DOI:
10.1039/D0RA09751A
(Paper)
RSC Adv., 2021,
11, 5896-5902
The effects of linalool acupoint application therapy on sleep regulation
Received
17th November 2020
, Accepted 8th December 2020
First published on 3rd February 2021
Abstract
The Shenque acupoint is located in the umbilicus of the human body. In the human body meridians, the Shenque acupoint can regulate body functions. The Shenque acupoint was one of the important acupuncture acupoints for the treatment of insomnia. However, the effect of linalool applied at the Shenque acupoint to improve sleep was unknown. This study explored the hypnotic and sedative effects of the main component of lavender, linalool, on the Shenque acupoint of mice and rats. The effects on the sleep latency and sleep duration were studied with the supra-threshold dose of pentobarbital sodium, and the effects on the sleep rate were studied with the sub-threshold dose of pentobarbital sodium. In order to further study the feasibility and superiority of linalool administered at the Shenque acupoint, a pharmacokinetic study was carried out. The pharmacodynamic results showed that the mice and rats treated with linalool at Shenque had the highest sleep rate, the shortest sleep latency, and the longest sleep duration compared with other groups. The Tmax and t1/2 of the LS were longer than those of the LO, and had the characteristics of sustained release. The relative bioavailability of LS was 323.0 ± 31.66%. This showed that the combination of linalool and the Shenque acupoint had greater medicinal effects. This development will provide a new direction for improving sleep.
1 Introduction
The Shenque acupoint is located in the umbilicus of the human body. In the human body meridians, the Shenque acupoint can regulate body functions. The Shenque acupoint was one of the important acupuncture acupoints for the treatment of insomnia.1 The ancient Chinese medicine book “Huang Di Nei Jing” first described the Shenque acupoint. The book contained many theoretical expositions on the function of the Shenque acupoint in the body. It created a precedent for the study of the Shenque acupoint.2 Modern medical studies showed that the structure of the umbilical arteries was different from that of other arteries.3 Due to the rich blood vessels in the umbilical area and the high sensitivity of the skin, medicine was easily absorbed through the skin and entered the blood circulation, thereby reducing the damage from the metabolism by the digestive tract and liver and allowing it to fully perform its role. Compared with oral or parenteral routes, Shenque acupoint administration possessed numerous advantages, such as avoiding the first-pass effect in the liver, reduced side effects, reaching the peak plasma concentration faster, and extending the effective time of the drug.4 When moxibustion (burning dried aromatic plants of the genus Artemisia) was applied at Shenque for 20 min per day, 6 days per week for 12 weeks, the learning capability of ApoE−/− mice was enhanced via inhibiting oxidized proteins (GFAP and β-amyloid) in astrocytes.5 Moxibustion at Shenque could also prolong the swimming time, and decrease the serum MDA, AST, ALT and BUN levels and LDH activity in rats after long-term fatigue.6 Y. Wu had shown that the effectiveness of a conventional basic treatment combined with a “Lunxieting” umbilical patch for the treatment of children with rotavirus gastroenteritis was 92%, and the treatment effectiveness of a conventional basic treatment combined with oral administration was 75%.7 These research results had given us great inspiration.
In recent years, researching natural products for the treatment of diseases has been recognized throughout the world. Lavender essential oil is a natural medicine commonly used in aromatherapy. It has many biological activities such as sedation, hypnosis, and anti-anxiety, anti-inflammatory and antioxidant behaviours, and so on.8–11 Linalool, as the main ingredient of lavender essential oil (Fig. 1), has also been proved to have sedative and hypnotic pharmacological effects.12 Linck found that inhaling different concentrations of linalool (1%, 3%) could prolong the sleep duration induced by pentobarbital, reduce the body temperature, and slow down the exercise behavior of mice, and does not affect the coordination of the mice.13 Studies found14 that linalool caused sedation, relaxation, and reduced aggression and hostility. In addition, linalool and lavender essential oil exhibited behavioural sedative-like effects by decreasing the renal sympathetic nerve activity and increasing the parasympathetic nerve activity.15,16 The sedative effect of linalool could be accomplished by the modulation of glutamatergic neurotransmission. In vitro and in vivo studies showed that linalool acted as a competitive antagonist of the excitatory neurotransmitter glutamate by binding to glutamatergic N-methyl-D-aspartate (NMDA) receptors.17,18 Linalool also reduced the potassium stimulated release of glutamate.19
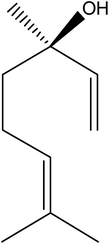 |
| Fig. 1 The chemical structure of linalool. | |
With changes in the environment, work pressure, and life pressure, more people have experienced a series of sub-health states, such as insomnia. The increase in the incidence of depression and other diseases has seriously affected people’s health and quality of life.20–22 At present, insomnia is mainly treated with sedative hypnotic drugs. However, these drugs have obvious side effects, including drug dependence, excessive sedation, cognitive impairment, and movement incongruity.23 In addition, for some people it is not suitable or inconvenient to use traditional applications of drugs, which increases the difficulty of drug treatment.24 Thus, finding an effective drug and a convenient route of administration has become a focus. “Yizongjinjian” said that Shenque could cure a variety of diseases.25 At present, there are no reports of the use of linalool at the Shenque acupoint to improve sleep. Therefore, in the present study we combined linalool with Shenque acupoint administration, and compared it with lavender oil and different administration methods. We determine the relative bioavailability of Shenque acupoint administration and oral administration to evaluate the advantages of Shenque acupoint administration, hoping to develop a better way to improve sleeping.
2 Materials and methods
2.1 Materials and reagents
Pentobarbital sodium, batch number: 6BR10450, was purchased from Glenview. Linalool, >98% (HPLC), was prepared in the laboratory from lavender essential oil which was purchased from Beijing Beida Zhengyuan Technology Co., Ltd. Diazepam was purchased from Beijing Yimin Pharmaceutical Co., Ltd. The medical vaporizer is a SS-7A compress vaporizer. Medical petroleum jelly was purchased from the Tianjin Da Mao chemical reagents factory. An Agilent 7890 B Gas Chromatographic system-FID detector (GC-FID) was used and was equipped with a HP-5 column (5% phenyl-methylpolysiloxane, 30 m × 0.320 mm × 0.25 mm) purchased from America. HPLC was performed on a HPLC system (LC3000, Beijing ChuangXinTongHeng Science & Technology Co., Ltd., Beijing, China) consisting of a P3000 pump and a UV3000 spectrophotometric detector at 203 nm (Beijing ChuangXinTongHeng Science & Technology Co., Ltd.) using a Promosil C18 column (250 mm × 4.6 mm, 5 μm).
The experimental drug dose for the mice was taken from the literature,24 and the experimental drug dose for the rats was comparable with that of the mice.
2.2 Purification and determination of linalool
We used silica gel column chromatography to obtain linalool from the lavender essential oil. The separation conditions were petroleum ether
:
ethyl acetate = 10
:
1. The linalool in the lavender essential oil was determined by gas chromatography (GC). The GC analytical conditions were: the temperature of the column oven was elevated from 80 °C (reserving 5 min) to 160 °C at a rate of 2 °C min−1, and nitrogen (99.999%) was used as carrier gas, whose flow rate was 1 mL min−1. The injection volume was 1 μL, and the split ratio was adjusted to 1
:
10.
2.3 Animals and treatment
Wistar rats and Kunming mice (Animal License no. SCXK (Liao) 2015-0001) were purchased from the experimental animal center of Shenyang Pharmaceutical University, with the ratio of females and males as half and half, and were put in cages and kept at a temperature of 23 ± 2 °C, a humidity of 55 ± 5% and a circadian rhythm of 12 h day/12 h night. The rats were allowed free access to water and food throughout the experimental period. Body weight was measured weekly, and food intake was recorded daily. All animal procedures were performed in accordance with the Guidelines for Care and Use of Laboratory Animals of Shenyang Pharmaceutical University and approved by the Institutional Animal Ethics Committee (approval protocol number: SYPU-IACUC-C2017-3.8-107; Liaoning, China).
2.4 Determination of the sub-threshold dose and threshold dose of pentobarbital sodium
The sub-threshold dose of pentobarbital sodium (the maximum dose at which the righting reflex of 90–100% of mice and rats does not disappear) was determined. The 40 mice were randomly assigned into 4 groups (10 mice in each group). They were injected intraperitoneally with pentobarbital sodium. The doses were 20, 25, 30 and 40 mg kg−1 of the body weight,13 and the sub-threshold dose was measured. The 24 rats were randomly assigned into 4 groups (6 rats in each group). They were injected intraperitoneally with pentobarbital sodium. The doses were 10, 15, 20 and 30 mg kg−1 of the body weight, and the sub-threshold dose was measured.
The threshold dose of pentobarbital sodium (the dose at which the righting reflex of 100% of the mice and rats disappears but they do not sleep longer) was measured. The groups of the mice and rats were the same as when measuring the sub-threshold. The mice were injected intraperitoneally with pentobarbital sodium (50, 55, 60, and 70 mg kg−1).13 The rats were injected intraperitoneally with pentobarbital sodium (30, 35, 40, and 50 mg kg−1), and the threshold dose was measured.
2.5 Determination of the sleep rate, sleep latency and sleep duration in the mice and rats
The mice were divided into 6 groups with 10 in each group: the linalool Shenque acupoint group (LS), 400 mg kg−1;26 the lavender Shenque acupoint group (LaS), 400 mg kg−1; the lavender oral group (LaO), 400 mg kg−1; the lavender inhalation group (LaI), 3%; the positive control group (diazepam), 5 mg kg−1; the control group.
The rats were divided into 6 groups with 10 in each group: the LS, 300 mg kg−1;26 the LaS, 300 mg kg−1; the LaO, 300 mg kg−1; the LaI, 3%; the positive control group (diazepam), 3.5 mg kg−1; the control group.
According to the above-mentioned administration doses, 30 minutes after the administration of the drug, the sub-threshold dose of pentobarbital sodium was injected intraperitoneally, and within 30 minutes if the righting reflex of the mice disappeared for more than 1 minute they were asleep. The number of sleeping mice was recorded, and the sleep rate was calculated.
According to the above-mentioned administration doses, 30 minutes after the administration of the drug, the supra-threshold dose of pentobarbital sodium was injected intraperitoneally, and if the mice’s righting reflex disappeared for more than 1 minute they were asleep. The time started at the pentobarbital sodium injection, and timing continued until the righting reflex disappeared and the recorded time was the sleep latency. The time from the righting reflex disappearing until the righting reflex reappearing was taken as the length of sleep. The sleep duration was generally more than 30 min after the drug action, but it was generally not more than 100 min, which was similar to the effectiveness of other experimental data.25
2.6 Determination of the relative bioavailability of linalool with Shenque acupoint administration
12 Wistar rats were randomly divided into 2 groups, the linalool oral group (LO) and the LS, the dosage was 200 mg kg−1, and they were fasted for 12 hours before the experiment. The LO received a 0.5% CMC-Na suspension with a linalool content of 10%, and the LS received a petroleum jelly mixture with a linalool content of 10% which was applied to the Shenque acupoint. After administration, blood was collected from the tail vein at the time points (5, 10, 15, 20, 30, 40, 50, 60, 120, 180, 240, 360, and 480 min) and placed in heparinized 1.5 mL centrifuge tubes. After centrifugation at 10
000 rpm and 25 °C for 10 min, the separated plasma was stored in a refrigerator (−20 °C) until the HPLC analysis.
2.6.1 Preparation of the standard solution and internal standard. a 400 μg mL−1 linalool stock solution and a 400 μg mL−1 nifedipine internal standard stock solution were prepared in acetonitrile solution and stored at 4 °C. After the stock solution was appropriately diluted, a known amount of linalool was added to the blank plasma to prepare standard curve working solutions with concentrations of 0.1, 0.2, 0.5, 1, 1.5, 2, and 3 μg mL−1. The concentrations of the low, medium and high plasma quality control sample working solutions were 0.2 μg mL−1, 1 μg mL−1 and 2.5 μg mL−1, respectively. The stock solution was diluted with acetonitrile to obtain the internal standard working solution with a concentration of 5 μg mL−1. All plasma samples were stored at −20 °C in the dark.
2.6.3 Validation of the analytical methods. The blank plasma, the 10 min plasma sample of the LO group combined with the internal standard, and the 10 min plasma sample of the LS group combined with the internal standard were investigated for specificity. The linear range is 0.1–3 μg mL−1 (n = 3), and the calibration curves of the peak area ratio (linalool peak area/internal standard (IS) peak area) and plasma concentration were drawn by the internal standard method. By analyzing the lowest concentration of analyte (n = 5), the lower limit of quantification was studied with acceptable accuracy and precision (<20%), and the detection limit was determined with a signal-to-noise ratio >5.The precision and accuracy (intraday and interday) of the low concentration (0.2 μg mL−1), medium concentration (1 μg mL−1) and high concentration (2.5 μg mL−1) plasma quality control samples (n = 5) were determined quantitatively. The precision and accuracy were expressed as the variation (CV%) and relative error (RE%), respectively. The peak area ratio of the extracted quality control sample was compared with that of the standard sample of the same concentration prepared in the solution to determine the recovery rate. In addition, we studied the stability of the quality control samples (n = 3) at room temperature, from the autosampler, and after three consecutive freeze–thaw cycles.
2.7 Statistical analysis
Statistical analysis was done using GraphPad Prism 8.0.2 statistical software, and the results were presented as mean ± s.e.m. Student’s t test was used. P < 0.05 means the difference was statistically significant. DAS 2.0 pharmacokinetic software analyzed the plasma concentration–time data of the rat plasma to obtain the pharmacokinetic related parameters.
3 Results and discussion
3.1 Purification and determination of linalool
We analyzed the lavender oil and linalool by GC, and identified the linalool in the lavender oil according to the retention time. From this, we concluded that linalool was the main component in lavender oil, which was the main functional calming substance of the lavender oil (Fig. 2).
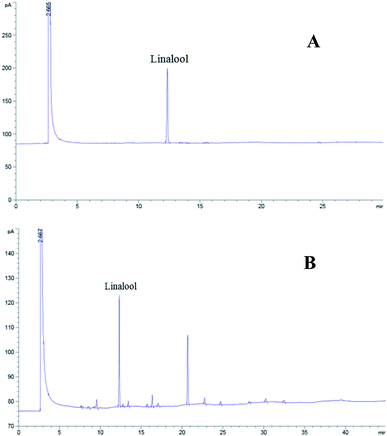 |
| Fig. 2 GC chromatograms of linalool (A) and lavender oil (B) (linalool RT = 12.34 min). | |
3.2 Determination of the supra-threshold and sub-threshold doses of pentobarbital sodium (for mice and rats)
In this study, we determined the supra-threshold dose and sub-threshold dose of pentobarbital sodium (for mice and rats). From the experimental results: the sub-threshold doses of pentobarbital sodium: 30 mg kg−1 in mice, 20 mg kg−1 in rats; the supra-threshold doses of pentobarbital sodium: 60 mg kg−1 in mice and 40 mg kg−1 in rats.
3.3 Effect on the sleep rate, sleep latency and sleep duration in mice
The sleep rate results showed that the sleep rate of the LS was 70%, which was significantly higher than that of other groups (Fig. 3A). The sleep latency results showed that the sleep latency of the LS was the shortest, 5.88 ± 1.72 min. It was significantly lower than that of the positive drug group and other experimental groups. The sleep duration results showed that the longest sleep duration was in the LS, 81.90 ± 19.70 min, which was significantly longer than that of the other groups. Compared with the positive control group, the LS and the LaS treatments showed the same or stronger efficacy than the positive control drugs. At the same time, for the comparison of the different administration methods of lavender, the Shenque acupoint administration method could be significantly better than the oral and inhalation methods according to different experimental indicators (Table 1).
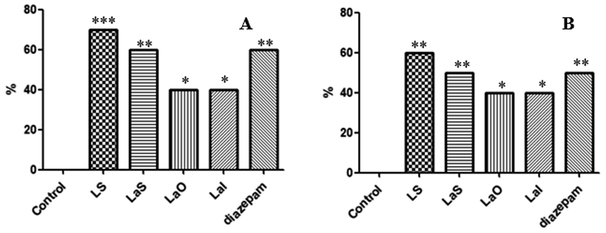 |
| Fig. 3 Determination of the sleep rates of rats and mice. (A) The sleep rate of mice. (B) The sleep rate of rats (LS: the linalool Shenque acupoint group; LaS: the lavender Shenque acupoint group; LaO: the lavender oral group; LaI: the lavender inhalation group; diazepam: the positive control group; ***P < 0.001, **P < 0.01, *P < 0.05). | |
Table 1 Sub-threshold dose sleep latency and sleep duration tests of pentobarbital sodium in mice and rats in the different groupsa
Groups |
Samples |
Mice |
Rats |
Dose (mg kg−1) |
Sleep latency (min) |
Sleep duration (min) |
Dose (mg kg−1) |
Sleep latency (min) |
Sleep duration (min) |
Compared with the control group, ***P < 0.001, **P < 0.01, *P < 0.05. |
Control |
10 |
|
11.90 ± 3.11 |
38.20 ± 15.67 |
|
8.50 ± 2.10 |
20.00 ± 5.30 |
LS |
10 |
400 |
5.88 ± 1.72*** |
81.90 ± 19.70*** |
300 |
5.10 ± 0.44** |
64.40 ± 11.29*** |
LaS |
10 |
400 |
6.20 ± 3.02* |
69.60 ± 11.90** |
300 |
5.56 ± 1.20* |
62.34 ± 12.20*** |
LaO |
10 |
400 |
8.30 ± 3.52* |
40.30 ± 8.32 |
300 |
7.42 ± 1.60 |
38.65 ± 8.45 |
LaI |
10 |
0.03 |
6.40 ± 3.10** |
58.75 ± 10.60** |
0.03 |
6.20 ± 0.70* |
50.20 ± 10.10** |
Diazepam |
10 |
5 |
6.20 ± 1.40*** |
65.47 ± 12.10** |
3.5 |
5.25 ± 0.48** |
63.50 ± 10.50*** |
3.4 Effect on the sleep rate, sleep latency, and sleep duration in rats
The sleep rate results showed that the sleep rate of the LS was 60%, which was significantly higher than that of the other groups (Fig. 3B). The sleep latency results showed that the sleep latency of the LS was the shortest, 5.10 ± 0.44 min. It was significantly lower than the sleep latencies of the positive drug group and the other experimental groups. The sleep duration results showed that the longest sleep duration was in the LS, 64.40 ± 11.29 min, which was significantly higher than that of the other groups. Compared with the positive control group, the LS and the LaS treatments showed the same or stronger efficacy than the positive drugs. The conclusion was the same as that for the mice (Table 1).
3.5 Determination of the relative bioavailability of linalool Shenque acupoint administration
3.5.1 Validation of the analytical methods. The retention time (RT) of linalool (1) was 25.9 min, and that of nifedipine (2) was 28.1 min (Fig. 4). In the range of 0.1–3 μg mL−1, linalool had a good linear relationship, and in the linear regression equation Y = 0.0007X + 0.0747 (r = 0.9982). The results of the verification process are shown in Table 2. The detection limit was 0.03 μg mL−1, and the lower limit of quantification was 0.1 μg mL−1. As shown in Fig. 4, plasma endogenous substances did not interfere with the internal standard and linalool chromatographic peaks, and the method had good specificity.
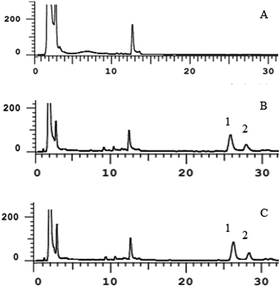 |
| Fig. 4 Specificity investigation. (A) Blank plasma; (B) blank plasma + linalool + internal standard; (C) plasma after oral administration of linalool for 10 min added with the internal standard. (1) Linalool; (2) nifedipine (internal standard). | |
Table 2 Results from the analytical methods validation
Parameter |
Result |
Linearity |
0.1–3 μg mL−1 |
Correlation coefficient |
r = 0.9982 |
Limit of quantification |
0.1 μg mL−1 |
Limit of detection |
0.03 μg mL−1 |
![[thin space (1/6-em)]](https://www.rsc.org/images/entities/char_2009.gif) |
Stability |
Short term |
87.56–100.2% |
Freeze and thaw cycles |
93.45–103.7% |
Autosampler |
95.92–100.7% |
![[thin space (1/6-em)]](https://www.rsc.org/images/entities/char_2009.gif) |
Precision (CV%) |
Intraday |
7.1 |
Interday |
9.0 |
![[thin space (1/6-em)]](https://www.rsc.org/images/entities/char_2009.gif) |
Accuracy (RE%) |
Intraday |
4.4 |
Interday |
6.5 |
Recovery (%) |
87 |
At room temperature, and after automatic injection and three consecutive freeze–thaw cycles, the linalool showed satisfactory stability. The deviation range is shown in Table 2. The precision and accuracy were expressed as coefficient of variation (CV%) and relative error (RE%), respectively. Both intra-day and inter-day analysis of the two parameters showed CV and RE < 15%, which proved the reliability of the quantitative method for linalool in plasma samples.
3.5.2 Pharmacokinetic analysis. The plasma concentration–time curves of the linalool group and the linalool Shenque group were consistent with non-compartment model. The average plasma concentration–time curve at each time point is shown in Fig. 5. The drug could still be detected in the plasma of the LS rats after 480 min. The level of the drug in the LO rat plasma was already below the detection limit after 240 minutes (Fig. 5). The Tmax, t1/2, Cmax and MRT0–t of the LS were significantly prolonged or increased compared with those of the LO (Table 3). The Tmax and t1/2 of the LS were longer than those of the LO, and had the characteristics of sustained release.
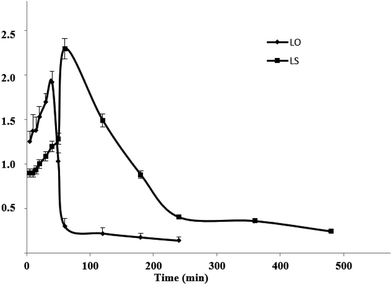 |
| Fig. 5 Plasma concentration–time curves for the LS and the LO (n = 6) (LS: the linalool Shenque acupoint group; LO: linalool oral group). | |
Table 3 Pharmacokinetic parameters after administration in rats (n = 6)a
Parameter |
LO |
LS |
***P < 0.001, *P < 0.05. |
t1/2 (min) |
164.7 ± 26.39 |
214.9 ± 151.4 |
Cmax (μg mL−1) |
1.92 ± 0.12 |
2.29 ± 0.15*** |
Tmax (min) |
40.0 ± 0.35 |
60.0 ± 0.42 |
AUC0–t (μg mL−1 min−1) |
116.2 ± 6.45 |
373.2 ± 15.96*** |
AUC0–∞ (μg mL−1 min−1) |
147.9 ± 10.51 |
452.9 ± 85.81*** |
MRT0–t (min) |
63.01 ± 7.19 |
155.5 ± 3.33*** |
MRT0–∞ (min) |
154.6 ± 18.91 |
282.9 ± 126.9*** |
Fr (%) |
— |
323.0 ± 31.66 |
3.5.3 Relative bioavailability. Relative bioavailability (Fr) is an important parameter for evaluating the effects of different administration methods,26 and its calculation is as follows:
Fr (%) = AUC0–t (LS)/AUC0–t (LO) |
LS: the linalool Shenque acupoint group; LO: the linalool oral group.The main pharmacokinetic parameters are shown in Table 3:
For the LO and the LS, the Cmax was 1.92 ± 0.12 and 2.29 ± 0.15 μg mL−1, respectively. The Tmax was 40 ± 0.35 and 60 ± 0.42 min, respectively. The t1/2 was 164.7 ± 26.39 and 214.9 ± 151.4 min, respectively. The AUC0–t was 116.2 ± 6.45 and 373.2 ± 15.96 μg mL−1 min−1, respectively. The AUC0–∞ was 147.9 ± 10.51 and 452.9 ± 85.81 μg mL−1 min−1, respectively. The MRT0–t was 63.01 ± 7.19 and 155.5 ± 3.33 min, respectively. The relative bioavailability of LS was 323.0 ± 31.66%.
4 Conclusions
In Chinese medicine, external treatment was one of the most precious therapies. It was famous for its safety, convenience and obvious effect. The treatment penetrated and was absorbed through the skin and entered the internal organs to achieve its purpose. The Shenque acupoint possesses abundant microcirculation, endothelial cells and microvascular endothelial cells, transient receptor potential vanilloid family channels, and neuropeptides secreted by endothelial cells.28 Interestingly, when the Valia-Chien diffusion cell method was used to conduct permeability tests with the fresh acupoint and non-acupoint skin of rats, the 24 h cumulative steady-state infiltration rate through the Shenque acupoint skin was higher than that through non-acupoint skin, indicating that the Shenque acupoint was a natural channel and carrier for drug delivery.29 In this study, the Shenque acupoint was combined with transdermal administration, and the drug penetrated into the blood through the Shenque acupoint to exert its effect. The dual action of the drugs and the meridian acupoints could achieve the purpose of improving sleep. The results showed that linalool combined with the Shenque acupoint could improve the sleep rate, shorten the sleep latency and prolong the sleep duration in rats and mice. In addition, we also found that the LS had a higher bioavailability than the LO, and the absorption and elimination of the LS was slower and longer than those of the LO. In summary, linalool Shenque acupoint administration is a potentially therapeutic approach to treat sleep disorders and improve sleep quality. At the same time, the design of the patch is also more conducive for use by different groups of people. Further experiments are needed to demonstrate the mechanism of improving sleep.
Conflicts of interest
There are no conflicts of interest regarding the publication of this paper.
Acknowledgements
This work was supported by the Liaoning (FGW) Engineering Technology Research Center for the industrial chromatographic preparation of natural innovative drug materials (2017-1007) and the Technology Platform of Industrialization Chromatographic Preparation for Standard Extract of Traditional Chinese Medicine (2010ZX09401-304-105B).
References
- H. T. Li, J. H. Liu and Q. X. Zhu, J. Integr. Med., 2005, 3, 398–399 Search PubMed.
- Y. M. Zhang, L. Xu, M. Ding, G. Y. Su and Y. Q. Zhao, J. Ethnopharmacol., 2019, 231, 486–493 CrossRef CAS.
- X. M. Feng, J. M. Sun and N. W. Xu, Nurse Education Today, 2018, 20, 71–74 Search PubMed.
- J. F. Jiang, W. F. Xu, X. G. Yu and X. J. Wu, Chin. Acupunct. Moxibustion, 2017, 12, 1304–1308 Search PubMed.
- J. T. Liu, B. X. Zhao, Y. X. Cui, Y. H. Huang, C. Huang, J. Huang, L. Han and L. X. Lao, Evidence-Based Complementary and Alternative Medicine, 2015, 6, 1–8 Search PubMed.
- L. G. Zhou, X. H. Zhou, X. K. Xu, Y. L. Liang, F. Gao, C. Zhang, L. H. Sun and X. S. Ma, Journal of Acupuncture and Tuina Science, 2017, 15, 387–391 CrossRef.
- Y. Wu, W. Q. Chen and Y. Jin, Chinese Journal of Integrated Traditional and Western Medicine, 2010, 30, 1275–1278 Search PubMed.
- M. D. Sanna, F. Les, V. Lopez and N. Galeotti, Front. Pharmacol., 2019, 10, 472 CrossRef CAS.
- Y. Zhong, Q. Zheng, P. Y. Hu, X. Y. Huang, M. Yang, G. L. Ren, Q. Du, J. Luo, K. N. Zhang, J. Li, H. X. Wu, Y. Y. Guo and S. S. Liu, BMC Complementary Altern. Med., 2019, 19, 306 CrossRef.
- S. Monika, G. Anna, K. Edward, W. O. Anna, J. B. Marta and L. Monika, Molecules, 2014, 19, 20929–20940 CrossRef.
- G. F. E. Cardia, S. E. Silva-Filho, E. L. Silva, N. S. Uchida, H. A. O. Cavalcante, L. L. Cassarotti, V. E. C. Salvadego, R. A. Spironello, C. A. Bersani-Amado and R. K. N. Cuman, Evidence-Based Complementary and Alternative Medicine, 2018, 2018, P1413940 CrossRef.
- A. C. Aprotosoaie, M. Hancianu, I. I. Costache and A. Miron, Flavour Fragrance J., 2014, 29, 193–219 CrossRef CAS.
- L. Viviane de Moura, L. S. Adriana, F. Micheli, P. Angelo Luis, H. Ana Paula, B. Franciele Dupont, C. Elina Bastos, N. Domingos Savio, H. M. Paulo Roberto and E. Elaine, Phytomedicine, 2009, 16, 303–307 CrossRef.
- D. Clara and B. Gerhard, Flavour Fragrance J., 2011, 26, 300–316 CrossRef.
- J. Shen, A. Niijima, M. Tanida, Y. Horii, T. Nakamura and K. Nagai, Neurosci. Lett., 2007, 416, 241–246 CrossRef CAS.
- A. T. Peana, P. S. D’Aquila, F. Panin, G. Serra, P. Pippiai and M. D. L. Maretti, Phytomedicine, 2002, 9, 721–726 CrossRef CAS.
- E. Elisabetsky, J. Marschner and D. O. Souza, Neurochem. Res., 1995, 20, 461–465 CrossRef CAS.
- E. Elisabetsky, L. F. Silva Brum and D. O. Souza, Phytomedicine, 1999, 6, 107–113 CrossRef CAS.
- L. F. Silva Brum, T. Emanuelli and D. O. Souza, Neurochem. Res., 2001, 26, 191–194 CrossRef CAS.
- M. Fornaro, A. Anastasia, S. Novello, A. Fusco, M. Solmi, F. Monaco, N. Veronese, D. D. Berardis and A. Bartolomeis, Bipolar Disord., 2018, 20, 195–227 CrossRef.
- M. Frey, V. Obermeier, R. Kries and G. Schulte-Körne, J. Psychiatr. Res., 2020, 129, 17–23 CrossRef.
- E. Kurczewska, E. Ferensztajn-Rochowiak, F. Rybakowski, M. Michalak and J. Rybakowski, World J. Biol. Psychiatry, 2020, 1–28 Search PubMed.
- S. Dujardin, A. Pijpers and D. Pevernagie, Sleep Medicine Clinics, 2020, 15, 133–145 CrossRef.
- A. Tobias, C. Stefano and G. Gabriella, Pharmacol. Rev., 2018, 70, 197–245 CrossRef.
- Y. Yang, N. Zhang and Q. Duan, Journal of Yunnan Chinese Medicine., 2018, 39, 88–90 Search PubMed.
- R. Alnamer, K. Alaoui, E. H. Bouidida, A. Benjouad and Y. Cherrah, Trends Pharmacol. Sci., 2012, 2012, 1–5 Search PubMed.
- F. Shi, Y. Zhao, C. K. Firempong and X. Xu, Pharm. Biol., 2016, 54, 2320–2328 CrossRef CAS.
- J. F. Jiang, W. F. Xu, X. G. Yu and X. J. Wu, Chin. Acupunct. Moxibustion, 2017, 12, 1304–1308 Search PubMed.
- L. Feng, M. H. Ding, Q. Shen, Q. H. Sun, S. L. Shi and J. Tradit, Chin. Med., 2016, 8, 1546–1552 Search PubMed.
|
This journal is © The Royal Society of Chemistry 2021 |
Click here to see how this site uses Cookies. View our privacy policy here.