DOI:
10.1039/D0QM00605J
(Research Article)
Mater. Chem. Front., 2021,
5, 438-449
Optical-switchable energy transfer controlled by multiple-responsive turn-on fluorescence via metal–ligand and host–guest interactions in diarylethene-based [2]pseudo-rotaxane polymers†
Received
18th August 2020
, Accepted 14th October 2020
First published on 19th October 2020
Abstract
Multi-responsive and optically-active diarylethene-based [2]pseudo-rotaxane polymers Zn2+-TC2/DS(O & C) were synthesized and prepared via metal–ligand and host–guest interactions, where Zn2+ ion was coordinated with two ligands (TC) containing a macrocyclic dibenzo-24-crown-8 (DB24C8) linked to a terpyridyl terminus to form a symmetrical host (Zn2+-TC2) and diarylethene (DAE) bearing two terminal secondary ammonium salts to form a symmetrical guest (DS). Upon irradiation of UV-light (λ = 355 nm), the prominent photo-induced electron transfer (PET) off process to promote optical-switchable Förster resonance energy transfer (FRET) process from the emissive metal-coordination Zn2+-TC2 host to the fluorescent DS(C) guest (in the closed form of emissive DAE unit) was explored for the multiple-responsive turn-on ratiometric fluorescence in supramolecular polymer Zn2+-TC2/DS(C). Remarkably, endowed with the novel features of reversible chemical/chelation-stimuli responsive dis-assembly/re-assembly of non-covalent interactions in response to pH/chelation, the polymer can be used for the sensing of pH and cyclen. Accordingly, the “molecular wire and stimuli-responsive effects” of diarylethene-based [2]pseudo-rotaxane polymer Zn2+-TC2/DS(C) reveal significant supramolecular interactions to elucidate the turn-on fluorescence via the controllable FRET-ON process from Zn2+-TC2 host donor to PET-OFF DS(C) guest acceptor, which pave a promising route to elaborate optical-switchable supramolecular platforms for the future energy transfer applications.
Introduction
Supramolecular self-assemblies have attracted significant interest in developing innovative concepts for the design multiple-stimuli-responsive smart and adaptive materials, and have also shown great potential in numerous research areas.1–5 The repeating units of supramolecular polymers are associated with reversible or dynamic non-covalent interactions of complementary receptor units,6–8 so the intrinsic dynamic properties of non-covalent interactions are usually responsive to a wide range of external stimuli, such as pH value,9 light,10 stress,11 temperature12 and chemical reaction.13–15 For instance, the host–guest interactions of a dibenzo-24-crown-8 (DB24C8) host and dibenzyl ammonium salt (DBAs) guest via the supportive hydrogen-bonding of (N+–H⋯O) and (C–H⋯O) are highly responsive towards the interactions with metal ions, pH value and temperature.16–18 The unique luminescence features of the metal–ligand-coordinated terpyridyl (tpy)-Zn2+ complex are well-utilized for the construction of photo-luminescent supramolecular coordination compounds and polymers.19–24 Notably, the host–guest and metal–ligand interactions are independent of each other and thus both of them could be facilitated to construct novel stimuli-responsive supramolecular materials.
Recently, photochromic fluorescent diarylethenes (DAEs) have received considerable attention due to their remarkable potential including optoelectronics.25–27 The reversible photo-isomerization of DAE materials can be interconverted between two states with different spectroscopic properties by irradiation with UV and visible light. To achieve a greater fluorescence contrast between the two states of DAE units, various fluorescent fluorophores have been attached to the diarylethene core.28 However, the fluorescence properties of some photochromic DAE derivatives were turned off due to the Förster resonance energy transfer (FRET) from the excited fluorophore to the photo-cyclized DAE core upon the irradiation of UV light.29,30 Despite the development of photochromic fluorescent DAEs, only a few turn-on fluorescent DAEs were reported to show high fluorescence in their photo-cyclized state upon UV irradiation.31–34 Nevertheless, very minor efforts have been explored in the insights of energy/electron modulation events by incorporating the photo-cyclized turn-on fluorescent DAEs with other fluorophores in supramolecular polymers via non-covalent interactions. Moreover, the photo-controllable supramolecular polymers are considered to be better candidates over small molecules for preparing light-responsive self-healing materials, where the assembly and disassembly of non-covalent connections within polymer backbones could be regulated via photo-switching the configuration of the photochromic units.35–39 So far, there have been only a few reports related to the coupled photochromic activities of non-emissive DAE units (in the closed form) with tpy ligands enabled by covalent linkages40–43 rather than host–guest approaches. Recently, a symmetrical non-emissive DAE guest (in the closed form) connected with two AIE-active pillar[5]arene hosts in a photo-controllable pseudo[3]rotaxane system was observed due to the reversible FRET behavior controlled by the photo-isomerization of the DAE unit.44 To the best of our knowledge, there is only one report on coupled photo-switchable FRET activities from an asymmetrical non-emissive DAE guest (in the closed form) complexed with an asymmetrical Eu3+-tpy host in a monomeric pseudo[2]rotaxane system rather than both symmetrical emissive DAE guests (in the closed form) complexed with the symmetrical Zn2+-tpy host in our supramolecular polymer approach.45 Swager et al. also reported that the “molecular wire and stimuli-responsive effects” of supramolecular polymers have contributed significantly to magnifying incredible sensory signals and sensitivities to enhance their interactions towards specific analysts as compared to analogous monomeric systems.46 Therefore, the multiple-responsive supramolecular polymer designs by the integration of the Zn2+-bis-terpyridyl host with the photochromic emissive DAE guest (in the closed form) would be more interesting for exploring their associated energy transfer properties in this study.
Herein, our strategy involves the integration of multiple-responsive host–guest, metal–ligand and photoactive interactions for the construction of the [2]pseudo-rotaxane polymer Zn2+-TC2/DS(O & C) in Chart 1 to investigate novel optical-switchable energy transfer phenomena. This multiple-responsive supramolecular polymer consists of optical-switchable turn-on fluorescence symmetrical diarylethene (DAE)-based guest DS bearing two terminal secondary ammonium salts and a Zn2+-TC2 host, which contains two macrocyclic dibenzo-24-crown-8 (DB24C8) termini linked to terpyridyl (tpy) ligands (TC2) coordinated with the Zn2+ metal ion. Notably, no such efficient control of energy transfer by optical-switchable and pH/chelation stimuli-response in supramolecular polymer has been reported to date, where the FRET process from the fluorescent metal-coordinated Zn2+-TC2 host to the fluorescent DS(C) guest (i.e., the photo-cyclized form of the emissive DAE unit) in a supramolecular polymer has been explored with ratiometric PL emissions. In this study, the first controllable energy transfer via the multiple-responsive capabilities of host–guest, metal–ligand and photoactive interactions in the supramolecular polymer Zn2+-TC2/DS(C) are investigated by means of acid–base and another competitive chelating ligand (i.e., cyclen).
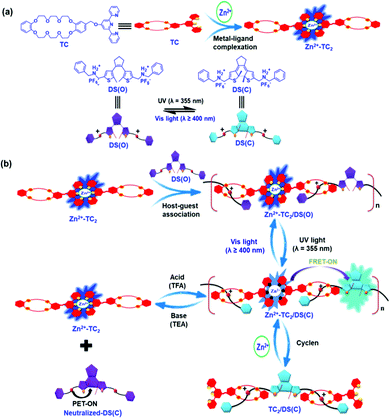 |
| Chart 1 Schematic pictorial representations of (a) chemical structures of the metal-coordinated Zn2+-TC2 host, optically-switchable DS(O & C) guest (i.e., open to closed form) before and after UV-irradiation, respectively. (b) The formation of a [2]pseudo-rotaxane polymer Zn2+-TC2/DS(O & C) and the novel features of the reversible optical/chemical/chelation-stimuli responsive interactions in response to light/pH/chelation. | |
Results and discussion
The formation of the coordination compound (Zn2+-TC2) via metal–ligand interactions
The capability of the terpyridyl (tpy) ligand (TC) coordinated with Zn2+ metal ions, i.e., Zn(OTf)2, to form the coordination compound (Zn2+-TC2) was investigated as shown in Fig. 1. The corresponding UV-Vis and PL spectral changes of ligand TC containing dibenzo-24-crown-8 (DB24C8) linked to the terpyridyl terminus (1 mM in CHCl3/CH3CN (1/1, v/v)) as coordinated with Zn2+ are demonstrated in Fig. 1(b) and (c), respectively. Upon the addition of Zn(OTf)2 (0 to 50 μL or 0.5 equiv.), the absorption bands of ligand TC at 242 and 279 nm were slightly red- and blue-shifted to 247 and 275 nm, respectively, and two new absorption bands with vibronic progressions at 311 and 322 nm were generated with clear isosbestic points at 269 and 301 nm (Fig. 1(b)). The related PL spectral changes of ligand TC upon the addition of Zn(OTf)2 are illustrated in Fig. 1(c). Upon the addition of Zn(OTf)2, the emission band (at 403 nm) of ligand TC was red-shifted to a more prominent band at 417 nm, along with a less pronounced new emission band at 338 nm (with a quantum yield of 18.40% for coordination compound Zn2+-TC2 in CHCl3/CH3CN (1/1, v/v) medium, using quinine hemisulfate standard). According to Fig. S1 in the ESI,† the metal–ligand complex formation between Zn2+ and ligand TC was verified by Job's plot to be a molar ratio of 1
:
2 from the free ligand form to the coordination compound Zn2+-TC2.
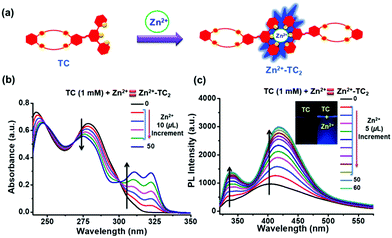 |
| Fig. 1 (a) Pictorial demonstration of the metal–ligand interaction of TC and Zn2+. (b) UV-Vis spectral changes of ligand TC (1 mM) upon the addition of Zn(OTf)2 (50 μL or 0.5 equiv. in CHCl3/CH3CN (1/1, v/v)). (c) PL spectral changes of ligand TC (1 mM) upon the addition of Zn(OTf)2 (50 μL or 1 equiv. in CHCl3/CH3CN (1/1, v/v)). Insets: Photoimages of TC in the absence and presence of Zn2+ in CHCl3/CH3CN (1/1, v/v) (λex = 300 nm for PL exp.). | |
1H-NMR titrations between ligand TC and Zn(OTf)2
To further explore insights into the formation of coordination compound Zn2+-TC2 with a molar ratio of the metal–ligand complex of 1
:
2, the coordination process was further investigated by 1H-NMR spectroscopy (400 MHz) in CDCl3/CD3CN (1/1, v/v) as shown in Fig. 2. Upon the addition of Zn2+ (0 to 0.5 equiv.), the chemical shifts of the pyridyl protons Ha and Hd in TC were shifted upfield to 0.96 and 0.120 ppm, while the downfield shifts of 0.186 and 0.23 ppm were observed for protons Hc and He, mainly ascribed to the shielding effect of the neighboring terpyridyl unit after complexation as illustrated in Fig. 2.
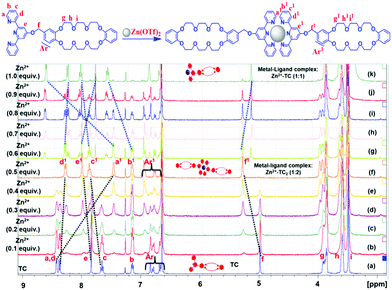 |
| Fig. 2
1H-NMR (400 MHz) titration during the formation of Zn2+-TC2 in CDCl3/CD3CN (1/1, v/v) with 1 equiv. of ligand TC complexed with increasing equivalents of Zn2+: (a) 0 equiv.; (b) 0.1 equiv.; (c) 0.2 equiv.; (d) 0.3 equiv.; (e) 0.4 equiv. (f) 0.5 equiv.; (g) 0.6 equiv.; (h) 0.7 equiv.; (i) 0.8 equiv.; (j) 0.9 equiv.; (k) 1.0 equiv. of Zn(OTf)2versus the terpyridyl unit (i.e., ligand TC). | |
The chemical shifts of the aromatic protons of crown ether DB24C8 (i.e., HAr) were shifted downfield along with a downfield shift of 0.3 ppm for the benzyl protons (Hf). According to 1H-NMR results, all of these chemical shifts are the characteristic properties of the efficient conversion from the free ligand form to the coordination compound Zn2+-TC2 with a metal–ligand complex molar ratio of 1
:
2. All these pieces of evidence are consistent with previously reported results,47,48 indicating the metal–ligand interactions between Zn2+ and terpyridine-based ligands. However, once the Zn2+ concentrations were oversupplied (i.e., 1 equiv. with an equal molar ratio of terpyridyl ligand TC), a third set of proton signals were ripened and gradually strengthened along with the deinsertion of the pre-formed Zn2+-TC2 (1
:
2) proton signals as shown in Fig. 2, signifying the formation of a 1
:
1 metal–ligand complex (Zn2+-TC) with the additional coordination sites saturated by solvent or triflate counterions.49,50
Optical switchable behaviour of guest DS
The optical switchable property of the diarylethene (DAE)-based secondary ammonium salt guest DS was measured in CHCl3/CH3CN (1/1, v/v) and demonstrated in Fig. 3(a) and Fig. S2 (ESI†). Upon UV-irradiation, the open-form of DAE in DS was optically switched to its closed-form, i.e., from the open-form of DS, i.e., DS(O) (O = open-form) to the closed-form of DS, i.e., DS(C) (C = closed-form). The UV/Vis spectral changes of DS during UV-irradiation are summarized in Fig. 3.
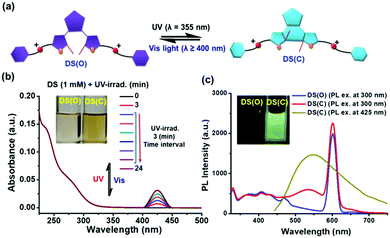 |
| Fig. 3 Optical-switchable DS(O & C) guest in CHCl3/CH3CN (1/1, v/v) before and after UV-irradiation (λ = 355 nm). (a) Pictorial diagram of DS(O & C) (the open to closed form) before and after UV-irradiation. (b) UV-Vis spectral changes of DS (from the open to closed form). Insets: Photoimages of DS(O) and DS(C) under UV-irradiation at 0 and 24 min, respectively. (c) PL spectral changes and photoimages (insets) of DS guest in CHCl3/CH3CN (1/1, v/v) before and after UV-irradiation (at λ = 355 nm for 24 min) (λex = 300 and 425 nm for PL exp.). | |
The UV-Vis spectrum of DS(O) exhibited absorption bands at 236 nm (n–π* transition of DAE) and 280 nm as shown in Fig. 3(b). After UV-irradiation (λ = 355 nm) for 0–24 min, a broad and intense absorption band at 425 nm was observed and saturated at 15 min due to the generation of DS(C) with the photo-cyclized form of the DAE unit as shown in Fig. 3(b). Even with a longer period of UV-irradiation (i.e., λ = 355 nm up to 24 min), the intensity of the absorption band at 425 nm was not altered and was thus saturated over 15 min of UV-irradiation for DS(C). On the contrary, DS(C) was reversible towards its original open-form DS(O) upon irradiation with visible light at λ ≥ 400 nm as illustrated in Fig. S2 (ESI†), where the de-cyclization process was completed within 25 min.
The PL spectral changes from DS(O) to DS(C) during UV-irradiation (i.e., λ = 355 nm up to 24 min) are illustrated in Fig. 3(c). Notably, during the photo-cyclization of the DAE unit, a less intense emission band at 545 nm was observed (λex = 300 nm for PL exp. with a quantum yield of 0.76% for DS(C) in CHCl3/CH3CN (1/1, v/v) medium by using quinine hemisulfate standard). This was due to the prohibition of the photo-induced electron transfer (PET) mechanism from the protonated ammonium group to the photo-cyclized form of DAE unit in DS(C) as shown in Fig. 3(c), which was also reported in the literature where the annihilation of PET (i.e., the PET-OFF process) was induced by the charge introduction to the fluorophore.51 However, the intensity of the emission band at 545 nm was 3-fold enhanced if DS(C) was excited at the newly photo-cyclized absorption band at λex = 425 nm, where the emission maxima of photo-cyclized DS(C) could be observed only at a critical concentration of ∼1 mM. We also explored the structural changes of the DAE unit in DS (from the open to the closed form) via1H-NMR spectroscopy as illustrated in Fig. S3 (ESI†). From the 1H-NMR spectra of DS(O) and DS(C) in Fig. S3 (ESI†), the chemical shift of the methyl proton (–CH3 group) on the thiophene ring of DAE in DS(O) is located at 1.92 ppm and the methyl proton (–CH3 group) is slightly shifted to up-field at 1.91 ppm for photo-cyclized DAE in DS(C). Moreover, after photo-cyclization, the methylene protons (i.e., –CH2 group, H4 and H5 protons) were shifted to up-field from 4.15 and 4.27 ppm to 4.14 and 4.25 ppm, respectively, which further confirmed the structural changes before and after UV-irradiation. Thus, the integrated areas of the methyl proton (–CH3 group) indicated 100% conversion of DS(O) to DS(C).
The formation of [2]pseudo-rotaxane polymer Zn2+-TC2/DS(O) via host–guest interactions
It is noteworthy that several research groups have explored the host–guest interactions between secondary ammonium salts and dibenzo-24-crown-8 (DB24C8) via the supportive hydrogen-bonding of [N+–H⋯O] and [C–H⋯O].16–18 Since we have investigated the formation of Zn2+-TC2 (1
:
2) by the complexation of Zn2+ with two equivalents of ligand TC containing DB24C8 linked to the terpyridyl terminus and the photo-switching behavior of the DAE unit from the open to the closed form in the guest DS bearing two terminal secondary ammonium salts in Fig. 1 and 3, respectively, a novel [2]pseudo-rotaxane polymer Zn2+-TC2/DS(O)via host–guest interaction is introduced in this study as illustrated in Fig. 4(a). We performed a series of important experiments to discover the host–guest interactions due to the impact of the optical-switchable properties of the DAE unit in the supramolecular polymer Zn2+-TC2/DS upon UV-irradiation and thus explored the associated properties of energy transfer from the metal–ligand complex Zn2+-TC2 to DS(C) by means of photo-cyclization events in the supramolecular polymer Zn2+-TC2/DS(C) as demonstrated in Fig. 5(a). For the formation of the supramolecular polymer Zn2+-TC2/DS(O), the corresponding PL spectral changes of metal–ligand complex Zn2+-TC2 that occurred upon the addition of DS(O) (1 mM or 1 equiv.) in CHCl3/CH3CN (1/1, v/v) are shown in Fig. 4(b). The emission bands of the metal–ligand complex Zn2+-TC2 at 338 and 417 nm were slightly increased, which was due to the host–guest complex formation of the supramolecular polymer Zn2+-TC2/DS(O) (1
:
1) and was saturated up to 100 μL of DS(O) in Fig. 4(b). According to Fig. 4(c), the host–guest complex formation between the Zn2+-TC2 host and DS(O) guest was verified by Job's plot with a molar ratio of 1
:
1 to generate the [2]pseudo-rotaxane polymer Zn2+-TC2/DS(O).
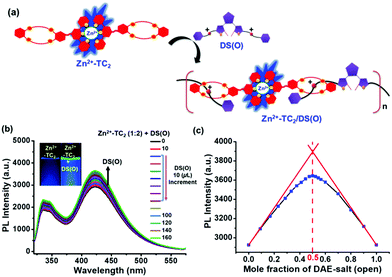 |
| Fig. 4 (a) Schematic demonstration of the supramolecular formation via the host–guest and metal–ligand interaction. (b) PL spectral changes of the metal–ligand complex Zn2+-TC2 (1 : 1) upon the addition of DS(O) (1 mM or 1 equiv.) in CHCl3/CH3CN (1/1, v/v). Insets: Photoimages of Zn2+-TC2 in the absence and presence of DS(O) in CHCl3/CH3CN (1/1, v/v). (c) Job's plot of the Zn2+-TC2/DS(O) complex upon the addition of DS(O) (1 mM or 1 equiv.) in CHCl3/CH3CN (1/1, v/v) (λex = 300 nm for PL exp.). | |
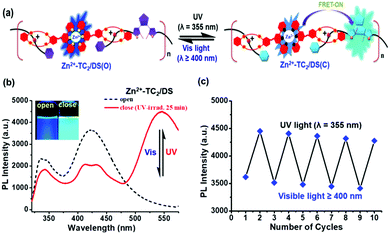 |
| Fig. 5 (a) Schematic demonstration of the optical-switchable behavior from the open to closed form of the DAE unit in the supramolecular polymer Zn2+-TC2/DS(O & C) (1 : 1) under UV and Vis light irradiation. (b) PL spectral changes of supramolecular polymer Zn2+-TC2/DS(C) after UV-irradiation (CHCl3/CH3CN (1/1, v/v). Insets: Photoimages of Zn2+-TC2/DS(O) and Zn2+-TC2/DS(C) under UV-irradiation at 0 and 25 min, respectively. (c) Reversible PL behavior at λ = 545 nm of Zn2+-TC2/DS(O to C) under UV and Vis light irradiation (λex = 300 nm for PL exp.). | |
Optical-switchable behaviour of the [2]pseudo-rotaxane polymer Zn2+-TC2/DS(O & C) under UV & vis-irradiation
It is well known that the photo-cyclized form of the DAE unit acts as an energy acceptor unit with a combination of other fluorophores.28–30 Therefore, we are more interested in the impact of the optical-switchable property of the DAE unit in the supramolecular polymer Zn2+-TC2/DS(C) after UV-irradiation and exploring the related properties of energy transfer from the metal–ligand complex Zn2+-TC2 to DS(C) by means of photo-cyclization events as demonstrated in Fig. 5(a). After UV-irradiation (λ = 355 nm), the corresponding PL spectral changes of supramolecular polymer Zn2+-TC2/DS(C) (1
:
1 mM in CHCl3/CH3CN 1/1, v/v) were saturated after 25 min, as illustrated in Fig. 5(b). In contrast to Zn2+-TC2/DS(O) before the photo-cyclization of the DAE unit, upon exposure to UV, the supramolecular polymer Zn2+-TC2/DS(C) showed a new major emission band of the DS(C) guest at 545 nm (with a quantum yield of 12.57% for DS(C) in CHCl3/CH3CN (1/1, v/v) medium by using quinine hemisulfate standard) and the emission band of the Zn2+-TC2 host at 417 nm decreased (with a quantum yield of 7.60% for Zn2+-TC2 in CHCl3/CH3CN (1/1, v/v) medium by using quinine hemisulfate standard) in Fig. 5(b) due to the FRET process from the energy donor Zn2+-TC2 host to the energy acceptor DS(C) guest (i.e., the FRET efficiency of supramolecular polymer Zn2+-TC2/DS(C), EFRET = 55%). As revealed in the inset of Fig. 5(b), the dark blue emission of the open form of the supramolecular polymer Zn2+-TC2/DS(O) changed to cyan (i.e., greenish-blue) for the closed form of Zn2+-TC2/DS(C).
Notably, the emission intensity of the photo-cyclized DAE unit at 545 nm was increased by about 10 times in the supramolecular polymer Zn2+-TC2/DS(C) (see Fig. 5) as compared to the emission intensity of the DS(C) guest (at 545 nm) alone (see Fig. 3(c)) due to the occurrence of the FRET event from the Zn2+-TC2 host to the DS(C) guest in addition to the prohibition of the PET phenomena from the ammonium group (i.e., coordinated with the macrocyclic host which acts as a strong donor) to the photo-cyclized DAE unit simultaneously in the supramolecular polymer Zn2+-TC2/DS(C). The “molecular wire and stimuli-responsive effects” of the supramolecular polymer also play a key role in enhancing the FRET behavior from the Zn2+-TC2 host to the DS(C) guest in this study.46 Therefore, it is significant that the exploration of the FRET behavior from the metal-coordinated Zn2+-TC2 host to the closed form of the DS(C) guest in our supramolecular polymer with two different PL emission peaks is much better clarified in contrast to the reported FRET in the monomeric system with a single PL emission peak.45 Moreover, the occurrence of the FRET event in the supramolecular polymer Zn2+-TC2/DS(C) is fully supported by the significant overlap of the emission (at 417 nm) in the Zn2+-TC2 host and the absorption (at 425 nm) in the DS(C) guest as shown in Fig. S4(a) (ESI†) (i.e., the integral of the overlapped area = 60%). As a result, the PL excitation spectrum of the supramolecular polymer Zn2+-TC2/DS(C) in Fig. S4(b) (ESI†) displayed the absorption bands of the Zn2+-TC2 host (306 and 333 nm) and the DS(C) guest (425 nm), which validated the occurrence of the FRET process in supramolecular polymer Zn2+-TC2/DS(C).
The preferential excitation of a donor fluorophore and recognition of the increased emission of an interacting acceptor fluorophore was proof of concept to support the FRET process.52 Therefore, another excitation-dependent control experiment was executed to confirm the FRET process from the Zn2+-TC2 host to the DS(C) guest in Zn2+-TC2/DS(C), where the emission spectra of Zn2+-TC2/DS(C) in CHCl3/CH3CN (1/1, v/v) solutions (1 mM) were recorded under various excitation wavelengths (from 300 to 330 nm) as shown in Fig. S5 (ESI†). It is worth noting that the optimal excitation wavelength for the occurrence of the strongest FRET process in Zn2+-TC2/DS(C) is 300 nm among all excitations (305, 310, 315, 320, 325 and 330 nm) owing to the formation of the isosbestic point (at 301 nm, see Fig. 1) when Zn2+ ions are coordinated with ligand TC. Thus, the supramolecular polymer Zn2+-TC2/DS(C) demonstrated excitation-dependent PL spectra which further confirmed the proposed FRET mechanism from Zn2+-TC2 to DS(C).
The reversibility of the energy transfer from the photo-cyclized DAE unit of DS(C) for the supramolecular polymer Zn2+-TC2/DS(C) to convert the de-cyclized DAE unit of DS(O) upon the irradiation of visible light at λ ≥ 400 nm without energy transfer for supramolecular polymer Zn2+-TC2/DS(O) was further verified as shown in Fig. 5(c). Therefore, the efficient energy transfer from the metal–ligand complex Zn2+-TC2 to the photo-cyclized DAE unit of DS(C) was only produced in the supramolecular polymer Zn2+-TC2/DS(C) by optical switching of the DAE unit from DS(O) to DS(C). According to Fig. S6 in the ESI,† the emissions of the Zn2+-TC2 host (at λmax = 417 nm) in the [2]pseudo-rotaxane polymer Zn2+-TC2/DS before and after UV-irradiation in CHCl3/CH3CN (1/1, v/v) displayed the fluorescence lifetime values of τ = 2.00 ns and τ = 1.40 ns, respectively. Thus, our findings for energy transfer from Zn2+-TC2 to DS(C) in Fig. 5 were further validated by the decrease in the fluorescence lifetime in the TRPL results of Fig. S6 (ESI†) for host emission in Zn2+-TC2/DS(C) under UV-irradiation.
1H-NMR titrations between the metal–ligand complex Zn2+-TC2 and DS(O) guest
The host–guest interactions between secondary ammonium salts and DB24C8 via supportive (N+–H⋯O) and (C–H⋯O) hydrogen-bonding in less polar solvents are well reported.16–181H-NMR measurements were carried out to prove the formation of the supramolecular polymer by mixing the DS(O) guest and metal–ligand complex Zn2+-TC2 in CDCl3/CD3CN (1/1, v/v) solution as demonstrated in Fig. 6. By mixing the equal molar ratio (1
:
1) of both the DS(O) guest and the Zn2+-TC2 host as shown in Fig. 6, both benzyl protons (H4, H5) of the DS(O) guest were shifted upfield by 0.35 and 0.15 ppm, respectively, and the alkyl ammonium protons (H6) split into a doublet with an upfield-shift of 0.89 ppm. Moreover, H7 protons of the thiophene ring in the DAE unit and aromatic protons Ar-H8 in the DS(O) guest were shifted downfield by 0.29 and 0.11 ppm, respectively. Likewise, the terpyridine and benzene aromatic protons (Ha, Hb, Hc, Hd, He and HAr) of the Zn2+-TC2 host were broadened and slightly downfield-shifted. According to the 1H-NMR results, the formation of the supramolecular polymer Zn2+-TC2/DS(O) was confirmed by the host–guest interaction of both the DS(O) guest and Zn2+-TC2 host.
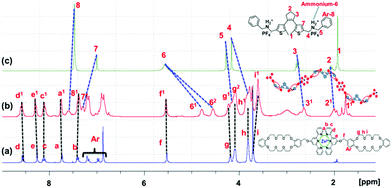 |
| Fig. 6
1H-NMR spectra (300 MHz, CDCl3/CD3CN (1/1, v/v) at 298 K for (a) the Zn2+-TC2 host, (b) the supramolecular polymer Zn2+-TC2/DS(O) and (c) the DS(O) guest. | |
The concentration-dependent 1H-NMR measurements for studying the formation of the supramolecular polymer Zn2+-TC2/DS(O) were carried out and are illustrated in Fig. S7 (ESI†) to indicate the gradual conversion from cyclic oligomers to a supramolecular polymer.53 Furthermore, the convincing results in the self-assembly mode between the Zn2+-TC2 host and guest DS(O & C) in supramolecular polymers were verified by two-dimensional diffusion-ordered NMR (DOSY) spectra (see Fig. S8 and S9, ESI†) and rotating-frame nuclear Overhauser effect NMR (ROESY) spectra (see Fig. S10, ESI†). Subsequently, we performed the SEM measurements by taking the morphological images of the metal-coordinated Zn2+-TC2 host and supramolecular polymers Zn2+-TC2/DS(O & C) to further elucidate the induced morphological changes via non-covalent interactions. As shown in Fig. S11 (ESI†), the metal-coordinated Zn2+-TC2 host exhibited a uniform morphological pattern of molten grains. There were no remarkable differences in the uniform morphological patterns between supramolecular polymers Zn2+-TC2/DS(O) and Zn2+-TC2/DS(C), which showed some granular images that were smaller as compared to the metal-coordinated Zn2+-TC2 host.
To compare the metal ion effects on the formation of supramolecular polymers, the demetalated component TC2/DS(C) was investigated for chelation with different metal ions (i.e., 0.5 equiv.) in CHCl3/CH3CN (1/1, v/v), and their PL spectra are shown in Fig. S12 (ESI†). Upon the addition of various metal ions Ag+, K+, Ca+, Na+, Cd2+, Cu2+, Fe2+, Hg2+, Mn2+, Ni2+, Zn2+, Pb2+, Al3+ and Fe3+ to demetalated TC2/DS(C) solutions in CHCl3/CH3CN (1/1, v/v), only Zn2+ and Cd2+ metal ions showed different extents of stimuli-responsive effects on the PL emissions of DS(C) at λ = 545 nm because of the FRET processes from emissive metal-coordination hosts Zn2+-TC2 and Cd2+-TC2 to the fluorescent DS(C) guest (in the closed form of the emissive DAE unit). Importantly, compared with the Cd2+-chelated supramolecular polymer Cd2+-TC2/DS(C), the formation of the supramolecular polymer Zn2+-TC2/DS(C) with Zn2+ ions displayed the most significant PL emission enhancement as verified by the appearance of the corresponding emission bands of the metal-coordinated Zn2+-TC2 host at 338 and 417 nm, as well as the DS(C) guest at 545 nm shown in Fig. S12 (ESI†), which might be due to the distinct electronic structures of chelating Zn2+ and Cd2+ metal ions. Therefore, the excellent selectivity of the demetalated component TC2/DS(C) toward Zn2+ ions over the other metal ions was verified in the pronounced PL response during the formation of supramolecular polymer Zn2+-TC2/DS(C).
A study of the [2]pseudo-rotaxane polymer Zn2+-TC2/DS(O) by concentration-dependent viscometry measurements
The viscometry studies were performed to prove the formation of the supramolecular polymer Zn2+-TC2/DS(O), where the polyelectrolyte effect was excluded by adding 0.05 M tetrabutylammonium hexafluorophosphate in the specific viscosity measurements in CDCl3/CD3CN (1/1, v/v).54 A double logarithmic plot of the specific viscosity versus the equal concentration of the DS(O) guest and Zn2+-TC2 host (1
:
1) is shown in Fig. S11 (ESI†), where the slopes of the curves were 0.0075 and 0.0253 in the low and high concentration ranges, respectively. The formation of the supramolecular polymer Zn2+-TC2/DS(O) was verified in Fig. S11 (ESI†) and calculated to be 25 mM as the critical polymerization concentration (CPC) at the slope change point, which indicated a ring-chain transition from cyclic oligomers to a linear supramolecular polymer.55
Dis-assembly/re-assembly of the [2]pseudo-rotaxane polymer Zn2+-TC2/DS(O & C) via interactions with base/acid
The impacts of the chemical-stimuli-responsive supramolecular assembly (i.e., host–guest interaction) on energy modulations from the Zn2+-TC2 host to the DS(C) guest in supramolecular polymer Zn2+-TC2/DS(C) were investigated. As illustrated in Fig. 7(a), the secondary ammonium salt was converted to free amine under a basic environment with trimethylamine (TEA), and it was reversed to the secondary ammonium salt under an acidic environment with trifluoroacetic acid (TFA), which induced the dis-assembly and re-assembly of the supramolecular polymer Zn2+-TC2/DS(C), respectively. The corresponding UV/Vis and PL spectral changes are displayed in Fig. 7(b) and (c), respectively. As demonstrated in Fig. 7(b), upon the addition of base (TEA), the absorption bands at 311 and 322 nm of Zn2+-TC2 host and at 425 nm of DS(C) guest in supramolecular polymer Zn2+-TC2/DS(C) were slightly reduced and were regained via the addition of an equal amount of acid (TFA). The corresponding PL spectra were obtained upon the addition of base (TEA); the dis-assembly of supramolecular polymer Zn2+-TC2/DS(C) occurred to turn off the FRET process and the recovered emissions of the monomer component of the Zn2+-TC2 host appeared at 338 and 422 nm, but the emission peak of neutralized-DS(C) at 545 nm was completely quenched. As illustrated in Fig. 3(c), this result confirmed the PET-ON process51 from the neutralized ammonium group to the photo-cyclized DAE unit, owing to the removal of charge as compared with the emission of the protonated DS(C) guest at 545 nm. Accordingly, the FRET-OFF process was induced by the disassembly of the host–guest interaction of the photo-cyclized supramolecular polymer Zn2+-TC2/DS(C) resulting in the full recovery of the emission intensity of the Zn2+-TC2 host along with the PET-ON process of neutralized-DS(C).
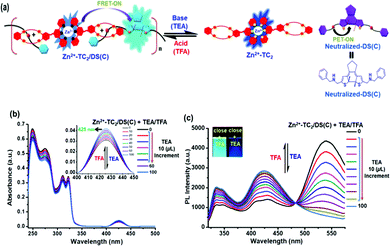 |
| Fig. 7 (a) Pictorial demonstration of supramolecular polymer Zn2+-TC2/DS(C) (1 : 1) dis-assembly/re-assembly by means of interactions with base/acid in CHCl3/CH3CN (1/1, v/v). (b) UV/Vis spectral changes of Zn2+-TC2/DS(C) upon the addition of TEA (0 to 100 μL) and TFA (0 to 100 μL) in CHCl3/CH3CN (1/1, v/v). (c) PL spectral changes of Zn2+-TC2/DS(C) upon the addition of TEA (0 to 100 μL) and TFA (0 to 100 μL) in CHCl3/CH3CN (1/1, v/v). Insets: Photoimages of Zn2+-TC2/DS(C) with acid/base in CHCl3/CH3CN (1/1, v/v). (λex = 300 nm for PL exp.). | |
After the addition of an equal amount of acid (TFA), the reversible PL behavior and the recovery of energy transfer from host to guest were observed to prove the re-assembly of supramolecular polymer Zn2+-TC2/DS(C) in Fig. 7(c). As illustrated in the inset of Fig. 7(c), the cyan emission color (i.e., greenish-blue) for supramolecular polymer Zn2+-TC2/DS(C) changed to dark blue for free components of the Zn2+-TC2 host and the neutralized-DS(C) guest after the dis-assembly of the supramolecular polymer. Likewise, similar experimental findings of the dis-assembly/re-assembly of supramolecular polymer Zn2+-TC2/DS(O)via base/acid interactions were also observed as summarized in Fig. S12 (ESI†). According to this result, the reversible chemical-stimuli-responsive energy transfer of the dis-assembly/re-assembly of supramolecular polymer Zn2+-TC2/DS(C)via the addition of base/acid was confirmed and can be used for the ratiometric sensing of pH. Therefore, the FRET process from the Zn2+-TC2 host to the turn-on fluorescence of the DS(C) guest in the closed form of the emissive DAE unit through the supramolecular host–guest interactions was further enhanced by the significant “molecular wire and stimuli-responsive effects” of supramolecular polymer Zn2+-TC2/DS(C) in this study.
Dis-assembly/re-assembly of [2]pseudo-rotaxane polymer Zn2+-TC2/DS(O & C)via interactions with chelating agent (cyclen)/Zn(OTf)2
It is noteworthy that cyclen (1,4,7,10-tetraazacyclododecane) has a higher binding affinity towards Zn2+ in contrast to terpyridine.56 Therefore, we performed experiments to investigate the chelation capability of Zn2+ ions via interactions with the competing ligand (cyclen) in the demetalation/re-metalation supramolecular polymer Zn2+-TC2/DS(C) as depicted in Fig. 8(a). The corresponding UV/Vis and PL spectral changes in the supramolecular polymer Zn2+-TC2/DS(C)via interactions with chelating agent cyclen and Zn(OTf)2 are displayed in Fig. 8(b) and (c). Upon the addition of cyclen, the absorption bands of 311 and 322 nm of Zn2+-TC2 host in supramolecular polymer Zn2+-TC2/DS(C) were diminished completely and the absorption bands of 311 and 322 nm were regained via the addition of an equal amount of Zn(OTf)2, respectively, as shown in Fig. 8(b).
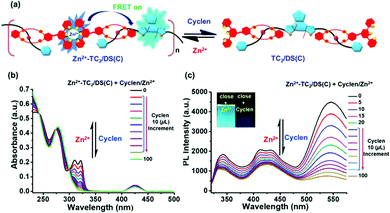 |
| Fig. 8 (a) Pictorial demonstration of supramolecular polymer Zn2+-TC2/DS(C) (1 : 1) dis-assembly/re-assembly by means of interactions of cyclen/Zn2+ in CHCl3/CH3CN (1/1, v/v). (b) UV/Vis spectral changes of Zn2+-TC2/DS(C) upon the addition of cyclen (0 to 100 μL) and Zn(OTf)2 in CHCl3/CH3CN (1/1, v/v). (c) PL spectral changes of Zn2+-TC2/DS(C) upon the addition of cyclen (0 to 100 μL) and Zn(OTf)2 in CHCl3/CH3CN (1/1, v/v). Insets: Photoimages of Zn2+-TC2/DS(C) in the absence and presence of cyclen (0 and 100 μL) (λex = 300 nm for PL exp.). | |
Upon the addition of cyclen, related PL spectral changes in the emission bands at 338 and 417 nm of Zn2+-TC2 host and the emission band at 545 nm of DS(C) guest in the supramolecular polymer Zn2+-TC2/DS(C) were all quenched significantly, which was due to the dis-assembly of the supramolecular polymer into the demetalated component TC2/DS(C) without energy transfer due to the lack of zinc-coordinated emission centers as shown in Fig. 8(c). On the contrary, after the addition of an equal amount of Zn(OTf)2, the re-assembly of supramolecular polymer Zn2+-TC2/DS(C) was further verified by the re-appearance of the corresponding emission bands as shown in Fig. 8(c). Similarly, the comparable experimental findings of the dis-assembly/re-assembly of supramolecular polymer Zn2+-TC2/DS(O)via chelating agent cyclen and Zn(OTf)2 were also observed as illustrated in Fig. S13 (ESI†). Hence, the reversible chelation-stimuli-responsive energy transfer for the demetalation/re-metalation of supramolecular polymer Zn2+-TC2/DS(C)via the addition of cyclen and Zn(OTf)2 was confirmed.
To investigate the temperature effect on the reversibility of non-covalent interactions in our supramolecular polymer, the PL spectral changes of the supramolecular polymer Zn2+-TC2/DS(C) in CHCl3/CH3CN (1/1, v/v) were measured within the temperature range of 40–60 °C as shown in Fig. S16 (ESI†). When the temperature was increased above 40 °C, the fluorescence intensity of Zn2+-TC2/DS(C) showed slight decreases in the emission maxima of the DS(C) guest (i.e., at 545 nm) in the supramolecular polymer Zn2+-TC2/DS(C), due to the reduction of the supramolecular interactions between the metal-coordinated Zn2+-TC2 host and the DS(C) guest in supramolecular polymer Zn2+-TC2/DS(C) by heating. The reversible fluorescence changes with temperature variations were maintained, but there were fewer PL changes affected by temperature in contrast to TEA and chelating agents in supramolecular systems (see Fig. 7 and 8). Accordingly, normal reduced non-covalent interactions by heating and reversible thermal-responsive phenomena were observed in the supramolecular polymer Zn2+-TC2/DS(C).
Conclusion
We have designed and constructed optical-switchable diarylethene-based [2]pseudo-rotaxane polymers Zn2+-TC2/DS(O & C) to elucidate the optical and multi-stimuli responsive energy transfer from the metal-coordinated Zn2+-TC2 host to the fluorescent DS(C) guest in the closed form of the emissive DAE unit. The confirmation of the supramolecular polymer Zn2+-TC2/DS(O & C) was further validated by 1H-NMR, UV/Vis and PL spectra along with specific viscosity measurements. Spectroscopic studies revealed that upon the UV irradiation of λ = 355 nm to cyclize the DAE unit of DS(C), the promoted FRET-ON process from the fluorescent metal-coordinated Zn2+-TC2 host to the PET-OFF DS(C) guest in the closed form of the emissive DAE unit was predominantly produced in the supramolecular polymer Zn2+-TC2/DS(C). The reversibility of energy transfer can be controlled by the de-cyclized DAE unit of DS(O) upon the irradiation of visible light at λ ≥ 400 nm to diminish energy transfer for supramolecular polymer Zn2+-TC2/DS(O). Endowed with the promoted FRET process, our supramolecular polymer displayed ratiometric PL emission peaks that could demonstrate a prominent FRET process triggered via multi-stimuli responses. In the dis-assembly/re-assembly of the host–guest interactions of the supramolecular polymer Zn2+-TC2/DS(C) in response to base/acid (TEA/TFA), the FRET-OFF process was induced by the disassembly of the host–guest interactions of photo-cyclized supramolecular polymer Zn2+-TC2/DS(C), resulting in the full recovery of the emission intensity of the Zn2+-TC2 host along with the PET-ON process of neutralized-DS(C). The reversible chelation-stimuli responsive energy transfer for the demetalation/re-metalation of the supramolecular polymer Zn2+-TC2/DS(C)via the addition of cyclen and Zn(OTf)2 was further confirmed by its controllable energy transfer. All these attractive findings illustrate the efficient control of energy transfer by the multiple-responsive capabilities of the host–guest, metal–ligand and photoactive interactions in the [2]pseudo-rotaxane polymer Zn2+-TC2/DS(C), which were investigated for the first time in this study. This can provide ideal viewpoints for the development of multi-responsive and photo-switchable energy transfer for versatile supramolecular applications.
Experimental section
Materials and characterization
All reagents and starting materials were commercially obtained with high purities and the solvents were dried by distillation under appropriate drying agents. In general, the progress of the reactions was monitored using TLC plates and the desired compounds were purified by column chromatography on silica gel. The characterization of the precursors and target compounds was performed via1H and 13C NMR spectra (recorded on a 300 MHz spectrometer and samples were dissolved in CDCl3 and DMSO-d6), mass spectra (HRMS, (obtained on a mass spectrometer) and elemental analysis (carried out using an Elemental Varo EL)). The chemical shifts were expressed in ppm and coupling constants (J) in Hz. UV-Vis absorption and fluorescence spectra were measured on a V-670 spectrophotometer and F-4500 fluorescence spectrophotometer, respectively. The UV-irradiation was carried out using a 500 W xenon arc lamp (Solar Electro-Optics Co., Ltd) with an intensity of 1.17 mW cm−2 as the light source.
The synthetic routes to obtaining the target components of the novel optical-switchable supramolecular polymers via multiple-responsive host–guest and metal–ligand interactions are outlined in Scheme 1. Zn2+ metal ion was coordinated with two TC ligands containing a macrocycle (DB24C8) linked to a terpyridyl terminus to form the Zn2+-TC2 host and diarylethene (DAE) bearing two terminal secondary ammonium salts to form the DS guest.
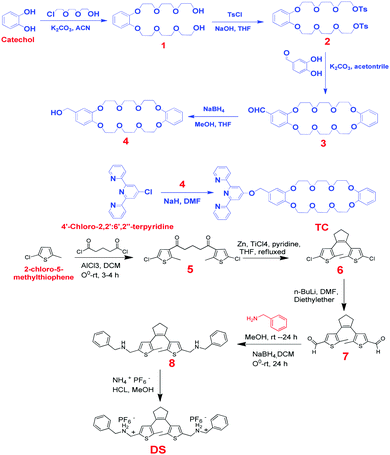 |
| Scheme 1 Synthetic routes to the ligand (TC) and guest (DS). | |
Commercially available starting compounds (catechol and 2-[2-(2-chloroethoxy)ethoxy]ethanol) have been used to obtain the intermediates 1 and 2 by tosylation protection of hydroxyl groups. Intermediate 3 was obtained by the cyclization of intermediate 2 with 3,4-dihydroxybenzaldehyde under refluxing conditions. Intermediate 4 was obtained by using reducing agent NaBH4. After that, ligand TC was synthesized with the tri-pyridyl intermediate under NaH reaction conditions with a desirable yield. Intermediate 7 was successfully synthesized in our previous report30 and was used in this study to synthesize the target guest DSvia the corresponding condensation with benzylamine to generate the Schiff base intermediate 8, where the reduction of compound 7 by NaBH4 produced the secondary amine 8, followed by sequential treatments with HCl and NH4PF6 to acquire guest DS. The synthetic routes of all intermediates and target compounds are provided in detail with their characterization data as follows.
Synthesis of compound 1
Catechol (5.5 g, 50 mmol), K2CO3 (20.81 g, 150 mmol) and KI (2.52 g, 12.50 mmol) were dissolved in acetonitrile (CH3CN, 500 mL) at room temperature under a N2 gas atmosphere. The reaction mixture was stirred at 40 °C for 1 h and followed by the addition of 2-[2-(2-chloroethoxy)ethoxy]ethanol (13 mL, 150 mmol) dropwise in the above reaction mixture and refluxed at 80 °C for 36 h. The progress of the reaction was monitored by TLC. After completion, the reaction was cooled to room temperature, filtered and evaporated to get the crude residue. The crude residue was subjected directly to column chromatography (silica gel, ethyl acetate/hexane = 3/2 and then ethyl acetate/MeOH = 9/1) to afford the red-brown oily desired precursor 1 with a yield of 13.5 g (72.1%).
1H-NMR (300 MHz, CDCl3): δ = 3.32 (s, 2H), 3.56–3.59 (m, 4H), 3.64–3.74 (m, 12H), 3.84–3.87 (m, 4H), 4.13–4.16 (m, 4h), 6.88 (s, 4H).; 13C-NMR (75 MHz, CDCl3): δ = 61.3, 68.5, 69.5, 70.1, 70.6, 72.6, 114.3, 121.5, 148.6; HRMS (ESI): [M + Na]+(calcd for C18H30NaO8): m/z = 397.1833; found: 397.1839; elemental analysis: calcd for C18H30O8: C = 57.74; H = 8.08; O = 34.18, found: C = 57.70; H = 8.08; O = 34.22.
Synthesis of compound 2
Compound 1 (5 g, 13.35 mmol) was dissolved in THF (200 mL) and cooled to 0 °C by using an ice bath. Then, NaOH (4.31 g, 106.85 mmol) was dissolved in H2O (100 mL) and added to the above reaction mixture with stirring for 30 min. After that, 4-toluenesulfonyl chloride (TsCl, 10.21 g, 53.42 mmol) was dissolved in THF (200 mL) and added dropwise to the above reaction mixture with vigorous stirring at 0 °C for up to 1 h. The reaction mixture was allowed to stir at room temperature overnight. The progress of the reaction was monitored by TLC. After completion, the reaction mixture was diluted with DCM (200 mL) and neutralized by 0.1 N HCl (200 mL × 2). The organic layer was separated and washed with a saturated solution of NaCl (200 mL × 2). The organic layer was dried over MgSO4, filtered and evaporated to get the crude residue, which was purified by column chromatography (silica gel, ethyl acetate/hexane = 3/2) to give a colorless oily compound 2 with a yield of 7.05 g (i.e., 78%).
1H-NMR (300 MHz, CDCl3): δ = 2.41 (s, 6H), 3.44–3.47 (m, 4H), 3.51–3.58 (m, 8H), 3,68 (m, 4H), 4.03–4.11 (m, 8H), 6.86–6.98 (m, 4H), 7.32 (d, J = 8.3 Hz, 4H), 7.78 (d, J = 8.3 Hz, 4H); 13C NMR (75 MHz, CDCl3): δ = 21.6, 68.6, 68.7, 69.4, 69.7, 70.6, 70.7, 114.6, 121.6, 127.9, 129.9, 132.8, 144.9, 148.8; HRMS (ESI): [M + 1]+(calcd for C32H43O12S2): m/z = 683.2190; found: 683.2190; elemental analysis for C32H42O12S2: calculated: C = 56.29; H = 6.20; O = 28.12; S = 9.39, found: C = 56.33; H = 6.19; O = 28.08; S = 9.40.
Synthesis of compound 3
3,4-Dihydroxybenzaldehyde (1.38 g, 9.99 mmol) and K2CO3 (16.30 g, 49.95 mmol) were mixed in acetonitrile (300 mL) at room temperature. The reaction mixture was heated under reflux for 1 h, followed by the addition of compound 2 (6.83 g, 9.99 mmol) in acetonitrile (100 mL) during reflux and allowed to stir for 24 h. The progress of the reaction mixture was monitored by TLC. After completion, the reaction mixture was cooled to room temperature and the solvent was removed by evaporation. The crude residue was dissolved in DCM (200 mL) and washed with 1 N HCl and saturated NaCl aqueous solution. After drying with MgSO4, filtration and removal of solvent, the crude product was purified by column chromatography (SiO2, ethyl acetate/MeOH = 10/1) to give a yellowish solid compound 3 with a yield of 3.7 g (i.e., 77% w/w).
1H-NMR (300 MHz, CDCl3): δ = 3.74(s, 8H), 3.78–3.95 (m, 8H), 4.19–4.33 (m, 8H), 6.96 (s, 4H), 7.09 (d, 1H), 7.47–7.53 (m, 2H), 9.84 (s, 1H); 13C-NMR (75 MHz, CDCl3): δ = 67.4, 67.7, 68.4, 69.7, 111, 116.9, 122.9, 123.6, 127.7, 131.4, 147.4, 148.1, 153.8, 162.3, 190.7; HRMS (ESI+): [M + Na]+ (calcd for C25H32NaO9): m/z = 499.1939; found: 499.1947.; Elemental Analysis: Calculated for C25H32O9: C = 63.01; H = 6.77; O = 30.22, found: C = 63.05; H = 6.75; O = 30.20.
Synthesis of compound 4
Compound 3 (2 g, 4.19 mmol) was dissolved in EtOH/CH2Cl2 (v/v = 50/50 mL) and the solution was cooled to 0 °C. NaBH4 (0.79 g, 12.59 mmol) was added slowly to the reaction mixture, which was allowed to warm up to room temperature and kept stirring overnight. The progress of the reaction was monitored by TLC. After completion, the reaction mixture was quenched by the addition of water (100 mL) and the product was extracted with CH2Cl2 (100 mL × 2). The combined organic layer was dried with MgSO4, filtered and the solvent removed to give a white solid compound 4 with a yield of 1.81 g (i.e., 90%).
1H-NMR (300 MHz, DMSO-d6): δ = 3.67 (s, 8H), 3.74–3.82 (m, 8H), 4.04–4.21 (m, 8H), 4.52 (s, 2H), 6.74–6.84 (m, 7H); 13C-NMR (75 MHz, CDCl3): δ = 69.4, 69.9, 71.2, 113.7, 114.1, 121.0, 121.4, 131.4, 148.4, 148.9; HRMS (ESI): [M + Na]+(calcd for C25H34NaO9): m/z = 501.2095; found: 501.2104; elemental analysis: calcd for C25H34O9: C = 62.75; H = 7.16; O = 30.09, found: C = 62.73; H = 7.17; O = 30.10.
Synthesis of compound TC
NaH (60%, 0.25 g, 6.25 mmol) was added to a stirred suspension of compound 4 (0.61 g, 1.25 mmol) in anhydrous DMF (15.0 mL) at 70 °C. After stirring for 30 min, 4′-chloro-2,2′:6′,2′′-terpyridine (0.37 g, 1.4 mmol) was added to the mixture and stirred for 48 h at 70 °C. After the reaction, the mixture was cooled to room temperature and DMF was removed by evaporation. The residue was dissolved in CHCl3 (100 mL) and washed with saturated NaCl aqueous solution. The organic layer was dried with MgSO4, filtered and concentrated by evaporation. The crude product TC was purified by column chromatography (neutral, aluminum oxide, DCM/hexane = 7/3 to EtOAc/DCM = 3/7) to give a white solid compound 5 with a yield of 0.75 g (i.e., 85%).
1H-NMR (300 MHz, CDCl3): δ = 3.82 (s, 8H), 3.91 (d, 8H) 4.12–4.18 (m, 8H), 21 (s, 2H), 6.85 (s, 5H), 6.92 (d, 2H), 7.29–7.34 (m, 2H), 7.80–7.86 (m, 2H), 8.09 (s, 2H), 8.61 (d, 2H, J = 7.9 Hz), 8.68 (d, 2H, J = 4.7 Hz).; 13C-NMR (75 MHz, CDCl3): δ = 69.4, 69.5, 69.9, 71.3, 107.7, 113.5, 113.8, 114.1, 120.8, 121.4, 121.5, 123.9, 129.1, 136.9, 149.1, 156.1, 157.2, 162.4, 167.0; HRMS (ESI): [M + Na]+(calcd for C40H43N3NaO9): m/z = 732.2892; found: 732.2884; elemental analysis: calculated for C40H43N3O9: C = 67.69; H = 6.11; N = 5.92; O = 20.29, found: C = 67.64; H = 6.10; N = 5.94; O = 20.32.
Synthesis of intermediates 5, 6 and 7
Intermediates 5, 6 and 7 were successfully synthesized as indicated in our previous report +.30
Synthesis of compound 8
Compound 7 (1 g, 3.16 mmol) and benzyl amine (0.92 g, 9.48 mmol) were dissolved in methanol (100 mL) at room temperature and allowed to stir for 24 h. After completion, the methanol was removed by evaporation and the residue was dissolved in CH2Cl2 (100 mL) and cooled to 0 °C using an ice bath. Then, NaBH4 (0.54 g, 15.8 mmol) was added in portions and the suspension was stirred for 1 h at 0 °C and further reacted for another 24 h at room temperature. The progress of the reaction was monitored by TLC and the reaction mixture was quenched with water (100 mL) after completion. The organic layer was separated and washed with water (50 mL × 2), then dried with MgSO4, filtered and concentrated by evaporation. The residue was purified by column chromatography (silica gel, ethyl acetate/hexane = 6
:
4) to give an orange-colored oily compound 8 with a yield of 1 g (i.e., 70%).
1H-NMR (300 MHz, DMSO-d6): δ = 1.86 (s, 6H), 1.96 (m, 2H), 2.71 (m, 4H), 3.61 (s, 4H), 3.68(s, 4H), 6.61 (s, 2H), 7.19–7.33 (m, 10H). 13C-NMR (75 MHz, CDCl3): δ = 14.44, 23.12, 38.46, 47.47, 52.43, 126.64, 127.20, 128.47, 128.56, 133.79, 134.62, 1336, 138.89, 139.77. HRMS (ESI): [M + 1]+ (calcd for C31H35N2S9): m/z = 499.2236; found: 499.2236. Elemental analysis: calculated for C31H34N2S2: C = 74.65; H = 6.87; N = 5.62; S = 12.86, found: C = 74.66; H = 6.90; N = 5.59; S = 12.85.
Synthesis of compound DS
Compound 8 (0.5 g, 1 mmol) was dissolved in methanol (50 mL) and conc. HCL was added to adjust the pH to less than 2, then the solvent was removed by evaporation to get the crude residue. After that, the crude residue was suspended in acetone (50 mL), followed by the addition of a saturated aqueous solution of NH4+PF6− until the suspension became clear. The solvent was removed by evaporation and the residue was filtered, and washed with water (200 mL). The product was dried in a vacuum oven at 100 °C to give a yellow solid guest DS with a yield of 0.75 g (i.e., 95%).
1 H-NMR (300 MHz, CD3CN): δ = 1.92 (s, 6H), 2.06 (m, 2H), 2.77 (m, 4H), 4.16 (s, 4H), 4.27 (s, 4H), 5.56 (s, 2H), 6.99 (s, 2H), 7.46 (s, 10H); 13C-NMR (75 MHz, CD3CN): δ = 14.5, 23.7, 39.1, 46.4, 51.8, 128.2, 130.1, 130.8, 131.2, 133.5, 135.8, 137.2, 183.7; elemental analysis: calculated for C31H36N2S9: C = 47.09; H = 4.59; N = 3.54; S = 8.11, found: C = 47.08; H = 4.61; N = 3.53; O = 8.12.
Conflicts of interest
There are no conflicts to declare.
Acknowledgements
The financial supports are provided by the Ministry of Science and Technology (MOST) in Taiwan through MOST 103-2113-M-009-018-MY3, MOST 103-2221-E-009-215-MY3, MOST 106-2113-M-009-012-MY3 and MOST 107-3017-F009-003 and Ministry of Education, Taiwan (SPROUT Project-Center for Emergent Functional Matter Science of National Chiao Tung University). In addition, thanks to Ms. Shou-Ling Huang of Ministry of Science and Technology (Instrumentation Center at National Taiwan University) for the assistance in two-dimensional diffusion-ordered (DOSY) and rotating-frame nuclear overhauser effect (ROESY) (500 MHz NMR) experiments.
References
- T. F. A. De Greef, M. M. J. Smulders, M. Wolffs, A. P. H. J. Schenning, R. P. Sijbesma and E. W. Meijer, Supramolecular polymerization, Chem. Rev., 2009, 109, 5687–5754 CrossRef CAS.
- T. Aida, E. W. Meijer and S. I. Stupp, Functional supramolecular polymers, Science, 2012, 335, 813–817 CrossRef CAS.
- X. Yan, F. Wang, B. Zheng and F. Huang, Stimuli-responsive supramolecular polymeric materials, Chem. Soc. Rev., 2012, 41, 6042–6065 RSC.
- F. Xu, J. Zhang, P. Zhang, X. Luan and Y. Mai, “Rod-coil’’ copolymers get self-assembled in solution, Mater. Chem. Front., 2019, 3, 2283–2307 RSC.
- D. Sakamaki, S. Ghosh and S. Seki, Dynamic covalent bonds: approaches from stable radical species, Mater. Chem. Front., 2019, 3, 2258–2282 RSC.
- M. Wehner and F. Wurthner, Supramolecular polymerization through kinetic pathway control and living chain growth, Nat. Rev. Chem., 2020, 4, 38–53 CrossRef CAS.
- Y. Zhang, Y. Qi, S. Ulrich, M. Barboiu and O. Ramstrom, Dynamic covalent polymers for biomedical applications, Mater. Chem. Front., 2020, 4, 489–506 RSC.
- P. Wei, X. Yan and F. Huang, Supramolecular polymers constructed by orthogonal self-assembly based on host–guest and metal–ligand interactions, Chem. Soc. Rev., 2015, 44, 815–832 RSC.
- J. Hatai, C. Hirschhauser, J. Niemeyer and C. Schmuck, Multi-stimuli-responsive supramolecular polymers based on noncovalent and dynamic covalent bonds, ACS Appl. Mater. Interfaces, 2020, 12, 2107–2115 CrossRef CAS.
- C.-W. Chu, L. Stricker, T. M. Kirse, M. Hayduk and B. J. Ravoo, Light-responsive arylazopyrazole gelators: from organic to aqueous media and from supramolecular to dynamic covalent chemistry, Chem. – Eur. J., 2019, 25, 6131–6140 CrossRef CAS.
- S. Lee, K. Y. Kim, S. H. Jung, J. H. Lee, M. Yamada, R. Sethy, T. Kawai and J. H. Jung, Finely controlled circularly polarized luminescence of a mechano-responsive supramolecular polymer, Angew. Chem., Int. Ed., 2019, 58, 18878–18882 CrossRef CAS.
- Y. Niu, X. Yuan, Y. Zhao, W. Zhang and L. Ren, Temperature and pH dual-responsive supramolecular polymer hydrogels hybridized with functional inorganic nanoparticles, Macromol. Chem. Phys., 2017, 218, 1600540 CrossRef.
- T. Xiao, L. Qi, W. Zhong, C. Lin, R. Wang and L. Wang, Stimuli-responsive nanocarriers constructed from pillar[n]arene-based supra-amphiphiles, Mater. Chem. Front., 2019, 3, 1973–1993 RSC.
- Z. Xu, D. Gonzalez-Abradelo, J. Li, C. A. Strassert, B. J. Ravoo and D.-S. Guo, Supramolecular color-tunable photo-luminescent materials based on a chromophore cascade as security inks with dual encryption, Mater. Chem. Front., 2017, 1, 1847–1852 RSC.
- N. Song, X.-Y. Lou, H. Yu, P. S. Weiss, B. Z. Tang and Y.-W. Yang, Pillar[5]arene-based tunable luminescent materials via supramolecular assembly-induced forster resonance energy transfer enhancement, Mater. Chem. Front., 2020, 4, 950–956 RSC.
- B. Zheng, F. Wang, S. Dong and F. Huang, Supramolecular polymers constructed by crown ether-based molecular recognition, Chem. Soc. Rev., 2012, 41, 1621–1636 RSC.
- M. Sarma, T. Chatterjee and S. K. Das, Ammonium–crown ether based host–guest systems: N–H⋯O hydrogen bond directed guest inclusion featuring N–H donor functionalities in angular geometry, RSC Adv., 2012, 2, 3920–3926 RSC.
- H. Zhu, L. Shangguan, B. Shi, G. Yu and F. Huang, Recent progress in macrocyclic amphiphiles and macrocyclic host-based supra-amphiphiles, Mater. Chem. Front., 2018, 2, 2152–2174 RSC.
- R. Shunmugam, G. J. Gabriel, K. A. Aamer and G. N. Tew, Metal–ligand–containing polymers: terpyridine as the supramolecular unit, Macromol. Rapid Commun., 2010, 31, 784–793 CrossRef CAS.
- Y. Li, D. J. Young and X. J. Loh, Fluorescent gels: a review of synthesis, properties, applications and challenges, Mater. Chem. Front., 2019, 3, 1489–1502 RSC.
- S. Chakraborty and G. R. Newkome, Terpyridine-based metallosupramolecular constructs: tailored monomers to precise 2D-motifs and 3D-metallocages, Chem. Soc. Rev., 2018, 47, 3991–4016 RSC.
- G.-Q. Yin, H. Wang, X.-Q. Wang, B. Song, L.-J. Chen, L. Wang, X.-Q. Hao, H.-B. Yang and X. Li, Self-assembly of emissive supramolecular rosettes with increasing complexity using multitopic terpyridine ligands, Nat. Commun., 2018, 9, 567–577 CrossRef.
- J. Huang, D. Liu, S.-C. Wang, M. Chen, H. Zhao, K. Li, Y.-T. Chan and P. Wang, Molecular lemniscates from organic-metal terpyridine-based self-assembly and host−guest recognition, Inorg. Chem., 2019, 58, 5051–5057 CrossRef CAS.
- L. Wang, B. Song, S. Khalife, Y. Li, L.-J. Ming, S. Bai, Y. Xu, H. Yu, M. Wang, H. Wang and X. Li, Introducing seven transition metal ions into terpyridine-based supramolecules: self-assembly and dynamic ligand exchange study, J. Am. Chem. Soc., 2020, 142, 1811–1821 CrossRef CAS.
- M. Irie, Diarylethenes for memories and switches, Chem. Rev., 2000, 100, 1685–1716 CrossRef CAS.
- J. Zhang and H. Tian, The endeavor of diarylethenes: new structures, high performance and bright future, Adv. Opt. Mater., 2018, 6, 1701278 CrossRef.
- Z. Zhang, W. Wang, P. Jin, J. Xue, L. Sun, J. Huang, J. Zhang and H. Tian, A building-block design for enhanced visible-light switching of diarylethenes, Nat. Commun., 2019, 10, 4232–4241 CrossRef.
- S.-Z. Pu, Q. Sun, C.-B. Fan, R.-J. Wang and G. Liu, Recent advances in diarylethene-based-multi-responsive molecular switches, J. Mater. Chem. C, 2016, 4, 3075–3093 RSC.
- T. Nakahama, D. Kitagawa, H. Sotome, S. Ito, H. Miyasaka and S. Kobatake, Fluorescence on/off switching in polymers bearing diarylethene and fluorene in their side chains, J. Phys. Chem. C, 2017, 121, 6272–6281 CrossRef CAS.
- R. Singh, H. Y. Wu, A. K. Dwivedi, A. Singh, C. M. Lin, P. Raghunath, M. C. Lin, T. K. Wu, K. H. Wei and H. C. Lin, Monomeric and aggregation emissions of tetraphenylethene in a photo-switchable polymer controlled by cyclization of diarylethene and solvent conditions, J. Mater. Chem. C, 2017, 5, 9952–9962 RSC.
- H.-H. Liu and Y. Chen, The photochromism and fluorescence of diarylethenes with a imidazole bridge unit: a strategy for the design of turn-on fluorescent diarylethene system, J. Phys. Chem. A, 2009, 113, 5550–5553 CrossRef CAS.
- S.-C. Pang, H. Hyun, S. Lee, D. Jang, M. J. Lee, S. H. Kang and K.-H. Ahn, Photoswitchable fluorescent diarylethene in a turn-on mode for live cell imaging, Chem. Commun., 2012, 48, 3745–3747 RSC.
- T. Sumi, T. Kaburagi, M. Morimoto, K. Une, H. Sotome, S. Ito, H. Miyasaka and M. Irie, Fluorescent photochromic diarylethene that turns on with visible light, Org. Lett., 2015, 17, 4802–4805 CrossRef CAS.
- Z. Xu, Q. T. Liu, X. Wang, Q. Liu, D. Hean, K. C. Chou and M. O. Wolf, Quinoline-containing diarylethenes: bridging between turn-on fluorescence, RGB switching and room temperature phosphorescence, Chem. Sci., 2020, 11, 2729–2734 RSC.
- Y. Wang, N. Ma, Z. Wang and X. Zhang, Photocontrolled reversible supramolecular assemblies of an azobenzene-containing surfactant with alpha-cyclodextrin, Angew. Chem., Int. Ed., 2007, 119, 2881–2884 CrossRef.
- X. Liao, G. Chen, X. Liu, W. Chen, F. Chen and M. Jiang, Photoresponsive pseudopolyrotaxane hydrogels based on competition of host−guest interactions, Angew. Chem., Int. Ed., 2010, 49, 4409–4413 CrossRef CAS.
- S. Yagai, K. Ohta, M. Gushiken, K. Iwai, A. Asano, S. Seki, Y. Kikkawa, M. Morimoto, A. Kitamura and T. Karatsu, Photoreversible supramolecular polymerisation and hierarchical organization of hydrogen-bonded supramolecular co-polymers composed of diarylethenes and oligothiophenes, Chem. – Eur. J., 2012, 18, 2244–2253 CrossRef CAS.
- G. Liu, Y. M. Zhang, C. Wang and Y. Liu, Dual visible light-triggered photoswitch of a diarylethene supramolecular assembly with cucurbit[8]uril, Chem. – Eur. J., 2017, 23, 14425–14429 CrossRef CAS.
- R. Liu, Y. Yang, Q. Cui, W. Xu, R. Peng and L. Li, Diarylethene-based conjugated polymer networks for ultrafast photochromic films, Chem. – Eur. J., 2018, 24, 17756–17766 CrossRef CAS.
- Y. W. Zhong, N. Vila, J. C. Henderson, S. F. Torres and H. D. Abruna, Dinuclear transition-metal terpyridine complexes with a dithienylcyclo-pentene bridge directed toward molecular electronic applications, Inorg. Chem., 2007, 46, 10470–10472 CrossRef CAS.
- F. Wehmeier and J. Mattay, A perfluorocyclopentene based diarylethene bearing two terpyridine moieties–synthesis, photochemical properties and influence of transition metal ions, Beilstein, J. Org. Chem., 2010, 6, 53–60 Search PubMed.
- S. Jing, C. Zheng, S. Pu, C. Fan and G. Liu, A highly selective ratiometric fluorescent chemosensor for Hg2+ based on a new diarylethene with a stilbene-linked terpyridine unit, Dyes Pigm., 2014, 107, 38–44 CrossRef CAS.
- S. Pu, H. Jia, C. Fan, G. Liu, Y. Fu and S. Jing, Highly selective fluorescent chemosensors for the detection of Hg2+ based on photochromic diarylethenes with a terminal terpyridine unit, Tetrahedron, 2015, 71, 3463–3471 CrossRef CAS.
- L. Ma, S. Wang, C. P. Li, D. Cao, T. Li and X. Ma, Photo-controlled fluorescence on/off switching of a pseudo[3]rotaxane between an AIE-active pillar[5]arene host and a photochromic bithienylethene guest, Chem. Commun., 2018, 54, 2405–2408 RSC.
- H. B. Cheng, H. Y. Zhang and Y. Liu, Dual-stimulus luminescent lanthanide molecular switch based on an unsymmetrical diarylperfluorocyclopentene, J. Am. Chem. Soc., 2013, 135, 10190–10193 CrossRef CAS.
- T. M. Swager, The molecular wire approach to sensory signal amplification, Acc. Chem. Res., 1998, 31, 201–207 CrossRef CAS.
- J. Zhang, J. Zhu, C. Lu, Z. Gu, T. He, A. Yang, H. Qiu, M. Zhang and S. Yin, A hyperbranched fluorescent supramolecular polymer with aggregation induced emission (AIE) properties, Polym. Chem., 2016, 7, 4317–4321 RSC.
- L. Xu, D. Chen, Q. Zhang, T. He, C. Lu, X. Shen, D. Tang, H. Qiu, M. Zhang and S. Yin, A fluorescent cross-linked supramolecular network formed by orthogonal metal-coordination and host–guest interactions for multiple ratiometric sensing, Polym. Chem., 2018, 9, 399–403 RSC.
- R. Dobrawa, M. Lysetska, P. Ballester, M. Grüne and F. Wurthner, Fluorescent supramolecular polymers:
metal directed self-assembly of perylene bisimide building blocks, Macromolecules, 2005, 38, 1315–1325 CrossRef CAS.
- Y. Ding, P. Wang, Y.-K. Tian, Y.-J. Tian and F. Wang, Formation of stimuli-responsive supramolecular polymeric assemblies via orthogonal metal–ligand and host–guest interactions, Chem. Commun., 2013, 49, 5951–5953 RSC.
- X. Zhou, F. Su, H. Lu, P. Senechal-Willis, Y. Tian, R. H. Johnson and D. R. Meldrum, An FRET-based ratiometric chemosensor for in vitro cellular fluorescence analyses of pH, Biomaterials, 2012, 33, 171–180 CrossRef CAS.
- P. Q. Nhien, T. T. K. Cuc, T. M. Khang, C. H. Wu, B. T. B. Hue, J. I. Wu, B. W. Mansel, H. L. Chen and H. C. Lin, Highly efficient Förster resonance energy transfer modulations of dual-AIEgens between a tetraphenylethylene donor and a merocyanine acceptor in photo-switchable [2]rotaxanes and reversible photo-patterning applications, ACS Appl. Mater. Interfaces, 2020, 12, 47921–47938 CrossRef CAS.
- J. Zhan, Q. Li, Q. Hu, Q. Wu, C. Li, H. Qiu, M. Zhang and S. Yin, A stimuli-responsive orthogonal supramolecular polymer network formed by metal–ligand and host–guest interactions, Chem. Commun., 2014, 50, 722–724 RSC.
- B. Gruber, E. Kataev, J. Aschenbrenner, S. Stadlbauer and B. Konig, Vesicles and micelles from amphiphilic zinc(II)–cyclen complexes as highly potent promoters of hydrolytic DNA cleavage, J. Am. Chem. Soc., 2011, 133, 20704–20707 CrossRef CAS.
- T. F. A. D. Greef and E. W. Meijer, Materials science: supramolecular polymers, Nature, 2008, 453, 171–173 CrossRef.
- H. F. Clausen, Y. S. Chen, D. Jayatilaka, J. Overgaard, G. A. Koutsantonis, M. A. Spackmanand and B. B. Iversen, Intermolecular interactions and electrostatic properties of the β-hydroquinone apohost: implications for supramolecular chemistry, J. Phys. Chem. A, 2011, 115, 12962–12972 CrossRef CAS.
Footnote |
† Electronic supplementary information (ESI) available: UV-Vis and PL spectra, 1H- and 13C-NMR data, mass data (HRMS-ESI) and elemental analysis (EA) data. See DOI: 10.1039/d0qm00605j |
|
This journal is © the Partner Organisations 2021 |