DOI:
10.1039/D1RA00353D
(Review Article)
RSC Adv., 2021,
11, 21447-21462
Ocean plastics: environmental implications and potential routes for mitigation – a perspective
Received
15th January 2021
, Accepted 16th May 2021
First published on 17th June 2021
Abstract
This review provides a current summary of the major sources and distribution of ocean plastic contamination, their potential environmental effects, and prospects towards the mitigation of plastic pollution. A characterization between micro and macro plastics has been established, along with a comprehensive discussion of the most common plastic waste sources that end up in aquatic environments within these categories. Distribution of these sources stems mainly from improper waste management, road runoff, and wastewater pathways, along with potential routes of prevention. The environmental impact of ocean plastics is not yet fully understood, and as such, current research on the potential adverse health effects and impact on marine habitats has been discussed. With increasing environmental damage and economic losses estimated at $US 1.5 trillion, the challenge of ocean plastics needs to be at the forefront of political and societal discussions. Efforts to increase the feasibility of collected ocean plastics through value-added commercial products and development of an international supply chain has been explored. An integrative, global approach towards addressing the growing ocean plastic problem has been presented.
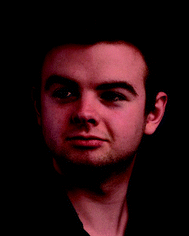 Ethan Watt | Ethan Watt is in his fourth year working to complete his bachelor's degree in Nanoscience at the University of Guelph. His studies have focused around physical chemistry and material science. He has served three co-op study terms at the Bioproducts Discovery and Development Center (BDDC) based at the University of Guelph under the guidance of Professor Amar Mohanty and Professor Manjusri Misra. His research interests include composite research involving the incorporation of biocarbon and nanofillers into polymeric matrices to generate green composites. |
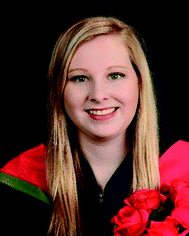 Maisyn Picard | Maisyn Picard has completed her bachelor's degree in Biomedical Engineering (with distinction) from the University of Guelph. Currently, she has completed her Master of Applied Science degree under the advice of Professor Amar Mohanty and Professor Manjusri Misra in Biological Engineering from the University of Guelph (Guelph, Ontario, Canada). Ms Picard's research interests include additive manufacturing, polymer processing and characterization, and sustainable materials development via a circular economic approach. |
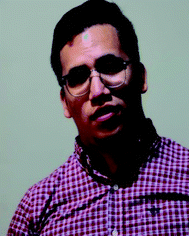 Benjamin Maldonado | Benjamin Maldonado completed his bachelor's degree in Plastics Engineering from the Universidad Autonoma del Estado de Mexico. Currently, he is completing his Master of Applied Science degree under the advice of Professor Amar Mohanty and Professor Manjusri Misra in Biological Engineering from the University of Guelph (Guelph, Ontario, Canada). Mr Benjamin interest includes Additive Manufacturing, injection molding design and product design. |
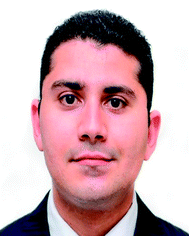 Mohamed A. Abdelwahab | Dr Mohamed A. Abdelwahab received his Ph.D. degree under the advice of Professor Emo Chiellini in Biomaterial from Pisa University, Italy in 2012 and M.Sc. degree under the advice of Professor Ahmed Akelah and Professor Tarek Agag in Polymer Chemistry from Tanta University, Tanta, Egypt. He joined the Tanta University Chemistry staff, Tanta, Egypt in 2018 as an Associate Professor. Currently, Dr Abdelwahab works as a Post-Doctoral researcher at the Bioproducts Discovery and Development Centre (BDDC), University of Guelph, Guelph, Ontario, Canada under the guidance of Professor Amar Mohanty and Professor Manjusri Misra. His research interests focus on polymer science, polymer processing, nanocomposites, biopolymers, renewable resources, and eco-compatible materials. He has published more than 37 peer-reviewed journal articles, two patents application (filed) and three book chapters. |
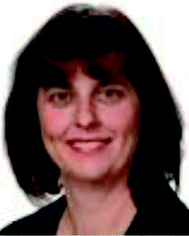 Deborah F. Mielewski | Dr Deborah Mielewski is the Technical Fellow, Sustainability at Ford Motor Company, Research and Innovation Center. Dr Mielewski has worked at Ford Research in automotive paint durability, polymer processing and materials development. She initiated the biomaterials program at Ford Research in 2001, and her team was the first to demonstrate soy-based foam that met all the requirements for automotive seating. Ford launched soy-based foam on the 2008 Mustang, and soy seat cushions, backs and headrests have found their way into every Ford North American built vehicle. Bio-based foams currently reduce greenhouse gas emissions by over 25 million pounds and reduce petroleum dependence by over 5 million pounds annually. Ford currently has over a dozen renewable materials in production vehicles, including natural fiber reinforced composites such as wheat straw, rice hulls and cellulose fiber from trees. These materials meet all the stringent requirements of automotive, many times exceeding the performance of traditional plastic composite materials. Late last year, Ford pioneered the introduction of the first bio-carbon made from McDonald's coffee chaff into the headlamp housings of the Lincoln Continental. Dr Mielewski is passionate about the work she does to reduce Ford's environmental footprint and believes that these new materials are going to dominate the market in the future. Her philosophy is to “do the right thing” in incremental, but ever advancing steps. She has appeared in a Ford national commercial, the NOVA “Making Things” series, and has been interviewed by countless media outlets, including Wall Street Journal, Time Inc. and CNN. She has over 60 referred journal publications and 20 U. S. patents. Her work has been acknowledged with awards such as the Henry Ford Technology Award, the R&D100 Award, the Free Press Automotive Leadership Award, the Environmental Management Association Award, 5 SPE Environmental Innovation Awards and the American Chemical Society's Industrial Innovation Award. She has spoken at prestigious outlets such as TED and the Smithsonian “Age of Plastics Symposium”. |
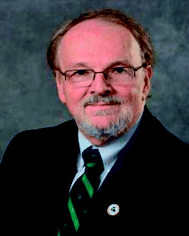 Lawrence T. Drzal | Dr Drzal is a University Distinguished Professor of Chemical Engineering and Materials Science at Michigan State University. He is Director of the Composite Materials and Structures Center at MSU, an interdisciplinary research center focusing on all aspects of polymeric, composite materials and their processing. He was co-Director of the NSF State/Industry/University Center for Low-Cost, High-Speed Polymer Composites Processing. From 2015 to 2018 he was the Vehicle Application Director of the Institute of Advanced Composite Manufacturing Innovation (IACMI). Dr Drzal is a founding member of the Adhesion Society (President 1998-2000) and the American Society for Composites. In 2007, Dr Drzal co-founded XG Sciences, Inc, a private Michigan company that is the world's largest manufacturer of graphene nanoplatelets using processes and technologies developed at MSU. He serves as its Chief Scientist. Dr Drzal's research is primarily in surface and interfacial aspects of polymers in adhesive joints and the fiber-matrix interphase in composite materials. This has consisted of research on carbon, glass, cellulose and aramid fiber surfaces: fiber/matrix adhesion measurement; surface modification by plasma, sulfonation and UV light in air; adhesion to thermoset and thermoplastic polymers; via microwave, e-beam, liquid and powder processing. His current research is directed at polymer nanocomposites and bio-composites, including graphene nano-platelets, their surface chemistry and processing to improve the mechanical, thermal, and electrical and barrier properties of the resulting multifunctional composites. Dr Drzal and his group members have published over 450 research papers (H index = 112), have been awarded 41 patents and over 600 presentations at national and international conferences. He was an editor of the CRC Press volume, “Natural Fibers, Biopolymers and Biocomposites,” Taylor & Francis Group, Boca Raton, FL (2005); He has received numerous awards for his research. |
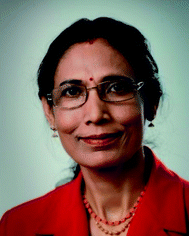 Manjusri Misra | Dr Manjusri Misra is a professor and Tier 1 Canada Research Chair (CRC) in Sustainable Biocomposites in the School of Engineering and holds a joint appointment in the Department of Plant Agriculture at the University of Guelph. As well, she is the Research Program Director of the Bioeconomy Panel for the Ontario Agri-Food Innovation Alliance, a program between the Ontario Ministry of Agriculture and Rural Affairs (OMAFRA) and the University of Guelph. She is a Fellow of the Royal Society of Chemistry (UK), the American Institute of Chemical Engineers (AIChE), and the Society of Plastic Engineers (SPE). Dr Misra's current research focuses primarily on novel bio-based composites and nanocomposites from agricultural, forestry and recycled resources for the sustainable bio-economy moving towards Circular Economy. She has authored more than 700 publications, including 407 peer-reviewed journal papers, 21 book chapters, and 53 patents. She was an editor of the CRC Press volume, “Natural Fibers, Biopolymers and Biocomposites,” Taylor & Francis Group, Boca Raton, FL (2005); American Scientific Publishers volume “Packaging Nanotechnology”, Valencia, California (2009); “Polymer Nanocomposites”, Springer (2014) and “Fiber Technology for Fiber-Reinforced Composites”, Woodhead Publishing (2017). She was the chief editor of “Biocomposites: Design and Mechanical Performance” Woodhead Publishing (2015). She was the President of the BioEnvironmental Polymer Society (BEPS) in 2009. In 2012, Dr Misra received the prestigious “Jim Hammar Memorial Award” from BEPS. In 2017, Dr Misra has also received the Andrew Chase Division Award in Chemical Engineering from the Forest Bioproducts Division of AIChE. She was selected as one of Canada's Most Powerful Women: Top 100 Award Winner in the Manulife Science and Technology from the Women Executive Network. In 2021, Dr Misra received the BEPS Lifetime Achievement Award. |
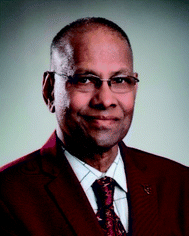 Amar K. Mohanty | Dr Amar Mohanty is a Professor and an OAC Distinguished Research Chair in Sustainable Biomaterials. He is an international leader in the field of biomaterials with a focus in engineering new sustainable materials. Prof. Mohanty's research interests have focused on the bioeconomy related to bioplastics, biobased materials, and sustainable composites. He has more than 800 publications to his credit, including 419 peer-reviewed journal papers and 67 patents awarded/applied. He has 39 418 citations of his research with an h-index of 90 (Google Scholar). Prof. Mohanty has received many awards, including the JL White Innovation Award from the Polymer Processing Society; Synergy Award for Innovation from Natural Sciences and Engineering Research Council of Canada (NSERC); Lifetime Achievement Award from the BioEnvironmental Polymer Society (BEPS); Andrew Chase Forest Products Division Award from the American Institute of Chemical Engineers (AIChE) and OAC Alumni Distinguished Researcher Award. Prof. Mohanty has been named a Fellow of the American Institute of Chemical Engineers, a Fellow of Royal Society of Chemistry (UK), a Fellow of the Society of Plastic Engineers, and a Fellow of the Royal Society of Canada. Prof. Mohanty is the Editor-in-Chief of Sustainable Composites, Composites Part C – Open Access. |
1. Introduction
With increasing awareness of the potential for more plastic than fish in the ocean, the challenge of removing, collecting and preventing waste from entering waterways is imperative in reducing such risks. Plastics can find their way to aquatic ecosystems through a variety of routes, including wastewater pathways, road runoff, and mismanaged waste. From a study by Jambeck et al.,1 and adapted from the online publication ‘Our World In Data’,2 a projected worldwide map for global mismanaged waste in at-risk areas for 2025 is displayed in Fig. 1 based on total predictions of 69.14 Mt of mismanaged plastic waste by 2025. This figure highlights the impact of effective waste management infrastructure, as waste produced within 50 km of coastlines is considered at high risk of entering oceans. Plastics generated in populations beyond 50 km of a coastline are considered unlikely to travel into ocean environments, and of the identified mismanaged waste in Fig. 1 anywhere between 15–40% is estimated to become marine debris.1 Some countries in Sub-Saharan Africa and South Asia, have inadequate disposal of 80–90% of total plastic waste.1 Overall, mismanaged plastic waste rates per capita have been found to be the highest in countries in the middle of the global income spectrum, as these regions have often undergone rapid industrialization but failed to improve waste management at the same rate.2 Infrastructure to limit mismanaged plastic waste includes, but is not limited to, user efforts to recycle or divert waste, end of life collection systems/transportation, and sorting and up-cycling facilities. If there was incentivized and/or public support for improved waste management infrastructure it is likely to reduce the environmental burden of negligent waste control.
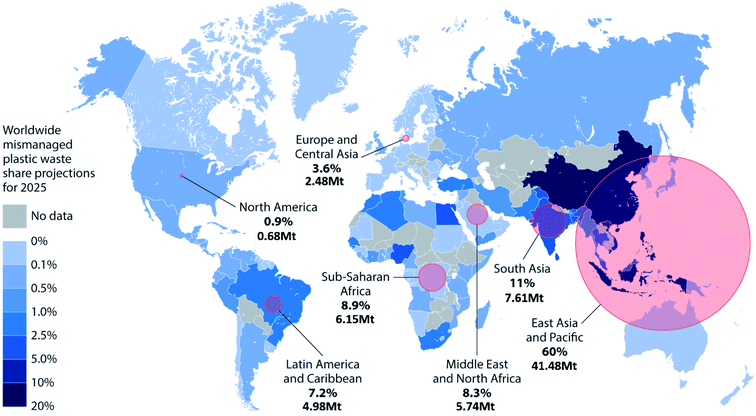 |
| Fig. 1 Projections for worldwide mismanaged plastic waste generated within 50 km of coastlines in 2025, with share contributions by each country indicated based on a total projected mismanaged waste of 69.14 Mt. Waste levels have been split into regions, indicated both numerically and visually with representatively sized circles.2 Estimates predict anywhere between 15–40% of these wastes to be converted to marine debris.1 | |
Some of the challenges of ocean plastic pollution can be addressed via environmental protection mechanisms like governmental legislation,3 infrastructure development, and ramped up collection efforts.4 As a global community, the collection of ocean waste would offer benefits to the environment by saving the health and lives of aquatic life as well as benefit society by leading to cleaner water systems. There are also potential economic benefits from fabricating up-cycled ocean plastics. The mass accumulation of nested plastics within the ocean is dependent on ocean currents and where the waste enters water systems.5 The next step in addressing the accumulated waste depends on collection effects and incentive for recycled oceanic plastics. Ocean plastics create considerable detriment in all oceanic levels, as plastics denser than water are accumulating rapidly at the bottom of the ocean floor and less dense polymers at the surface or shorelines. Special action is required to prevent accumulating plastics on the ocean floor as collection efforts are strenuous and economically unfeasible with current technology.
One method to improve interest in the collection of ocean waste is the potential economic viability for the plastics. A strategy to add value to such wastes is through a process called upcycling. Upcycling is a method of recycling plastics, which are then blended with other materials to generate novel, value-added products. The upcycling and recycling of ocean plastics contributes to a circular economic approach for sustainable product development where waste materials are given new life. This generates new economic value, but also helps to reduce the environmental burden of ocean plastics.6
A challenge with the implementation of recycled polymers is the loss in mechanical performance, structural integrity and/or thermal stability of the obtained materials.7 Polymer chains can change and result in degradation in a variety of ways, including submersion of plastics in water for extended periods of time, UV radiation from direct sunlight, and the salinity of the ocean water.8 The additives in the plastics also result in challenges with polymer processing or potential discoloration. To combat the effects of degradation, research has often combined compatibilizing agents with plastics to repair molecular chains and improve performances.9 Such strategies are being implemented with recycled ocean plastics and will likely continue if the goal is to upcycle oceanic plastics. As a result, upcycled ocean plastics come at a price premium and are of lower quality to virgin plastics. Plastic collection in oceanic environments is also a costly and time-consuming process with limited volume potential when compared to the volume of accumulated plastic waste.
Plastics collected from oceanic waste can be used in various processing methods, such as injection molding or three-dimensional (3D) printing.10 The recycled or upcycled materials could be used to generate value-added consumer products. There are many companies and non-profit organizations and academic institutions taking interest in such efforts. The hope is to develop products that align with a circular economic approach by resource retention, valorization, increased economic viability and sustainable product design.6,11
Plastics can be removed from aquatic environments through manual collection efforts12 and engineering designs.13 Some plastics with limited integrity are considered waste plastics as there is no opportunity to recycle or valorize for use in polymer applications. On the contrary, some plastics can be recycled and used to generate new value-added products. The challenges with these two types of materials is the requirement of collection and treatment options, as well as subsequent sorting if collected. Limited infrastructure and technology are feasible or viable to sort all collected oceanic plastic wastes.14
In addition to environmental destruction, microplastic accumulation, and limited collection or prevention efforts for oceanic plastics; some of the materials are quite resilient and may persist in the environment for thousands of years.15 Researchers have estimated half-lives for some plastics in marine environments, where half-life refers to the conversion of the first 50 wt% of the polymers mass. Excluding the effects of heat or UV radiation, high density polyethylene (HDPE) bottles have an estimated half-life of 23 to >2500 years; whereas HDPE pipes had an estimated half-life of 400 to >2500 years. Other plastics, like low density polyethylene (LDPE) bags had estimated half-lives of 1.4 to >2500 years. Fortunately, biodegradable plastic bags were suggested to have an improved half-life of 1.7 to 6.7 years.16 This review serves to summarize the most common sources of plastic pollution, their distribution routes into aquatic environments and potential societal and environmental impacts as a result of this. A focus on potential solutions to the ocean plastic crisis has been included, with the overall content of this review summarized in Fig. 2. The green arrows highlight where the impacts of oceanic plastics can be reduced and where there is potential for valorization of said wastes. The red arrows indicate the sources and negative impacts of oceanic plastics.
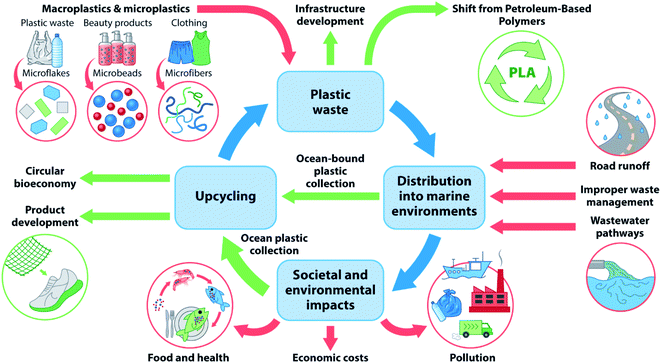 |
| Fig. 2 Summary of the lifecycle of ocean plastic waste, its ecological implications, and potential mitigation pathways. | |
2. Sources of ocean contamination
2.1. Macroplastics
Macroplastics identifies a size category for plastics that are larger than 5 cm in any one direction. Further size characterization of plastics are meso-plastics, which are in the range of 0.5 to 5 cm in any one dimension.17 These materials are found floating on the surface, at the depths of the ocean floor or even brought to land from the tides. There are many major contributors to macroplastics, including but not limited to, commercial fishing wastes, consumer products and construction wastes.18
2.1.1. Commercial fishing waste. Dumped fishing waste accounts for around 10% of total ocean plastic pollution. These fishing wastes have been found to be one of the largest contributions of macroplastic pollution found floating on ocean waters approximated at 70%. Reports in 2019 estimate that 640
000 tonnes of discarded fishing equipment, such as old fishing nets, are left in the ocean each year (Greenpeace).19 The fishing industry is largely unregulated when it comes to plastic waste, which allows large quantities of fishing equipment to be abandoned or dumped.19 This broken down fishing gear includes nets, traps, pots, or long lines to name some examples, and can continue to trap, entangle, and potentially kill marine life, as well as damage oceanic habitats.18 Fishing nets left in the water, referred to as ghost nets, can result in significant harm to marine ecosystems, as well as lost economic potential.20,21 This is one of the most apparent forms of marine plastic waste, and it can persist in these aquatic environments for extended periods and travel great distances through ocean currents. The challenge with fishing equipment which is submerged in marine environments for extended periods of time is the resulting ultraviolet (UV) and salinity damage, which causes depolymerization of the chains in plastics.20 The presence of ghost gear in aquatic environments has been found responsible for roughly 10% of the global decline in fish stocks, serving to increase fishing costs. Ghost gear also has economic impacts through expensive cleanup efforts, as well as through losses in tourism due to polluted shores.22
2.1.2. Consumer products. Consumer products contribute the largest proportion of plastic waste of all sectors. Plastic waste volume is affected not only by primary plastic use, but also product lifetime. Hence, plastic waste is elevated in consumer products as the majority are designed to have a limited lifespan, especially in packaging with an average ‘in-use’ lifetime below 6 months.23 In 2015, packaging waste totaled to 141 million tons, which was nearly half the global total of plastic waste.2 In 2019, the nonprofit environmental group Ocean Conservancy conducted its annual international coastal cleanup, which indicated the top consumer waste present in ocean ecosystems and beaches. Since 2017, the most collected items have been entirely plastic-based, and all fall under consumer products. Fig. 3 displays that the top consumer wastes that end up in the ocean include cigarette butts, food wrappers, straws and stirrers, forks, knives, and spoons, plastic beverage bottles and caps, plastic bags, plastic lids, and cups and plates.24 The majority of these constitute single-use plastics, including product or food wrappers, containers, and packaging, which are used once and then discarded.25
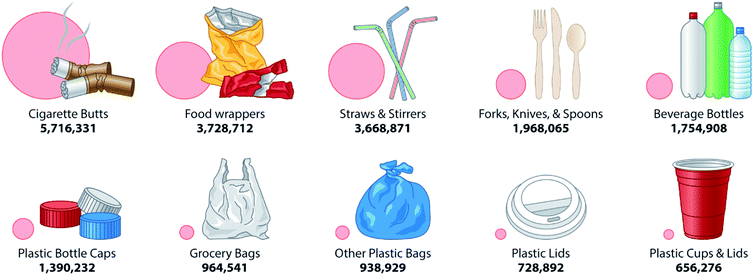 |
| Fig. 3 Ten most common items collected during the 2019 international coastal cleanup conducted by Ocean Conservancy, with the number of collected waste items indicated both numerically and visually.24 | |
These plastics have a damaging impact on marine ecosystems through the entanglement of and ingestion by marine life, and their buoyant nature allows them to float on the surface. Materials that float on the surface are those which possess a density less than or equal to that of water. Anything of greater density will sink to the ocean floor. However, studies have found that the ocean floor is the ultimate sink for a variety of marine debris and makes collection a serious challenge.26 Additionally, plastic pollution has a serious economic impact, with an estimated annual US$ 0.5 to 2.5 trillion loss via reductions in the oceans ability to provide oxygen, clean water, resources, seafood, cultural and recreational value, and regulation of the climate.27 Estimates in 2015 quote a global amount of mismanaged plastic waste at 80 million metric tonnes, and if current practices remain the same, this stands to triple by 2060 due to growing demand for plastic products according to research conducted by Lebreton and Andrady.28
2.1.3. Construction waste. In addition to packaging and commercial fishing waste, many macroplastics are associated with building and construction wastes. Waste from construction is the second largest waste producing sector, generating 65 million tonnes (Mt) of plastic waste every year (∼16% of all sectors).23 Nonetheless, only 4% of the plastic products related to this sector are leaked into the ocean. The construction sector produces solid waste, in which most of the residues are metal and concrete. In terms of plastic, polyvinyl chloride (PVC) is the most prevalent. The uses of PVC spans from window profiles, pipes, wires, coatings, floor tiles and many more. It is estimated that almost 25% of non-plastic waste generated in the construction industry is from packaging of building materials. These materials are unused and discarded, ending up as waste thrown into the ocean. In literature, it is proposed that one of the reasons for this high mismanagement of construction materials is because there are no financial incentives to recycle or use bio-degradable plastic.29
2.2. Microplastics
Exact definitions of microplastics vary between studies but are generally considered to be any plastics between 5 mm and 1 µm in diameter. Particles less than 1 µm then become classified as nanoplastics.30 There is a distinction between types of microplastics based on their origin, with primary microplastics being small particles or microfibers designed for commercial use.31 In contrast, secondary microplastics are those that are unintentionally fragmented.
2.2.1. Primary microplastics. There are a variety of sources of microplastics, with the most notable sources identified in Table 1, as adapted by a study conducted through the IUCN (International Union for Conservation of Nature).32 The most common sources of microplastics include plastic pellets, synthetic textiles, tires, road markings, marine coatings, personal care products, and a group of sources that frequently occur in urban environments that has been termed city dust. City dust includes abrasion of objects, such as synthetic footwear soles, abrasion of infrastructure, like building coatings, or intentional pouring of detergents.32 Due to a lack of regionalized and specific modelling, consumption of city dust products could not be accurately estimated in the study, and assessment of microplastic ocean release was based on basic extrapolation. The estimated global ocean release included both optimistic and pessimistic predictions, with optimistic estimates of annual microplastic release at 800 ktons per year and pessimistic estimates as high as 2500 ktons per year.30 This large range stems from the difficulty in accurately measuring microplastic ocean pollution due to identification problems at such small plastic sizes.
Table 1 Common microplastics with annual consumption and corresponding ocean release based on optimistic and pessimistic estimations, adapted from a report by the IUCN32
Microplastic source |
Global consumption (ktons per year) |
Global ocean release (ktons per year) |
Loss mechanism |
Plastic pellets |
257 000 |
2.4–7.5 |
Spillage during transport, recycling, and manufacturing |
Synthetic textiles |
42 500 |
280–875 |
Laundry induced fibre shedding and abrasion |
Tires |
6430 |
224–700 |
Abrasion during driving |
Road markings |
588 |
56–175 |
Vehicle abrasion and weathering |
Marine coatings |
452 |
29.6–92.5 |
Release during application, maintenance, disposal, and weathering |
Personal care products |
42 |
16–50 |
Direct pouring through product use |
City dust |
N/A |
192–600 |
Abrasion, pouring, and weathering |
The majority of microplastic releases occur through normal product use, followed by product maintenance, and minor contributions via production and transformation of the plastic, with corresponding percentages of 63, 36, and 1%, respectively. Of this, 77% comes from household use and only 23% from industrial activities.32 The majority of microplastic release is unintentional, apart from personal care products. Plastic microbeads (typically made of polyolefins, such as polypropylene (PP), polyethylene (PE), and polyamide (PA)) are commonly utilized as a scrubbing agent in cosmetics and personal care products, and are directly released into wastewater streams through regular product use.33 This can clearly be observed through the comparison of annual consumption and projected ocean release of this microplastic source. Other large contributors to ocean microplastic waste include synthetic textiles, tires, and dust sources due to abrasion and weathering.34 Polymer-based textiles are abraded during washing and result in sewer discharge of microplastic particulates. Car tires are another major source, as the tire surface is composed of natural rubber and synthetic polymers (styrene-butadiene rubber and other additives) that are degraded through constant abrasion and enter waste pathways through runoff.35 Dust sources cover many inputs that individually contribute minor amounts of microplastic pollution but grouped together become significant.
Microplastics possess a significant risk to aquatic life since these plastics can not only float but also sink to the ocean floor, increasing the area in which is contaminated with plastics.36 Furthermore, due to their small size they are considered available for consumption by organisms and can lead to bioaccumulation. This is made worse by the fact that their composition and relatively high surface area leads to leaching of toxic plasticizers and adhesion to organic pollutants.31 Unless waste management systems and microplastic sources are overhauled and revised, future predictions by Wijnen et al.37 estimate that microplastic waste could increase by as much as 51% by 2050.
2.2.2. Secondary microplastics. Some of the most common sources of secondary microplastics are those derived from macroplastics, namely bags, bottles, ropes and lids. Materials experience mechanical wearing and degradation from tidal waves over time, as well as exposure to salt and UV rays. Researchers measured the fragmentation time of a coffee cup lid made of polystyrene (PS). In only 56 days, the lid produced up to 126 million particles with an average size of 224 nm.38 The small particle size is concerning due to the likelihood of ingestion by marine life which confuse plastic particulates with food.38Researchers studied the effects of light on some plastics using a solar simulator. Samples were irradiated with simulated sunlight for two months to measure plastic scission mechanisms. The results showed that a major byproduct of plastic photodegradation was dissolved organic carbon microplastics, which had also undergone discoloration. Using linear extrapolation in this study, they were able to predict sample weight loss and calculate their corresponding lifetime. FTIR results showed that the buoyant North Pacific Gyre (NPG) fragment samples contain 67, 16, 15, 1.4 and 0.86% of PE, PP, ethylene–propylene copolymer, polyurethane and ethylene–vinyl acetate copolymer, respectively. The shortest lifetime after irradiation in a solar simulator system was a sample taken from the NPG (2.8 years), followed by postconsumer PP (4.3 years) and PE (33 years).39 Despite NPG containing 67% PE, its extrapolated lifetime of 2.8 years is significantly lower than postconsumer PE at 33 years, showing the influence of aquatic ecosystems on plastic fragments.
In contrast with primary microplastics, secondary microplastics have random shapes, which hinders their categorization when recovered. Fragmentation of microplastics can occur as a result of ultraviolet rays from the sun, starting photodegradation of the macromolecule chains and resulting in discoloration, increased brittleness and a reduction in the average molecular weight (Mw) of the plastic material. Color can be an indicator of the degree of degradation, as yellowed PE and PP plastics have been found to have an increased amount of polar functional groups, and thus a higher affinity for certain chemical pollutants.40 This yellowing arises from oxidation of phenolic antioxidant agents to quinoidal structures characterized by a yellow colour shift, giving them a greater ability to absorb PCBs. It is further notable that pigmentation itself has no correlation to the degree of degradation.
3. Environmental impacts of ocean plastics
Ocean plastics pose a serious threat to oceanic life and the stability of marine ecosystems due to their persistence. Plastic accounts for 50–80% of the accumulated debris in marine environments, and due to their buoyancy they are widespread.41 The most evident form of marine impact comes through an entanglement of marine creatures, which can serve to restrict natural movement, or in severe cases lead to injury and death through constriction. This is most commonly due to rope and netting, packaging materials, and discarded fishing materials.42 In addition to this, plastic has been seen to be a vector for disease in marine habitats, such as coral reefs. Microbes that cause white syndrome and black-band disease on coral tissue have been found to be effective at colonizing on plastic surfaces, as the likelihood of disease for reefs raised from 4% to 89% upon induction of plastics.43
The ingestion of ocean plastics by aquatic life is of great concern due to the host of effects they can have, and their potential unseen long-term effects. The size of microplastics leads to aquatic life, birds, and other mammals confusing the particles as food, such as plankton.44 The ingestion of plastics can lead to immediate effects, such as intestinal blockages or organ puncture. However, they have been observed to have other long-term effects, such as sex determination in some organisms, or nervous system damage in others.45 In addition to this, plastics can contain harmful compounds and absorb pollutants present in the ocean, which can have adverse effects on organisms once ingested. These health effects range from organ damage, reproductive changes, greater susceptibility to disease, and other potentially unknown long-term effects.46
Studies have begun to analyze the effect of microplastics on humans, as bioaccumulation up the food chain leads to their consumption. In a study performed in Newcastle, Australia, it was estimated that every week humans ingest an average of 5 grams of plastic.47 The study reveals that the route of plastic consumption is through food, beverages and inhalation. Weekly drinking water intake can lead to the ingestion of 1769 particles, and found to end up in the digestive system.47 In another study, the concentration of microplastics from various sources were characterized, with seafood having 1.48 microplastics per gram (MPs per g), bottled water having 94.37 MPs per L, tap water with 4.23 MPs per L and air having 9.90 MPs per m3.48 Microplastic concentrations are variable depending on the source, but water, air and seafood are the main contributors towards the ingestion of microplastics.
Additives are used in plastics for many reasons including, but not limited to, improved processability, enhanced barrier properties to increase product shelf life, UV stabilizers or colorants. Additives, such as tetrabromobisphenol A (TBBPA), hexabromocyclododecane (HBCDD), and polybrominated diphenyl ether (PBDE) may lead to toxic effects on flora and fauna, threatening the aquatic food chain and inevitably reaching humans.46 Other additives that have a significant impact on health are UV stabilizers, which are used in plastics to prevent degradation. The most common of these is benzotriazole, which has caught the attention of researchers due to its presence in polluted rivers and lakes.49 Similar to flame retardants, UV stabilizers tend to bioaccumulate and are associated with endocrine disruption and thyroid gland alterations, which are in charge of the development of reproductive organs.50 The key consideration here is that stabilizers are compounded into plastics at low concentrations between 1 to 5 weight percentage (wt%); however, their widespread use in many plastics makes this an issue.
4. Distribution of plastics into marine environments
4.1. Road runoff pathways
Road runoff is another major route of ocean plastic distribution, being the movement of plastic sources due to wind or rainfall. Some of the major sources of plastics coming from this pathway include tire abrasion, road markings, and plastic pellets.32 In developed countries, rural areas are a minor contributor to ocean plastic waste (3.5% of losses), while urban runoff is often collected in sewer systems and can either be dumped directly into aquatic environments or enter wastewater pathways. Road runoff is one of the largest microplastic distribution pathways, accounting for 66% of the total, and of this an estimated 32% end up as ocean releases.32 A study conducted in Denmark analyzed several stormwater basins to examine the effect of urban and highway stormwater runoff. All seven ponds had the presence of microplastics, ranging wildly from 490–22
894 items per m3, with the most prevalent polymers being PP, PS, PVC, PE, and polyester.51 These fragments can come from a multitude of sources such as vehicular debris, road construction, and additives or materials used in road maintenance. Perhaps unsurprisingly, the lowest microplastic concentrations came from ponds serving residential areas and highways, while the highest came from those close to industry and commerce. Where insufficient stormwater drainage systems are in place or entirely missing, the stormwater directly enter the oceans untreated. Many countries have enforcement agencies in place to ensure proper drainage at industries and construction sites, but this runoff can also come from many human activities, such as littering, poor recycling or sorting practices.52 Potential mitigation methods for road runoffs include permanent stormwater retention ponds, sediment traps, diversion trenches, grass strips, and slope protection.53 However, this will require substantial, costly infrastructure development worldwide.
4.2. Plastics from wastewater pathways
Microfibers are released from fabrics during the laundering process. It was estimated that nearly 2 Mt of microfibers are released into the ocean annually.54 The most common forms of microplastics that enter wastewater pathways include personal care products and cosmetics, being primary plastics, as well as abrasion of plastic products and tires, textiles, and road marking paints. Most of the microfibers are made from synthetic polymers, which are non-biodegradable and of a small size, allowing them to accumulate in waterways.54 Challenges with the leakage of microfibers exist because in many areas municipal water is discharged into the environment without any treatment procedures.55 In fact, estimates made by the United Nations suggest that as much as 80% of global wastewater remains untreated once released back into the environment.56 As global development continues, a growing number of people will have access to proper sanitation, meaning that the volume of wastewater will increase. To combat the accumulating amounts of microplastics, the implementation of wastewater treatment plants has been gaining interest and could be a viable solution to reduce the gross increase in microplastics in aquatic environments.57 Some treatment processes can trap microplastics in the sludge, but it is not an absolute means, and these particles can end up in soil through sludge application in agricultural processes. The microplastic particle shape and size affect how easily they can be removed from wastewater pathways, with microfibers from textiles being the hardest to capture due to their smooth surface and high length to width ratio. The technology to effectively capture microplastics in wastewater has been developed, with advanced tertiary treatment processes conducted in Finland achieving a 99.9% microplastic removal rate, while many less advanced processes only capture around 60%.58 To effectively control this plastic waste distribution route, treatment plants require an overhaul, and wastewater management costs are outweighed by the potential benefits for economic development, environmental protection, and human health.56,59 Another technique to diminish microplastics is the use of effective washing machine filters and laundry balls, which can easily be adopted by consumers to reduce microplastic fibers.60 Napper et al.61 studied the efficacy of some fiber devices on capturing microfibers released from wastewater in the washing machine. They found that Guppyfriend and XFiltra washing bags can reduce the fiber generated by the laundry process by approximately 80%.
4.3. Improper waste management
While great strides have been put forth to develop proper infrastructure for the collection of plastics, an estimated 32% escape these waste management systems globally.1 Over 80% of the plastic debris found in marine environments trace back to land-based sources, with the largest contributor stemming from industrialized areas due to solid waste disposal, littering, and plastic usage.62 This stems from the low residual value and the small size of many plastic packaging products, resulting in over 8 Mt of plastic leakage into the ocean annually.63 As of 1950, nearly half of all produced plastic products have been sent to landfills or disposed of in nature, with proper recycling of plastics only contributing to 9% of the total collection. Recycling rates vary wildly depending on the country, with rates as high as 30% in countries that have proper regulations and promote recycling, and close to 0% in developing economies that lack an industrial waste management system.64 As discussed previously and highlighted in Fig. 1, improper waste management is most prevalent in middle-income countries.
There are many obstacles that need to be overcome in terms of improving global recycling, as in many instances reclamation of plastic material is not an economically viable option due to the low price of virgin polymers compared to recycled plastics. Furthermore, due to the wide variety of available plastics and variable quality of the product after use, advanced processing technology and an in-depth knowledge is required to avoid defects and produce a high-quality plastic product.65 Another issue is that many plastic products are not recyclable, or of a composite nature with unrecyclable components. PVC is difficult to recycle and often incinerated, while difficulty in depolymerization of polyolefins limits their ability to be chemically recycled. The only closed-loop recyclable plastics are condensation polymers such as polyesters (e.g. PET) and polyamides (e.g. nylon 6).66 Improvement of recycling infrastructure is thus of great importance, as it contributes to a circular economy and hence diminishes ‘new’ plastic in circulation, as well as reduces plastic that could otherwise be improperly disposed of. These findings indicate that the development of waste management infrastructure is an essential component towards the reduction of plastic pollution, especially in middle-income countries. Moreover, there are efforts to improve recycling technology by designing packaging for recycling, reducing use of multilayers, diminishing pigments and additives, reducing dependence on single-use and disposable plastics, and changing overall community's habits.67
5. Applications of ocean plastics
5.1. Upcycling of ocean waste
Finding a solution to solve the environmental problem caused by single-use plastics is preferable over the outright banning of the products. There is an inherit dependency on the convenience and cost incentive of using plastics as compared to metals or ceramics in industry. There is also limited infrastructure currently to recycle or sort bioplastics. In reaction to the negative environmental impacts of plastic and to provide a solution, numerous industries and nations have been attempting to create an appropriate strategic vision, with plans of upcycling plastic waste to develop a circular economy. This means that the plastic recovered from rivers and oceans can be reused and enter a closed loop. Recently, the European Union established that more than 50% of plastic produced in Europe would be reused or recycled by 2030.68 Similarly, in 2019 Canada vowed to outlaw many common single-use plastics by as early as 2021 to decrease plastic waste in the ocean.69 This will require design enhancement and improvement of the sorting and collection of plastic, as well as creation of viable markets for recycling. Japan also attempted a new regulation to reduce the consumption of single-use plastics by 25% and shift towards biomass plastic use (∼2 M ton). This is proposed to be completed by 2030, and they also plan to reuse/recycle all packaging by 2025.70 Many US states and other countries globally applied a legislative initiative to force industries to reduce the use of single-use plastics (straw, PS foams, plastic bags and cutlery), with increased prices for all disposable plastics to discourage consumer use.71 Some companies and researchers have also begun to use ocean plastic waste for the fabrication of new products, which has been highlighted in Table 2. It is important to note, however, that many companies demonstrate the ability to utilize marine plastics in their products with the primary goal of garnering public approval. For example, the Coca-Cola company only produced around 300 sample plastic bottles made from 20–25% recycled marine plastics recovered from beaches and the Mediterranean Sea.72 The aim of this was the development of a closed-loop economy by decreasing the amount of virgin PET and increasing the amount of recycled plastics, although lacked high volume outputs. Improved processing technology and collection strategies can increase the feasibility of these products and help raise consumer awareness to the issue of ocean plastics.
Table 2 Upcycled products from recycled polymers and oceanic plastic wastes
Company/research |
Upcycled material |
New product |
Reference |
Ocean Plastic Parley and Adidas |
(1) About 16.5 recycled PET bottles per shoe |
New running shoes (11 million produced in 2019) |
73 and 74 |
(2) Nylon gill nets |
Unifi |
PET plastic from 4 billion bottles |
Yarn for clothes |
75 |
North Point |
HDPE collected by Plastic Bank |
Sunglasses |
76 |
Method |
Plastic debris |
Soap bottles |
77 |
Coca Cola |
Plastic from beach of Mediterranean sea |
20–25% recycled content soda bottles |
72 |
Research |
Waste LDPE/HDPE |
Sulfonated PE composites with carbon fibers and multi-walled carbon nanotubes (MWCNTs) |
78–80 |
Research |
Sulfonated waste LDPE/HDPE |
Porous material for gas caption and dye removal, lithium–sulfur batteries, and Li-ion battery anodes |
80 |
Research |
Waste PE |
High-voltage supercapacitor: Composites with 2.5 wt% graphene from pyrolyzed waste PE |
81 |
Research |
Waste PE |
Drug delivery: Magnetic Fe3O4@C core shell composites from catalytic pyrolysis of waste PE |
82 |
The activism conducted by these industries serve to motivate other industries to incorporate ocean plastic waste in their products. However, the success of these products requires a design plan for the collection and sorting of various types of plastic, as well as consumer willingness to purchase products that may be of lower quality or contaminated.83 A recent survey in the Netherlands showed that 59% of random consumers had the potential to pay a price premium for products made from ocean plastics.84 Initiatives such as these serve to increase awareness in the average consumer regarding the issue of ocean plastics in the hopes of changing mindsets, but by no means are a standalone solution to the problem as a whole due to the volume of ocean plastic waste and long-term economic cost. It is the hope that upcycling of ocean waste will instead serve to encourage industries to reuse and recycle plastic at the source instead of using virgin polymer due to pressures of brand perception regarding waste.
The traditional methods used for plastic waste management include incineration, as well as recycling via mechanical methods (downcycling), which leads to lower-value products compared to their originals (8% cascaded recycling according to MacArthur Foundation study 2016),63 with insufficient energy recovered.85 This suggests there is a limited number of time plastics can be recycled. There are efforts being made to improve the recyclability through chemical methods. Chemical recycling in the presence of a catalyst may require less energy but is not yet applied on a large scale, giving it potential to open the door for the production of high-quality plastics through recycling.86 Chemical recycling has been made as an alternative to less favorable mechanical recycling processes. However, the recycling and recovery processes are extremely low currently due to inefficiency of mechanical recycling, higher costs of recycling and lower product quality. Improvements to mechanical recycling require the development of sensors and robotics that can do a much better job at sorting. The challenge is that such systems require investment and infrastructure to do so. Europe has addressed some of the challenges with mechanical sorting by sorting household plastics into nearly 13 categories as compared to only 6 in Canada and the USA. An additional alternative to both mechanical and chemical recycling is to upcycle plastics, which includes valorization of the plastics into value-added applications. For materials where material recovery is not feasible, such as highly degraded ocean plastics, energy recovery methods like incineration are desirable for their ability to reduce waste mass and volume, as well as eliminating possibilities of waste putrefaction. Overall, integrated waste management systems that incorporate material recovery through recycling, thermal treatments, and biological treatments show the greatest promise for addressing concerns with plastic waste.
5.2. Challenges in product manufacturing
There are several challenges facing ocean-plastic product manufacturing that are limiting its effectiveness. Collection of ocean plastics can prove very challenging due to complex transport mechanisms via ocean currents.14 The environmental engineering company Ocean Cleanup is in the process of developing a system of sensors, ocean vessels, and platforms that can passively collect floating debris, but greater efficiency and economic feasibility is required.14 Furthermore, there is the issue of detection and collection of ocean microplastics or plastic products that have accumulated on the seafloor.30,62 There are further issues of varying levels of ocean plastic degradation once in marine environments due to wind, waves, UV radiation, bacteria, and seawater.8 Different polymers experience degradation at varying rates depending on their chemical structure, which dictates their susceptibility to biotic, abiotic, and photodegradation processes.8 As a consequence, re-use of these partially degraded plastics can prove difficult in many cases.14
The mixing of a variety of ocean plastics often leads to a gray resin that is undesirable for consumer products, as discovered by the company Method during development of soap bottles with 10 wt% HDPE beach waste.77 As colorants are limited by processing, formulation, and stability issues of the matrix and the colorant itself; this can pose another challenge for the development of ocean-based commercial products.87 There have also been studies looking into the economic feasibility of ocean plastics, as the additional collection and repurposing inevitably leads to a price premium for ocean-plastic products. Furthermore, perceived product contamination and functionality of recycled ocean plastic has a direct consequence on consumer demand, meaning that the fabricated products must be of a similar high quality to virgin polymers to be economically feasible.84
5.3. Use of ocean-bound waste
An alternative to the direct collection of plastic from ocean and river environments is the implementation of proper waste management to capture plastic bound for the ocean in at-risk populations. At-risk populations are defined as those that are within 50 km of a coastline that have no formal waste system, in which plastics are likely to reach the ocean.1 A study by Jambeck et al.1 estimated that of the 192 coastal countries analyzed in 2010, plastic waste generation within 50 km of the coastline was as high as 99.5 Mt, and of this 31.9 Mt was mismanaged. Based on these predictions, around 1.7–4.6% of the total plastic wastes generated by these counties enter ocean ecosystems, or between 4.8–12.7 Mt. This value has been predicted to increase dramatically by 2025, particularly if waste management infrastructure is not improved due to population and economic growth in middle-income countries. There are a variety of strategies to combat this growing issue, and if adequate waste disposal was improved by 50% in the top-20 ranked ocean plastic contributing countries, mismanaged plastic waste mass would decrease by 41% by 2025.1
Elimination of plastic waste directly at the source through proper waste management is often cited as the most viable route to ocean restoration.88 This requires an integrated system, with a focus on plastic life-cycle improvements and the four-R's hierarchy (reduce, reuse, recycle, recover) through government initiatives, consumer education, and infrastructure improvements.88 There have been recent efforts put forth to encourage companies to utilize recycled plastic materials and contribute to a circular economy, and consumer evaluations indicate a willingness to pay a price premium for recycled ocean plastic products that are of similar quality to virgin polymers.84 In Taiwan, waste disposal rates were reduced from 0.9 to 0.48 kg per capita through governmental policies that enforced waste sorting and banned plastic bags and tableware, with similar results seen recently in local Australian councils.88 Implementation of proper collection systems in at-risk populations, while promising, is a long-term goal and is currently unfeasible for developing countries due to its complexity and expensive nature.
6. Development of a supply chain
Recently, many countries, stakeholders, profits and non-profit organizations, as well as academic institutions and companies, have begun working together in collecting and cleaning plastic from the ocean. In 2018, HP and IKEA announced that 25 tonnes of ocean plastics would be collected and invested in a washing line in Haiti to upcycle the development of new HP ink Cartridges, providing job opportunities, nourishment and study access to children.89 By 2050, Dell pledged to enhance the incorporation of ocean-bound plastic in their products 10-fold and to open source its supply chain to motivate other industries to use waste ocean plastics.90 Plastic Bank and Oceanworks are two global marketplaces for ocean plastic polymers, blends and products. They have collected and sorted a high volume of plastic and guaranteed a consistent supply chain of ocean plastics. Plastic Bank has recovered more than 12 million kg of ocean-bound plastics, and collaborated with partners to produce different products that serve to help prevent plastics that are ultimately bound for the ocean.91
Collection of plastics prior to their entrance into the ocean is highly desirable due to the various issues found for ocean plastic manufacturing, including degradation, lengthy and expensive collection endeavors, and colorant issues. Thus, improving existing supply chains for recycling of post-consumer plastics to prevent their entry into aquatic ecosystems presents itself as an ideal route of mitigation. As such, there has been initiatives to make ocean-bound plastic products more feasible through development of a global supply chain, including waste management improvements in at-risk populations, material storage, and product development. In Haiti, HP has developed a bottle collection program in collaboration with the First Mile Coalition, which offers education to the children of plastic collectors. Envision Plastics, a HDPE plastic recycler, committed to the collection and recycling of 10 million lb of HDPE from at-risk communities to bring comfort to brands that have interest in creating sustainable products, as well as lowering the cost of ocean-bound plastics.90 In 2017, through a partnership between Dell and the nonprofit organization Lonely Whale, NextWave Plastics was cofounded, which is a consortium of companies that seek to keep plastics out of our oceans.92 This consortium now consists of ten companies with collection efforts in Chile, the Philippines, Indonesia, Cameroon, and Denmark.89 Substantial product development has already occurred, with Envision Plastics launching the first commercial product with 100% ocean-bound plastic content in 2018.90 Further, the automotive supplier DSM has recently developed a recycle-based polyamide named Akulon RePurposed from discarded fishing nets.93 However, further development and strengthening of this plastic supply chain is required to bring value-added uses to plastic collected in at-risk areas.
7. The future of plastics
To address concerns with oceanic plastics, there needs to be a combination of collection efforts, remediation efforts and general consumer awareness. Remediation is a common practice for reducing environmental damage and currently involves practices such as filtration, chemical degradation, distillation and coagulation of wastewater. Research is still looking for ways to address oceanic wastes through microbial or enzymatic degradation, nanotechnology or photocatalysts.94 As noted in Fig. 4, strategies to address ocean plastics can be categorized as either biotechnological tools, engineering tools, manual collection efforts or materials design. Biotechnological tools include micro-organisms, worms or enzymes designed to breakdown plastic wastes.95 Bacteria and fungi break down plastics through cleavage of bonds on polymer chains, whereas micro-organisms function through molecular uptake strategies.96 Other biotechnological organisms function through mineralization or bio-deterioration practices.96 Manual collection of ocean plastics has been a priority for many volunteer-based associations. There are also manual collection efforts that involve strategically designed equipment to collect waste. In 2010, Living Lands & Waters (LL&W) collected and removed 610
024 pounds of debris from the Mississippi River. One notable collection mechanism is the Ocean Plastic Harvester presented by Boyan Slat, which operates via an autonomous zero fossil fuel-based tug system and collects plastics headed to shore and is scheduled to harvest over 13
227 tons of waste plastic in its first year of operation.13 A simulation study of plastic debris in the ocean in 2019 showed that by 2030 waste plastic can be reduced by 25%. However, this requires cleaning of around 135 Mt of waste plastic from the ocean (∼15% of total ocean plastics) over the period of 2020–2030, with cost estimates ranging around 492–708 billion euros, constituting 0.7–1.0% of the total world GDP in 2017.97 There are current efforts for material design that focuses on the transition from single-use plastics to biodegradable.98 Biodegradable materials can be made from biological or synthetic sources but reduce to carbon dioxide and water in a relatively short period of time when subject to specific soil, compost or marine conditions.99,100 However, many of the polymeric materials that degrade in soil or compost are unable to breakdown in marine environments comparatively. In fact, some of the only marine biodegradable bioplastics are within the family of bioplastics known as polyhydroxyalkanoates.101,102 PHAs and other biodegradable plastics are typically much more costly and thus have less widespread use in industrial applications at the current time, making up <1% of total plastics. Furthermore, the lack of degraders in aquatic environments leads to many bioplastics like PLA having extremely low degradation times on the scale of years.103 As such, they do not pose an immediate solution to the plastic crisis and material design in the near future should focus more on increasing recyclability and reuse of plastics, notably from single-use and packaging sources through mandated governmental regulations as discussed previously.
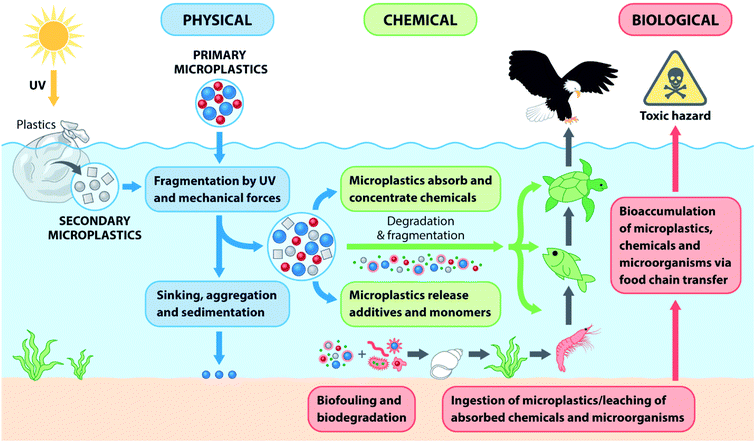 |
| Fig. 4 Summary of oceanic plastics accumulation and cycle with physical, chemical and biological degradation.104 This is an unofficial adaptation of an image that appeared in an ACS publication. ACS has not endorsed the content of this adaptation or the context of its use. | |
A shift away from the linear economy model is needed to address the issue of increasing plastic waste. This model includes the extraction of resources required to produce commercial plastics and products, distribution and then consumption, which end up in the natural environment (such as oceans) or landfills as a waste material.105 Around 95% of all packaging plastic is lost per year (equal to $80–120 billion) and consequently damages the marine environment by around $13 billion per year, which significantly contributes to the plastic waste crisis.106 On the other hand, the circular economy model is based on decreasing plastic waste through new manufacturing designs, product retention and the reuse of waste material. Shifting away from a linear economy requires a shift in the government's laws and approach from the consumers. Circular economy concepts help the environment by dropping green house gas emissions that arise during a products life cycle, reducing reliance on raw materials, as well as minimizing waste plastic going to landfills. Plastic manufacturing methods must shift towards designing long-lasting reusable materials through renewable feedstocks, as well as decreasing cycle losses by upcycling waste plastic.106 An even better model, being that of a circular bio-economy, consists of the conversion of biomass waste and renewable resources into value-added products. This can be achieved through replacement of fossil fuel based plastics with biobased plastics, adoption of renewable energy, increased recycling and decreasing plastic demand.107
8. Conclusions and recommendations
It is unsurprising that ocean plastic contamination has risen drastically as a function of our plastic dependence. These polluting sources vary greatly, with macroplastic sources largely coming from commercial products, fishing waste, and construction byproducts. Degradation of these plastics in aquatic environments can lead to the release of microplastics, as well as through abrasion and weathering of common plastic products. These plastics have a variety of means to reach aquatic environments, including through regular product use in wastewater streams, road runoff, and improper waste management infrastructure. The effect these plastics have on marine ecosystems are still not fully understood but can lead to bioaccumulation and harmful health effects in a variety of organisms, elevated pollution levels, create undesirable living conditions and lead to economic costs. Efforts have been made to develop new applications and a supply chain framework for collected ocean plastics to make them more industrially feasible, but a broader approach is required to address the problem of ocean plastics more thoroughly.108 The global ocean plastics problem is one of great complexity and deeply rooted in societal norms. Thus, an effective solution requires an integrative approach over both the short and long term. To reduce marine plastic pollution, immediate interventions are required that address the root cause of ocean plastics, such as through addressing gaps in waste management infrastructure worldwide. Ocean pollution can further be reduced through increasing awareness and education on the devastation that plastic pollution has on ocean ecosystems and the environment. To collect only 15% of ocean plastics via ocean and coastal cleanups is predicted to cost between 500–700 billion euros and results in plastics of mixed quality, and also does not serve to address the root cause to plastic pollution. However, while not significant from a collection to volume standpoint, cleanup provides benefits to localized areas and helps change mindsets in consumers, and consequently companies, in terms of recycling and waste plastics. The projected costs for these cleanups would be better spent funding prevention efforts such as improved recycling and waste management. In addition to this, there is a need for strong government leadership and intervention when it comes to plastic waste to develop a more permanent solution. Leading bodies need to support the development of viable alternatives to petroleum-based plastics, fund innovation, raise consumer awareness, and standardize plastics and their labelling to improve recycling rates.69 It would also be pertinent to provide the appropriate incentives for industry to use recycled plastics over virgin ones.
This integrative approach calls for a more complete understanding on the effect of plastic pollution on aquatic life and marine ecosystems. Plastic waste must be reduced at the source to prevent leakage, which requires innovation in recycling system management, as well as end-of-life treatments for both biodegradable and non-biodegradable plastics. In addition to this, policies should be set into place to reduce and control the negative aspects of plastic production, control their usage, and improve waste management. It is critical that our plastic dependency is reduced through efforts to ban or limit the use of disposable plastics through governmental policies, as is already being adopted in many countries. Key plastic manufacturers must also be held more responsible for the handling, collection, and reuse of their plastic products after use, as well as funding development of alternatives to non-degradable plastics. One option would be to create programs where producers of single-use plastics are responsible to take back their materials and find appropriate waste management strategies. Waste management and recycling infrastructure must be improved, especially in developing countries where the issue is greatest, through the establishment of an international aid program. The demand for renewable plastics could be increased through governmental taxes or fees on petroleum-based pollution plastics. Addressing the ocean plastic problem is a global effort, and one that must be addressed immediately to protect not only marine ecosystems, but the whole planet.
Conflicts of interest
There are no conflicts of interest.
Acknowledgements
The authors are thankful to: (i) the Ontario Ministry of Economic Development, Job Creation and Trade, Canada – Ontario Research Fund, Research Excellence Program Round 9 (ORF-RE09-078) Canada – (Project No. 053970 and 054345); (ii) the Ontario Ministry of Agriculture, Food and Rural Affairs (OMAFRA), University of Guelph, Bioeconomy Industrial Uses Research Program Theme (Project No. 030252, 030221, 030332 and 030485); (iii) the Natural Sciences and Engineering Research Council (NSERC), Canada Discovery Grants (Project No. 400320 and 401111); (iv) the NSERC, Canada Research Chair (CRC) program (Project No. 460788) and (v) the Agriculture and Agri-Food Canada (AAFC) and Competitive Green Technologies, Canada through AgSci Cluster Program (Project No. 054712) for their financial support to carry out this research.
References
- J. R. Jambeck, R. Geyer, C. Wilcox, T. R. Siegler, M. Perryman, A. Andrady, R. Narayan and K. L. Law, Science, 2015, 347, 768–771 CrossRef CAS PubMed.
- H. Ritchie and M. Roser, Plastic pollution, Our World in Data, https://ourworldindata.org/plastic-pollution, accessed January 2021 Search PubMed.
- E. McKinley, T. Acott and K. L. Yates, Environ. Sci. Policy, 2020, 108, 85–92 CrossRef.
- The Ocean CleanUp, Oceans: Cleaning up the garbage patches 2020, https://theoceancleanup.com/oceans/, accessed December 14, 2020 Search PubMed.
- L. Lebreton, B. Slat, F. Ferrari, B. Sainte-Rose, J. Aitken, R. Marthouse, S. Hajbane, S. Cunsolo, A. Schwarz, A. Levivier, K. Noble, P. Debeljak, H. Maral, R. Schoeneich-Argent, R. Brambini and J. Reisser, Sci. Rep., 2018, 8, 4666 CrossRef CAS PubMed.
- Ellen Macarthur Foundation, Circular Economy System Diagram, https://www.ellenmacarthurfoundation.org/circular-economy/concept/infographic, accessed January 2021 Search PubMed.
- I. Farina, N. Singh, F. Colangelo, R. Luciano, G. Bonazzi and F. Fraternali, Materials, 2019, 12, 3955 CrossRef CAS PubMed.
- K. Min, J. D. Cuiffi and R. T. Mathers, Nat. Commun., 2020, 11, 727 CrossRef CAS PubMed.
- I. C. Kim, K. H. Kwon and W. N. Kim, J. Appl. Polym. Sci., 2019, 136, 47197 CrossRef.
- K. Vones, D. Allan, I. Lambert and S. Vettese, Mater. Today Commun., 2018, 16, 56–59 CrossRef CAS.
- J. Cristobal Garcia, M. Vila, M. Giavini, C. Torres de Matos and S. Manfredi, Prevention of waste in the circular economy: Analysis of strategies and identification of sustainable targets. EUR 28422, Publications Office of the European Union, JRC105415 Luxembourg, 2016 Search PubMed.
- 4Ocean, Our Impact: Cleaning the ocean, rivers, and coastlines, one pound at a time, 2020, https://www.4ocean.com/pages/our-impact, accessed December 14, 2020 Search PubMed.
- D. Golden, J. Fitzimons, T. Doyle and D. Hayes, presented in part at the OCEANS 2019 MTS/IEEE SEATTLE, Seattle, WA, USA, 27–31 Oct. 2019 Search PubMed.
- A. Van Giezen and B. Wiegmans, Transp. Res. Interdiscip. Perspect., 2020, 5, 100115 Search PubMed.
- S. Patil, A. Bafana, P. K. Naoghare, K. Krishnamurthi and S. Sivanesan, Environ. Sci. Pollut. Res., 2021, 28, 4951–4974 CrossRef CAS PubMed.
- A. Chamas, H. Moon, J. Zheng, Y. Qiu, T. Tabassum, J. H. Jang, M. Abu-Omar, S. L. Scott and S. Suh, ACS Sustainable Chem. Eng., 2020, 8, 3494–3511 CrossRef CAS.
- S. Iwasaki, A. Isobe, S. i. Kako, K. Uchida and T. Tokai, Mar. Pollut. Bull., 2017, 121, 85–96 CrossRef CAS PubMed.
- W. C. Li, H. F. Tse and L. Fok, Sci. Total Environ., 2016, 566–567, 333–349 CrossRef CAS PubMed.
- T. Nguyen, Ghost fishing gear: A major source of marine plastic pollution. HillNotes – Libr. Parliam, https://hillnotes.ca/2020/01/30/ghost-fishing-gear-a-major-source-of-marine-plastic-pollution/, accessed December 14, 2020 Search PubMed.
- R. Skvorčinskienė, N. Striūgas, R. Navakas, R. Paulauskas, K. Zakarauskas and L. Vorotinskienė, Waste Biomass Valorization, 2019, 10, 3735–3744 CrossRef.
- F. M. Windsor, I. Durance, A. A. Horton, R. C. Thompson, C. R. Tyler and S. J. Ormerod, Glob. Change Biol., 2019, 25, 1207–1221 CrossRef PubMed.
- A. M. Scheld, D. M. Bilkovic and K. J. Havens, Sci. Rep., 2016, 6, 19671 CrossRef CAS PubMed.
- R. Geyer, J. R. Jambeck and K. L. Law, Sci. Adv., 2017, 3, e1700782 CrossRef PubMed.
- Ocean Conservancy, International Coastal Cleanup 2019 Report, https://oceanconservancy.org/wp-content/uploads/2019/09/Final-2019-ICC-Report.pdf, accessed January 2021 Search PubMed.
- A. Harper, Reinf. Plast., 2019, 63, 202–205 CrossRef.
- M. R. Gregory, Philos. Trans. R. Soc., B, 2009, 364, 2013–2025 CrossRef PubMed.
- N. J. Beaumont, M. Aanesen, M. C. Austen, T. Börger, J. R. Clark, M. Cole, T. Hooper, P. K. Lindeque, C. Pascoe and K. J. Wyles, Mar. Pollut. Bull., 2019, 142, 189–195 CrossRef CAS PubMed.
- L. Lebreton and A. Andrady, Palgrave Commun., 2019, 5, 6 CrossRef.
- D. Ray, Plastic waste in construction – is the sector doing enough?, https://www.building.co.uk/focus/plastic-waste-in-construction-is-the-sector-doing-enough/5098139.article, accessed December 14, 2020 Search PubMed.
- K. L. Law and R. C. Thompson, Science, 2014, 345, 144–145 CrossRef CAS PubMed.
- M. Cole, P. Lindeque, C. Halsband and T. S. Galloway, Mar. Pollut. Bull., 2011, 62, 2588–2597 CrossRef CAS PubMed.
- J. Boucher and D. Friot, Primary microplastics in the oceans: a global evaluation of sources, IUCN Gland, Switzerland, 2017 Search PubMed.
- P. K. Cheung and L. Fok, Mar. Pollut. Bull., 2016, 109, 582–585 CrossRef CAS PubMed.
- M. Siegfried, A. A. Koelmans, E. Besseling and C. Kroeze, Water Res., 2017, 127, 249–257 CrossRef CAS PubMed.
- V. Aj, L. Depoorter, R. Dröge, J. Kuenen and E. Devalk, Emission of microplastics and potential mitigation measures: Abrasive cleaning agents, paints and tyre wear, RIVM report, 2016 Search PubMed.
- D. Xanthos and T. R. Walker, Mar. Pollut. Bull., 2017, 118, 17–26 CrossRef CAS PubMed.
- J. van Wijnen, A. M. J. Ragas and C. Kroeze, Sci. Total Environ., 2019, 673, 392–401 CrossRef CAS PubMed.
- S. Lambert and M. Wagner, Chemosphere, 2016, 145, 265–268 CrossRef CAS PubMed.
- L. Zhu, S. Zhao, T. B. Bittar, A. Stubbins and D. Li, J. Hazard. Mater., 2020, 383, 121065 CrossRef CAS PubMed.
- Y. Ogata, H. Takada, K. Mizukawa, H. Hirai, S. Iwasa, S. Endo, Y. Mato, M. Saha, K. Okuda, A. Nakashima, M. Murakami, N. Zurcher, R. Booyatumanondo, M. P. Zakaria, L. Q. Dung, M. Gordon, C. Miguez, S. Suzuki, C. Moore, H. K. Karapanagioti, S. Weerts, T. McClurg, E. Burres, W. Smith, M. V. Velkenburg, J. S. Lang, R. C. Lang, D. Laursen, B. Danner, N. Stewardson and R. C. Thompson, Mar. Pollut. Bull., 2009, 58, 1437–1446 CrossRef CAS PubMed.
- D. K. A. Barnes, F. Galgani, R. C. Thompson and M. Barlaz, Philos. Trans. R. Soc., B, 2009, 364, 1985–1998 CrossRef CAS PubMed.
- J. S. Woods, G. Rødder and F. Verones, Ecol. Indic., 2019, 99, 61–66 CrossRef.
- J. B. Lamb, B. L. Willis, E. A. Fiorenza, C. S. Couch, R. Howard, D. N. Rader, J. D. True, L. A. Kelly, A. Ahmad, J. Jompa and C. D. Harvell, Science, 2018, 359, 460–462 CrossRef CAS PubMed.
- W. Wang, H. Gao, S. Jin, R. Li and G. Na, Ecotoxicol. Environ. Saf., 2019, 173, 110–117 CrossRef CAS PubMed.
- L. Seuront, Biol. Lett., 2018, 14, 20180453 CrossRef CAS PubMed.
- F. Gallo, C. Fossi, R. Weber, D. Santillo, J. Sousa, I. Ingram, A. Nadal and D. Romano, Environ. Sci. Eur., 2018, 30, 13 CrossRef PubMed.
- W. de Wit and N. Bigaud, No plastic in nature: Assessing plastic ingestion from nature to people, World Wildlife Fund, Gland, Switzerland, 2019 Search PubMed.
- K. D. Cox, G. A. Covernton, H. L. Davies, J. F. Dower, F. Juanes and S. E. Dudas, Environ. Sci. Technol., 2019, 53, 7068–7074 CrossRef CAS PubMed.
- M. Rani, W. J. Shim, G. M. Han, M. Jang, Y. K. Song and S. H. Hong, Sci. Total Environ., 2017, 579, 745–754 CrossRef CAS PubMed.
- J. M. Brausch and G. M. Rand, Chemosphere, 2011, 82, 1518–1532 CrossRef CAS PubMed.
- F. Liu, K. B. Olesen, A. R. Borregaard and J. Vollertsen, Sci. Total Environ., 2019, 671, 992–1000 CrossRef CAS.
- Featured story: Stormwater runoff prevent chemicals, garbage and other debris from winding up on the local beach, 2019, https://www3.epa.gov/region9/water/npdes/stormwater-feature.html, accessed December 14, 2020 Search PubMed.
- Nonpoint Source Runoff Pollution from Roads, Highways and Bridges, https://www.epa.gov/nps/nonpoint-source-roads-highways-and-bridges, accessed December 2020 Search PubMed.
- S. Mishra, C. c. Rath and A. P. Das, Mar. Pollut. Bull., 2019, 140, 188–197 CrossRef CAS PubMed.
- F. Belzagui, C. Gutiérrez-Bouzán, A. Álvarez-Sánchez and M. Vilaseca, Environ. Pollut., 2020, 265, 114889 CrossRef CAS PubMed.
- UN-Water, Water Quality and Wastewater. United Nations, Water Quality and Wastewater, https://www.unwater.org/water-facts/quality-and-wastewater-2/, accessed December 14, 2020 Search PubMed.
- S. Freeman, A. M. Booth, I. Sabbah, R. Tiller, J. Dierking, K. Klun, A. Rotter, E. Ben-David, J. Javidpour and D. L. Angel, J. Environ. Manage., 2020, 266, 110642 CrossRef CAS PubMed.
- P. L. Ngo, B. K. Pramanik, K. Shah and R. Roychand, Environ. Pollut., 2019, 255, 113326 CrossRef CAS PubMed.
- O. S. Ogunola, O. A. Onada and A. E. Falaye, Environ. Sci. Pollut. Res., 2018, 25, 9293–9310 CrossRef CAS PubMed.
- S. Rigby and P. Science, Microplastics: Laundry filters ‘dramatically’ reduce fibres, https://www.sciencefocus.com/news/microplastics-laundry-filters-dramatically-reduce-fibres/, accessed March 30, 2021 Search PubMed.
- I. E. Napper, A. C. Barrett and R. C. Thompson, Sci. Total Environ., 2020, 738, 140412 CrossRef CAS PubMed.
- J. G. B. Derraik, Mar. Pollut. Bull., 2002, 44, 842–852 CrossRef CAS PubMed.
- The new plastics economy: Rethinking the future of plastics, Ellen MacArthur Foundation, 2016, https://www.ellenmacarthurfoundation.org/publications/the-new-plastics-economy-rethinking-the-future-of-plastics Search PubMed.
- W. d'Ambrières, Field Actions Science Reports. The Journal of Field Actions, 2019, pp. 12–21 Search PubMed.
- O. I. Nkwachukwu, C. H. Chima, A. O. Ikenna and L. Albert, Int. J. Ind. Chem., 2013, 4, 34 CrossRef.
- T. Thiounn and R. C. Smith, J. Polym. Sci., 2020, 58, 1347–1364 CrossRef CAS.
- K. R. Vanapalli, H. B. Sharma, V. P. Ranjan, B. Samal, J. Bhattacharya, B. K. Dubey and S. Goel, Sci. Total Environ., 2021, 750, 141514 CrossRef CAS PubMed.
- E. Foschi and A. Bonoli, Adm. Sci., 2019, 9, 18 CrossRef.
- T. R. Walker and D. Xanthos, Resour., Conserv. Recycl., 2018, 133, 99–100 CrossRef.
- M. Teraishi, Japan Environment Quarterly, 2019, vol. 20, p. 3 Search PubMed.
- R. E. J. Schnurr, V. Alboiu, M. Chaudhary, R. A. Corbett, M. E. Quanz, K. Sankar, H. S. Srain, V. Thavarajah, D. Xanthos and T. R. Walker, Mar. Pollut. Bull., 2018, 137, 157–171 CrossRef CAS PubMed.
- Introducing a World-First: A Coke Bottle Made with Plastic from the Sea. Coca-Cola Co, https://www.coca-cola.eu/news/marine-bottle/, accessed December 14, 2020 Search PubMed.
- D. Howarth, Adidas launches trainers made from ocean plastic with Parley for the Oceans, https://www.dezeen.com/2016/06/08/adidas-trainers-parley-for-the-ocean-plastic-design-recycling/, accessed December 14, 2020 Search PubMed.
- D. Baier, T. M. Rausch and T. F. Wagner, Sustainability, 2020, 12, 2788 CrossRef.
- P. Kavilanz, The company turning 4 billion plastic bottles into clothes, https://money.cnn.com/2016/05/16/technology/plastic-bottles-fabric-repreve/index.html, accessed December 14, 2020 Search PubMed.
- M. Murray, Made on MVY: Sea plastic differently with Norton Point sunglasses, MVTimes, https://www.mvtimes.com/2016/06/08/made-mvy-sea-plastic-differently-norton-point-sunglasses/, accessed December 14, 2020 Search PubMed.
- Method – Beyond the Bottle, https://methodhome.com/beyond-the-bottle/ocean-plastic/, accessed December 14, 2020 Search PubMed.
- D. Zhang and Q. Sun, J. Appl. Polym. Sci., 1996, 62, 367–373 CrossRef CAS.
- V. G. Pol and P. Thiyagarajan, J. Environ. Monit., 2010, 12, 455–459 RSC.
- A. Panahi, Z. Wei, G. Song and Y. A. Levendis, Ind. Eng. Chem. Res., 2019, 58, 3009–3023 CrossRef CAS.
- Y. Lian, M. Ni, Z. Huang, R. Chen, L. Zhou, W. Utetiwabo and W. Yang, Chem. Eng. J., 2019, 366, 313–320 CrossRef CAS.
- S. Zhang, J. Li, T. Wen, J. Xu and X. Wang, RSC Adv., 2013, 3, 2754–2764 RSC.
- W. Baxter, M. Aurisicchio and P. Childs, J. Ind. Ecol., 2017, 21, 507–516 CrossRef.
- L. Magnier, R. Mugge and J. Schoormans, J. Cleaner Prod., 2019, 215, 84–98 CrossRef.
- A. Rahimi and J. M. García, Nat. Rev. Chem., 2017, 1, 0046 CrossRef.
- Editorial, Plastic upcycling, Nat. Catal., 2019, 2, 945–946 CrossRef.
- A. R. Patel, P. C. Heussen, E. Dorst, J. Hazekamp and K. P. Velikov, Food Chem., 2013, 141, 1466–1471 CrossRef CAS PubMed.
- J. C. Prata, A. L. P. Silva, J. P. da Costa, C. Mouneyrac, T. R. Walker, A. C. Duarte and T. Rocha-Santos, Int. J. Environ. Res. Public Health, 2019, 16, 2411–2430 CrossRef PubMed.
- HP Inc. and IKEA Join Initiative to Develop First Global Network of Ocean-Bound Plastics Supply Chains, 2018, https://www.csrwire.com/press_releases/41472-HP-Inc-and-IKEA-Join-Initiative-to-Develop-First-Global-Network-of-Ocean-Bound-Plastics-Supply-Chains, accessed December 14, 2020 Search PubMed.
- Trash as Value: Turning Ocean Waste into Viable Products, Plastic Technology, 2018, https://www.ptonline.com/articles/trash-as-value-turning-ocean-waste-into-viable-products, accessed December 14, 2020 Search PubMed.
- Social Plastic, Plastic Bank, https://plasticbank.com/social-plastic, accessed December 14, 2020 Search PubMed.
- J. Johnson, Dell wants “open source” solution to ocean debris, https://www.plasticsnews.com/article/20180510/NEWS/180519993/dell-wants-open-source-solution-to-ocean-debris, accessed December 14, 2020 Search PubMed.
- F. Esposito, DSM among firms advancing engineering resins at K 2019, Plastic News, https://www.plasticsnews.com/news/dsm-among-firms-advancing-engineering-resins-k-2019, accessed December 14, 2020 Search PubMed.
- R. P. Singh, S. Mishra and A. P. Das, Chemosphere, 2020, 257, 127199 CrossRef CAS PubMed.
- A. Paço, J. Jacinto, J. P. da Costa, P. S. M. Santos, R. Vitorino, A. C. Duarte and T. Rocha-Santos, Crit. Rev. Environ. Sci. Technol., 2019, 49, 410–441 CrossRef.
- V. M. Pathak and Navneet, Bioresour. Bioprocess., 2017, 4, 15 CrossRef.
- M. Cordier and T. Uehara, Sci. Total Environ., 2019, 670, 789–799 CrossRef CAS PubMed.
- T. Narancic and K. E. O'Connor, Microb. Biotechnol., 2017, 10, 1232–1235 CrossRef PubMed.
- S. Muniyasamy, A. Anstey, M. Reddy, M. Misra and A. K. Mohanty, J. Renewable Mater., 2013, 4, 253–272 CrossRef.
- F. Degli Innocenti and T. Breton, ACS Sustainable Chem. Eng., 2020, 8, 9239–9249 CrossRef CAS.
- K. W. Meereboer, M. Misra and A. K. Mohanty, Green Chem., 2020, 22, 5519–5558 RSC.
- L. P. Amaro, M. A. Abdelwahab, A. Morelli, F. Chiellini and E. Chiellini, in Recent advances in Biotechnology, ed. M. Koller, Bentham Science, 2016, vol. 2, ch. 1, pp. 3–74 Search PubMed.
- F. M. Lamberti, L. A. Román-Ramírez and J. Wood, J. Polym. Environ., 2020, 28, 2551–2571 CrossRef CAS.
- Y. Picó and D. Barceló, ACS Omega, 2019, 4, 6709–6719 CrossRef PubMed.
- World Economic Forum, The new plastics economy: Catalysing action, Ellen MacArthur Foundation and McKinsey & Company, 2017 Search PubMed.
- J. Payne, P. McKeown and M. D. Jones, Polym. Degrad. Stab., 2019, 165, 170–181 CrossRef CAS.
- J. Zheng and S. Suh, Nat. Clim. Change, 2019, 9, 374–378 CrossRef.
- A. K. Mohanty, S. Vivekanandhan, J.-M. Pin and M. Misra, Science, 2018, 362, 536–542 CrossRef CAS PubMed.
Footnote |
† On leave from Department of Chemistry, Faculty of Science, Tanta University, Tanta 31527, Egypt. |
|
This journal is © The Royal Society of Chemistry 2021 |
Click here to see how this site uses Cookies. View our privacy policy here.