DOI:
10.1039/D0RA09400E
(Paper)
RSC Adv., 2021,
11, 3143-3152
Synthesis of new pyridines with sulfonamide moiety via a cooperative vinylogous anomeric-based oxidation mechanism in the presence of a novel quinoline-based dendrimer-like ionic liquid†
Received
4th November 2020
, Accepted 30th November 2020
First published on 22nd January 2021
Abstract
In the present study, we reported the synthesis of a novel quinoline-based dendrimer-like ionic liquid. After characterization of the mentioned ionic liquid with suitable techniques such as Fourier transform infrared spectroscopy (FT-IR), energy dispersive X-ray spectroscopy (EDX), elemental mapping, thermogravimetric analysis (TGA) and derivative thermogravimetry (DTG), its catalytic performance was investigated in the synthesis of new pyridines with sulfonamide moiety via a cooperative vinylogous anomeric-based oxidation mechanism under mild reaction conditions. All target molecules were achieved in short reaction times and high yields.
Introduction
Ionic liquids (ILs) offer various promising capabilities which guarantee their applications in different technologies such as synthesis, catalysis, electrochemistry, medicine, extractants, surfactants, materials science and chemical engineering.1–7 Furthermore, ILs have shown excellent outcomes in the field of energy and environmental science.8,9 For example, an organic amine-based gelled room temperature IL, has been applied as a containment and decontaminating coating substrate for blister agent-contacted materials.10 Also, a task specific IL nanocomposite has been used for decontamination of water and soil from heavy metal ions such as Pb(II) and Cd(II).11 IL enhanced features include rational designable and superior synthetic flexibility, thermal and chemical stability, low vapor pressure and exceptional conductivity. These promising characteristics have led to ILs becoming a hot research field for multidisciplinary scientists.12–15
The sulfonamide structural kernel (also known as sulfa drugs), is a prominent core in medicinal chemistry as compounds bearing this functional group exhibit varied pharmaceutical effects. For example, several bioactive molecules containing sulfonamide group were used as inhibitors of HIV protease, antibacterials, antibiotic, antitumor, antihypertensive, anticonvulsants and antifungal agents. The biological importance of sulfonamide containing drugs are well demonstrated in the literatures.16–25 Some sulfonamide drugs are portrayed in Scheme 1.
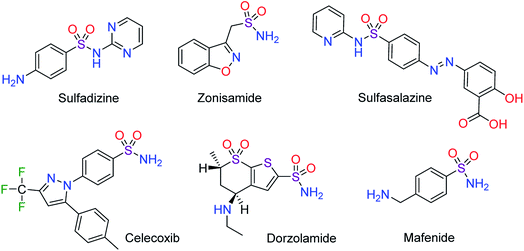 |
| Scheme 1 Selected examples of drugs with sulfonamide structural core. | |
Another prominent structure core, which ubiquitous in biologically active compounds, is pyridine motifs. Pyridines have a key role in the biological behavior of several natural molecules and also, they are responsible for the pharmaceutical activity of various influential drugs.26–29 In addition, pyridine derivatives represent numerous capabilities in diverse areas such as catalysis, coordination chemistry, polymer science, materials and surfaces engineering and agrochemistry.30–34 Therefore, pyridine and its derivatives (particularly 2-aminonicotinonitrile) have received extensive consideration and several efficacious methods have been developed by synthetic and medicinal chemists for their preparation.35–51
So, it is predicted that multicomponent synthesis52–56 of a single molecule bearing sulfonamide and pyridine centers simultaneously gives unique features to that structure inherited characteristics of both cores.
In addition to oxygen atom, which is responsible for anomeric interactions in various molecules,57–62 in non-oxygen heterocycles, other atoms such as nitrogen and sulfur play the role of donors in anomeric systems. For example, as depicted in Scheme 2, due to stereoelectronic factors, in the case of 1,3,5-tri-alkylhexahydro-1,3,5-triazines, as a conformationally biased system even a bulky group such as tert-butyl occupied axial position (up to 85%).63,64 In this situation, the anomeric interaction of nitrogen lone pairs with vacant antibonding orbitals of carbon–nitrogen bonds (nN → σ*C–N) is possible.
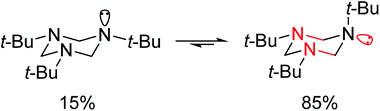 |
| Scheme 2 Extension of anomeric effect to 1,3,5-tri-alkylhexahydro-1,3,5-triazines. | |
In vinylogous anomeric effect, the anomeric interaction is transmitted from a donor through C
C bonds to an acceptor.65 One of the striking examples for the vinylogous anomeric effect can be found in Claisen rearrangement. The existence of γ-allylic O-atom within the structure leads to acceleration of the reaction rate (Scheme 3).64a,66 In these case, non-bonding electrons of γ-allylic O-atom interact with antibonding of C–O bond trough C
C bond.
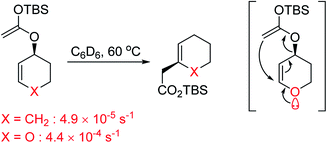 |
| Scheme 3 The existence of the γ-allylic O-atom leads to acceleration of the Claisen rearrangement rate. | |
In this investigation, we continued our previous efforts for the synthesis of pyridine derivatives via anomeric or cooperative vinylogous anomeric-based oxidation mechanism67–73 and reported the preparation of a new series of pyridines with sulfonamide moiety in the presence of a novel quinoline-based dendrimer-like ionic liquid TQoxyTtriFA (Schemes 4 and 5).
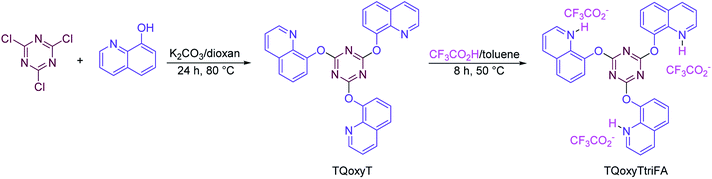 |
| Scheme 4 Synthetic route to TQoxyTtriFA as a novel quinoline-based dendrimer-like ionic liquid. | |
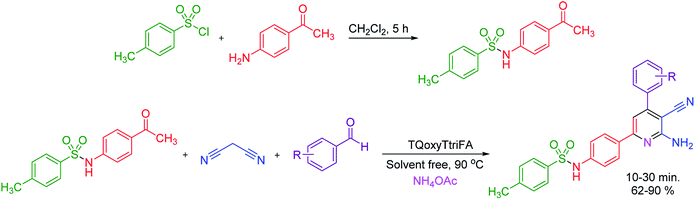 |
| Scheme 5 Synthesis of new pyridines with sulfonamide moiety in the presence of TQoxyTtriFA. | |
Results and discussion
The systematic study of the anomeric effect in target biological candidated molecules allows the design of synthetic strategies based on anomerically driven stereoselective reactions, or highly biased equilibria among isomeric products. To the best of our knowledge, many biological processes involve oxidation–reduction of substrates by NAD+/NADH and/or NADP+/NADPH systems, respectively. It is believed that the key feature of the oxidation mechanism in biological systems is the hydride transfer from carbon via anomeric-based oxidation.74–78 Thus, development of anomeric-based oxidation and/or aromatization mechanism leads to knowledge-based designing of biomimetic reactions in the future. With this aim, we decided to synthesize a range of new diversity of pyridines with sulfonamide moiety in the presence of a novel quinoline-based dendrimer-like ionic liquid.
The structure of the prepared novel quinoline-based dendrimer-like ionic liquid, namely TQoxyTtriFA was analyzed by several methods, which all endorsed each other. The more detailed data can be found in following.
The FT-IR spectrum of the catalyst verified the existence of characteristic functional groups including C
O (1678 cm−1) and acidic proton within the structure (3446 cm−1). Also, 1H NMR spectrum is in agreement with the structure and confirms the symmetrical structure of the catalyst. This claim is in full agreement with the results obtained from 13C NMR (for detail information, please see Spectral data section and ESI†).
Fig. 1 depicts the output data of EDX analysis, which confirmed the elemental content of TQoxyTtriFA. The anticipated elements are carbon, nitrogen, oxygen and fluorine. Also, more verification was accomplished by applying the elemental mapping analysis (Fig. 2).
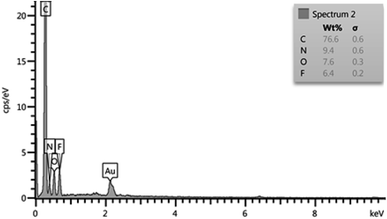 |
| Fig. 1 Output data of EDX analysis of TQoxyTtriFA. | |
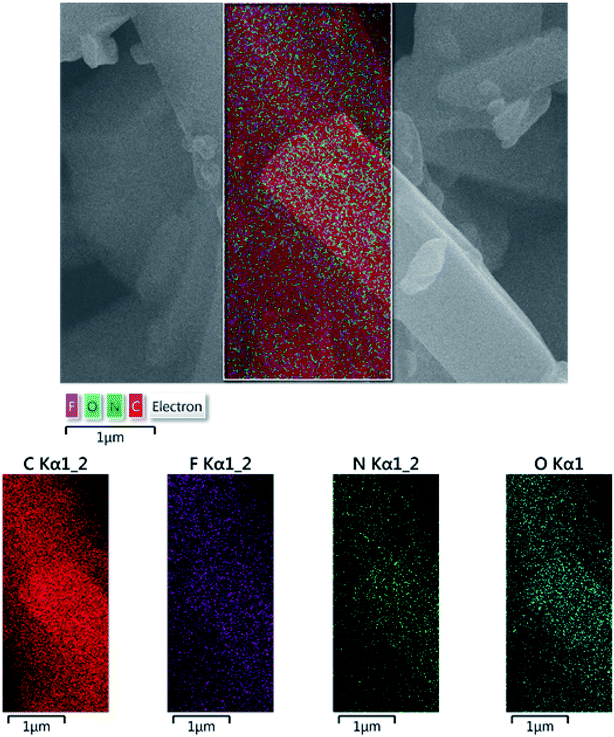 |
| Fig. 2 Elemental mapping analysis of TQoxyTtriFA. | |
In a separate study, the thermal behavior of the prepared TQoxyTtriFA was investigated from 0 °C up to 600 °C. The obtained TG and DTG plots are illustrated in Fig. 3. The weight loss occurred at around 150 °C, indicating the high thermal stability of TQoxyTtriFA.
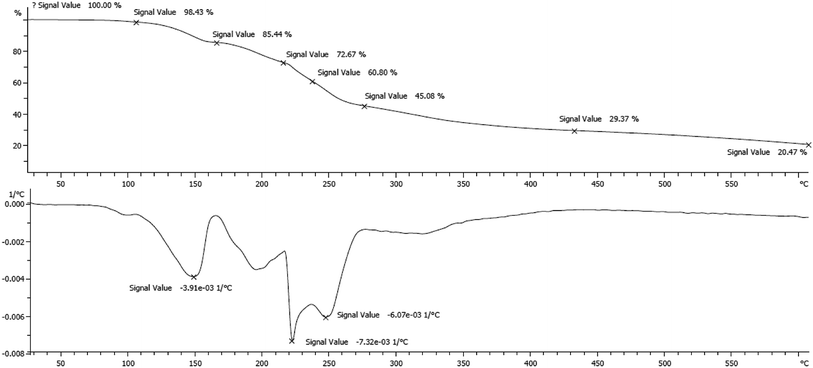 |
| Fig. 3 TG and DTG plots of TQoxyTtriFA. | |
To prove the catalytic activity of TQoxyTtriFA as a novel quinoline-based dendrimer-like ionic liquid, we examined it for the synthesis of a new pyridine series containing sulfonamide moiety. Our study began by examining the best reaction conditions by selecting a model reaction for the synthesis of molecule 1a. Our obtained data reveal that 90 °C and solvent free conditions are the optimal reaction parameters. The data are collected in Table 1.
Table 1 Optimizing of the reaction conditions for the synthesis of 1a
a
Entry |
Solvent |
Temperature (°C) |
Load of catalyst (mol%) |
Time (min) |
Yieldb (%) |
Reaction condition: benzaldehyde (1 mmol, 0.106 g), malononitrile (1 mmol, 0.066 g), N-(4-acetylphenyl)-4-methylbenzenesulfonamide (1 mmol, 0.289 g) and ammonium acetate (1 mmol, 0.077 g). Isolated yields. The achieved data from optimization of described reaction under air, argon and nitrogen atmospheres are similar. |
1 |
— |
100 |
2 |
15 |
84 |
2 |
— |
90 |
5 |
15 |
82 |
3c |
— |
90 |
2 |
15 |
80 |
4 |
|
90 |
1 |
20 |
71 |
5 |
— |
90 |
— |
15 |
Trace |
6 |
— |
90 |
— |
120 |
52 |
7 |
— |
80 |
2 |
20 |
72 |
8 |
— |
70 |
2 |
45 |
60 |
9 |
— |
60 |
2 |
15 |
20 |
10 |
— |
60 |
2 |
60 |
55 |
11 |
H2O |
Reflux |
2 |
120 |
— |
12 |
EtOH |
Reflux |
2 |
120 |
40 |
13 |
n-Hexane |
Reflux |
2 |
120 |
— |
14 |
EtOAc |
Reflux |
2 |
120 |
— |
15 |
CH2Cl2 |
Reflux |
2 |
120 |
— |
After determining the optimal conditions for the model reaction, we researched the productivity of the procedure for the synthesis of various pyridines containing sulfonamide moiety. The data, as collected in Table 2, show promising results. All new molecules were obtained in high yields at short reaction times via a catalytic one pot multicomponent reaction manner.
Table 2 Catalytic synthesis of a new library of pyridines with sulfonamide moietya
Reaction conditions: aryl aldehyde (1.0 mmol), malononitrile (1.0 mmol, 0.066 g), N-(4-acetylphenyl)-4-methylbenzenesulfonamide (1.0 mmol, 0.289 g) and ammonium acetate (1.0 mmol, 0.077 g), solvent free, 90 °C, catalyst = 2 mol%. Reported yields are referred to isolated yields. |
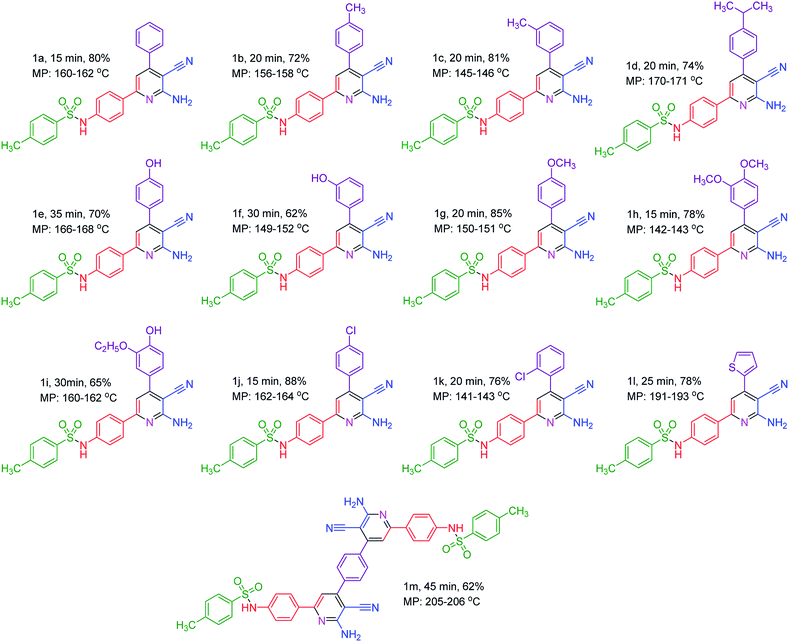 |
A plausible mechanism was suggested for the synthesis of 1a (Scheme 6). In this suggestion, Knoevenagel intermediate A (from the reaction of catalytic activated benzaldehyde and malononitrile) was treated with enamine intermediate B (from the reaction of in situ generated NH3 and N-(4-acetylphenyl)-4-methylbenzenesulfonamide). This catalytic process leads to the formation of intermediate C. Then, in the presence of the new described catalyst, intermediate C is converted to intermediate D, through a ring closer reaction. Afterwards, a tautomeric process leads to formation intermediate E. Finally, intermediate E undergoes a cooperative vinylogous anomeric-based oxidation (CVABO) mechanism for producing the target molecule 1a through unusual hydride transfer and releasing one molecular hydrogen (H2). The achieved data from optimization of described reaction under argon and nitrogen atmospheres verified our suggestion for oxidation and aromatization of intermediate E.73,74
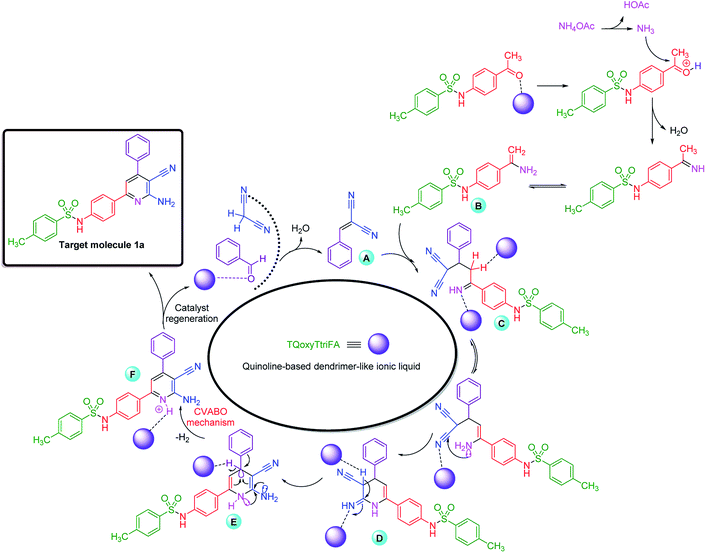 |
| Scheme 6 A catalytic plausible cooperative vinylogous anomeric-based oxidation (CVABO) mechanism for the synthesis of 1a. | |
Conclusion
Here, the synthesis and characterization of a novel quinoline-based dendrimer-like IL was reported. Several skills were applied for studding the structure and formation of the novel IL. Also, the catalytic performance of titled IL was successfully employed for the facile synthesis of a new pyridine series containing sulfonamide moiety. Also, a plausible mechanism for the synthesis of target sulfonamide containing pyridines through a vinylogous anomeric-based oxidation mechanism was proposed. We think that the obtained results from this research will support the idea of rational designs, syntheses and applications of tasked-specific catalysts, susceptible biological candidate molecules and biomimetic reactions for the development of anomeric-based oxidation and/or aromatization mechanism.
Materials and methods
Chemicals applied in this work for the synthesis of catalyst and products were supplied by Merck and Sigma-Aldrich chemical companies and used without further purification. Progress of the reactions was monitored by TLC using silica gel SIL G/UV 254 plates. Melting points were recorded on a Büchi B-545 apparatus in open capillary tubes. Fourier transform-infrared spectra (FT-IR) of the samples were recorded on a frontier FT-IR PerkinElmer with MID IR detector. The 1H NMR spectra (301 MHz) and 13C NMR (76 MHz) were recorded with a Bruker spectrometer or Varian Mercury 300 MHz Spectrometer (Varian Inv., Palo Alto, CA, USA) using CDCl3 or DMSOd6 as solvent. Thermal gravimetry analysis TG-DTG, were carried out on a STA 1500 from Rheometric Scientific at Day Petronic company. EDX and Elemental mapping analysis were performed using a SIGMA VP from Zeiss at Day Petronic company.
General procedure for the synthesis of TQoxyTtriFA as a novel quinoline-based dendrimer-like ionic liquid
At first, via the reaction of cyanuric chloride (1 eq.) and 8-hydroxy quinoline (3 eq.), the related intermediate TQoxyT was prepared.79 Afterwards, the reaction of TQoxyT (1 eq.) with trifluoroacetic acid (3 eq.) in toluene as solvent at room temperature leads to formation of TQoxyTtriFA as a novel quinoline-based dendrimer-like ionic liquid (Scheme 4).
General procedure for the synthesis of N-(4-acetylphenyl)-4-methylbenzenesulfonamide
Similar to reported procedure,80 N-(4-Acetylphenyl)-4-methylbenzenesulfonamide was prepared by the reaction of 4-aminoacetophenone (10.0 mmol, 1.35 g), 4-toluenesulfonyl chloride (10.0 mmol, 1.91 g) and 2 mol% of pyridine as catalyst in CH2Cl2 at room temperature for 5 h. Afterwards, the solvent was removed and the obtained solid was filtered and was thoroughly washed with water and air dried to afford the pure product (yields up to 88%).
General procedure for the synthesis of new pyridines with sulfonamide moiety in the presence of TQoxyTtriFA
The general protocol for the synthesis of sulfonamide containing pyridines are as follows. To a mixture of aryl aldehyde (1.0 mmol), malononitrile (1.0 mmol, 0.066 g), N-(4-acetylphenyl)-4-methylbenzenesulfonamide (1.0 mmol, 0.289 g) and NH4OAc (1.0 mmol, 0.077 g), 2 mol% of TQoxyTtriFA was added as catalyst. Then, the mixture was stirred and heated at 90 °C under solvent free conditions for appropriate times as reported in Table 2. The reaction progress and the purity of the molecules were checked out by TLC (n-hexane/ethylacetate (1
:
1). Eventually, the products were purified by using prepared silica plates using a mixture n-hexane/ethylacetate (1
:
1).
Spectral data
TQoxyTtriFA (quinoline-based dendrimer-like ionic liquid). MP = 94–96 °C, FT-IR (KBr, ν, cm−1): 3446, 3075, 1677, 1606, 1268. 1H NMR (301 MHz, DMSO) δppm 9.70 (bs, 1H, NH), 8.85 (dd, 3H, J = 4.3, 1.7 Hz, aromatic), 8.42 (dd, 3H, J = 8.4, 1.7 Hz, aromatic), 7.85 (dd, 3H, J = 7.4, 2.2 Hz, aromatic), 7.62–7.49 (m, 9H, aromatic). 13C NMR (76 MHz, DMSO) δ 174.1, 159.6, 159.1, 158.6, 158.2, 150.7, 147.2, 139.8, 137.2, 129.5, 126.9, 126.8, 122.7, 121.8, 117.5, 113.7.
N-(4-Acetylphenyl)-4-methylbenzenesulfonamide. MP = 196–198 °C, FT-IR (KBr, ν, cm−1): 3221, 3000, 1671, 1600, 1342, 1281, 1158, 912. 1H NMR (301 MHz, DMSO) δppm 10.18 (s, 1H, NH), 7.21 (d, 2H, J = 9 Hz, aromatic), 7.11 (d, 2H, J = 9 Hz, aromatic), 6.74 (d, 2H, J = 6 Hz, aromatic), 6.60 (d, 2H, J = 9 Hz, aromatic), 1.84 (s, 3H, CH3), 1.70 (s, 3H, CH3). 13C NMR (76 MHz, DMSO) δppm 197.0, 144.3, 142.9, 137.0, 132.5, 130.4, 130.4, 127.3, 118.4, 26.9, 21.5.
N-(4-(6-Amino-5-cyano-4-phenylpyridin-2-yl)phenyl)-4-methylbenzenesulfonamide (1a). MP = 160–162 °C, FT-IR (KBr, ν, cm−1): 3548, 3407, 3284, 2924, 2215, 1612, 1573, 1337, 1158, 921. 1H NMR (301 MHz, CDCl3) δppm 8.14–8.09 (m, 2H, aromatic), 7.96–7.91 (m, 2H, aromatic), 7.86–7.80 (m, 2H, aromatic), 7.76–7.71 (m, 3H, aromatic), 7.49–7.32 (m, 6H, aromatic and NH), 5.60 (bs, 2H, NH2), 2.61 (s, 3H, CH3). 13C NMR (76 MHz, CDCl3) δppm 160.3, 158.8, 155.4, 144.4, 138.6, 137.0, 136.1, 134.5, 130.1, 130.0, 129.1, 128.6, 128.3, 127.4, 120.8, 117.3, 111.0, 88.3, 21.7. TOF-HRMS (EI): m/z (M)+ + 1 calcd for C25H20N4O2S 441.1307; found 441.1199.
N-(4-(6-Amino-5-cyano-4-(p-tolyl)pyridin-2-yl)phenyl)-4-methylbenzenesulfonamide (1b). MP = 156–158 °C, FT-IR (KBr, ν, cm−1): 3521, 3396, 3276, 2929, 2217, 1610, 1577, 1339, 1159, 821. 1H NMR (301 MHz, CDCl3) δppm 8.01 (d, 2H, J = 9 Hz, aromatic), 7.86 (d, 2H, J = 9 Hz, aromatic), 7.66 (d, 2H, J = 9 Hz, aromatic), 7.47 (d, 2H, J = 9 Hz, aromatic), 7.38 (d, 2H, J = 9 Hz, aromatic), 7.32–7.29 (m, 3H, aromatic), 7.24 (s, 1H, NH), 5.51 (bs, 2H, NH2), 2.58 (s, 3H, CH3), 2.51 (s, 3H, CH3). 13C NMR (76 MHz, CDCl3) δppm 160.4, 158.7, 155.4, 144.3, 140.3, 138.6, 136.1, 134.6, 134.1, 129.9, 129.8, 128.6, 128.2, 127.4, 120.7, 117.4, 110.9, 88.2, 21.7, 21.5.
N-(4-(6-Amino-5-cyano-4-(m-tolyl)pyridin-2-yl)phenyl)-4-methylbenzenesulfonamide (1c). MP = 145–146 °C, FT-IR (KBr, ν, cm−1): 3520, 3389, 3273, 2982, 2218, 1611, 1553, 1336, 1158. 1H NMR (301 MHz, CDCl3) δppm 8.02 (d, 2H, J = 9 Hz, aromatic), 7.83 (d, 2H, J = 9 Hz, aromatic), 7.52–7.53 (m, 3H, aromatic), 7.45–7.44 (m, 1H, aromatic), 7.38–7.34 (m, 3H, aromatic), 7.29 (d, 2H, J = 9 Hz, aromatic), 7.23 (s, 1H, NH), 5.45 (bs, 2H, NH2), 2.57 (s, 3H, CH3), 2.50 (s, 3H, CH3). 13C NMR (76 MHz, CDCl3) δppm 160.2, 158.6, 155.4, 144.3, 138.5, 136.4, 134.5, 131.1, 130.7, 129.8, 128.9, 128.8, 128.5, 127.3, 125.3, 120.7, 117.2, 110.9, 88.3, 21.6, 21.5. TOF-HRMS (EI): m/z (M)+ + 1 calcd for C26H22N4O2S 455.1463; found 455.1349.
N-(4-(6-Amino-5-cyano-4-(4-isopropylphenyl)pyridin-2-yl)phenyl)-4-methylbenzenesulfonamide (1d). MP = 170–171 °C, FT-IR (KBr, ν, cm−1): 358, 3396, 3252, 2923, 2216, 1615, 1464, 1340, 1160, 818. 1H NMR (301 MHz, CDCl3) δppm 8.08 (d, 2H, J = 9 Hz, aromatic), 7.91 (d, 2H, J = 9 Hz, aromatic), 7.75 (d, 2H, J = 6 Hz, aromatic), 7.57 (d, 2H, J = 9 Hz, aromatic), 7.43 (d, 2H, J = 9 Hz, aromatic), 7.37–7.34 (m, 3H, aromatic), 7.31 (s, 1H, NH), 5.54 (bs, 2H, NH2), 3.18 (s, 1H, J = 6 Hz, CH), 2.57 (s, 3H, CH3), 1.50 (d, 6H, J = 6 Hz, CH3). 13C NMR (76 MHz, CDCl3) δppm 160.4, 158.7, 155.4, 151.2, 144.4, 138.6, 136.09, 134.7, 134.4, 130.0, 128.6, 128.3, 128.2, 127.4, 127.3, 120.8, 117.5, 111.0, 88.2, 34.2, 24.0, 21.7. TOF-HRMS (EI): m/z (M)+ + 1 calcd for C28H26N4O2S 483.1776; found 483.1651.
N-(4-(6-Amino-5-cyano-4-(4-hydroxyphenyl)pyridin-2-yl)phenyl)-4-methylbenzenesulfonamide (1e). MP = 166–168 °C, 1H NMR (301 MHz, DMSO) δppm 7.65–7.57 (m, 2H, aromatic), 7.39–7.32 (m, 2H, aromatic), 7.20–7.14 (m, 2H, aromatic), 7.03–6.96 (m, 3H, aromatic), 6.85–6.75 (m, 4H, aromatic), 6.60–6.54 (m, 2H, aromatic and NH), 6.46 (bs, 2H, NH2), 7.21–7.15 (m, 3H, CH3). 13C NMR (76 MHz, DMSO) δppm 161.6, 159.6, 158.5, 155.2, 143.5, 138.2, 137.3, 132.3, 130.5, 130.3, 128.8, 128.2, 127.4, 119.8, 118.3, 116.2, 108.9, 86.1, 21.6. TOF-HRMS (EI): m/z (M)+ + 1 calcd for C25H20N4O3S 457.1256; found 457.1143.
N-(4-(6-Amino-5-cyano-4-(3-hydroxyphenyl)pyridin-2-yl)phenyl)-4-methylbenzenesulfonamide (1f). MP = 149–152 °C, 1H NMR (301 MHz, DMSO) δppm 8.18–8.14 (m, 2H, aromatic), 7.92–7.87 (m, 2H, aromatic), 7.56–7.52 (m, 4H, aromatic), 7.38–7.29 (m, 4H, aromatic), 7.23–7.21 (m, 2H, aromatic), 7.13–7.07 (m, 3H, NH and NH2), 2.74–2.68 (m, 3H, CH3). 13C NMR (76 MHz, DMSO) δppm 161.4, 158.7, 158.2, 155.4, 143.4, 139.1, 138.5, 132.1, 130.5, 130.2, 128.8, 127.4, 119.8, 119.5, 117.8, 117.1, 115.68, 109.1, 86.4, 21.6.
N-(4-(6-Amino-5-cyano-4-(4-methoxyphenyl)pyridin-2-yl)phenyl)-4-methylbenzenesulfonamide (1g). MP = 150–151 °C, FT-IR (KBr, ν, cm−1): 3525, 3396, 3259, 2924, 2213, 1609, 1513, 1379, 1157, 829. 1H NMR (301 MHz, CDCl3) δppm 7.94 (d, 2H, J = 9 Hz, aromatic), 7.79 (d, 2H, J = 9 Hz, aromatic), 7.66 (d, 2H, J = 9 Hz, aromatic), 7.37–7.29 (m, 3H, aromatic), 7.26–7.21 (m, 2H, aromatic), 7.15 (s, 1H, NH), 7.10 (d, 2H, J = 6 Hz, aromatic), 5.42 (bs, 2H, NH2), 3.95 (s, 3H, OCH3), 2.44 (s, 3H, CH3). 13C NMR (76 MHz, CDCl3) δppm 161.1, 160.4, 158.6, 154.9, 144.2, 138.5, 136.1, 134.6, 129.9, 129.7, 129.2, 128.5, 127.4, 120.7, 117.5, 114.5, 110.7, 88.0, 55.5, 21.6. TOF-HRMS (EI): m/z (M)+ + 1 calcd for C26H22N4O3S 471.1413; found 471.1299.
N-(4-(6-Amino-5-cyano-4-(3,4-dimethoxyphenyl)pyridin-2-yl)phenyl)-4-methylbenzenesulfonamide (1h). MP = 142–143 °C, FT-IR (KBr, ν, cm−1): 3525, 3390, 3262, 2923, 2214, 1614, 1514, 1337, 1260, 1159, 816. 1H NMR (301 MHz, CDCl3) δppm 7.94 (d, 2H, J = 9 Hz, aromatic), 7.74 (d, 2H, J = 9 Hz, aromatic), 7.31–7.19 (m, 7H, aromatic), 7.15 (s, 1H, NH), 7.04 (d, 2H, J = 9 Hz, aromatic), 5.37 (bs, 2H, NH2), 4.0 (t, 6H, J = 1.5 Hz, OCH3), 2.42 (s, 3H, CH3). 13C NMR (76 MHz, CDCl3) δppm 160.4, 158.6, 155.0, 150.7, 149.2, 144.3, 138.5, 134.7, 129.9, 129.4, 128.6, 127.4, 121.2, 120.7, 117.9, 111.4, 111.3, 110.7, 56.26, 56.2, 21.7. TOF-HRMS (EI): m/z (M)+ + 1 calcd for C27H24N4O4S 501.1518; found 501.1393.
N-(4-(6-Amino-5-cyano-4-(3-ethoxy-4-hydroxyphenyl)pyridin-2-yl)phenyl)-4-methylbenzenesulfonamide (1i). MP = 160–162 °C, FT-IR (KBr, ν, cm−1): 3509, 3392, 3264, 2924, 2212, 1609, 1513, 1338, 1267, 1157, 821. 1H NMR (301 MHz, CDCl3) δppm 7.85 (d, 2H, J = 9 Hz, aromatic), 7.71 (d, 2H, J = 9 Hz, aromatic), 7.22 (d, 2H, J = 9 Hz, aromatic), 7.16–7.09 (m, 5H, aromatic), 7.07 (s, 1H, NH), 7.04–7.01 (d, 2H, J = 9 Hz, aromatic), 5.36 (bs, 2H, NH2), 4.18 (q, 2H, J = 9 Hz, CH2), 2.35 (s, 3H, CH3), 1.47 (t, 3H, J = 6 Hz, CH3). 13C NMR (76 MHz, CDCl3) δppm 160.5, 158.7, 155.1, 147.6, 146.0, 144.3, 138.6, 136.2, 134.5, 129.9, 128.8, 128.5, 127.4, 121.7, 120.7, 117.6, 115.0, 111.7, 110.7, 87.9, 65.0, 21.6, 14.9. TOF-HRMS (EI): m/z (M)+ + 1 calcd for C27H24N4O4S 501.1518; found 501.1386.
N-(4-(6-Amino-4-(4-chlorophenyl)-5-cyanopyridin-2-yl)phenyl)-4-methylbenzenesulfonamide (1j). MP = 182–184 °C, 1H NMR (301 MHz, DMSO) δppm 7.93 (d, 2H, J = 3 Hz, aromatic), 7.68–7.59 (m, 6H, aromatic), 7.30 (d, 2H, J = 6 Hz, aromatic), 7.21 (t, 1H, J = 6 Hz, aromatic), 7.14–7.09 (m, 3H, aromatic and NH), 6.94 (bs, 2H, NH2), 2.31 (s, 3H, CH3). 13C NMR (76 MHz, DMSO) δppm 161.3, 159.0, 153.8, 143.0, 136.5, 135.0, 130.8, 130.2, 130.0, 129.6, 129.3, 128.7, 127.2, 119.8, 119.0, 117.6, 109.0, 86.1, 21.5.
N-(4-(6-Amino-4-(2-chlorophenyl)-5-cyanopyridin-2-yl)phenyl)-4-methylbenzenesulfonamide (1k). MP = 141–143 °C, FT-IR (KBr, ν, cm−1): 3441, 3342, 3248, 2928, 2216, 1639, 1556, 1334, 1161, 759. 1H NMR (301 MHz, CDCl3) δppm 8.04 (d, 2H, J = 9 Hz, aromatic), 7.90 (d, 2H, J = 9 Hz, aromatic), 7.71 (d, 1H, J = 6 Hz, aromatic), 7.59–7.51 (m, 3H, aromatic), 7.44–7.32 (m, 5H, aromatic), 7.23 (s, 1H, NH), 5.56 (bs, 2H, NH2), 2.54 (s, 3H, CH3). 13C NMR (76 MHz, CDCl3) δppm 159.7, 158.7, 153.2, 144.3, 138.8, 136.0, 135.9, 134.2, 132.3, 130.8, 130.4, 130.6, 129.9, 128.6, 127.4, 127.2, 120.6, 116.34, 111.8, 90.0, 21.7. TOF-HRMS (EI): m/z (M)+ + 1 calcd for C25H19ClN4O2S 475.0917; found 475.0797.
N-(4-(6-Amino-5-cyano-4-(thiophen-2-yl)pyridin-2-yl)phenyl)-4-methylbenzenesulfonamide (1l). MP = 191–192 °C, 1H NMR (301 MHz, CDCl3) δppm 8.05–8.01 (m, 3H, aromatic), 7.90 (d, 2H, J = 9 Hz, aromatic), 7.69 (d, 1H, J = 6 Hz, aromatic), 7.42–7.33 (m, 7H, aromatic and NH), 5.55 (bs, 2H, NH2), 2.54 (s, 3H, CH3). 13C NMR (76 MHz, CDCl3) δppm 160.7, 159.0, 146.6, 144.3, 138.7, 138.3, 136.1, 134.3, 129.9, 128.9, 128.7, 128.6, 128.5, 128.3, 127.4, 120.6, 117.6, 109.7, 86.0, 21.6.
N,N′-((1,4-Phenylenebis(6-amino-5-cyanopyridine-4,2-diyl))bis(4,1-phenylene))bis(4-methyl benzenesulfonamide) (1m). MP = 205–208 °C, FT-IR (KBr, ν, cm−1): 3506–3388, 3272, 3000, 2225, 1630, 1576, 1344, 1162. 1H NMR (301 MHz, CDCl3) δppm 10.63 (bs, 2H, NH), 8.04 (dd, 2H, J = 8.9, 2.5 Hz, aromatic), 7.83–7.70 (m, 9H, aromatic), 7.59–7.56 (m, 3H, aromatic), 7.41–7.38 (m, 4H, aromatic), 7.23 (dd, 4H, J = 8.4, 1.8 Hz, aromatic), 7.01 (s, 2H, NH2), 6.84–6.71 (m, 4H, aromatic and NH2), 2.36 (s, 3H, CH3), 2.35 (s, 3H, CH3). TOF-HRMS (EI): m/z (M)+ + 1 calcd for C44H34N8O4S2 803.2144; found 803.5102.
Conflicts of interest
There are no conflicts to declare.
Acknowledgements
We thank the Bu-Ali Sina University and Iran National Science Foundation [(INSF), grant number: 98001912)] for financial support to our research group.
References
- S. Khazalpour, M. Yarie, E. Kianpour, A. Amani, S. Asadabadi, J. Yousefi Seyf, M. Rezaeivala, S. Azizian and M. A. Zolfigol, J. Iran. Chem. Soc., 2020, 17, 1775 CrossRef CAS.
- R. Hayes, G. G. Warr and R. Atkin, Chem. Rev., 2015, 115, 6357 CrossRef CAS.
- G. Chatel and D. R. Macfarlane, Chem. Soc. Rev., 2014, 43, 8132 RSC.
- K. Dong, X. Liu, H. Dong, X. Zhang and S. Zhang, Chem. Rev., 2017, 117, 6636 CrossRef CAS.
-
(a) T. Welton, Chem. Rev., 1999, 99, 2071 CrossRef CAS;
(b) L. B. Abdulrauf, A. Lawal, A. Y. Sirhan and G. H. Tan, Chromatographia, 2020, 83, 11 CrossRef CAS;
(c) S. K. Singh and A. W. Savoy, J. Mol. Liq., 2020, 297, 112038 CrossRef CAS;
(d) M. Bielejewski, M. Ghorbani, M. A. Zolfigol, J. Tritt-Goc, S. Noura, M. Narimani and M. Oftadeh, RSC Adv., 2016, 6, 108896 RSC.
- F. R. Moghadam, S. Azizian, M. Bayat, M. Yarie, E. Kianpour and M. A. Zolfigol, Fuel, 2017, 208, 214 CrossRef CAS.
- M. Kharazi, J. Saien, M. Yarie and M. A. Zolfigol, J. Pet. Sci. Eng., 2020, 195, 107543 CrossRef CAS.
-
(a) J. R. Naira, F. Colò, A. Kazzazib, M. Moreno, D. Bresser, R. Line, F. Bella, G. Meligrana, S. Fantini, E. Simonetti, G. B. Appetecchi, S. Passerini and C. Gerbaldi, J. Power Sources, 2019, 412, 398 CrossRef;
(b) A. Dokouzis, F. Bella, K. Theodosiou, C. Gerbaldi and G. Leftheriotis, Mater. Today Energy, 2020, 15, 100365 CrossRef.
- A. Scalia, F. Bella, A. Lamberti, C. Gerbaldi and E. Tresso, Enery, 2019, 166, 789 CrossRef CAS.
- B. A. Voss, R. D. Noble and D. L. Gin, Chem. Mater., 2012, 24, 1174 CrossRef CAS.
- M. E. Mahmoud, M. M. Osman, A. A. Yakout and A. M. Abdelfattah, J. Mol. Liq., 2018, 266, 834 CrossRef CAS.
- Z. He and P. Alexandridis, Adv. Colloid Interface Sci., 2017, 244, 54 CrossRef CAS.
- W. Qian, J. Texter and F. Yan, Chem. Soc. Rev., 2017, 46, 1124 RSC.
- B. Wang, L. Qin, T. Mu, Z. Xue and G. Gao, Chem. Rev., 2017, 117, 7113 CrossRef CAS.
-
(a) S. Zhang, J. Zhang, Y. Zhang and Y. Deng, Chem. Rev., 2017, 117, 6755 CrossRef CAS;
(b) J. Nowicki, M. Muszynski and J.-P. Mikkola, RSC Adv., 2016, 6, 9194 RSC.
-
(a) S. Yotphan, L. Sumunnee, D. Beukeaw, C. Buathongjan and V. Reutrakul, Org. Biomol. Chem., 2016, 14, 590 RSC;
(b) Z. Liu, A. Ebadi, M. Toughani, N. Mert and E. Vessally, RSC Adv., 2020, 10, 37299 RSC.
- R. A. Azzam, R. E. Elsayed and G. H. Elgemeie, ACS Omega, 2020, 5, 10401 CrossRef CAS.
- H. S. Ibrahim, W. M. Eldehna, H. A. Abdel-Aziz, M. M. Elaasser and M. M. Abdel-Azizm, Eur. J. Med. Chem., 2014, 85, 480 CrossRef CAS.
- M. Krasavin, A. Shetnev, T. Sharonova, S. Baykov, T. Tuccinardi, S. Kalinin and C. T. Supuran, Bioorg. Chem., 2018, 76, 88 CrossRef CAS.
- Y. Dong, J. Chen and H. Xu, Chem. Commun., 2019, 55, 2027 RSC.
- S. Bano, K. Javed, S. Ahmad, I. G. Rathish, S. Singh and M. S. Alam, Eur. J. Med. Chem., 2011, 46, 5763 CrossRef CAS.
- A. K. Ganguly, S. S. Alluri, D. Caroccia, D. Biswas, C. H. Wang, E. Kang and V. Munshi, J. Med. Chem., 2011, 54, 7176 CrossRef CAS.
- N. Siddiqui, S. N. Pandeya, S. A. Khan, J. Stables, A. Rana, M. Alam and M. A. Bhat, Bioorg. Med. Chem. Lett., 2007, 17, 255 CrossRef CAS.
- C. T. Supuran, A. Casini and A. Scozzafava, Med. Res. Rev., 2003, 23, 535 CrossRef CAS.
- M. Banerjee, A. Poddar, G. Mitra, A. Surolia and T. B. Owa, J. Med. Chem., 2005, 48, 547 CrossRef CAS.
- C. Allais, J. M. Grassot, J. Rodriguez and T. Constantieux, Chem. Rev., 2014, 114, 10829 CrossRef CAS.
- M. D. Hill, Chem.–Eur. J., 2010, 16, 12052 CrossRef CAS.
- M. Baumann and I. R. Baxendale, Beilstein J. Org. Chem., 2013, 9, 2265 CrossRef.
- J. A. Bull, J. J. Mousseau, G. Pelletier and A. B. Charette, Chem. Rev., 2012, 112, 2642 CrossRef CAS.
- N. De Rycke, F. Couty and O. R. David, Chem.–Eur. J., 2011, 17, 12852 CrossRef CAS.
- G. C. Fu, Acc. Chem. Res., 2004, 37, 542 CrossRef CAS.
- N. G. Kang, M. Changez and J. S. Lee, Macromolecules, 2007, 40, 8553 CrossRef CAS.
- R. Makiura, S. Motoyama, Y. Umemura, H. Yamanaka, O. Sakata and H. Kitagawa, Nat. Mater., 2010, 9, 565 CrossRef CAS.
- W. Zhang, Y. Chen, W. Chen, Z. Liu and Z. Li, J. Agric. Food Chem., 2010, 58, 6296 CrossRef CAS.
- J. C. Xiang, M. Wang, Y. Cheng and A. X. Wu, Org. Lett., 2016, 18, 24 CrossRef CAS.
- M. A. Gouda, M. A. Berghot, G. E. Abd El Ghani and A. E. G. M. Khalil, Synth. Commun., 2014, 44, 297 CrossRef CAS.
- A. Maleki, H. Movahed and P. Ravaghi, Carbohydr. Polym., 2017, 156, 259 CrossRef CAS.
- J. F. Zhou, Y. Z. Song, J. S. Lv, G. X. Gong and S. Tu, Synth. Commun., 2009, 39, 1443 CrossRef CAS.
- M. A. Radwan, F. M. Alminderej and H. M. Awad, Molecules, 2020, 25, 255 CrossRef CAS.
- M. Muthu, R. V. Priya, A. I. Almansour, R. S. Kumar and R. R. Kumar, Beilstein J. Org. Chem., 2018, 14, 2907 CrossRef CAS.
- T. A. Abdallah, J. Heterocycl. Chem., 2007, 44, 961 CrossRef CAS.
- Y. Hao, X. P. Xu, T. Chen, L. L. Zhao and S. J. Ji, Org. Biomol. Chem., 2012, 10, 724 RSC.
- J. F. Zhou, Y. Z. Song, J. S. Lv, G. X. Gong and S. Tu, Synth. Commun., 2009, 39, 1443 CrossRef CAS.
- M. Ghashang, K. Aswin and S. S. Mansoor, Res. Chem. Intermed., 2014, 40, 1135 CrossRef CAS.
- A. Maleki, H. Movahed and P. Ravaghi, Carbohydr. Polym., 2017, 156, 259 CrossRef CAS.
- S. K. Krishnammagari, S. G. Balwe, J. S. Kim, K. T. Lim and Y. T. Jeong, Monatsh. Chem., 2019, 150, 691 CrossRef CAS.
- N. A. Abdel-Latif, Sci. Pharm., 2005, 73, 193 CrossRef.
- D. Khalili, Tetrahedron Lett., 2016, 57, 1721 CrossRef CAS.
- F. Tamaddon, S. Ghazi and M. R. Noorbala, J. Mol. Catal. B: Enzym., 2016, 127, 89 CrossRef CAS.
- A. Yahyazadeh, E. Abbaspour-Gilandeh and M. Aghaei-Hashjin, Catal. Lett., 2018, 148, 1254 CrossRef CAS.
- M. M. Heravi, S. Y. S. Beheshtiha, M. Dehghani and N. Hosseintash, J. Iran. Chem. Soc., 2015, 12, 2075 CrossRef CAS.
- J. F. Allochio Filho, B. C. Lemos, A. S. de Souza, S. Pinheiro and S. J. Greco, Tetrahedron, 2017, 73, 6977 CrossRef CAS.
- S. Zhi, X. Ma and W. Zhang, Org. Biomol. Chem., 2019, 17, 7632 RSC.
- B. H. Rotstein, S. Zaretsky, V. Rai and A. K. Yudin, Chem. Rev., 2014, 114, 8323 CrossRef CAS.
- L. Levi and T. J. J. Müller, Chem. Soc. Rev., 2016, 45, 2825 RSC.
- T. Zarganes-Tzitzikas, A. L. Chandgude and A. Dömling, Chem. Rec., 2015, 15, 981 CrossRef CAS.
- J. T. Edward, Chem. Ind., 1955, 3, 1102 Search PubMed.
- J. M. Erhardt and J. D. Wuest, J. Am. Chem. Soc., 1980, 102, 6363 CrossRef CAS.
- E. Juaristi and G. Cuevas, Tetrahedron, 1992, 48, 5019 CrossRef CAS.
- S. A. Glover, Tetrahedron, 1998, 54, 7229 CrossRef CAS.
- S. A. Glover, A. A. Rosser, A. A. Taherpour and B. W. Greatrex, Aust. J. Chem., 2014, 67, 507 CrossRef CAS.
- S. A. Glover and A. A. Rosser, J. Phys. Org. Chem., 2015, 28, 215 CrossRef CAS.
- R. A. Jones, A. R. Katritzky and M. Snarey, J. Turk. Chem. Soc., Sect. B, 1970, 135 RSC.
-
(a) I. V. Alabugin, Stereoelectronic Effects. A Bridge Between Structure and Reactivity. 2016 CrossRef;
(b) I. V. Alabugin, G. , P. Gomes and M. Abdo, Comput. Mol. Sci., 2018, 9, e1389 Search PubMed;
(c) I. V. Alabugin, K. M. Gilmore and P. W. Peterson, Comput. Mol. Sci., 2011, 1, 109 CrossRef CAS;
(d) S. Z. Vatsadze, Y. D. Loginova, G. dos Passos Gomes and I. V. Alabugin, Chem.–Eur. J., 2017, 23, 3225 CrossRef CAS.
-
(a) A. R. Katritzky, P. J. Steel and S. N. Denisenko, Tetrahedron, 2001, 57, 3309 CrossRef CAS;
(b) A. Nowacki and B. Liberek, Carbohydr. Res., 2018, 462, 13 CrossRef CAS;
(c) D. P. Curran and Y. G. Suh, Carbohydr. Res., 1987, 171, 161 CrossRef CAS;
(d) M. D. Drew, M. C. Wall and J. T. Kim, Tetrahedron Lett., 2012, 53, 2833 CrossRef CAS;
(e) S. E. Denmark, M. S. Dappen, N. L. Sear and R. T. Jacobs, J. Am. Chem. Soc., 1990, 112, 3466 CrossRef CAS;
(f) A. Nowacki, D. Walczak and B. Liberek, Carbohydr. Res., 2012, 352, 177 CrossRef CAS;
(g) C. Jäkel and K. H. Dötz, J. Organomet. Chem., 2001, 624, 172 CrossRef;
(h) M. Asgari and D. Nori-Shargh, Struct. Chem., 2017, 28, 1803 CrossRef CAS;
(i) A. Nowacki and B. Liberek, Carbohydr. Res., 2013, 371, 1 CrossRef CAS.
- D. P. Curran and Y. G. Suh, J. Am. Chem. Soc., 1984, 106, 5002 CrossRef CAS.
- F. Karimi, M. A. Zolfigol and M. Yarie, Mol. Catal., 2019, 463, 20 CrossRef CAS.
- P. Ghasemi, M. Yarie, M. A. Zolfigol, A. A. Taherpour and M. Torabi, ACS Omega, 2020, 5, 3207 CrossRef CAS.
- F. Jalili, M. Zarei, M. A. Zolfigol, S. Rostamnia and A. R. Moosavi-Zare, Microporous Mesoporous Mater., 2020, 294, 109865 CrossRef CAS.
-
(a) F. Karimi, M. A. Zolfigol and M. Yarie, Mol. Catal., 2020, 497, 111201 CrossRef CAS;
(b) S. Noura, M. Ghorbani, M. A. Zolfigol, M. Narimani, M. Yarie and M. Oftadeh, J. Mol. Liq., 2018, 271, 778 CrossRef CAS.
- F. Karimi, M. Yarie and M. A. Zolfigol, RSC Adv., 2020, 10, 25828 RSC.
- M. Yarie, Iran. J. Catal., 2017, 7, 85 CAS.
- M. Yarie, Iran. J. Catal., 2020, 10, 79 CAS.
- G. Tabacchi, M. A. Vanoni, A. Gamba and E. Fois, ChemPhysChem, 2007, 8, 1283 CrossRef CAS.
- G. Hamasaka, H. Tsuji and Y. Uozumi, Synlett, 2015, 26, 2037 CrossRef CAS.
- T. He, R. Shi, Y. Gong, G. Jiang, M. Liu, S. Qian and Z. Wang, Synlett, 2016, 27, 1864 CrossRef CAS.
- C. B. Bai, N. X. Wang, Y. Xing and X. W. Lan, Synlett, 2017, 28, 402 CAS.
- X. Zhao, J. Xiaoa and W. Tang, Synthesis, 2017, 49, 3157 CrossRef CAS.
- A. El-Faham, K. A. Dahlous, Z. A. AL Othman, H. A. Al-Lohedan and G. A. El-Mahdy, Molecules, 2016, 21, 436 CrossRef.
- E. J. Kim, H. W. Ryu, M. J. Curtis-Long, J. Han, J. Y. Kim, J. K. Cho, D. Kang and K. H. Park, Bioorg. Med. Chem. Lett., 2010, 20, 4237 CrossRef CAS.
Footnote |
† Electronic supplementary information (ESI) available. See DOI: 10.1039/d0ra09400e |
|
This journal is © The Royal Society of Chemistry 2021 |
Click here to see how this site uses Cookies. View our privacy policy here.