DOI:
10.1039/D0RA09185E
(Paper)
RSC Adv., 2021,
11, 2397-2404
Ratio fluorescence detection of tetracycline by a Eu3+/NH2-MIL-53(Al) composite†
Received
28th October 2020
, Accepted 27th December 2020
First published on 12th January 2021
Abstract
Tetracycline detection has been a great concern because of its overuse and difficulty in degrading. Here, a detection method with ratio fluorescence was developed by synthesizing Eu3+ doped nanocomposites with NH2-MIL-53(Al) nanosheets. After adding tetracycline, the fluorescence intensity at 616 nm characteristic emission peak of Eu3+ was sensitized by the antenna effect generated from coordinating Eu3+ with tetracycline, but the fluorescence of NH2-MIL-53(Al) at 433 nm was quenched by the fluorescence resonance energy transfer between the Eu3+-tetracycline composition and NH2-MIL-53(Al). Therefore, the efficient detection of tetracycline was achieved based on this change of ratio fluorescence signal. The experimental results show that Eu3+/NH2-MIL-53(Al) has excellent selectivity, a wider linear range and a lower detection limit for detecting tetracycline. This method can afford favorable ideas for developing advanced chemical and biological sensors.
1. Introduction
Tetracycline (TC) has been used to treat bacterial infections due to its excellent antibacterial properties and good therapeutic effects.1–3 It is difficult to be degraded. Therefore, excessive use may cause it to accumulate in foods such as meat, eggs and milk, in water and soil, which seriously threatens human health.4–6 It is necessary to seek a highly sensitive and selective strategy to realize the detection of TC. At present, relatively mature detection methods include immunoassay, liquid chromatography-mass spectrometry, chemiluminescence, high performance liquid chromatography and so on.7–10 However, their applications are restricted owing to the methods are often time-consuming, requiring expensive equipment and complex sample preparation.11
Recently, novel fluorescence detection methods based on the design of highly effective fluorescence probes have caught widespread attention because of their obvious advantages, such as simple, sensitive, fast and easy operation with a lower cost. A variety of nanomaterial-based fluorescent probes have been reported for detecting TC, including water-soluble quantum dots (QD),12 metal nanoclusters (M NCs),13 and Ag nanoparticles (Ag NPs).14 These sensors usually detect the target object through the change of a single fluorescent signal. The accuracy of the detection results from these methods can be affected by some human and environmental factors.15 In order to effectively overcome these problems and achieve sensitive and accurate detection to a target, some ratiometric fluorescent probes have been proposed. For example, Li et al.16 designed a SiNPs-Eu sensor based on fluorescence resonance energy transfer for a ratio-type detection of TC. Recently, a new ratiometric fluorescence probe based on carbon dots was further developed for detecting TC.12,17
Liking metal–organic framework materials (MOFs), two-dimensional metal–organic framework nanosheets are coordinately assembled by metals and organic ligands. Compared with MOFs, MOFs nanosheets have many advantages, such as rich active sites, large specific surface area, high aspect ratio, adjustable porous structure and chemical composition, as well as ultra-thin thickness, which are widely used in the fields of catalysis, gas separation and chemical sensing.18,19 However, most MOFs are currently synthesized in organic solvent systems to ensure their topological structure, which leads to poor water stability and limits the application of water-soluble substances in aqueous systems.20 The introduction of NH2-MIL-53(Al) in water solvent through one-pot hydrothermal synthesis of –NH2 has the advantages of avoiding the complicated steps involved in post-modification and good water solubility. On the other hand, for the FL detection system, the introduction of –NH2 can improve the selectivity and sensitivity, thus significantly expanding the potential application of MOFs in FL analysis.21–24 The introduced –NH2 can react with TCs through hydrogen bond interaction, effectively speeding up the quenching of the fluorescence of NH2-MIL-53(Al). In addition, the MIL-type MOF composed of Al3+ and dicarboxylate ligand is stable to water and high temperature.20,25–27 Therefore, efficient sensors can be developed by using the advantages of the composite materials based on MOFs nanosheets.
In this paper, a novel ratio-type fluorescence sensor based on the composite material of MOFs nanosheets (Eu3+/NH2-MIL-53(Al)) was prepared and used for detecting tetracycline. First, the NH2-MIL-53(Al) nanosheets were prepared by hydrothermal method. The Eu3+/NH2-MIL-53(Al) nanocomposites were then obtained by doping Eu3+ ions into NH2-MIL-53(Al) nanosheets. Once tetracycline was added, Eu3+ acts as a response unit to coordinate with tetracycline to form Eu–TC complex, which sensitizes the characteristic emission peak of Eu3+ at 616 nm through the antenna effect. With the successive concentration increase of tetracycline, the characteristic the emission peak of Eu3+ at 616 nm increase continuously. Due to the overlap between the ultraviolet-visible absorption spectrum of Eu–TC complex and the fluorescence emission spectrum of NH2-MIL-53(Al), there might be a fluorescence resonance energy transfer effect between them, making the fluorescence intensity of NH2-MIL-53(Al) at 433 nm quenched, but the fluorescence intensity of Eu–TC complexes increases successively. Therefore, the highly sensitive detection of tetracycline can be achieved by using these ratio fluorescence signal change. The experimental results show that Eu3+/NH2-MIL-53(Al) has a relatively high selectivity, a wide linear range and a low detection limit for detecting tetracycline. The mechanism diagram of the ratio-type detection of tetracycline by Eu3+/NH2-MIL-53(Al) nanocomposite is shown in Scheme 1.
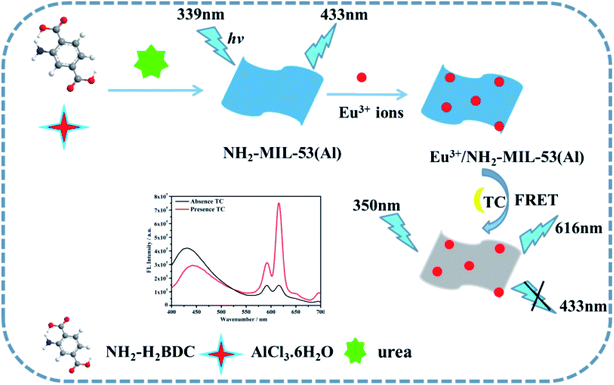 |
| Scheme 1 The synthesis of Eu3+/NH2-MIL-53(Al) composites and the mechanism for detecting TC. | |
2. Experimental section
2.1 Chemicals and instruments
Europium oxide, tetracycline and hydrochloric acid are purchased from Aladdin. Tris(hydroxymethyl)aminomethane was obtained from Shanghai Zhongqin Chemical Reagent. Absolute ethanol was from Tianjin Anlong. All reagents are analytical pure grade. Europium chloride was prepared from europium oxide. The water used in the experiment was ultrapure water (18.2 MΩ cm). Fluorescence spectrum were recorded by a FluoroMax-4 fluorescence spectrophotometer (American FEI Corporation). Other instruments are the PHS-3B pH acidity meter (Shanghai Precision Instrument Science Co., Ltd.), the Nicolet Impact-400 Fourier infrared spectrometer (Shimadzu, Nicolet Corporation), the XRD-6000 X-powder diffractometer (Shanghai Rente Testing Instrument Co., Ltd.), the UV-1102 UV spectrophotometer (Shanghai Tianmei Scientific Instrument Company), the DZF-6020 vacuum drying oven (Shanghai Yiheng Technology Co. Ltd), the BSA224S electronic balance (Beijing Sedorius Scientific Instruments) and the PCD-2000 thermostatic blast dryer (Shanghai Langgan Shiyan Shebei Co. LTD.).
2.2 Preparation of NH2-MIL-53(Al) nanosheets
NH2-MIL-53(Al) nanosheets were prepared as follows.28 3 mmol AlCl3·6H2O was dissolved into 15 mL ultrapure water. Then, 3 mmol NH2-H2BDC was added into the above solution under magnetic stirring. After stirring for 30 minutes, dropping 15 mL deionized water solution with 6 mmol urea into the above mixture, and stirring for 30 minutes. Furthermore, the prepared mixture was placed in a 70 mL autoclave and reacted in an oven at 150 °C for 5 hours. After cooling to the room temperature, the mixture was centrifuged at 8000 rpm and washed several times with ultrapure water. Then, the product was dispersed in 20 mL of DMF and 20 mL of methanol, and respectively stirred at room temperature for 1 day. Finally, the solvent was removed by centrifugation, and the desired product was obtained by drying.
2.3 Preparation of Eu3+/NH2-MIL-53(Al)
By referring the reported method and making slight modification, Eu3+/NH2-MIL-53(Al) composite material was prepared by chemical doping method.29 After immersing 50 mg NH2-MIL-53(Al) nanosheets into an ethanol solution containing EuCl3·6H2O (5 mL, 1 mmol) for 2 days, the undoped europium ions was removed by centrifuging and washing with ethanol solution. Then, the Eu3+/NH2-MIL-53(Al) nanocomposite was obtained by drying above remained solution at 60 °C.
2.4 Detection TC by NH2-MIL-53(Al) nanosheet
At room temperature, the specific process the fluorescence detection of tetracycline (pH = 5, 0.1 M) in Tris–HCl buffer solution was as follows. 2 mL Tris–HCl buffer solution (0.1 M, pH = 5) was added into 100 μL (0.005 mg mL−1) NH2-MIL-53(Al) aqueous solution. Then, the TC solutions with different concentrations were added into the above solution. Finally, the fluorescence intensity of NH2-MIL-53(Al) nanosheets was measured and observed at an excitation wavelength of 339 nm.
2.5 Eu3+/NH2-MIL-53(Al) nanocomposite testing TC
The specific process of fluorescence detection of tetracycline (pH = 9, 0.1 M) in Tris–HCl buffer solution at room temperature was as follows. 2 mL Tris–HCl buffer solution (0.1 M, pH = 9) was added into 100 μL (0.005 mg mL−1) Eu3+/NH2-MIL-53(Al) aqueous solution. Then, the TC solutions with different concentrations were added into the above solution, and reacting for 1 minute at room temperature. Finally, the change of the fluorescence signal of Eu3+/NH2-MIL-53(Al) nanocomposite was measured at the excitation wavelength of 339 nm.
3. Results and discussion
3.1 TEM image of NH2-MIL-53(Al)
The NH2-MIL-53(Al) nanosheets were characterized by TEM, PXRD, FT-IR and fluorescence spectroscopy. As shown in Fig. 1A and B, NH2-MIL-53(Al) is successfully prepared, and we can see the morphology of 2D nanosheets.
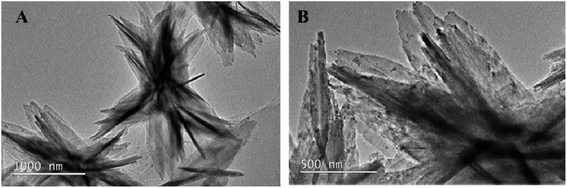 |
| Fig. 1 TEM image of NH2-MIL-53(Al). | |
3.2 Powder X-ray diffraction (PXRD) and Fourier infrared spectroscopy (FT-IR) characteristics of Eu3+/NH2-MIL-53(Al)
The X-ray diffraction (PXRD) Characteristics of NH2-MIL-53(Al)28 (black line) and Eu3+/NH2-MIL-53(Al) (red line) nanocomposites are shown in Fig. 2A. It is obvious that the crystal structure of NH2-MIL-53(Al) nanosheets is not changed after doping with Eu3+. In the Fourier infrared spectrum (FT-IR) of the nanocomposites (Fig. 2B), NH2-MIL-53(Al) nanosheets (blue line) is successfully prepared by comparing with the organic ligand NH2-H2BDC (red line). The slight shift of the peak in the Eu3+/NH2-MIL-53(Al) nanocomposite (green line) indicates that Eu3+ is doped into the NH2-MIL-53(Al) nanosheet.29
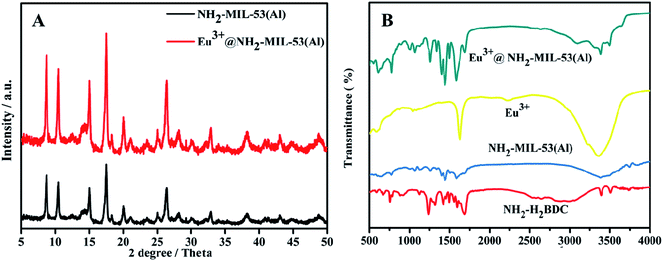 |
| Fig. 2 (A) PXRD diagram of NH2-MIL-53(Al) (black line) and Eu3+/NH2-MIL-53(Al) (red line). (B) FT-IR image of NH2–H2BDC (red line), NH2-MIL-53(Al) (blue line), Eu3+ (yellow line) and Eu3+/NH2-MIL-53(Al) (green line). | |
3.3 BET characterization of NH2-MIL-53(Al) and Eu3+/NH2-MIL-53(Al)
In order to further characterize the composite material, we use BET characterization, and the result is shown in Fig. 3.
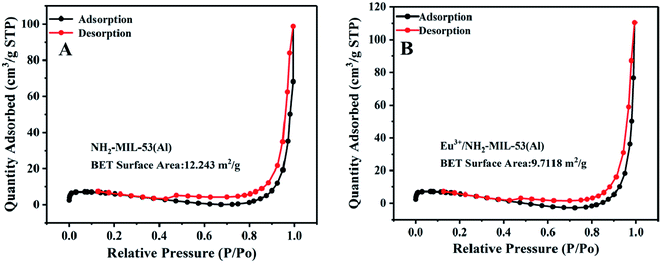 |
| Fig. 3 BET characterization of NH2-MIL-53(Al) (A)and Eu3+/NH2-MIL-53(Al) (B). | |
3.4 Characterization of the ultraviolet and fluorescence spectra of the material Eu3+/NH2-MIL-53(Al)
In the ultraviolet-visible absorption spectrum and fluorescence (FL) emission spectrum of Eu3+/NH2-MIL-53(Al) composites (Fig. 4), the Eu3+/NH2-MIL-53(Al) composite material has an absorption peak at 330 nm, and has the characteristic fluorescence emission peaks of NH2-MIL-53(Al) nanosheets at 433 nm and Eu3+ at 616 nm, indicating the Eu3+/NH2-MIL-53(Al) composites are prepared.
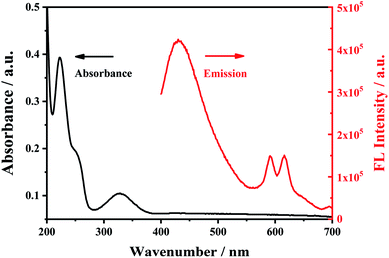 |
| Fig. 4 The UV-vis absorption spectra (black line) and fluorescence emission spectra of Eu3+/NH2-MIL-53(Al). | |
3.5 Condition optimization of the TC detection by NH2-MIL-53(Al) nanosheets
Before using composite materials to detect TC, a single material NH2-MIL-53(Al) nanosheets was used to perform the fluorescent detection on TC. In order to improve the accuracy of the detection results, the pH and response time during the experiment were optimized. The fluorescence intensity changed with the change of the pH value within the range of 4–9, and had the most response when pH = 5 (Fig. 5A). So, pH = 5 was selected as the optimal pH value. During the experiment, 20 μL TC (10−3 M) was added dropwise (Fig. 5B). Once TC was added, the fluorescence intensity of the nanosheets decreased rapidly, and did not change apparently with the increase of time. It is obvious that the fluorescence intensity of NH2-MIL-53(Al) can be barely impacted by the response time.
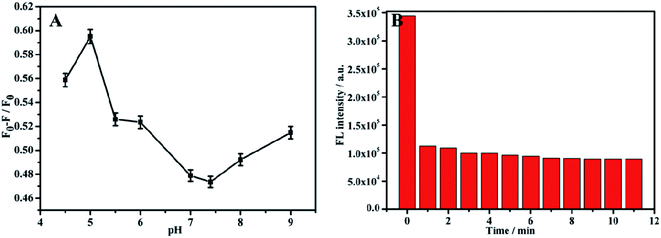 |
| Fig. 5 (A) Effect of different pH on TC detection of NH2-MIL-53(Al), (B) response time of NH2-MIL-53(Al) to TC. | |
3.6 Fluorescence detection of TC by NH2-MIL-53(Al)
TC was detected with the above-mentioned optimal conditions. With the TC concentration increase from 0–120 μM, the fluorescence intensity at 433 nm gradually decreases (Fig. 6A). Within the range of 1.5–70 μM TC concentration, the change in fluorescence intensity ((F0 − F)/F0) shows a good linear relationship with the TC concentration (Fig. 6B). The linear regression equation is (F0 − F)/F0 = 0.04409 [TC] + 0.01748, and the calculated minimum detection limit (LOD) is 0.92 μM.
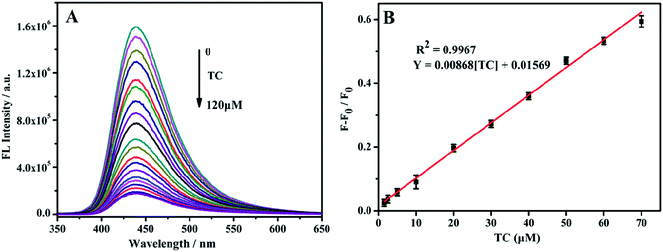 |
| Fig. 6 (A) The fluorescence emission spectra of NH2-MIL-53(Al) with the increase of TC concentration; (B) NH2-MIL-53(Al) nanosheets linear relationship between changes in fluorescence intensity and TC concentrations. | |
3.7 Fluorescence detection of TC by Eu3+/NH2-MIL-53(Al)
The response time of the fluorescent probe Eu3+/NH2-MIL-53(Al) for detecting tetracycline was studied (Fig. 7). The response time between the fluorescent signal of Eu3+/NH2-MIL-53(Al) composite and TC is very fast, which can be completed within 1 min. The fluorescence intensity ratio (I616/I433) basically unchanges with the further increase of the response time, indicating that the probe has a better response and stability for detecting TC.
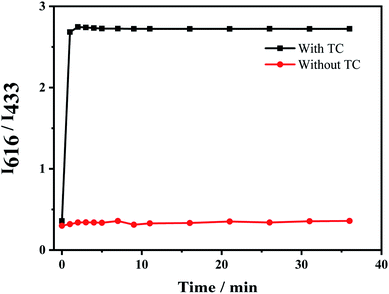 |
| Fig. 7 Response time of Eu3+/NH2-MIL-53(Al) to TC fluorescence detection. | |
Fig. 8A shows the response of TC solutions with different concentrations to the fluorescence intensity ratio (I616/I433) of Eu3+/NH2-MIL-53(Al) composite material. With the increase of the TC concentration, the fluorescence intensity of NH2-MIL-53(Al) at 433 nm is getting smaller and smaller, but the fluorescence intensity of Eu3+ is increasing at 616 nm. Therefore, the Eu3+/NH2-MIL-53(Al) composite material can be used as a ratiometric fluorescent probe for detecting TC. Fig. 7B is the relationship between different TC concentrations and the fluorescence intensity ratio (I616/I433). When the TC concentration changes from 0.5 to 60 μM, the concentration of TC and I616/I433 shows a good linear relationship. The linear equation is I616/I433 = 0.02919 [TC] + 0.05961 with a correlation coefficient R2 = 0.9905. The minimum detection limit of the composite material for TC is 0.16 μM, compared to the detection of TC by NH2-MIL-53(Al) nanosheets, the ratiometric fluorescent probes have a distinct lower detection limit.
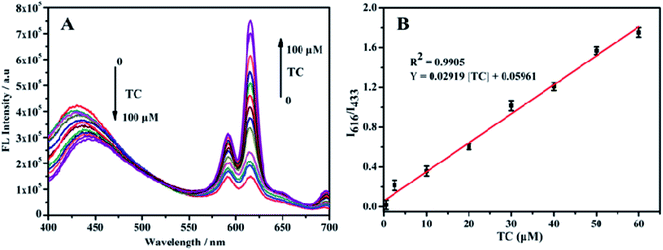 |
| Fig. 8 (A) The change in the ratio fluorescence (I616/I433) intensity of Eu3+/NH2-MIL-53(Al) after adding different concentrations of TC; (B) the linear relationship between TC concentrations and fluorescence intensity ratio (I616/I433). | |
3.8 The selectivity of the TC detection by Eu3+/NH2-MIL-53(Al)
The effect of some interferences like Co2+, Ca2+, Mg2+, Zn2+, K+, ascorbic acid (AA), cysteine (Lys) and glutathione (GSH) to the fluorescence intensity ratio (I616/I433) of Eu3+/NH2-MIL-53(Al) composite material was studied (Fig. 9). The results show that the fluorescence intensity ratio is obviously changed by adding TC, while other interferences have little effect on the fluorescence intensity ratio, indicating that the nanocomposite can achieve TC detection.
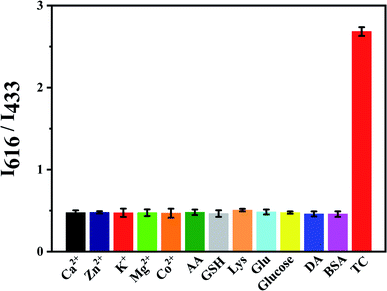 |
| Fig. 9 Selectivity detect of Eu3+/NH2-MIL-53(Al) for TC. | |
3.9 Detection of TC in water samples
In order to further evaluate the anti-interference ability of fluorescent probes and the feasibility of this dual emission ratio fluorescent probe for rapid and ultra-sensitive detection of TC in practical applications, we performed fluorescence spectroscopy on actual water samples (tap water) test. As shown in the ESI Table 2,† the fluorescence intensity ratio of tap water and deionized water solution gradually increases with the increase of TC concentration. In addition, the ratio of the fluorescence intensity of the probes in the two water samples was approximately the same, and no significant difference was observed. The fluorescence intensity ratio has a linear relationship with the TC concentration and a good recovery rate of standard addition is obtained. The results show that the detection system has the same results as the laboratory conditions of deionized water, which can effectively eliminate the interference of coexisting substances, which proves that the probe has excellent selectivity. At the same time, it proved the accuracy and reliability of the probe in measuring TC in environmental samples.
3.10 The mechanism verification of TC detection by Eu3+/NH2-MIL-53(Al)
In order to study the proposed detection mechanism, the characterization of ultraviolet-visible absorption spectrum and fluorescence spectrum was carried out. There is a spectral overlap between the ultraviolet-visible absorption spectrum of the complex TC–Eu3+/NH2-MIL-53(Al) and the fluorescence spectrum of NH2-MIL-53(Al) (Fig. 10), indicating that there may have a fluorescence resonance energy transfer effect between them. As an energy donor, the fluorescence intensity of NH2-MIL-53(Al) is quenched, while the fluorescence intensity of the TC–Eu3+/NH2-MIL-53(Al) complex as an energy acceptor is enhanced. Thereby, detecting tetracycline can be achieved by changing the ratio of fluorescence signals.
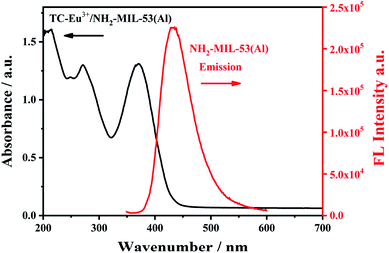 |
| Fig. 10 The UV-vis absorption spectrum (black line) of TC–Eu3+/NH2-MIL-53(Al) and the fluorescence emission spectrum of NH2-MIL-53(Al). | |
4. Conclusion
In summary, a ratio-based fluorescence detection method is proposed for detecting TC by preparing Eu3+/NH2-MIL-53(Al) nanocomposite. When TC is added, Eu3+ acts as a response unit to coordinate with tetracycline to form a complex, thereby sensitizing the characteristic emission peak of Eu3+ at 616 nm through the antenna effect. Due to the spectral overlap between the ultraviolet-visible absorption spectrum of the formed complex and the fluorescence spectrum of NH2-MIL-53(Al), indicating that there may be a fluorescence resonance energy transfer effect between them, so the fluorescence intensity of NH2-MIL-53(Al) at 433 nm is quenched. With the increase of TC concentration, the fluorescence at 433 nm decreases continuously, and the fluorescence at 616 nm increases. So, the purpose of detecting tetracycline can be achieved by changing the ratio of fluorescence signals. The linear range of this ratio fluorescent probe for detecting tetracycline is 0.5–60 μM, the minimum detection limit is 0.16 μM. The method provides a novel idea for detecting tetracycline with excellent selectivity.
Conflicts of interest
There are no conflicts to declare.
Acknowledgements
We are grateful for financial support by National Natural Science Foundation of China (Grant No. 21565022); Gansu Provincial Science and Technology Project (18YF1GA050); Gansu Provincial Computing Centre.
References
- I. Chopra and M. Roberts, Tetracycline antibiotics: mode of action, applications, molecular biology, and epidemiology of bacterial resistance, Microbiol. Mol. Biol. Rev., 2001, 65(2), 232–260 CrossRef CAS.
- T. Gan, Z. X. Shi, J. Y. Sun and Y. M. Liu, Simple and novel electrochemical sensor for the determination of tetracycline based on iron/zinc cations-exchanged montmorillonite catalyst, Talanta, 2014, 121, 187–193 CrossRef CAS.
- G. D. Wright, Solving the antibiotic crisis, ACS Infect. Dis., 2015, 1, 80–84 CrossRef CAS.
- A. S. Schmidt, M. S. Bruun, I. Dalsgaard and J. L. Larsen, Incidence, distribution, and spread of tetracycline resistance determinants and integron-associated antibiotic resistance genes among motile aeromonads from a fish farming environment, Appl. Environ. Microbiol., 2001, 67(12), 5675–5682 CrossRef CAS.
- M. H. Rahman, L. Nonaka, R. Tago and S. Suzuki, Occurrence of two genotypes of tetracycline (TC) resistance gene tet(M) in the TC-resistant bacteria in marine sediments of Japan, Environ. Sci. Technol., 2008, 42(14), 5055–5061 CrossRef CAS.
- M. H. Huang, W. Zhang, Y. Zheng and W. Zhang, Correlation among extracellular polymeric substances, tetracycline resistant bacteria and tetracycline resistance genes under trace tetracycline, Chemosphere, 2014, 117, 658–662 CrossRef CAS.
- D. S. Aga, R. Goldfish and P. Kulshrestha, Application of ELISA in determining the fate of tetracyclines in land-applied livestock wastes, Analyst, 2003, 128(6), 658–662 RSC.
- N. László, K. Lányi and P. Laczay, LC-MS study of the heat degradation of veterinary antibiotics in raw milk after boiling, Food Chem., 2018, 267, 178–186 CrossRef.
- A. Townshend, W. Ruengsitagoon, C. Thongpoon and S. Liawruangrath, Flow injection chemiluminescence determination of tetracycline, Anal. Chim. Acta, 2005, 541(1–2), 103–109 CrossRef.
- H. Xu, H. Y. Mi, M. M. Guan, H. Y. Shan, Q. Fei, Y. F. Huan, Z. Q. Zhang and G. D. Feng, Residue analysis of tetracyclines in milk by HPLC coupled with hollow fiber membranes-based dynamic liquid-liquid micro-extraction, Food Chem., 2017, 232, 198–202 CrossRef CAS.
- K. Granelli, C. Elgerud, A. Lundstrom, A. Ohlsson and P. Sjoberg, Rapid multiresidue analysis of antibiotics in muscle by liquid chromatography-tandem mass spectrometry, Anal. Chim. Acta, 2009, 637(1–2), 87–91 CrossRef CAS.
- W. Li, J. Zhu, G. Xie, Y. Ren and Y. Q. Zheng, Ratiometric system based on graphene quantum dots and Eu3+ for selective detection of tetracyclines, Anal. Chim. Acta, 2018, 1022, 131–137 CrossRef CAS.
- M. Ramezani, N. Mohammad Danesh, P. Lavaee, K. Abnous and S. Mohammad Taghdisi, A novel colorimetric triple-helix molecular switch aptasensor for ultrasensitive detection of tetracycline, Biosens. Bioelectron., 2015, 70, 181–187 CrossRef CAS.
- H. L. Tan and Y. Chen, Silver nanoparticle enhanced fluorescence of europium(III) for detection of tetracycline in milk, Sens. Actuators, B, 2012, 173, 262–267 CrossRef CAS.
- Z. Shojaeifard, B. Hemmateenejad and M. Shamsipur, Efficient on-off ratiometric fluorescence probe for cyanide ion based on perturbation of the interaction between gold nanoclusters and a copper(II)-phthalocyanine complex, ACS Appl. Mater. Interfaces, 2016, 8(24), 15177–15186 CrossRef CAS.
- X. Li, H. Ma, M. Deng, A. Iqbal, X. Liu, B. Li, W. Liu, J. Li and W. Qin, Europium functionalized ratiometric fluorescent transducer silicon nanoparticles based on FRET for highly sensitive detection of tetracycline, J. Mater. Chem. C, 2017, 5(8), 2149–2152 RSC.
- Z. Shen, C. Zhang, X. L. Yu, J. Li, Z. Y. Wang, Z. P. Zhang and B. H. Liu, Microwave-assisted synthesis of cyclen functional carbon dots to construct a ratiometric fluorescent probe for tetracycline detection, J. Mater. Chem. C, 2018, 6(36), 9636–9641 RSC.
- S. Furukawa, J. Reboul, S. Diring, K. Sumida and S. Kitagawa, Structuring of metal-organic frameworks at the mesoscopic/macroscopic scale, Chem. Soc. Rev., 2014, 43(16), 5700–5734 RSC.
- A. Carne, C. Carbonell, I. Imaz and D. Maspoch, Nanoscale metal-organic materials, Chem. Soc. Rev., 2011, 40(1), 291–305 RSC.
- Z. Xiang, C. Fang, S. Leng and D. Cao, An amino group functionalized metal-organic framework as a luminescent probe for highly selective sensing of Fe3+ ions, J. Mater. Chem. A, 2014, 2, 7662–7665 RSC.
- C. H. Fu, H. Q. Zhou, L. F. Tan, Z. B. Huang, Q. Wu, X. L. Ren, J. Ren and X. W. Meng, et al., Microwave-Activated Mn-Doped Zirconium Metal-Organic Framework Nanocubes for Highly Effective Combination of Micro-wave Dynamic and Thermal Therapies Against Cancer, ACS Nano, 2018, 12, 2201–2210 CrossRef CAS.
- B. Wang, X. L. Lv, D. W. Feng, L. H. Xie, J. Zhang, M. Li, Y. B. Xie, J. R. Li and H. C. Zhou, et al., Highly Stable Zr(IV)-Based Metal-Organic Frameworks for the Detection and Removal of Antibiotics and Organic Explosives in Water, J. Am. Chem. Soc., 2016, 138, 6204–6216 CrossRef CAS.
- W. Dong, X. Liu, W. Shi and Y. Huang, Metal-organic framework MIL-53(Fe): facile microwave-assisted synthesis and use as a highly active peroxidase mimetic for glucose biosensing, RSC Adv., 2015, 5, 17451–17457 RSC.
- T. Lu, L. C Zhang, M. X. Sun, D. Y. Deng, Y. Y. Su and Y. Lv, et al., Amino-Functionalized Metal-Organic Frameworks Nanoplates based Energy Transfer Probe for Highly Selective Fluorescence Detection of Free Chlorine, Anal. Chem., 2016, 88, 3413 CrossRef CAS.
- L. Zhang and L. Chen, A New Fluorescence Probe Based on Hybrid Mesoporous Silica/Quantum Dot/Molecularly Imprinted Polymer for Detection of Tetracycline, ACS Appl. Mater. Interfaces, 2016, 8, 16248–16256 CrossRef CAS.
- C. X. Yang, H. B. Ren and X. P. Yan, Fluorescent metal−organic framework MIL-53(Al) for highly selective and sensitive detection of Fe3+ in aqueous solution, Anal. Chem., 2013, 85, 7441–7446 CrossRef CAS.
- Y. R. Du, X. Q. Li, H. J. Zheng, X. J. Lv and Q. Jia, et al., Design of a calix[4]arene-functionalized metal-organic framework probe for highly sensitive and selective monitor of hippuric acid for indexing toluene exposure, Anal. Chim. Acta, 2018, 1001, 134–142 CrossRef CAS.
- T. Lu, L. Zhang, M. Sun, D. Deng, Y. Su and Y. Lv, Amino-functionalized metal-organic frameworks nanoplates-based energy transfer probe for highly selective fluorescence detection of free chlorine, Anal. Chem., 2016, 88(6), 3413–3420 CrossRef CAS.
- X. Y. Xu and B. Yan, Eu(III)-functionalized MIL-124 as fluorescent probe for highly selectively sensing ions and organic small molecules especially for Fe(III) and Fe(II), ACS Appl. Mater. Interfaces, 2015, 7(1), 721–729 CrossRef CAS.
Footnote |
† Electronic supplementary information (ESI) available. See DOI: 10.1039/d0ra09185e |
|
This journal is © The Royal Society of Chemistry 2021 |
Click here to see how this site uses Cookies. View our privacy policy here.