DOI:
10.1039/D0RA09648B
(Paper)
RSC Adv., 2021,
11, 1128-1133
Hydroboration of nitriles and imines by highly active zinc dihydride catalysts†
Received
13th November 2020
, Accepted 18th December 2020
First published on 4th January 2021
Abstract
Eco-friendly zinc dihydrides stabilized by N-heterocyclic carbenes were demonstrated to be highly efficient catalysts for the double hydroboration of nitriles with pinacolborane, exhibiting turnover frequencies up to 3000 h−1 at room temperature under solvent-free conditions. The reactions afforded corresponding diboronated amines with excellent yields and good functional group tolerance. A single Zn–H insertion product was isolated from a stoichiometric reaction of zinc dihydride with nitrile, and was proved to be an active species in this transformation. Kinetic studies were performed to give some insights into the catalytic reactions. In addition, zinc dihydride species also showed high activity for the hydroboration of imines to boronated amines.
Introduction
The development of efficient synthetic approaches for amines is of great significance because this class of compounds has a widespread application in the fine chemical, agrochemical, and pharmaceutical industries.1,2 In this context, catalytic reduction of unsaturated compounds containing e.g. C
N or C
N bonds with borane has recently emerged and attracted extensive attention, because it usually affords high reaction selectivity and employs mild reaction conditions.3 Moreover, corresponding hydroboration products, i.e. borylamines, can be used for the synthesis of a variety of organic compounds.4 The first example of nitrile dihydroboration was reported by Nikonov et al. using a molybdenum complex (2,6-iPr2-C6H3N)Mo(H)(Cl)(PMe3)3 (1a, Fig. 1), achieving a turnover frequency (TOF) up to 1.7 h−1.5 Since then, many efforts have been made for the development of catalysts used for this transformation.6 Over the last few years, it has been expanded to include a variety of metal complexes, including s-,7 p-,8 d-9 and f-block10 elements, and the representatives of effective complexes for the dihydroboration of nitriles are shown in Fig. 1. However, most of above complexes show low to mediate catalytic activity and some of them are unattractive due to their precious or combustible nature.
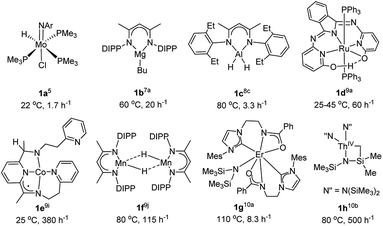 |
| Fig. 1 Selected metal complexes effective for the hydroboration of nitriles (reaction temperature and maximum TOF obtained were also listed). | |
Considering the biocompatibility, environmental friendliness and abundance of zinc ion, it is attractive to use zinc-based catalysts for various organic transformations. Zinc-based complexes have been successfully used in the hydroboration of a series of unsaturated molecules, including ketones,11 imines,12 alkynes,13 and N-heterocycles,14 but are rarely seen to be used in the hydroboration of organic nitriles. To the best of our knowledge, only one precedent was reported by Panda et al.15 recently by disclosing that the imidazoline-2-iminato ligand-based zinc alkyl complexes were active toward hydroboration of nitriles under heating conditions (60 °C), achieving a maximum TOF of 50 h−1. In our previous work, we synthesized two molecular zinc(II) dihydrides supported by phosphine-functionalized N-heterocyclic carbene ligands (Fig. 2), that were found to be efficient for hydroboration of thermodynamic stable and kinetic inert CO2 under mild conditions.16 Being inspired by this result, we herein investigated further of these complexes for the catalytic hydroboration of nitriles and imines. Gratifyingly, zinc dihydrides 2 exhibit remarkably high activity for hydroboration of a variety of nitriles and imines, producing boronated amines with a TOF of up to 3000 h−1 at room temperature in the absence of solvent. A plausible mechanism is also proposed based on the isolation of a catalytic intermediate and kinetic studies.
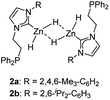 |
| Fig. 2 Zinc dihydrides used in this study. | |
Results and discussion
We initiated our study by using complex 2a (1 mol% based on Zn) for the reaction of benzonitrile with pinacolborane (HBpin) at room temperature in various solvents and the results are summarized in Table 1. The reactions gave low conversions of benzonitrile in the employed solvents (entries 1–3; 54% in C6D6, 29% in PhF, 26% in THF) in 2 h. It's noteworthy that in all of these cases, no intermediate species, i.e. PhCH
NBpin, was detected. Excitingly, a significant increase in activity was achieved when reaction was conducted in the absence of any solvent, which consumed all of the benzonitrile in a short time (8 min; entry 4), exclusively affording diboronated amine PhCH2N(Bpin)2 (5a) with a TOF of 750 h−1. To our knowledge, this represents the highest activity reported to date for the hydroboration of benzonitrile.7c,10b Complex 2b was also applied in this reaction under similar conditions, which resulted in a lower conversion of benzonitrile (85%; entry 5) probably due to the sterically bulky NHC ligand employed for complex 2b. To make a comparison, a tridentate monoanionic ligand-based zinc monohydride, i.e. LNNPZnH [LNNP = CH3C(2,6-iPr2C6H3N)CHC(CH3)(NCH2CH2PPh2)],17 previously reported by our group, was also prepared and examined under neat conditions. However, it is almost ineffective toward the current reaction with 1 mol% loading in 2 h (entry 6). NHC ligand employed in 2a alone was found inert for the reaction under the same conditions (entry 7).
Table 1 Zinc hydride-catalyzed hydroboration of benzonitrilea

|
Entry |
Catalyst |
Loading (mol%) |
Solvent |
Time (min) |
Conv.b (%) |
Reaction conditions: nitrile (0.30 mmol), pinacolborane (0.63 mmol), room temperature. Conversions were determined by 1H NMR spectroscopy (integration of residual benzonitrile vs. diborylamine product). |
1 |
2a |
0.5 |
C6D6 |
120 |
54 |
2 |
2a |
0.5 |
C6H5F |
120 |
29 |
3 |
2a |
0.5 |
THF |
120 |
26 |
4 |
2a |
0.5 |
Neat |
8 |
99 |
5 |
2b |
0.5 |
Neat |
8 |
85 |
6 |
LNNPZnH |
1 |
Neat |
120 |
Trace |
7 |
NHC |
1 |
Neat |
120 |
Trace |
Subsequently, reactions of a variety of nitriles with HBpin promoted by complex 2a were examined under our typical conditions (0.5 mol% catalyst loading, room temperature, in the absence of any solvent; Table 2). Reactions of para- or ortho-methyl-substituted benzonitriles with HBpin offered the corresponding diboronated amines 5b and 5c in 93% and 86% isolated yields, respectively. Additionally, benzonitriles containing polar functional groups are tolerated in the reaction. For example, methoxy, dimethylamino, halogen (F, Cl, and Br) substituted benzonitriles gave corresponding hydroboration products 5d–5h in 5–40 min with high isolated yields. Notably, the reaction was significantly accelerated when using para-trifluoromethyl substituted benzonitrile, leading to a full conversion of substrate only in 2 min. As a result, it afforded compound 5i with a TOF of 3000 h−1, which is the maximum value reported to date in nitrile hydroboration.7c,10b For polycyclic 1-naphthonitrile, the quantitative conversion was observed in 40 min, producing 5j in 90% isolated yield. Heterocyclic aromatic substrates comprised of a furan, thiophene or pyridine framework are applicable in current study to give anticipated dihydroboration products 5k–5m. The selectivity in the reduction of the nitriles when containing other unsaturated functional groups was also investigated. In the case of 4-acetylbenzonitrile, hydroboration of both C
O and C
N groups occurred at a comparable rate, and only 5n was isolated. In contrast, methyl 4-cyanobenzoate underwent selective hydroboration reaction only at C
N moiety even with excess amount of HBpin, affording compound 5o and keeping ester motif intact. Moreover, an intermolecular competition reaction between benzonitrile, styrene, and HBpin (1
:
1:2 molar ratio) was examined under above conditions. The hydroboration product 5a was exclusively formed while the styrene remained intact, thus indicating a preference of nitrile reduction over the alkene in the reaction. Furthermore, the scope of the substrates was expanded to the more challenging aliphatic nitriles. As shown in Table 2, all three selected nitriles were also successfully converted to the target borylamines 5p–5r, although the reactions required an elevated temperature (80 °C) and a prolonged reaction time (240 min) to achieve a complete conversion of nitriles.
Table 2 Hydroboration of various organic nitriles with HBpin catalyzed by 2aa
Reaction conditions: [2a] = 0.0015 mmol, [HBpin] = 0.63 mmol, [R-CN] = 0.30 mmol, room temperature. Conversions were determined by 1H NMR spectroscopy (integration of residual 3 vs. 5). Isolated yields were shown in the parentheses. 3.1 eq. HBpin was used. Reaction was conducted at 80 °C. |
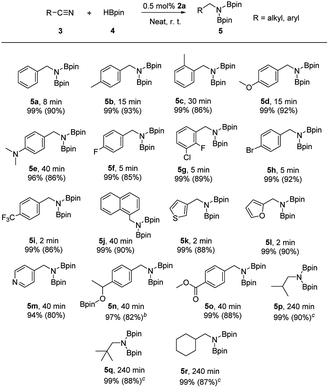 |
Inspired by the excellent performance of complex 2a in the hydroboration of C
N bond, we next examined its application in the imine hydroboration, which provides an efficient method for the synthesis of secondary amines.18 It was found that 2a also showed high activity toward reduction of a variety of imines with HBpin under neat condition, affording hydroborated product 7 at room temperature (Table 3). Reaction of N-benzylideneaniline with 1.1 equiv. of HBpin gave 7a in the presence of 0.5 mol% of zinc dihydride (2a), with complete consumption of imine in 15 min (TOF = 400 h−1).19 Subsequently, a wide range of C- and N-substituted imines were subjected to the reactions. For the imines bearing diverse substituents at C-phenyl ring, the expected hydroborated amines 7b–7h could be obtained in excellent isolated yields (82–94%) from 10 to 40 min. Among them, substrates with electron-withdrawing groups (–Cl, –Br, –CF3 or –NO2) proceeded rapidly in comparison to those with electron-donating groups (–OMe or –NMe2). Introduction of the substitute at N-phenyl of imine, either p-Cl or p-Me, slightly suppressed the rate of hydroboration reactions as in the cases of generation of 7i and 7j. Aliphatic N-substituted imines are also tolerated with current catalytic system and produced corresponding products 7k–7m, albeit requiring an elevated temperature (80 °C) and a prolonged reaction time (6–16 h). Heterocyclic C-substituted imine was applied under the given conditions, providing boronated aniline 7n in 40 min. When introducing an ester group to C-phenyl of imine, hydroboration reaction still occurred at C
N moiety even with excess amount of HBpin, affording compound 7o with 82% isolated yield.
Table 3 Hydroboration of various imines with HBpin catalyzed by 2aa
Reaction conditions: [2a] = 0.0015 mmol, [HBpin] = 0.33 mmol, [imine] = 0.30 mmol, room temperature. Conversions were determined by 1H NMR spectroscopy (integration of residual 6 vs. 7). Isolated yields were shown in the parentheses. Reaction was conducted at 80 °C. |
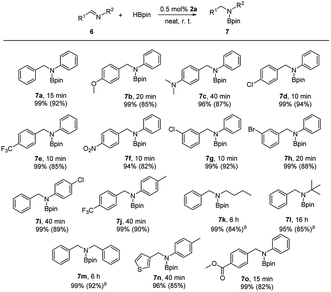 |
To gain more insights into the reaction mechanism, we conducted a stoichiometric reaction between the zinc dihydrides 2a and two equimolar amounts of p-MePhCN in toluene. The reaction rapidly occurred and gave complex 8 in 93% isolated yield (Scheme 1) through the insertion of one Zn–H into the –C
N moiety. It was fully characterized by multinuclear NMR spectroscopy and elemental analysis. Addition of an excessive amount of the nitrile did not lead to a further insertion reaction probably due to the steric hindrance. The 1H NMR spectrum showed a sharp signal at δ 9.39 ppm which was identified as a characteristic imine N
CH-R, while the signal of remaining Zn–H was located at δ 4.81 ppm. This was further confirmed by a control experiment using deuterium labeling complex 2a-D as a starting materiel (see the ESI for details†). Subsequently, complex 8 was directly employed as a catalyst for the reaction of p-MePhCN with pinacolborane under standard conditions. As expected, it efficiently provided the diboronated amine p-MePhCH2N(Bpin)2 (5b) in 88% isolated yield (Scheme 1), suggesting that complex 8 behaved as an active intermediate in the hydroboration of nitriles. Attempt to isolate other intermediate species by a stoichiometric reaction of complex 8 with pinacolborane failed because of the immediate generation of the corresponding diboronated amine 5b.
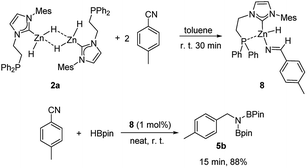 |
| Scheme 1 Synthesis of complex 8 and its use in the hydroboration. | |
Kinetic studies of the catalytic reactions were also performed in the presence of catalyst 2a and monitored by 1H NMR spectra (see the ESI for details†). Initial reactions were carried out using incremental catalyst starting concentrations in conjunction with a 1
:
2.1 molar ratio of p-MePhCN to HBpin. As shown in Fig. 3, plots of ln[(p-MePhCN)t/(p-MePhCN)0] versus time for all trials exhibited good straight lines, displaying a first-order kinetic behavior. As a result, pseudo-second order kinetic behavior on catalyst 2a was obtained (Fig. 4) which is distinct with that in the zinc alkyl-catalyzed hydroboration of nitrile.15 When large excess of p-MePhCN (5 equivalents) was used, a first-order dependence on HBpin was observed (ESI, Fig. S115†). A kinetic isotope effect (KIE, kH/kD) of 2.0 was obtained when replacement of HBpin by DBpin as the reductant in the reaction (ESI, Fig. S118†). In addition, a competitive deuterium labeling experiment using p-MePhCN, HBpin, and DBpin in a 1
:
2.1
:
2.1 molar ratio under our typical conditions was also conducted and showed a KIE value of 3.1 (ESI, Fig. S119†). The experimental evidence herein implied that the B–H bond breaking processes might be the rate-determining step.7a,10b
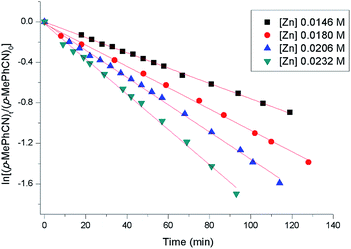 |
| Fig. 3 Plots of ln[(p-MePhCN)t/(p-MePhCN)0] versus time for the hydroboration of p-MePhCN by catalyst 2a. Reaction conditions: [p-MePhCN]0 = 0.40 M, [HBpin]0 = 0.84 M, [2a]0 = 0.0146–0.0232 M. | |
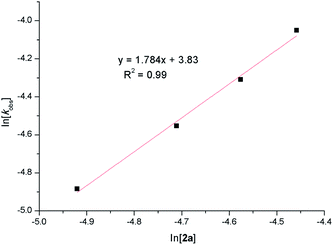 |
| Fig. 4 Plots of ln kobs vs. ln[2a] for the hydroboration of p-MePhCN by catalyst 2a. | |
Based on our observations and previous literature reports,7a,8c,15 a plausible mechanistic framework for zinc dihydrides-catalyzed nitrile hydroboration is depicted in Scheme 2. The reaction of zinc dihydride 2 with nitrile gave single insertion product 8, which then reacted with one molecule of HBpin to possibly give a zinc mixed hydride/amino 9. Finally, complex 9 underwent a σ-bond metathesis reaction with another molecule of HBpin to afford final product 5 with the regeneration of catalyst 2.
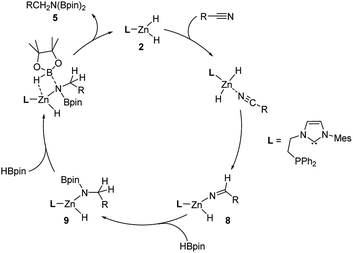 |
| Scheme 2 The proposed mechanism of hydroboration of nitriles catalyzed by 2. | |
Conclusions
In summary, the double hydroboration of nitriles to the corresponding diboronated amines have been achieved with broad substrate scope by using a molecular zinc dihydride based on NHC ligand at room temperature under solvent-free conditions. Complex 2a showed extremely high activity for this transformation, affording TOFs of up to 3000 h−1. In addition, complex 2a was also effective for the hydroboration of imines to boronated amines. The stoichiometric reaction of 2a with a nitrile led to the isolation of a single Zn–H insertion product 8, which was proved to be an active intermediate in the catalysis. Kinetic studies revealed a pseudo second-order dependence on the catalyst concentration. Further mechanistic studies and application to other catalytic reactions of this molecular zinc dihydride are underway in our laboratory.
Conflicts of interest
There are no conflicts to declare.
Acknowledgements
This work was supported by the National Natural Science Foundation of China (21871204).
Notes and references
-
(a) L. A. Oro, D. Carmona and J. M. Fraile, Hydrogenation reactions, in Metal-Catalysis in Industrial Organic Processes, ed. G. P. Chiusoli and P. M. Maitlis, RSC Publishing, London, 2006 Search PubMed;
(b) A. Ricci, Amino Group Chemistry: From Synthesis to Life Sciences, Wiley-VCH, Weinheim, 2008 Search PubMed.
-
(a) K. S. Hayes, Industrial processes for manufacturing amines, Appl. Catal., A, 2001, 221, 187–195 CrossRef CAS;
(b) J. S. Carey, D. Laffan, C. Thomson and M. T. Williams, Analysis of the reactions used for the preparation of drug candidate molecules, Org. Biomol. Chem., 2006, 4, 2337–2347 RSC;
(c) M. Pelckmans, T. Renders, S. Van de Vyver and B. F. Sels, Bio-based amines through sustainable heterogeneous catalysis, Green Chem., 2017, 19, 5303–5331 RSC.
- C. C. Chong and R. Kinjo, Catalytic hydroboration of carbonyl derivatives, imines, and carbon dioxide, ACS Catal., 2015, 5, 3238–3259 CrossRef CAS.
- Selected examples:
(a) M. Suginome, L. Uehlin and M. Murakami, Aminoboranes as “compatible” iminium ion generators in aminative C–C bond formations, J. Am. Chem. Soc., 2004, 126, 13196–13197 CrossRef CAS;
(b) E. Chong and S. A. Blum, Aminoboration: addition of B–N σ bonds across C–C π bonds, J. Am. Chem. Soc., 2015, 137, 10144–10147 CrossRef CAS;
(c) K. Yuan and S. Wang, trans-Aminoboration across internal alkynes catalyzed by B(C6F5)3 for the synthesis of borylated indoles, Org. Lett., 2017, 19, 1462–1465 CrossRef CAS;
(d) G. P. Junor, E. A. Romero, X. Chen, R. Jazzar and G. Bertrand, Readily available primary aminoboranes as powerful reagents for aldimine synthesis, Angew. Chem., Int. Ed., 2019, 58, 2875–2878 CrossRef CAS.
- A. Y. Khalimon, P. Farha, L. G. Kuzmina and G. I. Nikonov, Catalytic hydroboration by an imido-hydrido complex of Mo(IV), Chem. Commun., 2012, 48, 455–457 RSC.
-
(a) M. Itazaki and H. Nakazawa, Selective double addition reaction of an E–H bond (E = Si, B) to a C≡N triple bond of organonitriles, Molecules, 2018, 23, 2769 CrossRef;
(b) D. Hayrapetyan and A. Y. Khalimon, Catalytic nitrile hydroboration: a route to N,N-diborylamines and uses thereof, Chem.–Asian J., 2020, 15, 2575–2587 CrossRef CAS.
-
(a) C. Weetman, M. D. Anker, M. Arrowsmith, M. S. Hill, G. Kociok-Köhn, D. J. Liptrot and M. F. Mahon, Magnesium-catalysed nitrile hydroboration, Chem. Sci., 2016, 7, 628–641 RSC;
(b) J. Li, M. Luo, X. Sheng, H. Hua, W. Yao, S. A. Pullarkat, L. Xu and M. Ma, Unsymmetrical β-diketiminate magnesium(I) complexes: syntheses and application in catalytic hydroboration of alkyne, nitrile and carbonyl compounds, Org. Chem. Front., 2018, 5, 3538–3547 RSC;
(c) D. Bedi, A. Brar and M. Findlater, Transition metal- and solvent-free double hydroboration of nitriles, Green Chem., 2020, 22, 1125–1128 RSC;
(d) P. Ghosh and A. Jacobi von Wangelin, Lithium amide catalyzed hydroboration of nitriles, Org. Chem. Front., 2020, 7, 960–966 RSC.
-
(a) Y. Ding, X. Ma, Y. Liu, W. Liu, Z. Yang and H. W. Roesky, Alkylaluminum complexes as precatalysts in hydroboration of nitriles and carbodiimides, Organometallics, 2019, 38, 3092–3097 CrossRef CAS;
(b) A. Harinath, J. Bhattacharjee and T. K. Panda, Catalytic hydroboration of organic nitriles promoted by aluminum complex, Adv. Synth. Catal., 2019, 361, 850–857 CrossRef CAS;
(c) W. Liu, Y. Ding, D. Jin, Q. Shen, B. Yan, X. Ma and Z. Yang, Organic aluminum hydrides catalyze nitrile hydroboration, Green Chem., 2019, 21, 3812–3815 RSC;
(d) N. Sarkar, S. Bera and S. Nembenna, Aluminum-catalyzed selective hydroboration of nitriles and alkynes: a multifunctional catalyst, J. Org. Chem., 2020, 85, 4999–5009 CrossRef CAS.
- Selected examples:
(a) J. B. Geri and N. K. Szymczak, A proton-switchable bifunctional ruthenium complex that catalyzes nitrile hydroboration, J. Am. Chem. Soc., 2015, 137, 12808–12814 CrossRef CAS;
(b) A. Y. Khalimon, P. M. Farha and G. I. Nikonov, Imido-hydrido complexes of Mo(IV): catalysis and mechanistic aspects of hydroboration reactions, Dalton Trans., 2015, 44, 18945–18956 RSC;
(c) A. Kaithal, B. Chatterjee and C. Gunanathan, Ruthenium-catalyzed selective hydroboration of nitriles and imines, J. Org. Chem., 2016, 81, 11153–11161 CrossRef CAS;
(d) G. Nakamura, Y. Nakajima, K. Matsumoto, V. Srinivas and S. Shimada, Nitrile hydroboration reactions catalysed by simple nickel salts, bis(acetylacetonato)nickel(II) and its derivatives, Catal. Sci. Technol., 2017, 7, 3196–3199 RSC;
(e) A. D. Ibrahim, S. W. Entsminger and A. R. Fout, Insights into a chemoselective cobalt catalyst for the hydroboration of alkenes and nitriles, ACS Catal., 2017, 7, 3730–3734 CrossRef CAS;
(f) M. Ito, M. Itazaki and H. Nakazawa, Selective double hydroboration and dihydroborylsilylation of organonitriles by an iron-indium cooperative catalytic system, Inorg. Chem., 2017, 56, 13709–13714 CrossRef CAS;
(g) H. Ben-Daat, C. L. Rock, M. Flores, T. L. Groy, A. C. Bowman and R. J. Trovitch, Hydroboration of alkynes and nitriles using an α-diimine cobalt hydride catalyst, Chem. Commun., 2017, 53, 7333–7336 RSC;
(h) T. Kitano, T. Komuro and H. Tobita, Double and single hydroboration of nitriles catalyzed by a ruthenium-bis(silyl)xanthene complex: application to one-pot synthesis of diarylamines and N-arylimines, Organometallics, 2019, 38, 1417–1420 CrossRef CAS;
(i) C. Ghosh, S. Kim, M. R. Mena, J.-H. Kim, R. Pal, C. L. Rock, T. L. Groy, M.-H. Baik and R. J. Trovitch, Efficient cobalt catalyst for ambient-temperature nitrile dihydroboration, the elucidation of a chelate-assisted borylation mechanism, and a new synthetic route to amides, J. Am. Chem. Soc., 2019, 141, 15327–15337 CrossRef CAS;
(j) T. T. Nguyen, J.-H. Kim, S. Kim, C. Oh, M. Flores, T. L. Groy, M.-H. Baik and R. J. Trovitch, Scope and mechanism of nitrile dihydroboration mediated by a β-diketiminate manganese hydride catalyst, Chem. Commun., 2020, 56, 3959–3962 RSC;
(k) J. Pecak, W. Eder, B. Stöger, S. Realista, P. N. Martinho, M. J. Calhorda, W. Linert and K. Kirchner, Synthesis, characterization, and catalytic reactivity of {CoNO}8PCP pincer complexes, Organometallics, 2020, 39, 2594–2601 CrossRef CAS;
(l) K. A. Gudun, A. Slamova, D. Hayrapetyan and A. Y. Khalimon, Efficient Co-catalyzed double hydroboration of nitriles: application to one-pot conversion of nitriles to aldimines, Chem.–Eur. J., 2020, 26, 4963–4968 CrossRef CAS.
-
(a) Z. Huang, S. Wang, X. Zhu, Q. Yuan, Y. Wei, S. Zhou and X. Mu, Well-defined amidate-functionalized N-heterocyclic carbene-supported rare-earth metal complexes as catalysts for efficient hydroboration of unactivated imines and Nitriles, Inorg. Chem., 2018, 57, 15069–15078 CrossRef CAS;
(b) S. Saha and M. S. Eisen, Catalytic recycling of a Th–H bond via single or double hydroboration of inactivated imines or nitriles, ACS Catal., 2019, 9, 5947–5956 CrossRef CAS.
-
(a) P. A. Lummis, M. R. Momeni, M. W. Lui, R. McDonald, M. J. Ferguson, M. Miskolzie, A. Brown and E. Rivard, Accessing zinc monohydride cations through coordinative interactions, Angew. Chem., Int. Ed., 2014, 53, 9347–9351 CrossRef CAS;
(b) M. J. C. Dawkins, E. Middleton, C. E. Kefalidis, D. Dange, M. M. Juckel, L. Maron and C. Jones, Two-coordinate terminal zinc hydride complexes: synthesis, structure and preliminary reactivity studies, Chem. Commun., 2016, 52, 10490–10492 RSC.
- D. Mukherjee, A.-K. Wiegand, T. P. Spaniol and J. Okuda, Zinc hydridotriphenylborates supported by a neutral macrocyclic polyamine, Dalton Trans., 2017, 46, 6183–6186 RSC.
-
(a) S. Mandal, S. Mandal and K. Geetharani, Zinc-catalysed hydroboration of terminal and internal alkynes, Chem.–Asian J., 2019, 14, 4553–4556 CrossRef CAS;
(b) R. J. Procter, M. Uzelac, J. Cid, P. J. Rushworth and M. J. Ingleson, Low-coordinate NHC-zinc hydride complexes catalyze alkyne C–H borylation and hydroboration using pinacolborane, ACS Catal., 2019, 6, 5760–5771 CrossRef;
(c) M. Uzelac, K. Yuan and M. J. Ingleson, A comparison of two zinc hydride catalysts for terminal alkyne C–H borylation/hydroboration and the formation of 1,1,1-triborylalkanes by tandem catalysis using Zn–H and B–H compounds, Organometallics, 2020, 39, 1332–1338 CrossRef CAS.
-
(a) J. L. Lortie, T. Dudding, B. M. Gabidullin and G. I. Nikonov, Zinc-catalyzed hydrosilylation and hydroboration of N-Heterocycles, ACS Catal., 2017, 7, 8454–8459 CrossRef CAS;
(b) K. K. Krishnan, S. M. Ujwaldev, S. Saranya, G. Anilkumar and M. Beller, Recent advances and perspectives in the synthesis of heterocycles via zinc catalysis, Adv. Synth. Catal., 2019, 361, 382–404 CrossRef;
(c) X. Wang, Y. Zhang, D. Yuan and Y. Yao, Regioselective hydroboration and hydrosilylation of N-heteroarenes catalyzed by a zinc alkyl complex, Org. Lett., 2020, 22, 5695–5700 CrossRef CAS.
- S. Das, J. Bhattacharjee and T. K. Panda, An imidazolin-2-iminato ligand organozinc complex as a catalyst for hydroboration of organic nitriles, New J. Chem., 2019, 43, 16812–16818 RSC.
- X. Wang, K. Chang and X. Xu, Hydroboration of carbon dioxide enabled by molecular zinc dihydrides, Dalton Trans., 2020, 49, 7324–7327 RSC.
- M. Chen, S. Jiang, L. Maron and X. Xu, Transition metal-induced dehydrogenative coupling of zinc hydrides, Dalton Trans., 2019, 48, 1931–1935 RSC.
- Selected examples for the hydroboration of imines:
(a) R. T. Baker, J. C. Calabrese and S. A. Westcott, Coinage metal-catalyzed hydroboration of imines, J. Organomet. Chem., 1995, 498, 109–117 CrossRef CAS;
(b) C. M. Vogels, P. E. O'Connor, T. E. Phillips, K. J. Watson, M. P. Shaver, P. G. Hayes and S. A. Westcott, Rhodium-catalyzed hydroborations of allylamine and allylimines, Can. J. Chem., 2001, 79, 1898–1905 CrossRef CAS;
(c) P. Eisenberger, A. M. Bailey and C. M. Crudden, Taking the F out of FLP: simple Lewis acid–base pairs for mild reductions with neutral boranes via borenium ion catalysis, J. Am. Chem. Soc., 2012, 134, 17384–17387 CrossRef CAS;
(d) M. Arrowsmith, M. S. Hill and G. Kociok-Köhn, Magnesium catalysis of imine hydroboration, Chem.–Eur. J., 2013, 19, 2776–2783 CrossRef CAS;
(e) R. Arévalo, C. M. Vogels, G. A. MacNeil, L. Riera, J. Pérez and S. A. Westcott, Rhenium-catalysed hydroboration of aldehydes and aldimines, Dalton Trans., 2017, 46, 7750–7757 RSC;
(f) Q. Yin, Y. Soltani, R. L. Melen and M. Oestreich, BArF3-catalyzed imine hydroboration with pinacolborane not requiring the assistance of an additional Lewis base, Organometallics, 2017, 36, 2381–2384 CrossRef CAS;
(g) V. A. Pollard, M. Á. Fuentes, A. R. Kennedy, R. McLellan and R. E. Mulvey, Comparing neutral (monometallic) and anionic (bimetallic) aluminum complexes in hydroboration catalysis: influences of lithium cooperation and ligand set, Angew. Chem., Int. Ed., 2018, 57, 10651–10655 CrossRef CAS;
(h) B. S. N. Huchenski and A. W. H. Speed, Protic additives or impurities promote imine reduction with pinacolborane, Org. Biomol. Chem., 2019, 17, 1999–2004 RSC;
(i) D. Yan, X. Wu, J. Xiao, Z. Zhu, X. Xu, X. Bao, Y. Yao, Q. Shen and M. Xue, n-Butyllithium catalyzed hydroboration of imines and alkynes, Org. Chem. Front., 2019, 6, 648–653 RSC;
(j) V. K. Pandey, S. N. R. Donthireddy and A. Rit, Catalyst-free and solvent-free facile hydroboration of imines, Chem.–Asian J., 2019, 14, 3255–3258 CrossRef CAS.
- Hydroboration of N-benzylideneaniline with HBpin occurred under catalyst-free and solvent-free conditions, however, prolonged reaction time (6 h) was required to achieve a high yield. For comparisons, see ref. 18j.
Footnote |
† Electronic supplementary information (ESI) available. See DOI: 10.1039/d0ra09648b |
|
This journal is © The Royal Society of Chemistry 2021 |
Click here to see how this site uses Cookies. View our privacy policy here.