DOI:
10.1039/D0RA09848E
(Review Article)
RSC Adv., 2021,
11, 13138-13151
Intermolecular difunctionalization of alkenes: synthesis of β-hydroxy sulfides
Received
19th November 2020
, Accepted 23rd March 2021
First published on 7th April 2021
Abstract
Direct difunctionalization of carbon–carbon double bonds is one of the most powerful tools available for concomitant introduction of two functional groups into olefinic substrates. In this context, vicinal hydroxysulfenylation of unactivated alkenes has emerged as a novel and straightforward strategy for the fabrication of β-hydroxy sulfides, which are extremely valuable starting materials in constructing various natural products, pharmaceuticals, and fine chemicals. The aim of this review is to summarize the most representative and important reports on the preparation of β-hydroxy sulfides through intermolecular hydroxysulfenylation of the corresponding alkenes with special emphasis on the mechanistic features of the reactions.
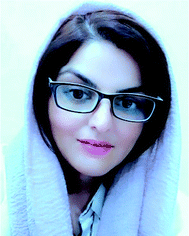 Bayan Azizi | Bayan Azizi was born in Iraq, in 1986. She received her bachelor degree in Applied Chemistry from Razi University, Iran in 2008. Also, she has received her master degree in Physical Chemistry from Kurdistan University in 2012. After graduation, she has worked at the Quality Control Laboratory in Jahad Daneshgahi (Oslub Sanat Company), Iran and Payam Noor University, Kermanshah in 2013. She was been lecturer at the Technical College of Applied Science, Sulaimani Polytechnic University, Kurdistan Region of Iraq from 2014 to 2018. Currently she is a lecturer at the department of Medical Laboratory Science, University of Human Development, Kurdistan Region of Iraq where she has been since 2018. Her research interests include nano-technology, quantum chemistry, physical chemistry, and clinical chemistry. |
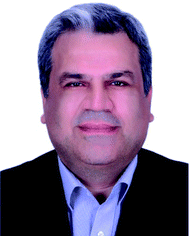 Mohammad Reza Poor Heravi | Mohammad Reza Poor Heravi was born in Seiyahkal-Lahijan, Guilan, Iran in 1965. He received a B.Sc. degree in pure Chemistry from Tabriz University, Iran, in 1987, and a M.Sc. degree in Organic Chemistry from Tabriz University, Iran in 1990 with Professor A. Shahrisa, and a Ph.D. degree from Isfahan University, Iran, with Professor H. Loghmanim. In 2006 he was a visiting researcher at Sheffield University, UK, with Professor Richard F. W. Jackson. He became a sciences faculty member of Payame Noor University in 1990, and associate Professor in 2012. His research field includes applications of solvent-free conditions, ionic liquids, fluorination reactions, designing fluorinating reagents and ultrasound irradiation in organic synthesis, and the study of methodology in organic chemistry. |
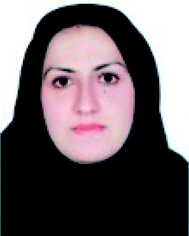 Zinatossadat Hossaini | Zinatossadat Hossaini was born in Tehran, Iran, in 1976. She received her B.S. degree in pure chemistry from the University of Alzahra, Tehran, Iran, and her M.S. degree in organic chemistry from Tarbiat Modares University, Tehran, Iran, in 2003 under the supervision of Prof. I. Yavari. She completed her Ph.D. degree in 2008 under the supervision of Prof. I. Yavari. Now she is working at Islamic Azad University as an associate Professor in organic chemistry. Her research interests include synthesis of organic compounds, synthesis of nanocatalysts and new methodologies in organic synthesis and spectral studies of organic compounds. |
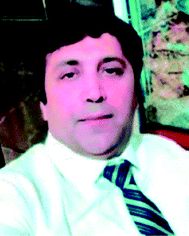 Abdolghaffar Ebadi | Dr. Abdol Ghaffar Ebadi finished his doctoral degree in Environmental Biotechnology (Algology) from Tajik Academy of Sciences. Now he is a researcher in TAS in Tajikistan and faculty member at the Islamic Azad University of Jouybar in Mazandaran. Dr Ebadi has published more than 400 scientific papers in qualified international journals and attended more than 50 international conferences. He has cooperation with many research project teams around world such as in China, Malaysia, and Thailand. His interests are Environmental Biotechnology, Biochemistry, and Gene pathways in phytoremediation processes. |
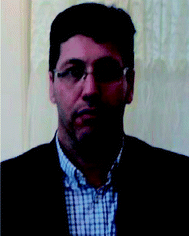 Esmail Vessally | Esmail Vessally was born in Sharabiyan, Sarab, Iran, in 1973. He received his B.S. degree in pure chemistry from the University of Tabriz, Tabriz, Iran, and his M.S. degree in organic chemistry from Tehran University, Tehran, Iran, in 1999 under the supervision of Prof. H. Pirelahi. He completed his Ph.D. degree in 2005 under the supervision of Prof. M. Z. Kassaee. Now he is working at Payame Noor University as Professor in organic chemistry. His research interests include theoretical organic chemistry, new methodologies in organic synthesis and spectral studies of organic compounds. |
1. Introduction
Organosulfur compounds are ubiquitous in natural products, pharmaceuticals, and agrochemicals.1–3 In particular, organic sulfides widely exist in many useful commercialized drugs.4 In this family of organosulfur compounds, β-hydroxy containing sulfides have recently attracted significant attention due to their fascinating biological activities, such as antimicrobial, antitumor, anti-HIV, and anti-inflammatory activities (Scheme 1).4,5 One of the most straightforward synthetic procedure for the preparation of β-hydroxy sulfides is the thiolysis of epoxides with thiols6 or disulfides.7 However, this pathway generally suffers from certain disadvantages, such as low yields, poor regioselectivity, harsh reaction conditions, and the formation of undesirable by-products via rearrangement of epoxides and oxidation of thiols. Thus, the development of a convenient and truly efficient synthetic strategy for the preparation of the titled compounds remains a challenge.
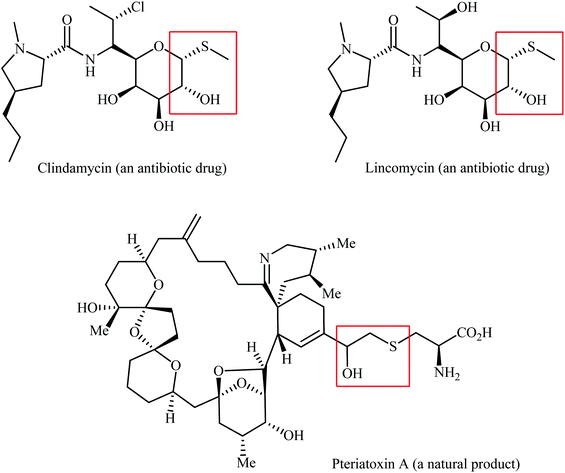 |
| Scheme 1 Bioactive compounds containing a β-hydroxy sulfide unite. | |
Direct difunctionalization of C–C unsaturated bonds is one of the most powerful synthetic methodologies toward polyfunctionalized molecules in a very simple manner.8 In this context, 1,2-hydroxysulfenylation of alkenes with various sulfenyl sources provides an excellent route to the synthesis of β-hydroxy sulfides in one step (Fig. 1). High atom- and step-economy efficiency, high functional group tolerance, good regioselectivity, and the use of inexpensive easily available starting materials are the main advantages motioned for this page of β-hydroxy sulfide synthesis. Recently, Kinfe and co-workers highlighted this synthetic strategy in their interesting review paper entitled “β-hydroxy sulfides and their syntheses”.9 However, several important examples were omitted and generally the explanation of mechanistic pathways of the reactions were ignored. In connection with our recent works on organosulfur chemistry10 and modern organic synthesis,11 herein we will attempt to provide a comprehensive overview of the synthesis of β-hydroxy sulfides through intermolecular difunctionalization of the respective alkenes with particular emphasize on the mechanistic aspect of reactions which may allow possible new insights into catalyst improvement.
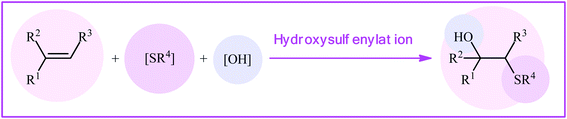 |
| Fig. 1 Direct one-pot hydroxysulfenylation of alkenes. | |
2. Metal-catalyzed/mediated reactions
The fabrication of β-hydroxy sulfides through metal-mediated direct hydroxysulfenylation of alkenes was accomplished first in 1978 by Trost et al.12 They showed that treatment of aliphatic alkenes 1 with aromatic disulfides 2 in the presence of Pb(OAc)4 as an oxidant and CF3CO2H as the source of the hydroxyl oxygen in DCM furnished trifluoroacetoxy sulfide intermediate I that after hydrolysis in the work-up converted to the corresponding β-hydroxy sulfides 3 in moderate to almost quantitative yields (Scheme 2). The reaction is notable in that both terminal and internal alkenes were tolerated. However, the regioselectivity was poor and in the cases of unsymmetrical alkenes a mixture of two possible isomers were obtained. It is worthwhile to note that under the identical conditions, alkenes bearing a carboxylic acid group underwent sulfenyllactonization to give sulfenylated lactones with good to excellent yields. Eight years later, Mellor's research team improved the efficiency of this process in the terms of regioselectivity and product yields by replacing of Pb(OAc)4 with Mn(OAc)3 and using allylic esters and amides as the substrates.13 Afterwards, El-Samii successfully applied this procedure in the hydroxysulfenylation of a small series of butadiene derivatives with diphenyldisulfane and di-p-tolyldisulfane.14 Of note, Pb(OAc)4 was also found to be effective oxidant for this transformation, however Cu(OAc)2 and Fe(OAc)2 proved to be completely ineffective.
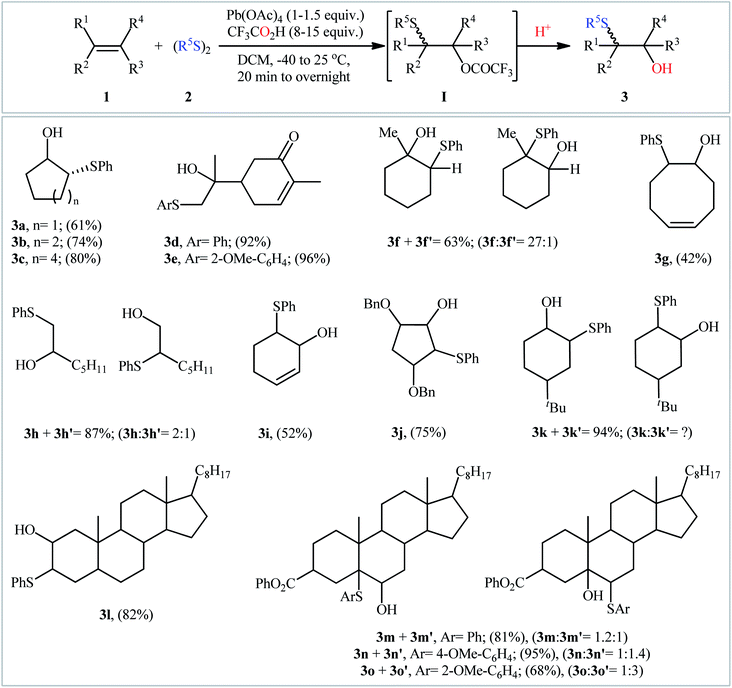 |
| Scheme 2 Pb-catalyzed three component reaction between alkenes 1, disulfides 2, and CF3CO2H. | |
In 2008, Movassagh and Navidi provided a further example of β-hydroxy sulfide derivatives 6 synthesis from the corresponding styrenes 4 and aromatic disulfides 5 assisted by Zn/AlCl3 system in MeCN/H2O (4
:
1) under an oxygen atmosphere (Scheme 3).15 Here, both electron-donating and electron-withdrawing substituents on the styrenes and the disulfides were compatible with the reaction condition and afforded the target products in good yields with high regioselectivities. However, the procedure was unsuccessful for aliphatic alkenes. It should be mentioned that the presence of oxygen is crucial for the success of this reaction since in its absence, the addition of hydrogen and thiolate anion across the double bond ensues, yielding the undesired sulfides.
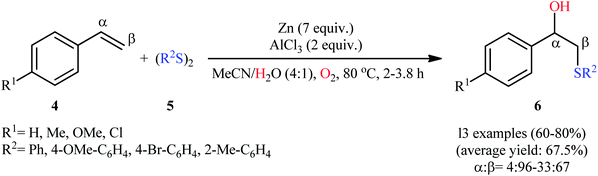 |
| Scheme 3 Movassagh's synthesis of β-hydroxy sulfides 6. | |
Following these works, Li's research group presented an efficient Cu(II)-catalyzed hydroxysulfenylation of a wide range of alkenes 7 and aromatic thiols 8 into the corresponding β-hydroxy sulfides 9.16 The reaction proceeded under an oxygen atmosphere using 5 mol% of Cu(OAc)2 as catalyst and 2 equiv. of PhCO2H as additive in DCE at 50 °C, tolerated a variety of common functional groups (e.g., fluoro, bromo, nitro, hydroxyl, methoxy, ketone, ester, and amine functionalities) and provided the expected products in moderate to excellent yields and outstanding regioselectivities, in which SR group predominantly added to the less hindered carbon atom of the C
C bond (Scheme 4). Noteworthy, this synthetic procedure was also successfully applied in the high yielding preparation of bicalutamide, an anticancer drug. It is worthwhile to note that other metal catalysts, such as FeCl2, FeCl3, Fe(OAc)2, Mn(OAc)2, CoCl2, CuCl, CuCl2, and CuBr were also found to promote this difunctionalization reaction; albeit at lower efficiencies. Based on a series of control experiments, the authors suggested that the reaction starts with the formation of thiyl radical II via the oxidation of thiol 8 with O2, which after addition to alkene 7 leads to the formation of carbon-centered radical III. Next, this intermediate undergoes reaction with the in situ generated peroxy-copper species IV to afford the CuII/IIIOOR′ species V that, after protodemetalation under acidic conditions forms hydroperoxide intermediate VI. Finally, reduction of VI by the copper catalyst leads to the final product 9 (Scheme 5).
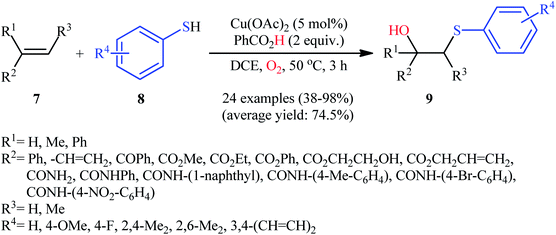 |
| Scheme 4 Cu-catalyzed hydroxysulfenylation of alkenes 7 with aromatic thiols 8 in the presence of PhCO2H. | |
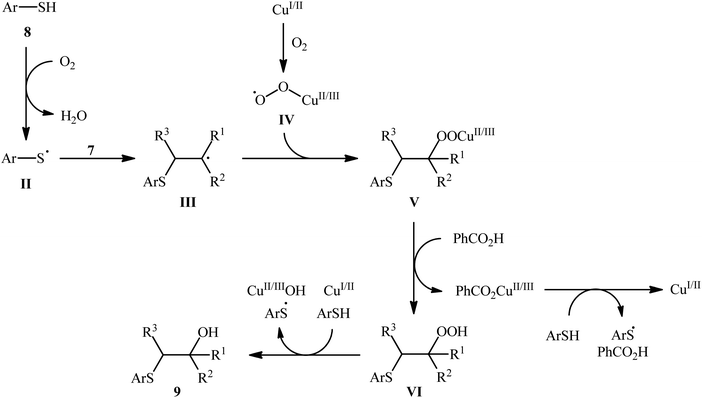 |
| Scheme 5 Plausible reaction mechanism for the formation of β-hydroxy sulfides 9. | |
3. Halogen-catalyzed/mediated reactions
In 2017, Peddinti's research team described an interesting metal-free molecular iodine-catalyzed direct hydroxysulfenylation of styrene derivatives 10 with thiophenols 11 using DMSO as the oxygen source as well as the solvent.17 The reaction proceeded slowly at 60 °C under additive-free conditions, tolerated both electron-rich and electron-poor substrates, and provided the desired β-hydroxy sulfides 12 in moderate to excellent yields (Scheme 6a). Importantly, the reaction showed outstanding regioselectivity, in which SAr group predominantly added to the terminal carbon atom of the double bond. The radical trapping experiments with 2,2,6,6-tetramethyl-1-piperidinyloxy (TEMPO) pointed toward the radical pathway of the reaction. The plausible mechanistic cycle proposed by the authors for this difunctionalization reaction is illustrated in Scheme 6b. Initially, the nucleophilic thiophenol 11 attacks the electrophilic iodine centre leading to the generation of hypoiodothioite intermediate VII. Next, this intermediate liberates thiyl radical (ArS˙); which reacts with electron-rich styrene 10 to deliver carbon-centered radical VIII and iodine free radical (I˙). Subsequently, with the aim of iodine free radical, DMSO attacks at benzylic position of intermediate VIII to yield intermediate IX. Finally, the attack of in situ generated HI on IX affords the expected β-hydroxy sulfides 12 and regenerates molecular iodine.
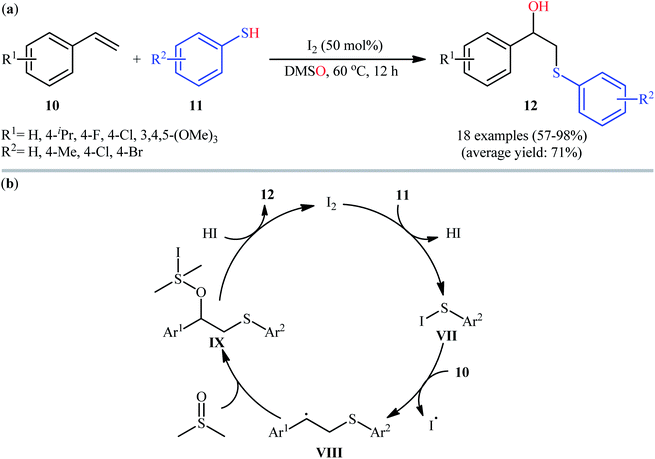 |
| Scheme 6 (a) Three component reaction between styrenes 10, thiophenols 11 and DMSO using I2 as the catalyst; (b) mechanistic insights on I2-catalyzed hydroxysulfenylation of styrenes 10 with thiophenols 11 and DMSO. | |
In this context, Lin and Yan along with their co-workers developed an interesting HBr-catalyzed regioselective hydroxysulfenylation of styrenes 13 employing thiosulfates 14 as thiol sources.18 Using 50 mol% of HBr as catalyst and 2 equiv. of H2O2 as oxidant, a library of terminal styrenes 13 underwent regioselective hydroxysulfenylation with S-aryl thiosulfates 14 to afford the corresponding β-hydroxy sulfides 15 in good to high yields within 30 min (Scheme 7). However, when aliphatic alkenes and S-alkyl thiosulfates were employed under the identical conditions, only trace amounts of target products were obtained. Noteworthy, the optimized condition was also effective for the site-selective sulfenylation of 4-hydroxycoumarins at the C3-position.
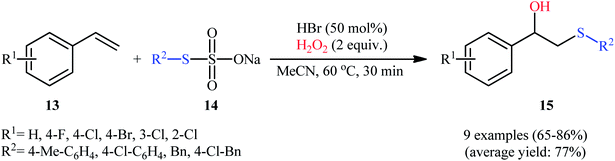 |
| Scheme 7 HBr-catalyzed hydroxysulfenylation of styrenes 13 employing thiosulfates 14 as thiol sources. | |
Recently, Li and Chen described a unique NH4I-promoted hydroxymethylthiolation of various terminal alkenes 16 using DMSO as an easy-handling methanesulfenyl source and water as an oxygen source.19 The reaction was carried out in a 1
:
1 mixture of DMSO and H2O under additive-free conditions and afforded the desired hydroxysulfenylation products 17 in moderate to high yields and outstanding regioselectivities (Scheme 8a). Although the reaction tolerated various functional groups, the need for elevated temperature (130 °C) may limit its range of application. It is worthwhile to note that when the reaction was carried out in dry DMSO, bis-methylsulfanes were obtained as the sole products. The mechanism of this reaction probably involves the initial formation of the iodine radical (I˙) and methanthiol X through a series of transformations with NH4I and DMSO at high temperature, which after reaction with each other leads to the radical XI and HI. Subsequently, regioselective addition of this intermediate to the terminal C
C double bond of alkene 16 gives carbon-centered radical XII which undergoes further single-electron oxidation to form a β-MeS-substituted carbocation XIII. Next, intramolecular cyclization of carbocation intermediate XIII furnishes thiiranium ion XVI. Finally, the nucleophilic attack of H2O on the thiiranium ion XVI produces the observed hydroxysulfenylation product 17 (Scheme 8b).
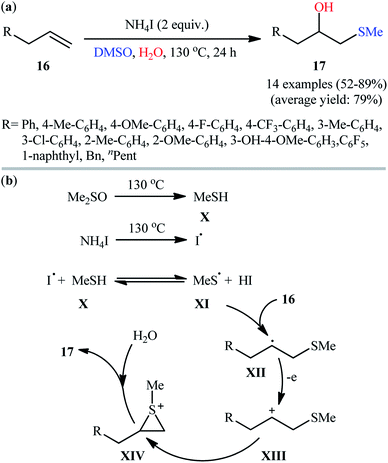 |
| Scheme 8 (a) NH4I-promoted hydroxymethylthiolation of terminal alkenes 16 with DMSO and water; (b) proposed mechanism for the formation of β-hydroxy sulfides 17. | |
Very recently, Ni and Niu along with their colleagues extended the substrate scope of this chemistry to disulfides.20 Thus, a large number of β-hydroxy sulfides 20 were selectively synthesized in moderate to high yields via hydroxysulfenylation of the corresponding alkenes 18 with symmetrical aromatic disulfides 19 employing molecular iodine as the catalyst and air as the oxidant (Scheme 9). Although various terminal and internal aromatic and aliphatic alkenes bearing both electron-donating and electron-withdrawing groups were well tolerated under the reaction conditions, the scope of disulfides was limited to the electron-rich and slightly electron-poor aromatic disulfides.
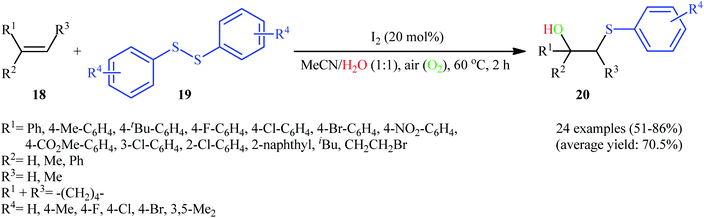 |
| Scheme 9 I2-catalyzed aerobic hydroxysulfenylation of alkenes 18 with disulfides 19 developed by Ni and Niu. | |
4. Acid-mediated reactions
In 2008, Naito and colleagues demonstrated the usefulness of triethylborane (Et3B) as a Lewis acid promoter for the direct hydroxysulfenylation of olefinic double bond.21 They showed that the three-component reaction between α,β-unsaturated imines 21, thiols 22, and oxygen in the presence of a catalytic amount of Et3B in DCM at room temperature afforded the corresponding β-hydroxy sulfides 23 in relatively poor to good yields and excellent regioselectivities (Scheme 10). The relative anti- and syn-geometric configurations of products were determined from NOESY experiments. The results indicated the preferential formation of the anti-isomers. The authors explained the observed anti/syn selectivity by invoking conformer XX, in which the C–S bond is eclipsed by the p orbital of the radical center, because electronic and steric effects (Scheme 11).
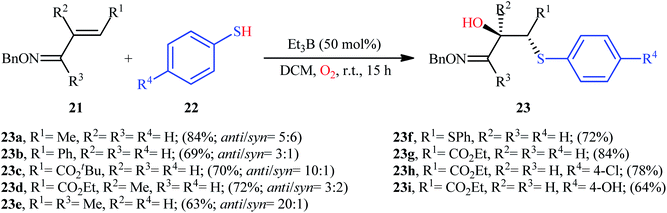 |
| Scheme 10 Selected examples of Et3B-catalyzed hydroxysulfenylation of α,β-unsaturated imines 21, thiols 22, and O2. | |
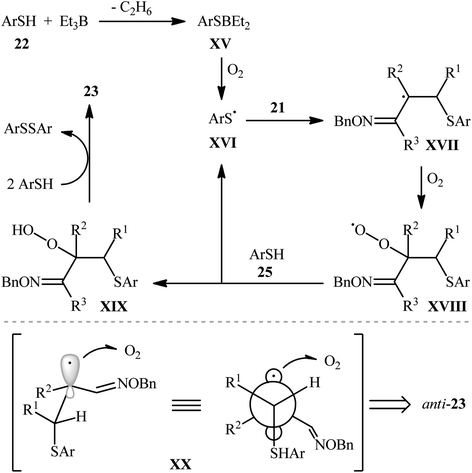 |
| Scheme 11 Proposed mechanism for the reaction in Scheme 10. | |
Eight years later, Shi's research team described an interesting Brønsted acid-promoted regioselective hydroxysulfenylation of alkenes with aromatic thiols employing air as oxygen source.22 Using a stoichiometric amount of racemic phosphoric acid 24 as the mediator, a number of styrene derivatives 25 underwent hydroxysulfenylation with ortho-mercaptobenzyl alcohols 26 to afford the corresponding β-hydroxy sulfides 27 in moderate to high yields within 12 h (Scheme 12). In addition, 1H-indene as a cyclic styrene analogue was also successfully employed under the optimized conditions in the reaction with 2-(2-mercaptophenyl)propan-2-ol, offering the desired β-hydroxy sulfide product in a considerable yield of 63%. Interestingly, when thiophenols were subjected to the reactions with styrene under the identical conditions, instead of the expected β-hydroxy sulfide products, the β-hydroxy sulfoxides were obtained as the sole products.
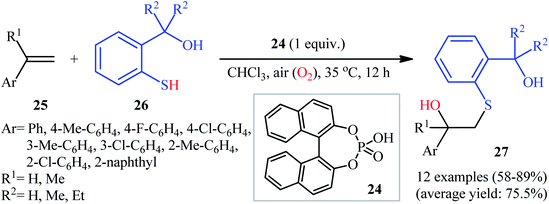 |
| Scheme 12 Brønsted acid-catalyzed aerobic hydroxysulfenylation of styrenes 25 with mercaptobenzyl alcohols 26. | |
Very recently, Duan and Li along with their co-workers studied the metal-catalyzed hydroxysulfenylation of unactivated C
C double bonds with sulfonyl hydrazides as the sulfenyl sources and water as the oxygen source.23 Thus, in the presence of FeBr3/bpy/Na2S2O8 combination as a catalytic system in 1,4-dioxane/H2O (20
:
1) under air atmosphere, hydroxysulfenylation of N-allyl-N-sulfonamides 28 with various aromatic sulfonyl hydrazides 29 furnished the corresponding β-hydroxy sulfides 30 in modestly to high yields, ranging from 35% to 80% (Scheme 13a). In this investigation the authors found some limitations in their methodology when they attempted to use sterically hindered N-allyl-N-sulfonamides and secondary N-allyl-N-sulfonamides as the substrates. Unfortunately, in these cases, no desired products were observed. The results also revealed that the hydroxysulfenylation reaction could not proceed without sulfonyl groups in the substrates. Butane-1-sulfonohydrazide did not work well in the reaction and therefore no other aliphatic sulfonyl hydrazides were examined in the protocol. The authors proposed mechanistic pathway for this difunctionalization reaction is illustrated in Scheme 13b. It consists of the following key steps: (i) reaction of sulfonyl hydrazide 29 with bromine source in the presence of Na2S2O8 to form the sulfenyl bromide intermediate XXI; (ii) electrophilic addition of sulfenyl bromide XXI to alkene 28 to produce thiiranium ion intermediate XXII; and (iii) ring-opening of thiiranium ion XXII with H2O under acidic conditions to produce the desired β-hydroxy sulfides 30.
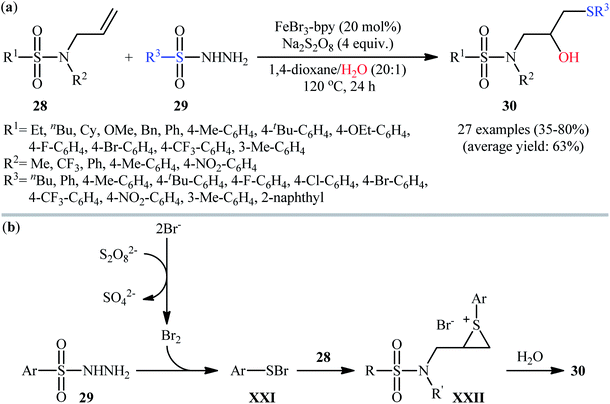 |
| Scheme 13 (a) Fe-catalyzed hydroxysulfenylation of N-allylsulfonamides 28 with sulfonyl hydrazides 29 developed by Duan and Li; (b) mechanistic proposal for the formation of β-hydroxy sulfides 30. | |
5. Catalyst-free reactions
After pioneering works by the groups of Kharasch24 and Beckwith25 on catalyst-free intermolecular hydroxysulfenylation of a small library of alkenes with thiols and dioxygen, the first general report of the direct synthesis of β-hydroxylated sulfides via concomitant introduction of sulfenyl and hydroxyl moieties to the respective alkenes under catalyst- and additive-free conditions was published in 2015 by Lei et al.26 In this investigation, fifteen β-hydroxy sulfides 33 were efficiently prepared through the treatment of corresponding alkenes 31 with electron-rich aromatic thiols 32 under an atmosphere of molecular oxygen as the sole oxidant in DMSO followed by Ph3P workup (Scheme 14). Although the yields and regioselectivities for this synthetic strategy were good, the diastereoselectivities were modest at the best and a mixture of isomers was usually obtained. Of note, the reaction can be enlarged to gram scale with negligible decrease in yield. Interestingly, when the solvent was changed to DCM and the workup step was ignored, β-hydroxy sulfoxides were exclusively obtained instead of β-hydroxy sulfide products.
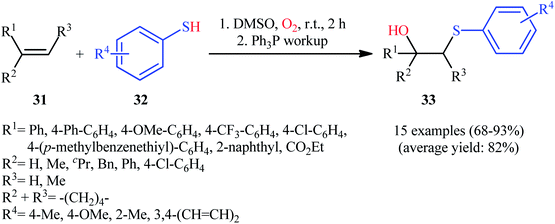 |
| Scheme 14 Catalyst-free intermolecular hydroxysulfenylation of alkenes 31 with electron-rich aromatic thiols 32. | |
In a related study, Huo and colleagues synthesized a library of β-hydroxy sulfide derivatives 36 in moderate to excellent yields, ranging from 46% to 96%, via the reaction of electron-deficient alkenes 34 with various thiophenoles 35 in a binary solvent MeCN/DCE with ratio 5
:
1 under the open air and catalyst-free conditions (Scheme 15).27 Notably, that the identical condition was also successfully used for hydroxysulfenylation of a series of electron-rich alkenes such as styrenes and 1,3-butadienes. The authors also nicely applied their methodology in the gram scale synthesis of bicalutamide.
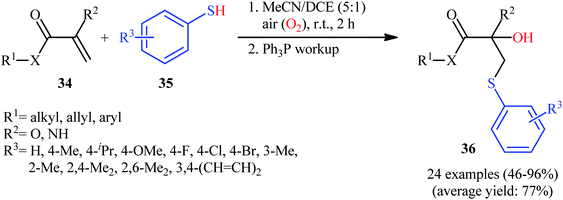 |
| Scheme 15 Huo's synthesis of β-hydroxy sulfide derivatives 36. | |
6. Miscellaneous reactions
In 2006, the group of Rao disclosed that the treatment of terminal alkenes 37 with aromatic thiols 38 in the presence of 1 equiv. of non-toxic β-cyclodextrin (β-CD) in neat water under oxygen atmosphere afforded the corresponding β-hydroxy sulfide derivatives 39 through an anti-Markovnikov addition procedure (Scheme 16a).28 The reaction conditions allowed the use of substrates bearing a series of important functional groups including Cl, Br, OMe, and CO2Me groups. This made possible the further manipulation of the end products. This green protocol is also applicable for the hydroxysulfenylation of internal alkenes. It should be mentioned that β-cyclodextrin can be easily recovered from the reaction mixture and reused for the next reaction runs. As proposed by the authors, β-cyclodextrin catalyzes this hydroxysulfenylation reaction via reversible formation of host–guest complexes by non-covalent bonding as seen in Scheme 16b. Subsequently, Kamal and co-workers reported the synthesis of twenty β-hydroxy sulfides from various terminal and internal alkenes and aromatic thiols using a mixture of ionic liquid [bmim][BF4] and water in the presence of aerial oxygen under neutral conditions.29
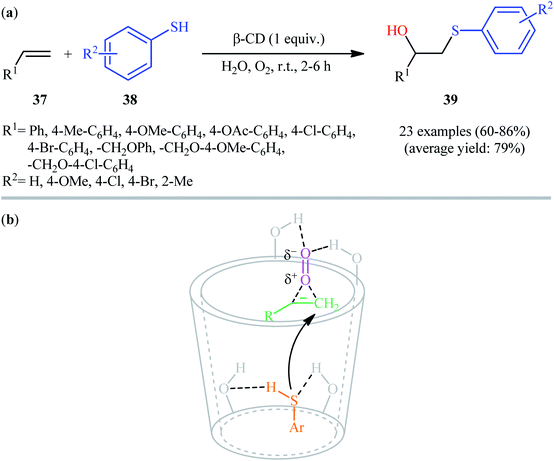 |
| Scheme 16 (a) Synthesis of β-hydroxy sulfides 39 from terminal alkenes 37 and aromatic thiols 38 in the presence of β-CD in neat water; (b) schematic illustration of reaction mechanism for hydroxysulfenylation reactions using β-CD as a mediator. | |
Inspired by these results, in 2017, Feng and co-workers investigated the possibility of synthesizing β-hydroxy sulfide through the auto-oxidative radical hydroxysulfenylation of the respective alkenes in surfactant/H2O system.30 To determine the optimum conditions, they carefully investigated the activities of different surfactant (e.g., SDS, tergitol, brij L4, brij L23, brij O20, brij C20, TPGS-750-M) in the hydroxysulfenylation of 1,1-diphenylethylene with 4-chlorobenzenethiol under oxygen atmosphere, as a model reaction. The optimal system was recognized using 2 wt% brij L4 at room temperature. A variety of terminal styrenes, as well as α-substituted and β-substituted styrenes 40 were reacted well with functionalized aromatic thiols 41 under the standard reaction conditions to provide the expected hydroxysulfenylated products 42 in fair to high yields (Scheme 17). 1,2-Diphenylethene was also tested and gave product but in rather poor yield. However, neither aliphatic alkenes nor aliphatic thiols were examined in this synthetic strategy. It is important to mentioned that the reaction could be easily scaled up to the gram-scale as exemplified by the fubrication of 2-((3,5-dimethylphenyl)thio)-1,1-diphenylethanol on a 0.79 g scale (67%).
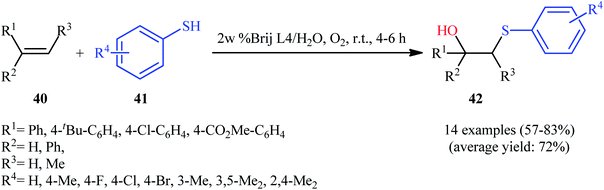 |
| Scheme 17 Feng's synthesis of β-hydroxy sulfides 42. | |
In 2015, Pan and Zou along with their colleagues developed a convenient and mild approach for regioselective synthesis of β-hydroxy sulfides 45 through the tert-butyl hydroperoxide (tBuOOH)-initiated air oxidative radical hydroxysulfenylation of styrenes 43 with arylthiols 44.31 This radical difunctionalization reaction was run in DMF at room temperature under ambient air and provided substituted β-hydroxy sulfides 45 in poor to excellent yields (Scheme 18a); nonetheless the reaction failed completely for heteroaryl thiols, nitro and amino-substituted aryl arylthiols, aliphatic alkenes, and nitro-substituted styrenes. Interestingly, when styrenes bearing an easily removable leaving group at the α-position were used as substrates, β-oxosulfides were obtained as the sole products. In a similar manner, the reaction of styrenes bearing a leaving group at the β-position with arylthiols gave the corresponding β-oxythioacetals. At the same year, Yadav's research team presented the high yielding synthesis of a library of β-hydroxy sulfides 48 via rongalite-mediated hydroxysulfenylation of styrenes 46 with aromatic disulfides 47 employing 1.5 equiv. of K2CO3 as the base in a 4
:
1 mixture of MeCN and H2O at room temperature (Scheme 18b).32 According to the authors proposed mechanism, this conversion is likely to follow the pathway illustrated in Scheme 19. Initially, thermal decomposition of rongalite generates formaldehyde and HSO2− anion XXIII, which reacts with the disulfide 47 to give radicals XXIV and XXVI along with the thiolate anion XXV. Subsequently, the thiolate anion XXV reacts with oxygen to form a thiyl radical XXIV and peroxide anion. Next, this radical regioselectively adds to the terminal C–C double bond of styrene 46 to produce benzylic radical XXVII that after reaction with O2 provides a peroxy radical XXVIII. The newly formed reacts with XXVII to form radical XXIX. Finally, the alkoxy radical XXIX abstracts a hydrogen atom from XXVI to afford hydroxysulfenylated product 48. It should be mentioned that aliphatic alkenes and dialkyl disulfides were completely inert in the present protocol. The authors speculated that the unreactivity of aliphatic alkenes in this strategy is related to the far less stability of the alkyl free radicals formed after the attack of the thiyl radical on the aliphatic alkenes. They also explained the reason of unreactivity of dialkyl disulfides to their strong S–S bond.
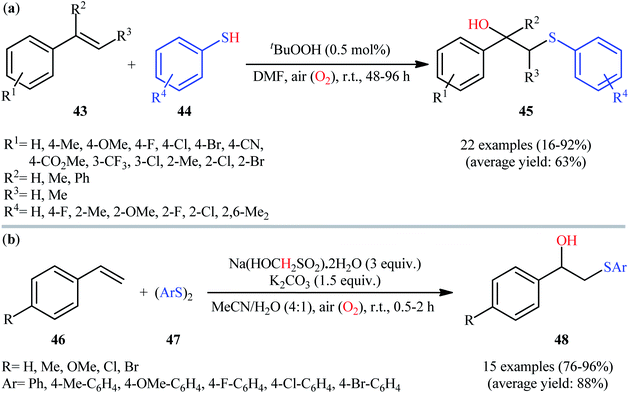 |
| Scheme 18 (a) TBHP-initiated air oxidative radical hydroxysulfenylation of styrenes 43 with arylthiols 44; (b) rongalite-mediated hydroxysulfenylation of styrenes 46 with aromatic disulfides 47 under ambient air. | |
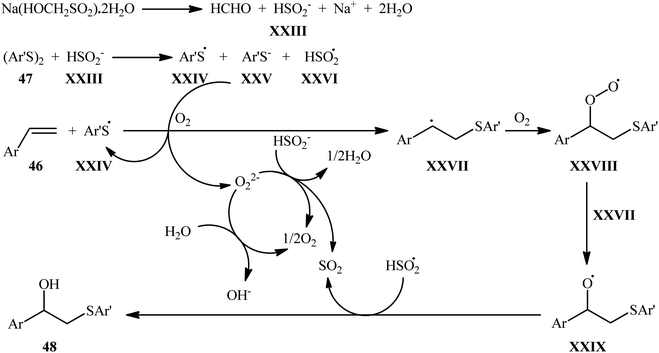 |
| Scheme 19 Proposed mechanism for the generation of β-hydroxy sulfides 48. | |
Finally, it should be noted that transition metal complexes play important role in synthesis of β-hydroxy sulfides and other compounds.33–54
7. Conclusion
1,2-Difunctionalization of alkenes has received continuous interests as it provides a valuable and versatile synthetic route to introduce two functional groups at the C
C bonds in a single click. Among them, intermolecular hydroxysulfenylation of alkenes is one of the most efficient and convenient pathways to the construction of biologically and synthetically important β-hydroxysulfides through the simultaneous C–O and C–S bond formation. As illustrated, various thiols, disulfides, sulfonyl hydrazides, and thiosulfates were successfully employed as the sulfenyl sources in this chemistry. Moreover, most of the developed systems were compatible with both terminal and internal alkenes. Despite the remarkable accomplishments during the past few years on this interesting page of β-hydroxysulfides synthesis, the construction of chiral β-hydroxysulfides through this chemistry, has, to date, been rarely described. Therefore, the development of chiral catalysts and ligands that allow asymmetric synthesis of titled compounds would be highly desirable. It is our hope that this Mini-Review will stimulate continued interest in the fabrication of β-hydroxysulfide derivatives directly from alkenes and make it a prolonged and prominent research arena for developing straightforward and extremely effective methodology used for pharmaceutical products syntheses.
Conflicts of interest
There are no conflicts to declare.
References
-
(a) C. Christophersen and U. Anthoni, Sulfur Rep., 1986, 4, 365–442 CrossRef CAS;
(b) S. Shafiei and S. Davaran, Chem. Rev. Lett., 2020, 3, 19–22 Search PubMed;
(c) S. Majedi and S. Majedi, J. Chem. Lett., 2020, 1, 2–8 Search PubMed;
(d) F. Nareetsile, J. T. P. Matshwele, S. Ndlovu and M. Ngaski, Chem. Rev. Lett., 2020, 3, 140–160 Search PubMed;
(e) F. Behmagham, Z. Asadi and Y. J. Sadeghi, Chem. Rev. Lett., 2018, 1, 68–76 Search PubMed.
- M. Feng, B. Tang, S. H Liang and X. Jiang, Curr. Top. Med. Chem., 2016, 16, 1200–1216 CrossRef CAS PubMed.
- P. Devendar and G.-F. Yang, Top. Curr. Chem., 2019, 375, 82 CrossRef PubMed.
- K. A. Scott and J. T. Njardarson, Top. Curr. Chem., 2018, 376, 5 CrossRef PubMed.
-
(a) S. S. Cho, L. N. Jungheim and A. J. Baxter, Bioorg. Med. Chem. Lett., 1994, 4, 715–720 CrossRef CAS;
(b) G. Valoti, M. I. Nicoletti, A. Pellegrino, J. Jimeno, H. Hendriks, M. D'Incalci, G. Faircloth and R. Giavazzi, Clin. Cancer Res., 1998, 4, 1977–1983 CAS;
(c) F. Viola, G. Balliano, P. Milla, L. Cattel, F. Rocco and M. Ceruti, Bioorg. Med. Chem., 2000, 8, 223–232 CrossRef CAS PubMed;
(d) R. S. Pavelyev, S. G. Gnevashev, R. M. Vafina, O. I. Gnezdilov, A. B. Dobrynin, S. A. Lisovskaya, L. E. Nikitina and E. N. Klimovitskii, Mendeleev Commun., 2012, 22, 127–128 CrossRef CAS;
(e) B. Guruswamy and R. Arul, Phosphorus, Sulfur Silicon Relat. Elem., 2013, 188, 1205–1213 CrossRef CAS;
(f) R. S. Pavelyev, R. M. Vafina, K. V. Balakin, O. I. Gnezdilov, A. B. Dobrynin, O. A. Lodochnikova, R. Z. Musin, G. A. Chmutova, S. A. Lisovskaya and L. E. Nikitina, J. Chem., 2018, 3589342 Search PubMed.
-
(a) F. Fringuelli, F. Pizzo, S. Tortoioli and L. Vaccaro, J. Org. Chem., 2003, 68, 8248–8251 CrossRef CAS PubMed;
(b) F. Fringuelli, F. Pizzo and L. Vaccaro, J. Org. Chem., 2004, 69, 2315–2321 CrossRef CAS PubMed;
(c) M. M. Mojtahedi, M. S. Abaee, A. Rajabi, P. Mahmoodi and S. Bagherpoor, J. Mol. Catal. A: Chem., 2012, 361, 68–71 CrossRef;
(d) N. Azizi and M. Edrisi, Tetrahedron Lett., 2016, 57, 525–528 CrossRef CAS.
-
(a) M. M. Khodaei, A. R. Khosropour and K. Ghozati, J. Braz. Chem. Soc., 2005, 16, 673–676 CrossRef CAS;
(b) B. C. Ranu and T. Mandal, Can. J. Chem., 2006, 84, 762–770 CrossRef CAS;
(c) V. Ganesh and S. Chandrasekaran, Synthesis, 2009, 3267–3278 CAS;
(d) M. Soleiman-Beigi and H. Kohzadi, Arabian J. Chem., 2019, 12, 1532–1536 CrossRef CAS.
-
(a) E. M. Beccalli, G. Broggini, S. Gazzola and A. Mazza, Org. Biomol. Chem., 2014, 12, 6767–6789 RSC;
(b) J. B. Peng, Adv. Synth. Catal., 2020, 362, 3059–3080 CrossRef CAS;
(c) J. Lin, R. J. Song, M. Hu and J. H. Li, Chem. Rec., 2019, 19, 440–451 CrossRef CAS PubMed;
(d) N. Yue and F. R. Sheykhahmad, J. Fluorine Chem., 2020, 109629 CrossRef CAS;
(e) L. Feng, X. Li, B. Liu and E. Vessally, J. CO2 Util., 2020, 40, 101220 CrossRef CAS.
- M. B. Marakalala, E. M. Mmutlane and H. H. Kinfe, Beilstein J. Org. Chem., 2018, 14, 1668–1692 CrossRef CAS PubMed.
-
(a) S. Arshadi, E. Vessally, L. Edjlali, R. Hosseinzadeh-Khanmiri and E. Ghorbani-Kalhor, Beilstein J. Org. Chem., 2017, 13, 625–638 CrossRef CAS PubMed;
(b) E. Vessally, K. Didehban, M. Babazadeh, A. Hosseinian and L. Edjlali, J. CO2 Util., 2017, 21, 480–490 CrossRef CAS;
(c) E. Vessally, K. Didehban, R. Mohammadi, A. Hosseinian and M. Babazadeh, J. Sulfur Chem., 2018, 39, 332–349 CrossRef CAS;
(d) E. Vessally, R. Mohammadi, A. Hosseinian, K. Didehban and L. Edjlali, J. Sulfur Chem., 2018, 39, 443–463 CrossRef CAS;
(e) A. Hosseinian, L. Zare Fekri, A. Monfared, E. Vessally and M. Nikpassand, J. Sulfur Chem., 2018, 39, 674–698 CrossRef CAS;
(f) A. Hosseinian, P. D. K. Nezhad, S. Ahmadi, Z. Rahmani and A. Monfared, J. Sulfur Chem., 2019, 40, 88–112 CrossRef CAS;
(g) A. Monfared, S. Ahmadi, Z. Rahmani, P. D. K. Nezhad and A. Hosseinian, J. Sulfur Chem., 2019, 40, 209–231 CrossRef CAS;
(h) A. Hosseinian, S. Arshadi, S. Sarhandi, A. Monfared and E. Vessally, J. Sulfur Chem., 2019, 40, 289–311 CrossRef CAS;
(i) F. A. H. Nasab, L. Z. Fekri, A. Monfared, A. Hosseinian and E. Vessally, RSC Adv., 2018, 8, 18456–18469 RSC;
(j) A. Hosseinian, S. Ahmadi, F. A. H. Nasab, R. Mohammadi and E. Vessally, Top. Curr. Chem., 2018, 376, 39 CrossRef PubMed;
(k) E. Vessally, A. Monfared, Z. Eskandari, M. Abdoli and A. Hosseinian, Phosphorus, Sulfur Silicon Relat. Elem., 2020, 196, 1–5 CrossRef.
-
(a) S. Arshadi, E. Vessally, L. Edjlali, E. Ghorbani-Kalhor and R. Hosseinzadeh-Khanmiri, RSC Adv., 2017, 7, 13198–13211 RSC;
(b) K. Nejati, S. Ahmadi, M. Nikpassand, P. D. K. Nezhad and E. Vessally, RSC Adv., 2018, 8, 19125–19143 RSC;
(c) A. Monfared, R. Mohammadi, S. Ahmadi, M. Nikpassand and A. Hosseinian, RSC Adv., 2019, 9, 3185–3202 RSC;
(d) A. Hosseinian, R. Mohammadi, S. Ahmadi, A. Monfared and Z. Rahmani, RSC Adv., 2018, 8, 33828–33844 RSC;
(e) A. Hosseinian, S. Ahmadi, R. Mohammadi, A. Monfared and Z. Rahmani, J. CO2 Util., 2018, 27, 381–389 CrossRef CAS;
(f) A. Monfared, R. Mohammadi, A. Hosseinian, S. Sarhandi and P. D. Nezhad, RSC Adv., 2019, 9, 3884–3899 RSC;
(g) S. Ebrahimiasl, F. Behmagham, S. Abdolmohammadi, R. Kojabad and E. Vessally, Curr. Org. Chem., 2019, 23, 2489–2503 CrossRef CAS;
(h) J. Wang, P. Sub, S. Abdolmohammadi and E. Vessally, RSC Adv., 2019, 9, 41684–41702 RSC;
(i) Y. Yang, D. Zhang and E. Vessally, Top. Curr. Chem., 2020, 378, 37 CrossRef CAS PubMed;
(j) Z. He, D. Wu and E. Vessally, Top. Curr. Chem., 2020, 378, 46 CrossRef CAS PubMed;
(k) S. Sarhandi, M. Daghagheleh, M. Vali, R. Moghadami and E. Vessally, Chem. Rev. Lett., 2018, 1, 9–15 Search PubMed;
(l) L. Sreerama, E. Vessally and F. Behmagham, J. Chem. Lett., 2020, 1, 9–18 Search PubMed;
(m) S. Mohammadi, M. Musavi, F. Abdollahzadeh, S. Babadoust and A. Hosseinian, Chem. Rev. Lett., 2018, 1, 49–55 Search PubMed;
(n) M. Daghagheleh, M. Vali, Z. Rahmani, S. Sarhandi and E. Vessally, Chem. Rev. Lett., 2018, 1, 23–30 Search PubMed;
(o) S. Farshbaf, L. Sreerama, T. Khodayari and E. Vessally, Chem. Rev. Lett., 2018, 1, 56–67 Search PubMed;
(p) S. Majedi, L. Sreerama, E. Vessally and F. Behmagham, J. Chem. Lett., 2020, 1, 25–31 Search PubMed;
(q) S. Majedi, S. Majedi and F. Behmagham, Chem. Rev. Lett., 2019, 2, 187–192 Search PubMed;
(r) E. A. Mahmood, B. Azizi and S. Majedi, Chem. Rev. Lett., 2020, 3, 2–8 Search PubMed;
(s) S. Shahidi, P. Farajzadeh, P. Ojaghloo, A. Karbakhshzadeh and A. Hosseinian, Chem. Rev. Lett., 2018, 1, 37–44 Search PubMed;
(t) M. R. J. Sarvestani, N. Mert, P. Charehjou and E. Vessally, J. Chem. Lett., 2020, 1, 93–102 Search PubMed.
- B. M. Trost, M. Ochiai and P. G. McDougal, J. Am. Chem. Soc., 1978, 100, 7103–7106 CrossRef CAS.
- Z. K. Abd El Samii, M. I. Al Ashmawy and J. M. Mellor, Tetrahedron Lett., 1986, 27, 5289–5292 CrossRef CAS.
- Z. K. Abd El-Samii, Arch. Pharm., 1991, 324, 461–463 CrossRef CAS.
- B. Movassagh and M. Navidi, Tetrahedron Lett., 2008, 49, 6712–6714 CrossRef CAS.
- H. Xi, B. Deng, Z. Zong, S. Lu and Z. Li, Org. Lett., 2015, 17, 1180–1183 CrossRef CAS PubMed.
- P. Tehri, B. Aegurula and R. K. Peddinti, Tetrahedron Lett., 2017, 58, 2062–2065 CrossRef CAS.
- R. Zhang, S. Jin, Y. Wan, S. Lin and Z. Yan, Tetrahedron Lett., 2018, 59, 841–847 CrossRef CAS.
- R. He, X. Chen, Y. Li, Q. Liu, C. Liao, L. Chen and Y. Huang, J. Org. Chem., 2019, 84, 8750–8758 CrossRef CAS PubMed.
- B.-q. Ni, Y. He, X. Rong and T.-f. Niu, Synlett, 2019, 30, 1830–1834 CrossRef CAS.
- M. Ueda, H. Miyabe, H. Shimizu, H. Sugino, O. Miyata and T. Naito, Angew. Chem., Int. Ed., 2008, 120, 5682–5686 CrossRef.
- J.-J. Zhao, M. Tang, H.-H. Zhang, M.-M. Xu and F. Shi, Chem. Commun., 2016, 52, 5953–5956 RSC.
- Z.-Q. Xu, L.-C. Zheng, L. Li, L. Duan and Y.-M. Li, Tetrahedron, 2019, 75, 643–651 CrossRef CAS.
- M. Kharasch, W. Nudenberg and G. Mantell, J. Org. Chem., 1951, 16, 524–532 CrossRef CAS.
- A. L. Beckwith and R. D. Wagner, J. Org. Chem., 1981, 46, 3638–3645 CrossRef CAS.
- H. Wang, Q. Lu, C. Qian, C. Liu, W. Liu, K. Chen and A. Lei, Angew. Chem., Int. Ed., 2016, 55, 1094–1097 CrossRef CAS PubMed.
- C. Huo, Y. Wang, Y. Yuan, F. Chen and J. Tang, Chem. Commun., 2016, 52, 7233–7236 RSC.
- K. Surendra, N. S. Krishnaveni, R. Sridhar and K. R. Rao, J. Org. Chem., 2006, 71, 5819–5821 CrossRef CAS PubMed.
- A. Kamal and D. R. Reddy, J. Mol. Catal. A: Chem., 2007, 272, 26–30 CrossRef CAS.
- B. Zhang, T. Liu, Y. Bian, T. Lu and J. Feng, ACS Sustainable Chem. Eng., 2018, 6, 2651–2655 CrossRef CAS.
- S.-F. Zhou, X. Pan, Z.-H. Zhou, A. Shoberu and J.-P. Zou, J. Org. Chem., 2015, 80, 3682–3687 CrossRef CAS PubMed.
- V. K. Yadav, V. P. Srivastava and L. D. S. Yadav, Tetrahedron Lett., 2015, 56, 2892–2895 CrossRef CAS.
- P. Xu, W. Lu, J. Zhang and L. Zhang, ACS Sustainable Chem. Eng., 2020, 8, 12366–12377 CrossRef CAS.
- P. Wang, T. Yao, Z. Li, W. Wei, Q. Xie, W. Duan and H. Han, Compos. Sci. Technol., 2020, 198, 108307 CrossRef CAS.
- R. Zhaoa, et al., Cem. Concr. Res., 2021, 144, 106420 CrossRef.
- J. Zhu, K. Yang, Y. Chen, G. Fan, L. Zhang, B. Guo and R. Zhao, J. Hazard. Mater., 2021, 409, 124504 CrossRef CAS PubMed.
- H. Zhang, W. Guan, L. Zhang, X. Guan and S. Wang, ACS Omega, 2020, 5, 18007–18012 CrossRef CAS PubMed.
- H. Zhang, M. Sun, L. Song, J. Guo and L. Zhang, Biochem. Eng. J., 2019, 147, 146–152 CrossRef CAS.
- J. Zhu, Y. Chen, L. Zhang, B. Guo, G. Fan, X. Guan and R. Zhao, J. Cleaner Prod., 2021, 295, 126405 CrossRef CAS.
- Y. Liu, B. Hu, S. Wu, M. Wang, Z. Zhang, B. Cui and M. Du, Appl. Catal., B, 2019, 258, 117970 CrossRef CAS.
- Y. Song, M. Xu, Z. Li, L. He, M. Hu, L. He and M. Du, Sens. Actuators, B, 2020, 321, 128527 CrossRef CAS.
- M. Wang, M. Hu, Z. Li, L. He, Y. Song, Q. Jia and M. Du, Biosens. Bioelectron., 2019, 142, 111536 CrossRef PubMed.
- M. Wang, L. Yang, B. Hu, J. Liu, L. He, Q. Jia and Z. Zhang, Biosens. Bioelectron., 2018, 113, 16 CrossRef CAS PubMed.
- Z. Lei, S. Hao, J. Yang, L. Zhang, B. Fang, K. Wei and C. Weif, Chemosphere, 2020, 128646 Search PubMed.
- Y. Wang, K. Huang, X. Lai, Z. Shi, J. Liu and G. Qiu, Org. Biomol. Chem., 2021, 1940–1944 RSC.
- Y. Qi, J. Wei, R. Qu, G. Al-Basher, X. Pan, A. A. Dar and Z. Wang, Chem. Eng. J., 2021, 403, 126396 CrossRef CAS.
- Y. Liu, T. Xu, Y. Liu, Y. Gao and C. Di, J. Mater. Res. Technol., 2020, 9, 8283–8288 CrossRef CAS.
- C. L. Shen, Q. Lou, J. H. Zang, K. K. Liu, S. N. Qu, L. Dong and C. X. Shan, Adv. Sci., 2020, 7, 1903525 CrossRef CAS PubMed.
- S. Ahmadi, A. Hosseinian, P. D. Kheirollahi Nezhad, A. Monfared and E. Vessally, Iran. J. Chem. Chem. Eng., 2019, 38, 1–19 CAS.
- W. Xiang, J. Chang, R. Qu, G. Albasher, Z. Wang, D. Zhou and C. Sun, Chemosphere, 2021, 265, 129112 CrossRef CAS PubMed.
- Y. Wang, K. Huang, X. Lai, Z. Shi, J. Liu and G. Qiu, Org. Biomol. Chem., 2021, 1940–1944 RSC.
- F. Gharibzadeh, E. Vessally, L. Edjlali, M. Es haghi and R. Mohammadi, Iran. J. Chem. Chem. Eng., 2020, 39, 51–62 Search PubMed.
- H. Zhang, W. Guan, L. Zhang, X. Guan and S. Wang, ACS Omega, 2020, 5, 18007–18012 CrossRef CAS PubMed.
- E. Vessally, S. Mohammadi, M. Abdoli, A. Hosseinian and P. Ojaghloo, Iran. J. Chem. Chem. Eng., 2020, 39, 11–19 CAS.
|
This journal is © The Royal Society of Chemistry 2021 |
Click here to see how this site uses Cookies. View our privacy policy here.