DOI:
10.1039/D1RA01622A
(Paper)
RSC Adv., 2021,
11, 16275-16284
Curcumin encapsulation in Pickering emulsions co-stabilized by starch nanoparticles and chitin nanofibers
Received
1st March 2021
, Accepted 21st April 2021
First published on 5th May 2021
Abstract
This study examined the stability and release of curcumin encapsulated in Pickering emulsions stabilized by starch nanoparticles and chitin nanofibers under different conditions. Curcumin stability under UV exposure and the release of curcumin from Pickering emulsions in excess water were evaluated over 24 h; while the storage stability of curcumin was assessed over 16 d. The amount of curcumin remaining in the emulsions was quantified spectrophotometrically to characterize its stability and kinetics of release. The progress of lipid oxidation was also monitored by determining peroxide (PV) and p-anisidine (AV) values. The results of passive release measurements indicated over 60% of curcumin was retained after 24 h. SNP/ChF-stabilized Pickering emulsions showed approximately 50% and 45% of curcumin retention upon 16 d of storage and under UV exposure, respectively. Moreover, significant improvement in the curcumin retention was found when higher concentrations of both solid nanoparticles were used. The degradation kinetics of curcumin over storage time and under UV exposure were found to follow first order kinetics. When both emulsifiers were doubled (C4S2), shelf-life was extended to longer than 60 d (AV < 10). This study provides a promising approach to protect encapsulated curcumin, which could potentially be used in functional food products with extended shelf-life.
1. Introduction
Curcumin is a natural polyphenolic compound with many biological and pharmacological activities including anti-inflammatory, antioxidant, anticarcinogenic, antimutagenic and anticoagulant, among others.1–5 Curcumin is safe for human consumption at higher doses (8–12 g day−1) than those needed to trigger health benefits.6–8 These characteristics have motivated researchers to study the possibility of using curcumin as an ingredient in functional food products. However, delivering curcumin to the body has always been a challenge due to its poor solubility in water, rapid metabolic degradation, and rapid elimination from the body.9 Curcumin can be degraded when exposed to UV light through the process of photochemical degradation.10
In recent years, much research has been conducted exploring the ability of different systems to protect bioactive compounds and its delivery efficiency.11,12 Due to the excellent stability and scarce safety concerns of Pickering emulsions, they have been considered as ideal candidates to protect and deliver curcumin.13 In Pickering emulsions, the presence of a dense physical barrier at the interface can hinder curcumin degradation. Tikekar et al. found that a larger amount of curcumin is retained when encapsulated in Pickering emulsions when compared to water.14 Additionally, different aspects of encapsulation of curcumin in Pickering emulsions have been studied, such as photostability, oxidative stability, delivery efficiency, and release of bioactive ingredients.14,15
Among all the Pickering stabilizers, starch nanoparticles have received considerable attention, as they stabilize emulsions by forming a 3-D structure to inhibit the upward movement of droplets.16–17 On the other hand, chitin has shown to have a large impact on emulsion stability. For instance, Zhang et al. found that low concentration of regenerated chitin nanofibers could produce stable emulsions;18 furthermore Zhu et al. effectively prepared high-internal-phase Pickering emulsion using chitin nanofibers.19 Even though a great deal of work has been performed on the use of starch or chitin nanoparticles to stabilize Pickering emulsions in an effort to deliver bioactive ingredients,20–23 the combined use of these nanoparticles to stabilize emulsions as potential delivery system remains largely unexplored.
In a previous work, we demonstrated that the combination of 1% w/v starch nanoparticles (SNPs) and 0.2% w/v chitin nanofibers (ChFs) have a synergistic effect on the physical stabilization of emulsions (Lee, Tarté, & Acevedo, Food Chemistry, submitted). This work focuses on studying encapsulation ability, chemical stability and in vitro release of curcumin using SNP/ChF-stabilized Pickering emulsions. Therefore, in the current study, we prepared and optimized SNP/ChF-stabilized Pickering emulsions in an attempt to determine their suitability as a curcumin delivery system.
2. Materials and methods
2.1. Materials
Waxy corn starch (Novation® Prima 350) was generously provided by Ingredion Incorporated (Westchester, IL, United States), and soybean oil was donated by ADM (Chicago, IL, United States). Chitin powder (from shrimp shells, practical grade, white to brown powder) was purchased from Thermo Fisher Scientific (Waltham, MA, United States), and n-Octenyl Succinic Anhydride (OSA) powder (95% purity, 147 °C flash point) was obtained from TCI America (Portland, OR, United States). Curcumin (mixture of curcumin, demethoxycurcumin, and bisdemethoxycurcumin, 98% assay, 183 °C melting point) was purchased from ACROS Organics (Fair Lawn, NJ, United States). All chemicals used were of analytical grade and commercially available.
2.2. Preparation of starch nanoparticles
Starch nanoparticles were prepared according to the method reported previously (Lee et al., Food Chemistry, submitted). Briefly, 4 g of waxy maize starch powder were dispersed in 200 mL of water and gelatinized at 100 °C for 30 min. The starch solution was cooled to 20 °C, ethanol was poured slowly into the starch solution, and the mixture subsequently centrifuged at 3000×g. The supernatant was discarded, and the precipitate was left in the hood overnight (to allow for the ethanol to evaporate) and freeze-dried. The dried starch was modified chemically with 3% w/v octenyl succinic anhydride (OSA) to increase its hydrophobicity, as previously described.24 The process was followed by centrifuging the suspension at 3000×g for 10 min. After removal of the supernatant, the modified starch was freeze-dried for further experiments.
2.3. Preparation of chitin nanofibers
The preparation of the chitin nanofibers was adapted from previous work (Lee et al., Food Chem., submitted). Briefly, 20 g of chitin powder was incubated in 200 mL of 1 M NaOH and 1 M HCl to remove the residual protein and calcium carbonate. The pellets were washed with water until a constant pH (∼4.2) is reached. Purified chitin was stored at −20 °C overnight and freeze-dried for subsequent storage. Three g of purified chitin were wetted with water and mixed with 150 mL of phosphoric acid in a shaking bath for 12 h to form a clear solution. Afterward, 750 mL of water was added to dilute the chitin suspensions to obtain a milky dispersion, followed by centrifugation at 17
000×g (Beckman Coulter J-20 XPI, Indianapolis IN, USA) for 15 min. After the supernatant was removed, the pellets were dialyzed with water until a constant pH value (∼4.2). The concentration of chitin in the final dispersion was gravimetrically determined to be 1.0% w/v.
2.4. Curcumin–oil blend preparation
Curcumin was added to soybean oil at a level of 1000 μg mL−1, mixed at 75 °C and stirred for 30 min. A preliminary study to determine the effects of heat and sonication treatment on curcumin solubility in oil was performed. Curcumin–oil blends were prepared in two batches: with and without 2 min sonication. The sonication treatment was performed with a probe with a 1.27 cm diameter at 35% pressure amplitude for 2 min (Sonics and Materials VC-750, Newtown, CT, USA). In order to control the temperature during the sonication process, a double-walled cylindrical chamber with a circulating coolant fluid through a jacket was used. Each batch was divided into portions and mixed at 25 °C, 50 °C, and 75 °C for 120 min. The concentration of curcumin in oil was measured every 30 min as shown in Table 1.
Table 1 Changes in the concentration of curcumin dissolved in oil with or without sonication when subjected to different temperatures. 1000 μg of curcumin was initially added to 1 mL of soybean oil
Temperature (°C) |
Sonication treatment |
Treatment time (min) |
Concentration (μg mL−1 oil) |
25 |
No |
0 |
130.62 ± 1.75 |
30 |
170.12 ± 12.22 |
60 |
202.22 ± 8.73 |
90 |
219.51 ± 5.24 |
120 |
163.95 ± 5.98 |
Yes |
0 |
140.49 ± 1.75 |
30 |
171.36 ± 0.01 |
60 |
224.44 ± 8.73 |
90 |
217.04 ± 15.71 |
120 |
167.65 ± 1.75 |
50 |
No |
0 |
154.07 ± 17.46 |
30 |
230.62 ± 45.39 |
60 |
246.67 ± 33.17 |
90 |
324.44 ± 6.98 |
120 |
240.49 ± 0.01 |
Yes |
0 |
188.64 ± 41.90 |
30 |
218.27 ± 52.38 |
60 |
273.83 ± 8.73 |
90 |
304.69 ± 0.01 |
120 |
267.65 ± 0.01 |
75 |
No |
0 |
242.96 ± 3.49 |
30 |
335.56 ± 12.22 |
60 |
257.78 ± 8.73 |
90 |
321.98 ± 13.97 |
120 |
270.12 ± 0.01 |
Yes |
0 |
215.80 ± 3.49 |
30 |
367.65 ± 40.15 |
60 |
275.06 ± 0.01 |
90 |
336.79 ± 0.01 |
120 |
302.22 ± 0.01 |
At each time, an aliquot of the curcumin–oil blend was separated and centrifuged at 13
200×g for 10 min to separate the undissolved curcumin. The undissolved curcumin was precipitated, and the supernatant was collected for measurement. A volume of 0.50 mL of the curcumin–oil blend was added to 4.50 mL of methanol to mimic the oil fraction used in emulsion preparation. With the proper dilution, 200 μL of the blend was mixed with 1.2 mL of methanol, and the absorbance was measured at 425 nm using a UV-Vis spectrophotometer (Thermo Fisher Scientific GEN10S Vis, Madison WI, USA).25 The following calibration curve was developed: absorbance = 0.0405 × curcumin [microgram mL−1] − 0.0384 (R2 = 0.96). The initial concentration of curcumin in soybean oil, when heated at 75 °C with sonication, was found to be the highest, 368 μg mL−1 (Table 1). Despite the sonication treatment being an effective method to improve the dissolution of curcumin in oil, a rancid aroma was perceived in all sonicated curcumin–oil blends, which is undesirable. As a result, heating at 75 °C for 30 min without sonication was selected as the standard method to dissolve curcumin in oil (335 μg mL−1, Table 1).
2.5. Preparation of oil-in-water emulsion
Chitin solution was diluted to concentrations of: 0.2% w/v and 0.4% w/v. Modified starch (1–2% w/v) was added to the chitin solution, followed by the addition of curcumin–oil blend to form a 10% oil-in-water Pickering emulsion. The mixture was emulsified using a high-shear mixer at 22
000 rpm for 2 min. The emulsions were prepared with different concentrations of ChFs and SNPs, as described in Table 2. The emulsions were termed according to the concentrations of nanoparticles used for their stabilization. For instance, sample C2S1 contains 0.2% w/v ChFs and 1% w/v SNPs. and A curcumin–oil blend was evaluated as control to determine the degradation rate of curcumin when it is not under protection. The formulations of the CSNP/ChF-stabilized Pickering emulsions in this work were selected according to the results reported in our previous work. The smallest droplet sizes and highest EI values upon storage were observed in emulsions stabilized with 0.2% ChFs and 1.0% of SNPs (C2S1samples) (Lee et al., Food Chem., submitted). Higher concentrations of solid nanoparticles were chosen in this study to investigate if encapsulation ability of the emulsion system could be further improved.
Table 2 Formations of Pickering emulsions
Sample |
ChFs concentration (%) |
SNPs concentration (%) |
Curcumin concentration (mg mL−1 oil) |
Bulk oil |
— |
— |
335 |
Control |
0.2 |
1.0 |
0 |
C2S1 |
0.2 |
1.0 |
335 |
C2S2 |
0.2 |
2.0 |
335 |
C4S1 |
0.4 |
1.0 |
335 |
C4S2 |
0.4 |
2.0 |
335 |
2.6. Characterization of curcumin concentration in SNP/ChF-stabilized Pickering emulsions
2.6.1. Measurement of retained curcumin in the systems. To be able to measure retained curcumin, methanol was used to break the emulsions. 200 μL of the emulsion sample were added to 1 mL of methanol and centrifuged at 13
200×g for 10 min in order to remove the solid nanoparticles.14 The absorbance of the remained curcumin was measured as described in Section 2.4. The residual curcumin level was obtained as: |
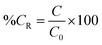 | (1) |
where, C = quantity of curcumin (mg) at any designated time point during UV radiation, C0 = initial quantity of curcumin (mg).At least three replicates were analyzed and the results are shown as the average ± standard deviation.
2.6.2. Microscopic observations of Pickering emulsions. Emulsions were observed under an optical microscope (BX53, Olympus Corporation, Canter Valley, PA, USA) connected to a digital color video camera (QImaging Micropublisher, Surrey, BC, Canada) and CellSens Dimension software (Olympus Corporation, Center Valley, PA, USA). A drop of the sample was carefully placed over a glass slide and covered with a glass coverslip. All observations were obtained using a 40× objective lens at room temperature. Additionally, auto-exposure of the video camera was used to obtain the images. During each microscopic observation, at least five images from each replicate were taken and analyzed. All the observations were carried out at room temperature.
2.7. Release of curcumin from the Pickering emulsions in excess water
Release of curcumin was measured based on the decrease in curcumin concentration in the emulsions as a function of time during dialysis in excess water. 10 mL of the Pickering emulsions encapsulating curcumin were placed in a presoaked dialysis cassette (molecular weight cut off 3500 Da). This dialysis cassette was placed in a 1 L of distilled water at room temperature. Water in the dialysis system was replaced with freshwater after every measurement to maintain a relatively constant concentration gradient across the dialysis membrane. After definite time intervals (0, 1, 2, 3, 6, 9, 12, and 24 h), 200 μL of the emulsion samples were collected, and curcumin concentration encapsulated in the emulsion sample was measured as described in Section 2.6.1.
2.8. Storage stability of curcumin in the Pickering emulsion
Curcumin stability in SNP/ChF-stabilized Pickering emulsions was measured as a function of storage time. Emulsions were stored in the dark at 20 °C for 16 d. After determined time intervals (0, 1, 2, 3, 4, 6, 8, 10, 12, 14, and 16 d), curcumin concentration in the emulsions was measured as described earlier in Section 2.6.1.
2.9. Stability of encapsulated curcumin in emulsions against ultraviolet (UV) light exposure
The curcumin–oil blend and SNP/ChF-stabilized Pickering emulsions were exposed to UV radiation (6 W, 365 nm) via a UV lamp in a lightproof cabinet. The remaining curcumin concentration in the emulsions was measured at t = 0, 1, 2, 3, 6, 9, 12, and 24 h of irradiation. At the designated time points, 200 μL of the sample were collected for measurement according to the procedure explained in Section 2.6.1.
2.10. Degradation kinetics of curcumin
Zero-, first-, and second-order models were used to characterize the degradation kinetics of curcumin under different conditions. R2 values were obtained to determine the quality and the best fit.
The zero-, first- and second-order kinetics models are defined respectively as:
|
ln(m0 − mt) = ln(m0) − k1t
| (3) |
|
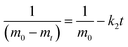 | (4) |
where,
mt = quantity of the curcumin released (mg) over time
t,
mb = quantity of the curcumin solution before release (mg; it is usually 0),
k0 = zero-order release rate constant (mg d
−1 & mg h
−1);
k1 = first-order rate constant (d
−1 & h
−1);
k2 = second-order rate constant [(mg d)
−1 & (mg h)
−1]; and
t = time (d & h).
Additionally, the half time (t1/2), which is the time necessary for curcumin quantity to decrease to 50% of its initial concentration, was calculated as:
|
t1/2 = −ln(0.5) × k−1
| (5) |
The decimal reduction time (
D value), which is the time necessary for the curcumin concentration to decrease by 90%, was calculated as:
|
D = ln 10 × k−1
| (6) |
where,
k = reaction rate constant.
2.11. Lipid oxidation measurements of Pickering emulsions
The prepared emulsions were placed into glass vials, sealed hermetically, covered with aluminum foil and placed in an oven at 45 °C for 60 d. Aliquots were withdrawn periodically to measure oxidation products. As our previous work showed that emulsions stabilized by 0.2% ChFs and 1% SNPs were stable (Lee et al., Food Chem., submitted), this emulsion formulation was used as control. The effect of curcumin on the lipid oxidation was determined by comparing the control and the C2S1 sample. Higher concentrations of solid nanoparticles were used to investigate if the lipid oxidation can be further reduced.
2.11.1. Peroxide value (PV) determination. Primary oxidation product was determined by the peroxide value (PV) method, according to Shantha & Decker with modifications.26 Briefly, 200 μL of Pickering emulsions were mixed with 1 mL of 2-propanol/isooctane (1
:
3, v/v) solvent mixture, vortexed for 30 s, and centrifuged at 10
000×g for 5 min. After that, 200 μL of the organic phase (transparent upper solvent layer) were added to 2.8 mL of methanol/1-butanol mixture (2
:
1, v/v) and 30 μL of thiocyanate/ferrous iron solution. The thiocyanate/ferrous iron solution was prepared by mixing 15 μL of 3.94 M NH4SCN (ammonium thiocyanate solution) and 15 μL of Fe2+ (ferrous iron solution). The ferrous iron solution was obtained from the supernatant of a mixture of 25 mL BaCl2 solution (0.13 M of BaCl2 in 0.4 M HCl) and 25 mL of 0.144 M FeSO4 solution. After each addition, the reaction tubes were vortexed for a few seconds and kept in dark conditions for 20 min. Afterward, the absorbance of the obtained solutions was measured at 510 nm using a UV-visible spectrophotometer (Thermo Scientific Genesys 10S Vis, Madison WI, USA). The measurements were performed in triplicate for all samples, and the average ± STD are reported.
2.11.2. p-Anisidine value (AV) determination. Secondary products of lipid oxidation were measured using the p-anisidine value (AV) method (AOCS) CD 18-90 (1998). This method is based on the reaction of p-anisidine with unsaturated aldehydes, resulting in the formation of a yellowish product that absorbs at wavelength 350 nm. The AV values were calculated using the method described in AOCS CD 18-90 (1998): |
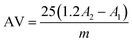 | (7) |
where, m = mass of the sample (g).
2.12. Statistical analysis
Statistical analysis was performed using a one-way analysis of variance (ANOVA) procedure with JMP Pro version 13.0 statistical analysis software (SAS Institute, Cary, NC, USA), and the differences between the means of the trials were determined by using Tukey's honest significant difference (HSD) test (P < 0.05). All samples were prepared in three replicates and analyzed, and the results are presented as the mean ± standard deviation.
3. Results
3.1. Release profiles of encapsulated curcumin from the Pickering emulsions in excess water
The release profiles over 24 h incubation in water of curcumin encapsulated in SNP/ChF-stabilized Pickering emulsions are shown in Fig. 1.
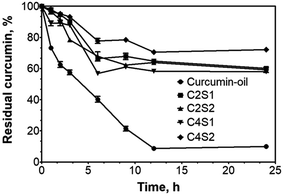 |
| Fig. 1 Release profiles of curcumin from curcumin–oil blend and SNP/ChF-stabilized Pickering emulsions over 24 hours of storage in excess water. Data points represent the average ± standard deviation (n = 3). | |
An initial steady and significant release of curcumin as a function of incubation time was observed in the curcumin–oil blend. When curcumin is not encapsulated in SNP/ChF-stabilized Pickering emulsions (curcumin–oil blend), approximately 40% of curcumin was lost in the first 2 h, and 90% of curcumin was released after 24 h incubation time. When the emulsion system was used to encapsulate curcumin (C2S1), its retention was significantly improved, only 35% of curcumin was released after 9 h. Interestingly, our results showed a steady decrease in the percent of residual curcumin during the first 9 h, followed by a stabilization and plateau at longer times. Different studies have previously reported that about 25–50% of curcumin was lost in excess water within 8 h.27–30 These results supported our findings and suggested that C2S1 has similar encapsulation and retention efficiency as those reported for other systems.
Similar results to those for C2S1 were observed in C2S2 and C4S1, which implies that an increase in either solid nanoparticles (SNPs or ChFs) does not significantly improve the curcumin retention in excess water. Nevertheless, it is noteworthy that doubling the concentrations of both solid nanoparticles (C4S2) significantly improved the curcumin retention when compared to C2S1. In C4S2, 80% of curcumin remained encapsulated after 9 h, while 75% of curcumin was successfully retained after 24 h. Furthermore, this system seems to have superior performance than previously reported similar emulsion systems.27–30
3.2. Storage stability of curcumin in the Pickering emulsions
The preservation of curcumin in a curcumin–oil blend and SNP/ChF-stabilized Pickering emulsions as a function of time at 20 °C under dark conditions is depicted in Fig. 2A. In the curcumin–oil blend (not encapsulation), approximately half of the curcumin was degraded after 6 d of storage and only 20% of curcumin remained after 16 d. A significant improvement in curcumin stability was observed when SNP/ChF-stabilized Pickering emulsions were used to protect curcumin. In C2S1, the percent residual curcumin that remained in the system after 16 d storage was approximately 50%, which is close to 2.5 times higher than the amount of curcumin remaining in the curcumin–oil blend. These results are supported by previous works where emulsions were stabilized by a combination of two different particles including chitosan-gum arabic nanoparticles, carboxymethyl cellulose-cationic chitosan nanoparticles or milled starch nanoparticles. The aforementioned studies reported approximately 10% curcumin degradation after 24 hours storage.31–33 Nevertheless, the mentioned emulsions systems showed a shorter curcumin half-life (50% curcumin degradation) when compared to C2S1 which suggested emulsions stabilized by ChFs/SNPs show advantage in curcumin encapsulation. Notably, the % residual curcumin is also much higher in these SNP/ChF-stabilized Pickering emulsion compared to other encapsulation systems previously studied. For instance, about 80% of curcumin was retained after 24 h when curcumin was protected by starch granules.34 Moreover, studies have shown that approximately 40% of curcumin was usually retained after 24 h of storage by many encapsulation systems, such as by sodium dodecyl sulfate micelles and liposomes.35,36 Furthermore, chitosan-tripolyphosphate-nanoparticles-stabilized Pickering emulsions were reported to have a half-life of 5 d.37 Comparatively, SNP/ChFs-stabilized Pickering emulsions showed to have a curcumin extended half-life (16 d) and thus, they are more effective in encapsulating curcumin.
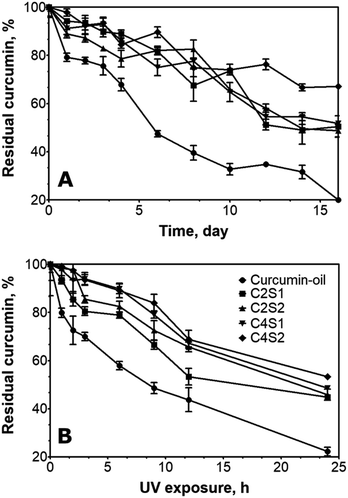 |
| Fig. 2 Residual curcumin level of curcumin–oil blend and Pickering emulsions stabilized by different concentrations of solid nanoparticles (A) during 16 days of storage at 20 °C under dark conditions; (B) under UV exposure for 24 h. Data points represent the average ± standard deviation (n = 3). | |
The results indicate that no significant difference (P < 0.05) on the percentage of residual curcumin was observed when either solid nanoparticles was increased from C2S1 (C2S2 and C4S1). This observation indicates that doubling the amount of one type of solid nanoparticle was not enough to have a significant improvement on curcumin retention. By doubling the concentrations of both solid nanoparticles (C4S2), the retention of curcumin was significantly improved to approximately 70% after 24 h, which is about 3.5 and 1.3 times higher than the curcumin–oil blend and C2S1, respectively. This phenomenon could be attributed to the high concentrations of solid nanoparticles, which formed a denser layer at the water–oil interface and thus prevented the interaction between pro-oxidants and curcumin.38
3.3. Stability of encapsulated curcumin upon ultraviolet (UV) light exposure
Pickering emulsions have been proven to be a promising approach to deliver sensitive compounds in the nutraceuticals field.39 Optimized SNP/ChF-stabilized Pickering emulsions were used to evaluate their ability to protect curcumin when subjected to UV irradiation. The residual curcumin concentration as a function of irradiation time is shown in Fig. 2B.
When curcumin–oil blend was exposed to UV radiation, the degradation was maximal as UV induced about 50% degradation of curcumin upon 9 h of UV exposure and only 20% of curcumin remained after 24 h. Under these conditions, the energy transmitted to curcumin was maximum, and thus, the degradation rate was the highest. The energy gained from the UV light broke down the carbonyl groups of curcumin and, quickly, oxidized the intermediates (ferulic aldehydes) to ferulic acid, vanillin, or vanillin acid.40,41 In the case of encapsulated curcumin, curcumin stability against UV radiation was significantly improved. For example, in the C2S1 sample, approximately 70% of curcumin was retained after 9 h of radiation, which is 2.5 times more than in the bulk oil, while approximately 45% of curcumin remained after 24 h. This is because in these emulsions, the solid nanoparticles loaded at the interface are able to shelter the curcumin-containing oil droplets from direct contact with UV light; as a result, the curcumin degradation is significantly reduced.42
Previous works studied the UV stability of curcumin encapsulated on Pickering emulsion systems under similar or less severe irradiation conditions and found that Pickering emulsions stabilized by kafirin nanoparticles and Tween-80 retained about 60% and 50% of curcumin after 270 min of UV exposure.43 Another study reported that in ovotransferrin fibril-stabilized Pickering emulsions, about 40% of curcumin was lost after 12 h of UV irradiation.44 Hence, SNP/ChF-stabilized Pickering emulsions seem to be a more efficient system to protect curcumin from UV degradation.
To determine if the stability of encapsulated curcumin can further be improved, SNPs and/or ChFs amounts were doubled and the Pickering emulsions were analyzed (Fig. 2B). The results agreed with our previous discussions that duplicating the concentration of either SNPs (C2S2) or ChFs (C4S1) did not lead to a significant improvement from C2S1 in curcumin stability (p < 0.05) upon UV exposure. On the other hand, after 24 hours of UV light exposure, C4S2 retained the highest amount of curcumin (approximately 50%). This indicates that both solid nanoparticles needed to be doubled in order to significantly increase the curcumin retention. This observation suggests that, as discussed in previously, a thicker barrier at the oil–water interface reduces the amount of curcumin that can be degraded by UV light.
3.4. Curcumin degradation kinetics
The analysis of the degradation kinetics of curcumin in SNP/ChF-stabilized Pickering emulsions using the zero, first and second kinetic mathematical models are shown in Tables 3 and 4. Analysis of the data showed that the curcumin retention results during storage and UV exposure was fitted best by the first-order kinetic model with the highest regression coefficients (R > 0.923).
Table 3 Kinetic parameters of curcumin degradation over 16 days of storage time in bulk soybean oil and in SNP/ChF-stabilized Pickering emulsions when analyzed with different kinetic models: reaction rate coefficient (k), correlation coefficient (R2), half-life (T0.5) and D-value of degradation
Kinetic model |
Kinetic parameters |
Oil |
C2S1 |
C2S2 |
C4S1 |
C4S2 |
Zero order |
k0 × 10−1 (mg d−1) |
1.25 ± 0.04 |
0.54 ± 0.06 |
0.44 ± 0.01 |
0.44 ± 0.02 |
0.35 ± 0.01 |
R2 |
0.8964 |
0.9624 |
0.9484 |
0.9788 |
0.9324 |
t0.5 (d) |
5.55 ± 0.18 |
12.92 ± 1.54 |
15.61 ± 0.42 |
15.70 ± 0.85 |
19.75 ± 0.30 |
D value (d) |
18.44 ± 0.58 |
42.91 ± 5.11 |
51.85 ± 1.41 |
52.16 ± 2.84 |
65.61 ± 1.00 |
First order |
k1 × 10−2 (d−1) |
5.09 ± 0.04 |
2.50 ± 0.35 |
2.27 ± 0.06 |
2.20 ± 0.11 |
1.51 ± 0.02 |
R2 |
0.9427 |
0.9799 |
0.9536 |
0.9786 |
0.9304 |
t0.5 (d) |
13.62 ± 0.11 |
28.05 ± 3.90 |
30.50 ± 0.77 |
31.61 ± 1.64 |
45.81 ± 0.63 |
D value (d) |
45.24 ± 0.38 |
93.19 ± 12.94 |
101.33 ± 2.56 |
105.02 ± 5.46 |
152.17 ± 2.11 |
Second order |
k2 × 10−2 (mg d−1) |
2.17 ± 0.03 |
1.17 ± 0.19 |
1.18 ± 0.07 |
1.10 ± 0.06 |
0.66 ± 0.01 |
R2 |
0.9371 |
0.9657 |
0.9212 |
0.9755 |
0.9135 |
t0.5 (d) |
31.94 ± 0.42 |
60.31 ± 9.88 |
59.04 ± 3.51 |
62.95 ± 3.43 |
105.58 ± 1.84 |
D value (d) |
106.12 ± 1.38 |
200.34 ± 32.83 |
196.14 ± 11.66 |
209.11 ± 11.40 |
350.72 ± 6.11 |
Best fit |
First order |
Table 4 Kinetic parameters of curcumin degradation occurred under UV light exposure when analyzed with different kinetic models: reaction rate coefficient (k), correlation coefficient (R2), half-life (T0.5) and D-value of degradation
Kinetic model |
Kinetic parameters |
Oil |
C2S1 |
C2S2 |
C4S1 |
C4S2 |
Zero order |
k0 × 10−1 (mg h−1) |
3.77 ± 0.06 |
0.99 ± 0.20 |
0.39 ± 0.01 |
0.42 ± 0.08 |
0.35 ± 0.02 |
R2 |
0.8591 |
0.9340 |
0.9416 |
0.9737 |
0.9137 |
t0.5 (h) |
1.84 ± 0.03 |
7.33 ± 2.11 |
17.71 ± 0.10 |
16.87 ± 3.22 |
19.82 ± 1.05 |
D value (h) |
6.12 ± 0.09 |
24.34 ± 7.00 |
58.82 ± 0.32 |
56.04 ± 10.70 |
65.85 ± 3.49 |
First order |
k1 × 10−2 (h−1) |
5.28 ± 0.23 |
2.54 ± 0.19 |
2.09 ± 0.06 |
1.99 ± 0.25 |
1.45 ± 0.01 |
R2 |
0.9675 |
0.9433 |
0.9698 |
0.9841 |
0.9273 |
t0.5 (h) |
13.15 ± 0.58 |
27.42 ± 2.07 |
33.26 ± 1.02 |
35.19 ± 4.39 |
47.80 ± 0.33 |
D value (h) |
43.69 ± 1.93 |
91.09 ± 6.86 |
110.49 ± 3.37 |
116.91 ± 14.58 |
158.80 ± 1.10 |
Second order |
k2 × 10−3 (mg h−1) |
8.20 ± 0.85 |
6.05 ± 0.21 |
9.87 ± 2.34 |
9.55 ± 0.50 |
6.03 ± 0.3 |
R2 |
0.9631 |
0.9007 |
0.9467 |
0.9681 |
0.9070 |
t0.5 (h) |
84.99 ± 8.79 |
114.64 ± 4.02 |
61.97 ± 3.13 |
72.68 ± 3.77 |
115.02 ± 4.76 |
D value (h) |
282.31 ± 29.21 |
380.83 ± 13.35 |
205.85 ± 10.40 |
241.43 ± 12.51 |
382.08 ± 15.82 |
Best fit |
First order |
The degradation kinetics parameters of curcumin in curcumin–oil blend and SNP/ChF-stabilized Pickering emulsions over 16 d of storage under dark conditions (Table 3) indicate that the degradation rate of curcumin in C2S1 is half the value of the curcumin–oil blend. In addition, C2S1 showed that the half-life could be extended from 13 d to 28 d, while the time required for 90% of the curcumin to be degraded was increased to 3 months.; i.e., 48 d longer than for the curcumin–oil blend. When either of the Pickering stabilizers was doubled (samples C2S2 and C4S1), no significant differences were found in the kinetic values, half-life, and D-value. However, by comparing both samples (C2S2 and C4S1) to C2S1, a 3 day extension in half-life was observed. Additionally, the time required for 90% of curcumin in C2S2 and C4S1 to be degraded increased to approximately 103 d, about 10 d longer than for C2S1. Even though ∼31 d of half-life and ∼101 d of D-value is an improvement on curcumin stability relative to the curcumin–oil blend, it might not be enough for the food industry. Hence, by simultaneously doubling the concentrations of both solid nanoparticles (C4S2), the degradation rate was found to be the lowest, while the half-life and D-value were remarkably extended by 50% (P < 0.05). This implies that doubling the concentrations of both stabilizers can positively affect the stability of the encapsulated curcumin.
Table 4 presents the kinetic parameters of curcumin stability when exposed to UV radiation. As expected from the previous results, the curcumin degradation rate was the highest when it was not protected from UV degradation. When curcumin was encapsulated in Pickering emulsions (C2S1), a 40% of reduction in degradation rate was noticed when compared to the curcumin–oil blend. As a result, a 14 h extension in the half-life was shown in C2S1 emulsions compared to the curcumin–oil blend (∼13 h); i.e., in C2S1 emulsions, ∼27 h of UV exposure time is required to degrade 50% of curcumin. Furthermore, the time required to degrade 90% of the curcumin was extended by 33 h in the comparison with the curcumin–oil blend. Although the degradation rates in C2S2 and C4S1 decreased ∼1.25 times, while the half-life and D-value were increased ∼1.25 times in comparison to C2S1, no significant differences were found (P < 0.05). This observation indicates that doubling the concentrations of either solid nanoparticle did not have a significant impact on the curcumin protection. As previously discussed (Fig. 2B), the protective effect of the emulsion system on curcumin was found to be the highest with the synchronized two-fold increase from the C2S1 of both solid nanoparticles. The degradation rate was found to be the lowest (P < 0.05) and the half-life and D-value were further extended ∼3.5 times as compared to the curcumin–oil blend. As previously stated, this phenomenon can be explained by the superior droplet coverage that reduces the interaction between UV light and curcumin.
3.5. Microstructure of SNP/ChF-stabilized Pickering emulsions
The optical microscopic analysis showed the presence of coalescence in most of SNP/ChF-stabilized Pickering emulsions over 16 d (storage stability), as shown in Fig. 3. The inserts show the visual appearance of the emulsions. The overall droplet sizes and degree of coalescence of emulsions slightly increased over 16 d of storage. Nevertheless, the C4S2 was the exception as it showed excellent storage stability, as evidenced by minimal degree of coalescence, and unaffected droplet size after 16 d of storage. These findings are consistent with our previous work where Pickering emulsions stabilized by 0.2% w/v of ChFs and 1% w/v of SNPs (C2S1) was stable up to 28 d of storage (P < 0.05) (Lee et al., Food Chem., submitted). In addition, observation of the microscopic appearance and the yellow intensity of the prepared emulsions suggested that SNP/ChF-stabilized Pickering emulsions could be a candidate to provide excellent protection on the curcumin, as each sample remained similar after 16 d.
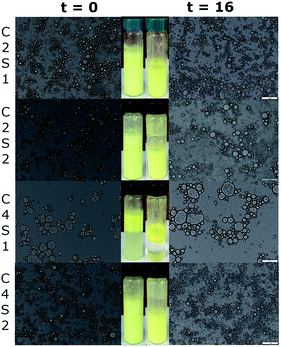 |
| Fig. 3 Representative optical micrographs of SNP/ChF-stabilized Pickering emulsions over 16 days of storage at room temperature. Insets are the glass vials that show the appearance looks of emulsions. All samples were kept under dark conditions without exposure to light. The scale bar is 50 μm. | |
3.6. Oxidative stability
Lipid oxidation is the leading cause of deterioration of food quality, leading to rancidity and shortening of shelf life. Oxidation affects all significant components in foods; however, carbohydrate is less sensitive as compared to lipid and protein.45 In order to investigate the impacts of concentrations of solid nanoparticles and curcumin on the oxidative stability of the oil, the progress of lipid oxidation was monitored for 60 d.
According to Fig. 4, lipid oxidation was significantly affected by the solid nanoparticle content used to stabilize the emulsions. Fig. 4A and B shows PV and AV values of the emulsions, respectively. PV and AV for emulsions that contained curcumin were lower than for the control (emulsion without curcumin). This indicated that curcumin is able to reduce the formation of hydroperoxides, which participate as reactants for the production of aldehydes.46 In addition, chitin can act as antioxidant to reduce the progress of lipid oxidation.47 It is possible that with the increased amounts of solid nanoparticles, ChFs that were not absorbed to the interphase may locate in the aqueous phase and play a role delaying oxidation. As shown in Fig. 4A, the PV values for all samples were below 10 mEq/kg oil, which is the limit commonly used to indicate oxidation of oil.48,49 The results suggest that all samples were not considerably oxidized.
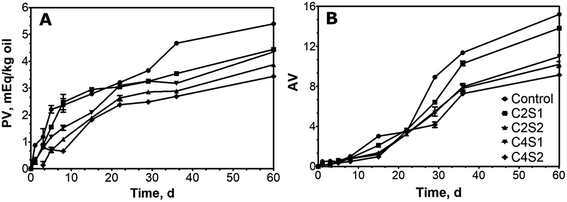 |
| Fig. 4 Progress of hydroperoxide concentration (A) and p-anisidine value (B) in different SNP/ChF-stabilized Pickering emulsions. The graph on the left shows hydroperoxide concentration (primary products), while the graph on the right shows p-anisidine value (secondary products). Data points represent the average ± standard deviation (n = 3). The error bars that are not visible are smaller than symbols. | |
Furthermore, AV of 10 is the standard used in the industry to determine good quality of an edible oil.50 In this experiment, the control and C2S1 samples exceeded AV values of 10 on day 36, indicating that both emulsions were oxidized (Fig. 4B). Despite having high AV values, the C2S1 sample showed lower AV values than the control even after 60 d of storage under 45 °C storage (P < 0.05). The difference in the AV values between the C2S1 and the control shows the antioxidant effect of curcumin. In addition, our results suggest that the combined use of multiple solid nanoparticles exerted a positive impact against oxidation. In a previous study, Kargar et al. reported in sodium-caseinate-stabilized Pickering emulsions, hydroperoxide concentrations and AV values of approximately 8 mM and 2.5, respectively, after 7 d of storage at 45 °C.38 Similarly, they also found that Tween-20-stabilized emulsions had hydroperoxide concentration and AV values of approximately 23 mM and 6, respectively.38
In this study, when either solid nanoparticle was increased (samples C2S2 and C4S1), the oxidative stability was significantly improved as they exceeded AV of 10 on day 60 (P < 0.05). By doubling the concentrations of both solid nanoparticles (C4S2), the shelf life was extended to longer than 60 d. It can be hypothesized that the higher concentrations of solid nanoparticles provided better area coverage and reduced the interactions between oil and oxygen.38 With higher concentrations of solid nanoparticles at the water–oil interface, the formation of dense barriers prevents the pro-oxidants in the aqueous phase from interacting directly with susceptible unsaturated fatty acids prone to oxidize.
As shown in Fig. 5, emulsion droplets became significantly larger with coalescence being observed in some samples after 60 d incubation. By comparing the control and sample C2S1, droplet size and degree of coalescence were observed to be similar. This is because they are stabilized by equal concentrations of solid nanoparticles (0.2% w/v ChFs and 1% w/v SNPs). It is apparent that yellow intensity, which indicates the presence of curcumin, decreased over time. The reduction in color intensity is probably due to interactions occurring between curcumin and pro-oxidants from the aqueous phase leading to the oxidation of curcumin. When observing the glass vials upon 60-d incubation, the yellow color was more intense at higher stabilizer concentrations, which is due to the formation of thicker droplet surfaces. When either solid nanoparticle was doubled (C2S2 and C4S1), the difference in droplet sizes was large, even on d 0. Freshly prepared C4S1 was found to have larger droplets than all other samples. Hence, we hypothesize that long-fibril ChFs dominated the surface of oil droplets, and since they cannot completely cover the droplets' surface, this resulted in low external coverage. The same observation was also reported in our previous work (Lee et al., Food Chem., submitted). Furthermore, C4S2 was able to maintain limited coalescence and a slight increase in droplet size after storage. This observation indicates that emulsion stability can be improved by doubling the concentrations of both stabilizers.
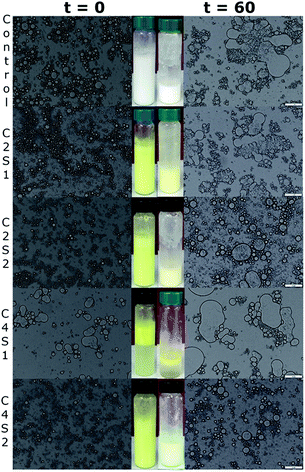 |
| Fig. 5 Representative optical micrographs of SNP/ChF-stabilized Pickering emulsions that were stored under oxidative conditions for 60 days. Insets are the glass vials that show the appearance looks of emulsions. All samples were kept under dark conditions without exposure to light. The scale bar is 50 μm. | |
4. Conclusion
In this work, curcumin has been successfully encapsulated in SNP/ChF-stabilized Pickering emulsions and its stability under different conditions was evaluated. The sonication treatment was an efficient method to improve solubility of curcumin in oil. However, it can lead to oxidation and thus to lower the consumer acceptance. Hence, the use of heating treatment was more suitable to dissolve the curcumin in oil while maintaining emulsion quality. The results on the passive release of curcumin encapsulated in SNP/ChF-stabilized Pickering emulsions suggest that these emulsions are a promising system to effectively encapsulate and retain curcumin. By its encapsulation in SNP/ChF-stabilized emulsions, curcumin stability was significantly improved after 16 d of storage and 24 h of UV exposure. Additionally, an increase in either one of the solid nanoparticles (C2S2 and C4S1) did not significantly improve the protective effect of Pickering emulsions on curcumin, but rather a significant improvement in curcumin retention was found when the concentrations of both solid nanoparticles were doubled (C4S2). Oxidative stability could be improved in SNP/ChF-stabilized Pickering emulsions as they were chemically stable for 36 d (exceeded 10 AV on day 36); with a further increase to at least 60 d when the concentration of stabilizers was increased. The use of multiple clean-label stabilizers could be an ideal carrier to entrap, protect and deliver bioactive ingredients.
Conflicts of interest
The authors declare no conflicts of interest.
Acknowledgements
This paper is a product of the Iowa Agriculture and Home Economics Experiment Station, Ames, Iowa Project No. IOW03902. This work was sponsored by Hatch Act and State of Iowa funds.
References
- I. Chattopadhyay, K. Biswas, U. Bandyopadhyay and R. K. Banerjee, Curr. Sci., 2004, 87, 44 CAS.
- R. K. Maheshwari, A. K. Singh, J. Gaddipati and R. C. Srimal, Life Sci., 2006, 78, 2081 CrossRef CAS PubMed.
- B. B. Aggarwal, T. -J. Surh, and, S. Shishodia. The Molecular Targets and Therapeutic Uses of Curcumin in Health and Disease, 2007. DOI:10.1007/978-0-387-46401-5.
- T. Hamaguchi, K. Ono and M. Yamada, CNS Neurosci. Ther., 2010, 16, 285 CrossRef CAS.
- A. Noorafshan and S. Ashkani-Esfahani, Curr. Pharm. Des., 2013, 19, 2032 CAS.
- P. Anand, A. B. Kunnumakkara, R. A. Newman and B. B. Aggarwal, Mol. Pharm., 2007, 4, 807 CrossRef CAS.
- A. S. Jiménez-Osorio, W. R. García-Niño, S. González-Reyes, A. Álvarez-Mejía, S. Guerra-León, J. Salazar-Segovia, I. Falcón, H. Montes de Oca-Solano, M. Madero and J. Pedraza-Chaverri, J. Ren. Nutr., 2016, 26, 237 CrossRef.
- A. Amalraj, K. Varma, J. Jacob, C. Divya, A. B. Kunnumakkara, S. J. Stohs and S. Gopi, J. Med. Food, 2017, 20, 1022 CrossRef CAS PubMed.
- S. S. Bansal, M. Goel, F. Aqil, M. V. Vadhanam and R. V. Gupta, Canc. Prev. Res., 2011, 4, 1158 CrossRef CAS PubMed.
- M. Kharat, Z. Du, G. Zhang and D. J. McClements, J. Agric. Food Chem., 2017, 65, 1525 CrossRef CAS PubMed.
- M. R. I. Shishir, L. Xie, C. Sun, X. Zheng and W. Chen, Trends Food Sci. Technol., 2018, 78, 34–60 CrossRef CAS.
- A. Rashidinejad, and, S. M. Jafari. Nanoencapsulation of bioactive food ingredients. in: Handbook of Food Nanotechnology. Elsevier; 2020, pp. 279–344 Search PubMed.
- Y. Chen, Y. Wang, X. Shi, M. Jin, W. Cheng, L. Ren and Y. Wang, Carbon, 2017, 111, 38 CrossRef CAS.
- R. V. Tikekar, Y. Pan and N. Nitin, Food Res. Int., 2013, 51, 370 CrossRef CAS.
- S. Simovic, H. Hui, Y. Song, A. K. Davey, T. Rades and C. A. Prestidge, J. Controlled Release, 2010, 143, 367 CrossRef CAS PubMed.
- W. W. Mwangi, K. W. Ho, B. T. Tey and E. S. Chan, Food Hydrocolloids, 2016, 60, 543 CrossRef CAS.
- M. R. Barkhordari and M. Fathi, Food Hydrocolloids, 2018, 82, 338 CrossRef CAS.
- Y. Zhang, Z. Chen, W. Bian, L. Feng, Z. Wu, P. Wang, X. Zeng and T. Wu, Food Chem., 2015, 183, 115 CrossRef CAS PubMed.
- Y. Zhu, S. Huan, L. Bai, A. Ketola, X. Shi and X. Zhang, et al., ACS Appl. Mater. Interfaces, 2020, 12, 11240–11251 CrossRef CAS PubMed.
- Y. Tan, K. Xu, C. Niu, C. Liu, Y. Li, P. Wang and B. P. Binks, Food Hydrocolloids, 2014, 36, 70–75 CrossRef CAS.
- C. Li, P. Sun and C. Yang, Starch/Staerke, 2012, 64(6), 497–502 CrossRef CAS.
- A. Timgren, M. Rayner, M. Sjöö and P. Dejmek, Procedia Food Sci., 2011, 1, 95–103 CrossRef CAS.
- A. Timgren, M. Rayner, P. Dejmek, D. Marku and M. Sjöö, Food Sci. Nutr., 2013, 1(2), 157–171 CrossRef CAS PubMed.
- P. Dejmek, M. Rayner, A. Timgren and M. Sj, J. Sci. Food Agric., 2012, 92, 1841 CrossRef PubMed.
- C. T. Ho, J. Liq. Chromatogr., 1988, 11, 2295 Search PubMed.
- N. C. Shantha and E. A. Decker, J. AOAC Int., 1994, 77, 421 CrossRef CAS PubMed.
- M. H. Lee, H. Y. Lin, H. C. Chen and J. L. Thomas, Langmuir, 2008, 24, 1707 CrossRef CAS PubMed.
- A. Mukerjee and J. K. Vishwanatha, Anticancer Res., 2009, 29, 3867 CAS.
- R. K. Das, N. Kasoju and U. Bora, Nanomed. Nanotechnol. Biol. Med., 2010, 6, 153 CrossRef CAS PubMed.
- A. P. Nayak, W. Tiyaboonchai, S. Patankar, B. Madhusudhan and E. B. Souto, Colloids Surf., B, 2010, 81, 263 CrossRef CAS PubMed.
- J. Han, F. Chen, C. Gao, Y. Zhang and X. Tang, Int. J. Biol. Macromol., 2020, 157, 202–211 CrossRef CAS PubMed.
- X. Lu, C. Li and Q. Huang, Int. J. Biol. Macromol., 2019, 139, 917–924 CrossRef CAS PubMed.
- X. Zhu, J. Chen, Y. Hu, N. Zhang, Y. Fu and X. Chen, Food Hydrocolloids, 2021, 110, 106135 CrossRef CAS.
- A. Marefati, M. Bertrand, M. Sjöö, P. Dejme and M. Rayner, Food Hydrocolloids, 2017, 63, 309 CrossRef CAS.
- M. H. M. Leung, H. Colangelo and T. W. Kee, Langmuir, 2008, 24, 5672 CrossRef CAS PubMed.
- C. Chen, T. D. Johnston, H. Jeon, R. Gedaly, P. P. McHugn, T. G. Burke and D. Ranjan, Int. J. Pharm., 2009, 366, 133 CrossRef CAS PubMed.
- B. R. Shah, Y. Li, W. Jin, Y. An, L. He and Z. Li, Food Hydrocolloids, 2016, 52, 369 CrossRef CAS.
- M. Kargar, F. Spyropoulos and I. T. Norton, J. Colloid Interface Sci., 2011, 357, 527 CrossRef CAS PubMed.
- Y. Shao and C. H. Tang, Food Res. Int., 2016, 79, 64 CrossRef CAS.
- W. Xue, C. Li and S. H. I. Wen, Study on the photo-stability of curcuminoid, J. Anhui Univ., 2012, 36(May), 8–13 Search PubMed.
- K. I. Priyadarsini, Molecules, 2014, 19(12), 20091–20112 CrossRef PubMed.
- W. Xue, C. Li and S. Wen, J. Anhui Univ., 2012, 36, 8 Search PubMed.
- J. Xiao, C. Li and Q. Huang, J. Agric. Food Chem., 2015, 63, 10263 CrossRef CAS.
- Z. Wei, J. Zhu, Y. Cheng and Q. Huang, Food Res. Int., 2019, 125, 108602 CrossRef CAS PubMed.
- R. D. Bernardini, P. Harnedy, D. Bolton, J. Kerry, E. O'Neill, A. M. Mullen and M. Hayes, Food Chem., 2011, 124, 1296 CrossRef.
- L. Mei, D. J. McClements, J. Wu and E. A. Decker, Food Chem., 1998, 61, 307 CrossRef CAS.
- D. H. Ngo, and, S. K. Kim. Antioxidant Effects of Chitin, Chitosan, and Their Derivatives, Elsevier Inc., 1st edn, 2014 Search PubMed.
- F. D. Gunstone, Oils and Fats in the Food Industry: Food Industry Briefing Series, John Wiley & Sons Ltd, West Sussex, UK, 2009 Search PubMed.
- A. Sebastian, S. M. Ghazani and A. G. Marangoni, Food Res. Int., 2014, 64, 420 CrossRef CAS PubMed.
- J. B. Rossell. Rancidity, in Foods, Aspen Publishers Inc., Gaithersburg, 1994, p. 514 Search PubMed.
|
This journal is © The Royal Society of Chemistry 2021 |
Click here to see how this site uses Cookies. View our privacy policy here.