect the efficiency of the process. Organic solvents can access into plant cell via membrane, thus dissolving the lipids and lipoprotein of the plant cell organelles and releasing their contents.4 Chloroform and petroleum ether are strong non-polar solvents, while acetone is a polar aprotic solvent. Therefore, more chlorophyll was dissolved in these non-polar solvents, which were further discarded. On the other hand, considerably higher chlorophyll content was remained in the extract dechlorophyllized using acetone.
Table 1 Chlorophyll contents and color of betel leaf ethanolic extract (BLEE) before and after dechlorophyllization using different organic solvents and sedimentation processa
Samples |
Chlorophyll A (mg g−1) |
Chlorophyll B (mg g−1) |
Total chlorophyll (mg g−1) |
L* |
a* |
b* |
Chroma |
Hue angle |
ΔE* |
Values are mean ± standard deviation (n = 3). Different lowercase superscripts within the same column indicate significant difference (p < 0.05). |
BLEE-CON |
0.488 ± 0.004a |
1.055 ± 0.028a |
1.543 ± 0.025a |
0.306 ± 0.08d |
−0.998 ± 0.07d |
−3.602 ± 0.49c |
3.74 ± 0.46a |
254.22 ± 2.96a |
91.62 ± 0.08a |
BLEE-ACT |
0.388 ± 0.003b |
0.875 ± 0.012b |
1.263 ± 0.015b |
0.952 ± 0.06c |
−0.744 ± 0.16c |
−1.58 ± 0.14b |
1.75 ± 0.12b |
244.84 ± 6.02ab |
90.9 ± 0.06b |
BLEE-CF |
0.313 ± 0.015c |
0.731 ± 0.003c |
1.044 ± 0.012c |
1.172 ± 0.06b |
−0.604 ± 0.06b |
−1.186 ± 0.17a |
1.33 ± 0.17c |
242.81 ± 2.91ab |
90.67 ± 0.06bc |
BLEE-PET |
0.312 ± 0.018c |
0.712 ± 0.009c |
1.024 ± 0.019c |
1.196 ± 0.06b |
−0.552 ± 0.04b |
−1.008 ± 0.64a |
1.14 ± 0.06c |
229.31 ± 27.61b |
90.65 ± 0.06bc |
BLEE-SED |
0.082 ± 0.007d |
0.184 ± 0.006d |
0.267 ± 0.009d |
1.82 ± 0.21a |
0.838 ± 0.11a |
−0.982 ± 0.41a |
1.29 ± 0.08c |
130.66 ± 6.56c |
90.48 ± 0.53c |
When sedimentation process was used for chlorophyll removal, it was found that this process had more profound effect as compared to conventional dechlorophyllization methods using organic solvents (p < 0.05). Water added to the extract after ethanol removal made the medium more polar, causing the chlorophyll molecules to precipitate and leaving the top layers with negligible chlorophyll. It could be obviously detected by the difference in color between the top and lower layers of the extract. Extracts dechlorophyllized using organic solvents still had substantive amount of chlorophyll remained after extraction. Therefore, BLEE-SED had less chlorophyll contents (p < 0.05) than the other extracts. This finding was similar to results observed by Tagrida and Benjakul13 who found that betel leaf extract dechlorophyllized by sedimentation using all water/extract ratios (1
:
1, 1
:
2, and 1
:
3) had less chlorophyll content as compared to the control (without dechlorophyllization). Water was able to increase the polarity of the medium, thus allowing the chlorophyll molecules which are non-polar in nature to precipitate and subsequently removed. This process yielded the extract with less chlorophyll content. Similar trend was found with chlorophyll A and chlorophyll B, in which the control had the highest contents. Also, the extract dechlorophyllized using sedimentation process (BLEE-SED) had the lowest chlorophyll A and B contents (p < 0.05). Chlorophyll A and B are types of chlorophyll that aid in absorbing the light required for photosynthesis, chlorophyll A is an electron donor in electron transport system, while chlorophyll B expands the absorption spectrum, thus converting a wider range of sun energy into chemical energy.22 Chlorophyll B was more dominant in all extracts, which was in tandem with the report of Olatunde et al.4 Usually, chlorophyll A content is higher than chlorophyll B content, however certain environmental conditions may lead to the difference in the chlorophyll a/b content ratio. The insufficient exposure to sunlight (shading) could make the betel leaves fight with other plants or themselves over sunlight.23 This would induce the production of higher levels of chlorophyll B in the leaves to capture as much sunlight as they could, whereas chlorophyll A cannot carry on photosynthesis.24 Hence, betel leaves appear dark green and have higher content of chlorophyll B.
3.2. Effect of different dechlorophyllization methods on the color of BLEEs
When estimating the color of the extracts (Table 1), all the dechlorophyllized extracts had different colors from the control (p < 0.05). Among the extracts dechlorophyllized using organic solvents, BLEE-CF and BLEE-PET showed similar L*, a*, and b* values (p > 0.05). However, both the extracts exhibited higher L*, a*, and b* values than BLEE-ACT (p < 0.05). Lightness (L*) was higher in BLEE-CF and BLEE-PET than BLEE-ACT, indicating ineffectiveness of acetone to remove pigments, mainly chlorophylls of the extracts, compared to chloroform or petroleum ether. This was also ascertained by greenness/redness (a*) parameter, which showed less negative value in BLEE-CF and BLEE-PET than BLEE-ACT. This signified less greenness in both extracts than BLEE-ACT. Yellowness (b*) was higher in BLEE-CF and BLEE-PET, reflecting more yellowness for both extracts than BLEE-ACT. It was noted that BLEE-SED had the highest L*, a*, and b*, denoting that this extract was lighter with lesser greenness and slightly pale yellowish than other extracts dechlorophyllized by organic solvents (p < 0.05). This reconfirmed the ability of sedimentation process to reduce the color of betel leaf extract more efficiently than organic solvents. A negative correlation was observed between chlorophyll content of the extracts and their color parameters (L*, a* and b*), while it was positively correlated with the chroma and hue angle (h°). This indicated the influence of chlorophyll on the color of the extracts and implied that the higher the chlorophyll content in the extract, the color, especially greenness, was more dominant. The results reconfirmed that sedimentation process had the potential in removal of chlorophyll, thus lowering greenness caused by chlorophylls.
3.3. Extraction yield, total phenolic content (TPC) and total flavonoid content (TFC) of BLLEs
Extraction yields were different (p < 0.05) among all the extracts and the control (Table 2). BLEE-CON showed highest yield, mostly attributed to the abundance of chlorophyll, while differences in yields between BLEE-ACT, BLEE-CF and BLEE-PET could possibly be governed by solvents used. Different types of organic solvents used differ in polarity and penetration capacity into plant cell walls. Thus, the targeted products got solvated and separated from the structural components differently.25 Lowest yield was observed in BLEE-SED, in which the settled chlorophyll was removed from the extract, thereby reducing its final yield. Extraction yield of the extracts dechlorophyllized using organic solvents was higher than that of BLEE-SED.
Table 2 Extraction yield, total phenolic content, total flavonoid content and antioxidant activities of betel leaf ethanolic extract (BLEE) before and after dechlorophyllization using different organic solvents and sedimentation processa
Samples |
Extraction yield (%) |
TPC (mg GAE per g dry extract) |
TFC (mg CE per g dry extract) |
DPPH-RA (μmol TE per g dry extract) |
ABTS-RA (μmol TE per g dry extract) |
FRAP (μmol TE per g dry extract) |
MC-A (μmol EDTA per g dry extract) |
Values are mean ± standard deviation (n = 3). Different lowercase superscripts within the same column indicate significant difference (p < 0.05). |
BLEE-CON |
14.93 ± 0.02a |
130.6 ± 3.86b |
108.94 ± 4.47b |
105.67 ± 1.62a |
2164.2 ± 36.09b |
1213.04 ± 5.2b |
149.64 ± 5.32b |
BLEE-ACT |
12.90 ± 0.09b |
98.72 ± 5.07c |
64.05 ± 4.91c |
84.66 ± 0.44b |
1766.3 ± 16.16c |
998.43 ± 5.8c |
131.88 ± 1.75c |
BLEE-CF |
12.38 ± 0.02c |
69.27 ± 4.92d |
48.44 ± 4.34d |
80.45 ± 3.11c |
1529.6 ± 38.88d |
788.17 ± 7.7d |
92.46 ± 3.27d |
BLEE-PET |
12.25 ± 0.01d |
53.54 ± 6.45e |
32.16 ± 6.72e |
75.58 ± 2.08d |
1168.7 ± 36.02e |
693.04 ± 8.06e |
83.97 ± 2.25e |
BLEE-SED |
11.06 ± 0.01e |
171.73 ± 4.42a |
134.05 ± 2.42a |
108.74 ± 0.21a |
2503.03 ± 24.2a |
1394.14 ± 4.34a |
220.11 ± 2.3a |
TPC of the extracts varied (Table 2). BLEE-SED had the highest TPC followed by BLEE-CON, whereas lower TPC was observed in the extracts dechlorophyllized using organic solvents. Previous studies also reported lower TPC of extracts from lead (Leucaena leucocephala) seeds and guava leaves, after dechlorophyllization using organic solvents.4,9 The reduction in TPC could be related to the loss in polyphenols from these extracts during dechlorophyllization process. The differences in TPC among BLEE-ACT, BLEE-CF and BLEE-PET were related to the varying polarities of different solvents along with their penetration capacity through the plant cells. Higher TPC in BLEE-SED than other extracts could be associated with high solubility of polyphenols in aqueous phase, while chlorophyll was precipitated.26 Therefore, addition of water to ethanolic extract at appropriate ratio could increase the polarity of the extraction medium, causing the chlorophyll molecules to settle and increase the proportion of polyphenols in the final extract. Loss of polyphenols during dechlorophyllization using solvents as well as remaining chlorophyll more likely reduced TPC of the extracts. These drawbacks were depleted in sedimentation process, making this technique more effective in chlorophyll removal as well as augmenting TPC.
Difference in TFC of extracts showed similar trend to that of TPC (p < 0.05), BLEE-SED had the highest TFC (Table 2), and it was observed that TFC of extracts dechlorophyllized using organic solvents was drastically reduced. This might be attributed to co-extraction of flavonoids during chlorophyll removal. Difference in TFC among these extracts could be related to difference in polarity of solvents and the solubility of the flavonoids.18 Flavonoids are natural phenolic secondary metabolites found in plants and known for their pharmaceutical and nutraceutical benefits. This is mainly caused by their valuable bioactivities coupled with their abilities to modulate important cellular enzyme functions.27 High chlorophyll content in BLEE-CON plausibly interacted with the flavonoid compounds, causing a reduction in their free form. This affirmed the efficacy of sedimentation process for maintaining polyphenols in the extracts.
3.4. Antioxidant activities
Antioxidant activities of the dechlorophyllized extracts differed (p < 0.05) compared to those of BLEE-CON (Table 2). BLEE-SED exhibited the highest DPPH-RA and ABTS-RA (p < 0.05). It also had the most prominent FRAP and MC-A (p < 0.05). This indicates the higher ability of BLEE-SED to donate electrons to ABTS and DPPH radicals with the profound capability to reduce ferric ions to ferrous ions as well as the higher capacity to chelate transition metals. The reduced activity of extracts dechlorophyllized using organic solvents was plausibly related to the high loss of polyphenols during the process. The difference in activity between these extracts was more likely associated with the differences in the polarity of different solvents used. Higher activity of BLEE-SED was connected to the low chlorophyll content and high TPC, thus augmenting its interactions with radicals. A high positive correlation was noticed between TPC and TFC and the correlation was also obtained between all the antioxidant activities, which suggested that polyphenols within the different extracts associated with antioxidant activities and the difference in their content determined antioxidant activity. Strong correlation between TPC and antioxidant activities confirmed that polyphenols are responsible for antioxidant activities of the extracts.4,9,13
3.5. Antibacterial activities
3.5.1. Minimum inhibitory concentration (MIC) and minimum bactericidal concentration (MBC) of BLEEs. MICs and MBCs of BLEE without and with dechlorophyllization against some G+-Bac and G−-Bac are presented in Table 3. BLEE-SED had the lowest MIC against all bacteria tested, ranging from 0.39 to 3.12 mg ml−1, followed by BLEE-CON. BLEE dechlorophyllized using organic solvents had the higher MIC against the tested bacteria, indicating lower antibacterial activity of these extracts. Differences in MIC values between all the extracts could be related to differences in content and types of polyphenols. These compounds were capable of interacting with the bacterial cell wall, thus causing disturbance to its constituents. This phenomenon promoted the leakage of bacterial cell and penetration of other compounds and subsequently affected the internal organelles and nuclei. It caused growth inhibition or death to the bacterial cells.20 Since BLEE-SED had the highest TPC, its antibacterial activity was greater than the others, which had lesser antibacterial activity (p < 0.05). Effect of the extracts varied among the different bacteria tested. All the extracts had lower inhibiting activity towards G+-Bac. This could be due to the presence of more peptidoglycan layers in their cell wall, giving them higher resistance. MBC is the lowest concentration required from an antibacterial agent to completely kill certain bacterium.20 Lowest MBC values were acquired by BLEE-SED ranging from 1.56 to 6.25 mg ml−1. An antibacterial agent is considered as bactericidal if the MBC is 4 time higher than its corresponding MIC.28 Variations of MBCs among the different extracts were related with variations of MICs as well as the resistivity of the tested bacteria. Due to the higher antioxidant and antibacterial activities of BLEE-SED as well as its lowest chlorophyll content and highest color reduction, it was chosen for further study.
Table 3 Minimum inhibitory concentration (MIC) and minimum bactericidal concentration (MBC) of betel leaf ethanolic extracts (BLEE) before and after dechlorophyllization using different organic solvents and sedimentation processa
Samples |
MICb (mg ml−1) |
MBCb (mg ml−1) |
SA |
EC |
LM |
PA |
SA |
EC |
LM |
PA |
SA, Staphylococcus aureus; EC, Escherichia coli; LM, Listeria monocytogenes; PA, Pseudomonas aeruginosa; MIC, minimum inhibitory concentration; MBC, minimum bactericidal concentration. Different lowercase superscripts within the same column indicate significant difference (p < 0.05). Mean (n = 3). |
BLEE-CON |
3.12c |
1.56c |
3.12c |
0.78b |
6.25c |
6.25c |
6.25b |
1.56c |
BLEE-ACT |
12.5b |
12.5a |
12.5a |
6.25a |
25a |
25a |
25a |
12.5b |
BLEE-CF |
12.5b |
6.25b |
6.25b |
6.25a |
12.5b |
12.5b |
25a |
12.5b |
BLEE-PET |
25a |
12.5a |
12.5a |
6.25a |
25a |
25a |
25a |
25a |
BLEE-SED |
1.56d |
0.39d |
3.12c |
0.78b |
3.12d |
1.56d |
6.25b |
1.56c |
3.5.2. Time – kill kinetics. Different concentrations of BLEE-SED were employed to test its antibacterial efficacy against some G+-Bac (S. aureus and L. monocytogenes) and G−-Bac (P. aeruginosa and E. coli) using time – kill kinetics assay (Fig. 1a). Distilled water with no extract was served as the control. Counts of the control and that treated with 0.5 MIC BLEE-SED continuously increased, however lower growth rate for BLEE-SED at 0.5 MIC was obtained compared to the control (p < 0.05) after 24 h of incubation, suggesting that BLEE-SED had quite low antibacterial activity at that concentration. A reduction ≥ 3
log CFU ml−1 in bacterial count is defined as bactericidal activity, while reduction lower than that value is considered as bacteriostatic activity.29 At MIC level (1.56 mg ml−1), BLEE-SED exhibited a bacteriostatic activity toward S. aureus with a 0.96
log CFU ml−1 reduction after 24 h of incubation, while it was about 0.88
log CFU ml−1 reduction for L. monocytogenes at MIC level (3.12 mg ml−1). The reduction rate was 1.46 and 1.12
log CFU ml−1 for P. aeruginosa and E. coli, respectively, at their MIC level (0.78 and 0.39 mg ml−1, respectively). Bactericidal activity was achieved after 24 h at 4 MIC for all the tested isolates as the reduction rate surpassed the 3
log CFU ml−1 limit. For S. aureus and L. monocytogenes, it was 3.07 and 3.01
log CFU ml−1 reduction, respectively. The reduction rate was 3.43 and 3.31
log CFU ml−1 for P. aeruginosa and E. coli, respectively. At 2 MIC level, BLEE-SED exhibited a bactericidal activity only on P. aeruginosa and E. coli with reduction rate of 3.1 and 3.06
log CFU ml−1, respectively. The same level of BLEE-SED had a bacteriostatic activity on S. aureus and L. monocytogenes with 2.91 and 2.5
log CFU ml−1. The reduction rate is noted to be higher for the G−-Bac (P. aeruginosa and E. coli) than that of the G+-Bac at all concentrations used. This was mainly attributed to the lack of thick layers of peptidoglycan in the cell walls of the G−-Bac,20 making those more sensitive towards BLEE-SED. Nevertheless, BLEE-SED showed notable antibacterial efficacy at various concentrations for inhibiting the growth of both selected G+-Bac and G−-Bac.
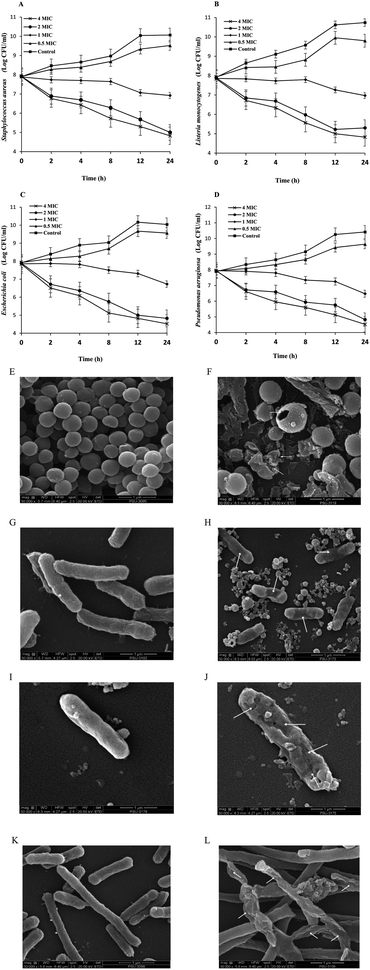 |
| Fig. 1 Time-kill curves toward different microorganisms (a) including Staphylococcus aureus (A), Listeria monocytogenes (B), Escherichia coli (C) and Pseudomonas aeruginosa (D) without and with BLEE-SED treatment at different concentrations and scanning electron microscope photographs (b) of treated and untreated Staphylococcus aureus (E and F), Listeria monocytogenes (G and H), Escherichia coli (I and J), and Pseudomonas aeruginosa (K and L). Magnification ×30 000. Bars represent standard deviation (n = 3). | |
3.5.3. Effect of BLEE-SED on morphology of bacterial cells. Effect of BLEE-SED on tested bacteria morphology was visualized by comparing the morphology of their cell walls before and after treatment with BLEE-SED at MIC level with the aid of scanning electron microscope (×30
000). Bacterial cell walls without treatment (control) appeared intact with no apparent damage (Fig. 1E, G, I and K). On the other hand, the difference could be detected after treatment with BLEE-SED, as the cell walls of S. aureus, L. monocytogenes, P. aeruginosa and E. coli appeared shrunken or destroyed as pointed by arrows (Fig. 1F, H, J and L). A number of pours were obtained. Leakage of cytoplasmic substances from bacterial cells as a consequence of destruction of their cell walls by coconut husk ethanolic extract was also reported by Olatunde et al.20 When bacterial organelles and DNA were destroyed, their division and growth abilities as well as their cell wall repair mechanisms were negatively affected. Deformity of the bacterial cells took place when Rhodomyrtus tomentosa ethanolic leaf extract was applied.19
3.6. Influence of BLEE-SED on quality changes of tilapia fillets during refrigerated storage
3.6.1. Changes in microbial load. Microbiological changes of tilapia fillets treated with BLEE-SED at different concentrations were assessed by monitoring bacterial count during 12 days of storage (Fig. 2). TVC of the control and samples treated with BLEE-SED at various concentrations at day 0 ranged from 3.8 to 4.0
log CFU g−1, indicating that microbial contamination probably took place during the preparation of samples. Microbial load increased rapidly (p < 0.05) to 6.8
log CFU g−1 for the control at day 3. The growth continued to reach 8.3
log CFU g−1 at day 12 of storage. The marginal limit accepted for fresh and marine water species is 6.0
log CFU g−1.19 TVC of the treated samples still had the increase in bacterial count (p < 0.05). Nevertheless, the increase rate was lower than that of the control. After 6 days, samples treated with 200 ppm BLEE-SED had TVC of 6.2
log CFU g−1, while samples treated with 400 and 600 ppm of the BLEE-SED had the load lower than the acceptable marginal limit after day 9, reaching 5.7 and 5.5
log CFU g−1, respectively. These results implied the ability of BLEE-SED at 400 and 600 ppm to retard the microbial proliferation up to 9 days of storage at 4 °C. Asian sea bass slices stored under refrigerated storage had a reduced TVC when treated with coconut husk ethanolic extract.20 At day 12, the samples treated with BLEE-SED at 400 and 600 ppm had TVC of 6.21 and 6.13
log CFU g−1, respectively.
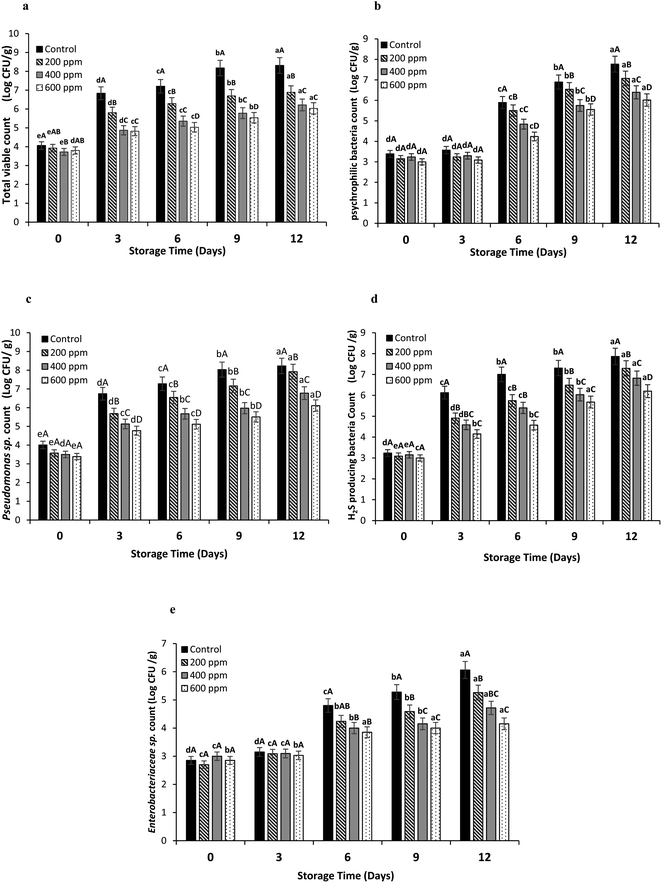 |
| Fig. 2 Total viable count (a), psychrophilic bacteria count (b), Pseudomonas sp. count (c), hydrogen sulfide producing bacteria count (d), and Enterobacteriaceae sp. count (e) of Nile tilapia fillets without and with the treatment by BLEE-SED at various concentrations during 12 days of storage at 4 °C. Bars represent standard deviation (n = 3). Different lowercase letters on bars within same treatment indicate significant difference (p < 0.05). Different uppercase letters on bars within the same storage time indicate significant difference (p < 0.05). | |
Psychrophilic bacteria count (PBC) for the control and samples treated with BLEE-SED at various concentrations was augmented rapidly after day 3 (p < 0.05). However, this increase varied among the control and the treated samples (p < 0.05). PBC of the control exceeded the acceptable marginal limit of 6.0
log CFU g−1, reaching 6.8
log CFU g−1 at day 9, while the treated samples had the microbial load within the acceptable range. All the treated samples exceeded the limit at day 12 except the sample treated with 600 ppm BLEE-SED, which had PBC of 6.01
log CFU g−1. The result signified the effectiveness of BLEE-SED at high concentration to retard the growth of psychrophilic bacteria.
Lower Pseudomonas count was found as the concentration of BLEE-SED increased (p < 0.05). Untreated samples (control) had the highest count at day 12 with the count of 8.2
log CFU g−1. Pseudomonas count reached 7.9, 6.7, and 6.1
log CFU g−1 at day 12 when treated with 200, 400, and 600 ppm BLEE-SED, respectively. Although the count was augmented for the treated samples (p < 0.05), the increasing rate was lesser than that of the control (p < 0.05), suggesting the capability of the extract especially at an elevated concentration to reduce the proliferation rate of Pseudomonas sp. Pseudomonas sp. were closely connected to food spoilage incidents.21 They are known for their high survival rate in chilled temperatures, majorly causing food spoilage. Different plant extracts were used for retardation of Pseudomonas growth, thus avoiding their contribution to spoilage.30
Hydrogen sulfide (H2S) producing bacteria count in all the samples increased up to 12 days. It was noted that the samples treated with BLEE-SED at higher concentration (p < 0.05) had the lower increasing rate for H2S producing bacteria. At day 12, untreated samples had the highest count (7.8
log CFU g−1) followed by samples treated with BLEE-SED at 200, 400 and 600 ppm having the counts of 7.2, 6.8, 6.2
log CFU g−1, respectively. The result confirmed the effectiveness of BLEE-SED for retarding the growth of spoilage bacteria such as the H2S producing bacteria which is known for its ability to breakdown amino acids like methionine and L-cysteine along with other proteins found in fish meat, thus producing the unpleasant odor of H2S.31
The count of Enterobacteriaceae sp. was generally lower than those of other bacteria. The presence of these bacteria was associated with inappropriate or unhygienic preparation and handling of fish.32 Although, low count of Enterobacteriaceae sp. was detected, the growth could take place when favorable conditions for their growth became dominant. The control samples had an increase in Enterobacteriaceae sp. count by 6.06 log
CFU g−1, while the counts were 5.2, 4.7, and 4.1 log
CFU g−1 for 200, 400, and 600 ppm BLEE-SED treated samples, respectivley, at day 12. An initial count ranging from 2.6 to 3
log CFU g−1 was noticeable. This suggested the ability of BLEE-SED to inhibit Enterobacteriaceae sp. growth in tilapia fillets during storage.
3.6.2. Changes in chemical characteristics. Total volatile base (TVB) content is an indicator for the quality of fish and fish products. The elevated TVB content reflects poor quality of fish as a consequence of spoilage, induced mainly by proliferation of microorganisms.7 For the control and treated samples (Fig. 3a), TVB contents were in the range of 5.9–6.8 mg N per 100 g at day 0. At day 12, TVB content of the control was drastically increased and reached 62.8 mg N per 100 g (p < 0.05). TVB contents of the treated sample were also increased, however the increasing rate was lesser than the control (p < 0.05). After 12 days, samples treated with 200, 400, and 600 ppm BLEE-SED had TVB contents of 44.6, 30.5, and 22.3 mg N per 100, respectively. TVB content of 30–35 mg N per 100 g is considered as an accepted limit for fish freshness.33 Therefore, treatment with BLEE-SED at 400 and 600 ppm could notably lower the formation of degradable products mediated by microorganisms.
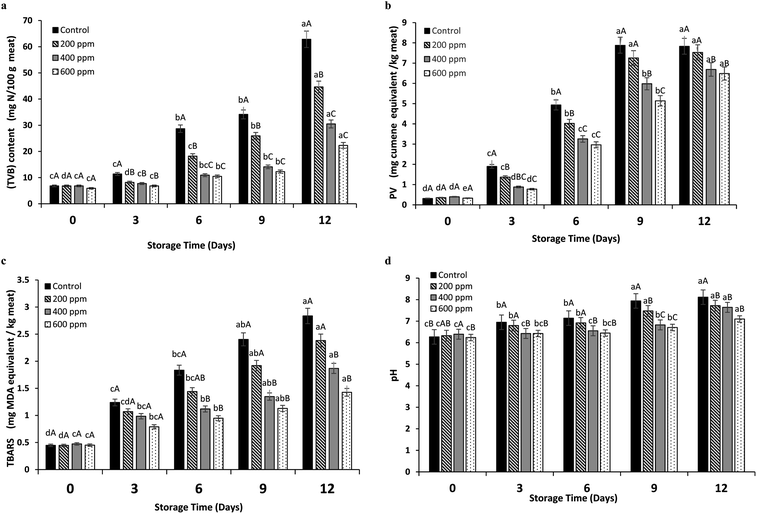 |
| Fig. 3 Total volatile base (TVB) content (a), peroxide value (PV) (b), thiobarbituric acid reactive substances (TBARS) (c), and pH (d) of Nile tilapia fillets without and with the treatment by BLEE-SED at various concentrations during 12 days of storage at 4 °C. Bars represent standard deviation (n = 3). Different lowercase letters on bars within same treatments indicate significant difference (p < 0.05). Different uppercase letters on bars within the same storage time indicate significant difference (p < 0.05). | |
During the storage, tilapia fillets underwent lipid oxidation as ascertained by hydroperoxides formed (Fig. 3b). Untreated samples showed high PV as compared to the samples treated with BLEE-SED at high concentrations (p < 0.05) during storage. No difference (p > 0.05) between PVs of the control at days 9 and 12 of storage was noted, as it reached 7.88 and 7.83 mg cumene hydroperoxide per kg, respectively. This suggested that the formation of primary oxidation products was retarded, and decomposition occurred to high extent. Similar observations were reported previously in fish slices treated with plant extracts.20 Lower PVs were found in samples treated with 400 and 600 ppm BLEE-SED after 12 days of storage (6.68 and 6.48 mg cumene hydroperoxide per kg, respectively), while samples treated with 200 ppm BLEE-SED had similar PV to the control (p > 0.05). Thus, it can be concluded that BLEE-SED at 400 and 600 ppm could retard the auto-oxidation of fats in tilapia fillets owing to its antioxidant activities.
At beginning of storage, TBARS values were in the range of 0.44–0.47 mg MDA per kg for the untreated and treated samples with BLEE-SED (Fig. 3c). TBARS are decomposition products of lipid peroxidation. Their formation is linked to high quantity of unsaturated fatty acids within samples, which underwent oxidation rapidly.34 Presence of high microbial load could lead to enzymatic degradation, thus liberation of free fatty acids that are easily oxidized.35 The increasing rate of TBARS values was lesser in the treated samples than the control throughout storage (p < 0.05). At day 12, samples treated with BLEE-SED at 400 and 600 ppm had lower TBARS values (1.86 and 1.42 mg MDA per kg, respectively) than the control (2.83 mg MDA per kg). Treatment with 200 ppm BLEE-SED showed no difference in TBARS (p > 0.05) from the control (2.38 mg MDA per kg). Therefore, high oxidation of lipids induced by free radicals and enzymatic degradation mediated by microorganisms was retarded by the treatment of BLEE-SED at 400 or 600 ppm.
An increase in the pH was noted during storage of the control and the treated tilapia fillets with BLEE-SED at different concentrations (p < 0.05). The increase in the pH could be related to the formation of basic compounds like ammonia and trimethylamine (TMA) as induced by proliferation of microorganisms during storage.20 At day 0, the pH of the control and BLEE-SED treated samples was in the range of 6.2–6.3 (p > 0.05). At day 12, pH of the control increased rapidly to reach 8.1, while pH of samples treated with 600 ppm BLEE-SED was 7.1 which was slightly lower than those samples treated with 200 and 400 ppm BLEE-SED with pH of 7.72 and 7.64, respectively. The lower pH of the treated samples was in accordance with their lower TVB content and microbial load, affirming the ability of BLEE-SED to retard microbial proliferation associated with lower decomposition of the samples.
Based on the keeping quality of tilapia fillets, BLEE-SED showed superior antioxidant and antibacterial properties to other extracts. That might be caused by the different types and contents of active components present in this extract. Recently, Tagrida and Benjakul13 found that compounds such as vitexin 4′-O-galactoside and isovitexin were the major components identified in betel leaf ethanolic extracts dechlorophyllized using sedimentation process. These compounds were reported to have antioxidant, antimicrobial and anticancer activities.36 Kaempferol derivatives, hydrocinnamic acid, citric acid and epigallocatechin were also detected. Those compounds are known for their high bioactivities including antimicrobial activity and can be used in several nutraceutical and pharmaceutical applications.37,38
4. Conclusion
Betel leaf ethanolic extract dechlorophyllized by sedimentation (BLEE-SED) showed superior antioxidant and antibacterial activities to other extracts dechlorophyllized using organic solvents. It had lowest chlorophyll content and pale color. It was also rich in phenolic compounds and flavonoids. This led to higher antioxidant and antibacterial activities of BLEE-SED. Nile tilapia fillets treated with BLEE-SED, especially at 400 and 600 ppm, had the retarded chemical changes as well as lowered microbial growth than the untreated samples during 12 days of storage at 4 °C, indicating the efficiency of BLEE-SED in lowering microbial proliferation during storage and reducing quality deterioration. Thus, BLEE-SED at 400 or 600 ppm could be used for shelf-life extension of Nile tilapia fillets up to 9 days without any discoloration or change in taste. BLEE-SED could be used as the natural antioxidant in foods rich in lipids, which are prone to the oxidation and as the natural preservative, which can be applied in perishable foods, such as shellfish, meat or poultry, etc. In addition, it could be applied in pharmaceutical industries, particularly nutraceutical or functional ingredient for health benefit in growing functional food market.
Conflicts of interest
There are no conflicts to declare.
Acknowledgements
The authors were deeply grateful for the generous financial support, provided by the Prince of Songkhla University (PSU), Hat Yai (Grant No. AGR6402088N).
References
- L. M. Neira, S. P. Agustinelli, R. A. Ruseckaite and J. F. Martucci, Packag. Technol. Sci., 2019, 32, 471–480 CrossRef CAS.
- G. Bono, C. O. R. Okpala, S. Vitale, V. Ferrantelli, A. D. Noto, A. Costa, C. Di Bella and D. L. Monaco, Food Sci. Nutr., 2017, 5, 1049–1056 CrossRef CAS PubMed.
- A. Hassoun and Ö. E. Çoban, Trends Food Sci. Technol., 2017, 68, 26–36 CrossRef CAS.
- O. O. Olatunde, S. Benjakul and K. Vongkamjan, J. Food Biochem., 2018, 42, e12600 CrossRef.
- K. A. Shiekh, S. Benjakul and T. Sae-Leaw, Food Chem., 2019, 270, 554–561 CrossRef CAS PubMed.
- P. Srisapoome and N. Areechon, Fish Shellfish Immunol., 2017, 67, 199–210 CrossRef CAS PubMed.
- A. G. Tacon, Rev. Fish. Sci., 2020, 28, 43–56 CrossRef.
- B. Salehi, Z. A. Zakaria, R. Gyawali, S. A. Ibrahim, J. Rajkovic, Z. K. Shinwari, T. Khan, J. Sharifi-Rad, A. Ozleyen and E. Turkdonmez, Molecules, 2019, 24, 1364 CrossRef PubMed.
- S. Benjakul, P. Kittiphattanabawon, P. Sumpavapol and S. Maqsood, J. Food Sci. Technol., 2014, 51, 3026–3037 CrossRef CAS PubMed.
- L. C. Pabón-Baquero, Á. M. Otálvaro-Álvarez, M. R. R. Fernández and M. P. Chaparro-González, Antioxidants in Foods and Its Applications, 2018 Search PubMed.
- T. Li, J. Xu, H. Wu, G. Wang, S. Dai, J. Fan, H. He and W. Xiang, Mar. Drugs, 2016, 14, 162 CrossRef PubMed.
- A. Udayan, M. Arumugam and A. Pandey, in Algal Green Chemistry, Elsevier, 2017, pp. 65–89 Search PubMed.
- M. Tagrida and S. Benjakul, J. Food Biochem., 2020, 44, e13508 CrossRef CAS PubMed.
- K. Tzima, N. P. Brunton and D. K. Rai, J. Food Biochem., 2020, 44, e13148 CrossRef PubMed.
- S. B. Kim, J. Bisson, J. B. Friesen, G. F. Pauli and C. Simmler, J. Nat. Prod., 2020, 83, 1846–1858 CrossRef CAS PubMed.
- O. O. Olatunde, S. L. Della Tan, K. A. Shiekh, S. Benjakul and N. P. Nirmal, Food Chem., 2021, 341, 128251 CrossRef CAS PubMed.
- L. Chotphruethipong, S. Benjakul and K. Kijroongrojana, J. Food Biochem., 2017, 41, e12379 CrossRef.
- S. Wonghirundecha, S. Benjakul and P. Sumpavapol, Songklanakarin J. Sci. Technol., 2014, 36, 300–308 Search PubMed.
- G. F. Odedina, K. Vongkamjan and S. P. Voravuthikunchai, Nutrients, 2015, 7, 7451–7468 CrossRef CAS PubMed.
- O. O. Olatunde, S. Benjakul and K. Vongkamjan, Int. J. Food Sci. Technol., 2019, 54, 810–822 CrossRef CAS.
- Y. A. Arfat, S. Benjakul, K. Vongkamjan, P. Sumpavapol and S. Yarnpakdee, J. Food Sci. Technol., 2015, 52, 6182–6193 CrossRef CAS PubMed.
- M. Chen, Annu. Rev. Biochem., 2014, 83, 317–340 CrossRef CAS PubMed.
- Y. Dai, Z. Shen, Y. Liu, L. Wang, D. Hannaway and H. Lu, Environ. Exp. Bot., 2009, 65, 177–182 CrossRef CAS.
- A. V. Ruban, Commun.
Integr. Biol., 2009, 2, 50–55 CrossRef CAS PubMed.
- K. Miazek, L. Kratky, R. Sulc, T. Jirout, M. Aguedo, A. Richel and D. Goffin, Int. J. Mol. Sci., 2017, 18, 1429 CrossRef PubMed.
- A. Cvetanović, J. Švarc-Gajić, P. Mašković, S. Savić and L. Nikolić, Ind. Crops Prod., 2015, 65, 582–591 CrossRef.
- A. Panche, A. Diwan and S. Chandra, J. Nutr. Sci., 2016, 5, e47 CrossRef CAS PubMed.
- Y. Yuniati, N. Hasanah, S. Ismail, S. Anitasari and S. Paramita, African Journal of Infectious Diseases, 2018, 12, 62–67 CrossRef PubMed.
- F. Campanile, D. Bongiorno, G. Mongelli, G. Zanghì and S. Stefani, Diagn. Microbiol. Infect. Dis., 2019, 93, 77–81 CrossRef CAS PubMed.
- M. Sterniša, F. Bucar, O. Kunert and S. S. Možina, Int. J. Food Microbiol., 2020, 328, 108664 CrossRef PubMed.
- C. Szabo, Biochem. Pharmacol., 2018, 149, 5–19 CrossRef CAS PubMed.
- M. Gullian Klanian, M. Delgadillo Díaz and M. J. Sánchez Solís, High-Throughput, 2018, 7, 25 CrossRef PubMed.
- P. Castro, J. C. P. Padrón, M. J. C. Cansino, E. S. Velázquez and R. M. De Larriva, Food Control, 2006, 17, 245–248 CrossRef CAS.
- J. Monserrat, A. Seixas, M. Ferreira-Cravo, M. Bürguer-Mendonça, S. Garcia, C. Kaufmann Jr and J. Ventura-Lima, Ecotoxicol. Environ. Saf., 2017, 140, 103–108 CrossRef CAS PubMed.
- Y. Xu, L. Li, J. Mac Regenstein, P. Gao, J. Zang, W. Xia and Q. Jiang, Food Chem., 2018, 256, 259–267 CrossRef CAS PubMed.
- F. Babaei, A. Moafizad, Z. Darvishvand, M. Mirzababaei, H. Hosseinzadeh and M. Nassiri-Asl, Food Sci. Nutr., 2020, 8, 2569–2580 CrossRef CAS PubMed.
- Y. Shen, X. Song, L. Li, J. Sun, Y. Jaiswal, J. Huang, C. Liu, W. Yang, L. Williams and H. Zhang, Biomed. Pharmacother., 2019, 111, 579–587 CrossRef CAS PubMed.
- M. S. Mohamed, M. S. Khalil, M. A. Tagrida and Y. A. Mawgoud, Res. J. Pharm., Biol. Chem. Sci., 2017, 8, 914–930 CAS.
|
This journal is © The Royal Society of Chemistry 2021 |
Click here to see how this site uses Cookies. View our privacy policy here.