DOI:
10.1039/D1NJ04548B
(Paper)
New J. Chem., 2022,
46, 322-327
Transition-metal-free synthesis of trifluoromethylated benzoxazines via a visible-light-promoted tandem difunctionalization of o-vinylanilides with trifluoromethylsulfinate†
Received
22nd September 2021
, Accepted 18th November 2021
First published on 19th November 2021
Abstract
9,10-Phenanthrenedione (PQ) visible-light photocatalysis for the synthesis of a series of trifluoromethylated benzoxazines in good to excellent yields has been developed by using N-(2-vinylphenyl)amides and trifluoromethylsulfinate as precursors. A plausible mechanism for the reaction is reported. It involves the oxidative difunctionalization of N-(2-vinylphenyl)amides through cascade C–O and C–C bond formation. This novel and operationally simple methodology features tolerance to diverse functional groups, is transition-metal-free and cost-effective, and can be applied in an open environment.
Introduction
Benzoxazines and their derivatives are an important class of heterocycles frequently found in many natural products1 and biologically active compounds.2 Likewise, as the key structural motif enhances physical, chemical and biological properties such as solubility, lipophilicity, metabolic stability, binding selectivity, etc., the trifluoromethyl (–CF3) functionality has widely been installed in a variety of drugs (celecoxib)3 and organic functional materials (silica aerogels4 and carbon fibers).5 Appealingly, the trifluoromethylated benzoxazine efavirenz shows anti-HIV activity.6 As a result, development of a practical method for the synthesis of trifluoromethylated benzoxazines has drawn significant attention from chemists, pharmacists and biologists. In 2015, Deng and co-workers7 reported a visible-light photocatalysis method for the preparation of CF3-containing benzoxazines from o-vinylanilides, Umemoto's reagent, [Ru(bpy)3]2+ and a base (Scheme 1a).7 In the same year, Jana and co-workers8 disclosed a copper-catalyzed approach for the synthesis of trifluoromethylated benzoxazines from o-vinylanilides and Umemoto's reagent (Scheme 1b) at 80 °C.8 Despite being effective and versatile, both of these methods (Scheme 1) are restricted to transition-metal catalysts and Umemoto's reagent (costly, 1 g@120 USD) as the CF3 source. As residual transition-metal contamination could adversely affect the biological and functional properties of the final products,9 and most transition-metal catalysts are expensive and require harsh reaction conditions for their activation, the development of a transition metal-free and inexpensive strategy to access trifluoromethylated benzoxazines is in great demand.
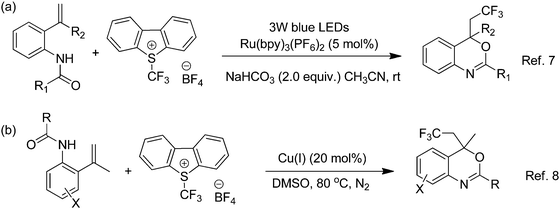 |
| Scheme 1 Two different protocols for the synthesis of trifluoromethylated benzoxazines reported in the literature.7,8 | |
Quinones are low-cost and low-molecular-weight organic compounds compared to other organo-photoredox catalysts.10 Many of them have absorption bands in the visible range and show three readily available oxidation states by single electron transfer. Furthermore, many quinones like 2,3-dichloro-5,6-dicyano-1,4-benzoquinone (DDQ),11 9,10-phenanthrenedione (PQ)12 and anthraquinone (AQ)13 have the ability to participate in photoinduced electron transfer processes and can be easily recovered from the reduced compound (dihydroquinone) by treating with nitrates, metal oxides, or molecular oxygen.11–13 To the best of our knowledge, however, quinone has not yet been used as a catalyst in the synthesis of trifluoromethylated benzoxazines under visible-light photocatalysis.
In continuation of our studies on visible-light-induced photoredox catalysis,14 we demonstrate herein a novel, cost-effective and transition-metal-free protocol for the preparation of trifluoromethylated benzoxazines from o-vinylanilides and trifluoromethylsulfinate by employing PQ (8 mol%) as the photocatalyst, CH3CN as the solvent and a white LED as the irradiation source in air atmosphere under ambient conditions (cf.Scheme 2). Furthermore, a plausible mechanism for the reaction is reported (vide infra).
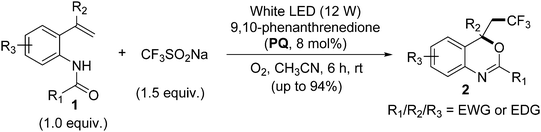 |
| Scheme 2 The synthesis of trifluoromethylated benzoxazines from N-(2-vinylphenyl)amides and trifluoromethylsulfinate employing PQ, a white LED and CH3CN in air atmosphere under ambient conditions reported in this work. EWD: electron withdrawing group. EDG: electron donating group. | |
Results and discussion
Our initial studies to optimize reaction conditions were conducted by employing N-(2-(prop-1-en-2-yl)phenyl)benzamide (1a, 90 mg, 0.3 mmol, 1.0 equiv.) as the model substrate, trifluoromethyl sulfinate (47 mg, 0.3 mmol, 1.0 equiv.) as the –CF3 source, acetonitrile (3 mL) as the solvent, oxygen from open air as the terminal oxidant and a white LED (12 W, at a distance of 2 cm) as the irradiation source under ambient conditions. Under these conditions, a series of quinone photocatalysts (5 mol%) were screened and the results are summarized in Table 1 (entries 1, 2, 3, 4, 5 and 6). Among the photocatalysts screened, 2,3-dichloro-5,6-dicyano-1,4-benzoquinone (DDQ, entry 1), 9,10-phenanthrenedione (PQ, entry 3) and anthraquinone (AQ, entry 5) afforded the desired 4-(2,2,2-trifluoroethyl)-4-methyl-2-phenyl-4H-benzo[d][1,3]oxazine (2a) in 27–48% yields after 20 h. The product (2a) was characterized by NMR and mass analysis (cf. ESI†). In view of the good performance (Table 1, entry 3), ready availability and high stability, we decided to continue the optimization of reaction conditions using PQ as the photocatalyst. Next, the impact of the amount of PQ on the product yield was explored. With an increase in the catalyst amount from 5.0 mol% to 6.0–10.0 mol%, there was a clear decrease in the reaction time with 58–64% yield of (2a) (Table 1, entries 7, 8, 9 and 10). In any case, no product was obtained without a photocatalyst (Table 1, entry 11). Afterwards, the influence of solvents on the formation of (2a) was explored (Table 1, entries 12, 13 14, 15 and 16). The examination revealed that still CH3CN was the best fit (Table 1, entry 9) among all other solvents tested including toluene, methanol, C2H4Cl2, ethyl acetate and THF (Table 1, entries 12, 13, 14, 15 and 16). Likewise, the white LED as the irradiation source (12 W) afforded (2a) in good yield (Table 1, entry 9) compared with the reactions performed using either green LEDs or blue LEDs (Table 1, entries 17 and 18). To improve the product yield, we then investigated the stoichiometry of trifluoromethylsulfinate to substrate (1a). An excellent yield (93%) of (2a) was noticed when increasing the amount of trifluoromethylsulfinate to 1.5 equiv. (Table 1, entries 19 and 20); however, further increasing the amount of trifluoromethylsulfinate did not improve the reaction efficiency drastically (Table 1, entries 21 and 22). From the control experiments, it was found that this reaction did not occur in the absence of any of the reaction parameters (Table 1). Thus, the optimized conditions for the synthesis of trifluoromethylated benzoxazines from N-(2-vinylphenyl)amides (1.0 equiv.) were trifluoromethylsulfinate (1.5 equiv.), 8.0 mol% of 9,10-phenanthrenedione, a white LED (12 W), and CH3CN in an open air atmosphere under ambient conditions with 6 h stirring (Table 1, entry 20).
Table 1 Selected results of screening the optimal conditions for the photocatalytic synthesis of trifluoromethylated benzoxazines (2) from N-(2-vinylphenyl)amides (1)a
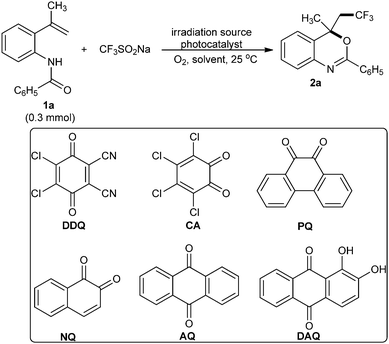
|
Entry |
Photocatalystb (mol%) |
CF3SO2Na (equiv.) |
Solventc |
Time (h) |
Yield of 2ad (%) |
Reaction conditions: unless stated otherwise all reactions were performed in a vial using N-(2-(1-phenylvinyl)phenyl)benzamide (1a, 0.3 mmol), photocatalyst and solvent under white LED (12 W) irradiation and exposure to air atmosphere in ambient conditions.
Commercially available high purity catalysts were purchased and utilized as such.
Solvents were purified before use.
Isolated yield of (2a).
A green LED used instead of a white LED.
A blue LED used instead of a white LED.
Reaction performed in the dark. T(p-CH3)PPT: 2,4,6-tris(para-methylphenyl)pyrilium tetrafluoroborate. EY: eosin Y. MB: methylene blue. Mes-Acr+: 9-mesityl-10-methylacridinium perchlorate. NR: no reaction. ND: not detected.
|
1 |
DDQ (5) |
1.0 |
CH3CN |
20 |
27 |
2 |
CA (5) |
1.0 |
CH3CN |
20 |
NR |
3 |
PQ (5) |
1.0 |
CH3CN |
20 |
48 |
4 |
NQ (5) |
1.0 |
CH3CN |
20 |
NR |
5 |
AQ (5) |
1.0 |
CH3CN |
20 |
33 |
6 |
DAQ (5) |
1.0 |
CH3CN |
20 |
NR |
7 |
PQ (6) |
1.0 |
CH3CN |
20 |
50 |
8 |
PQ (7) |
1.0 |
CH3CN |
15 |
54 |
9 |
PQ (8) |
1.0 |
CH3CN |
11 |
63 |
10 |
PQ (10) |
1.0 |
CH3CN |
11 |
64 |
11 |
PQ (0) |
1.0 |
CH3CN |
24 |
NR |
12 |
PQ (8) |
1.0 |
Toluene |
11 |
15 |
13 |
PQ (8) |
1.0 |
CH3OH |
11 |
NR |
14 |
PQ (8) |
1.0 |
C2H4Cl2 |
11 |
39 |
15 |
PQ (8) |
1.0 |
Ethyl acetate |
11 |
NR |
16 |
PQ (8) |
1.0 |
THF |
11 |
21 |
17 |
PQ (8) |
1.0 |
CH3CN |
11 |
37e |
18 |
PQ (8) |
1.0 |
CH3CN |
11 |
40f |
19 |
PQ (8) |
1.2 |
CH3CN |
9 |
72 |
20
|
PQ
(8)
|
1.5
|
CH
3
CN
|
6
|
93
|
21 |
PQ (8) |
1.8 |
CH3CN |
5 |
95 |
22 |
PQ (8) |
2.0 |
CH3CN |
4 |
94 |
23 |
PQ (8) |
1.5 |
CH3CN |
6 |
NRg |
24 |
T(p-CH3)PPT (8) |
1.5 |
CH3CN |
24 |
ND |
25 |
T(p-CH3)PPT (20) |
1.5 |
CH3CN |
24 |
ND |
26 |
EY (8) |
1.5 |
CH3CN |
24 |
48 |
27 |
EY (20) |
1.5 |
CH3CN |
24 |
75 |
28 |
MB (8) |
1.5 |
CH3CN |
24 |
18 |
29 |
MB (20) |
1.5 |
CH3CN |
24 |
39 |
30 |
Mes-Acr+ (8) |
1.5 |
CH3CN |
24 |
ND |
31 |
Mes-Acr+ (20) |
1.5 |
CH3CN |
24 |
ND |
With the optimized conditions in hand (Table 1, entry 20), the substrate scope and functional group tolerance were studied and the obtained results are presented in Table 2. Generally, the reaction tolerated o-vinylanilides bearing both electron-donating and electron-withdrawing groups and gave the expected trifluoromethylated benzoxazines (2) in good to excellent yields. For example, the amide ring of (1) with electron donating substituents such as –CH3, t-butyl and –OCH3 as well as electron-withdrawing groups such as –NO2, –F and –Cl gave the expected trifluoromethylated benzoxazines (2b–2h) in 76–89% yields (Table 2). Moreover, substrates with various N-substituents such as thiophenyl, furanyl, –CH3 and t-butyl were tolerated and trifluoromethylated benzoxazines (2i–2j) and (2l–2m) were afforded in good yields (Table 2). Nevertheless, no desired product (2k) could be obtained when benzyl-substituted N-(2-vinylphenyl)amide was treated with trifluoromethylsulfinate (cf.Table 2). Next, we studied the scope of substituted alkenes (2n–2t) in the reaction system. Various substituents including ethyl, phenyl, tolyl and 4-fluorophenyl at the C-2 position (α-position) of styrene gave the products in excellent yields. Nevertheless, no desired product (2n or 2s) could be obtained when either N-(2-(1-(4-methoxyphenyl)vinyl)phenyl)benzamide or a mono-substituted alkene such as N-(2-vinylphenyl)benzamide was treated with trifluoromethylsulfinate (cf.Table 2). Subsequently, the substrate with the –CH3 substituent in the aniline ring underwent trifluoromethylation smoothly and yielded 2u in 74% yield.
Table 2 Substrate scope for the synthesis of trifluoromethylated benzoxazines from N-(2-vinylphenyl)amides and trifluoromethylsulfinatea
Unless stated otherwise all reactions were performed in a vial using N-(2-vinylphenyl)amides (1b–1u, 1.0 mmol), trifluoromethylsulfinate (1.5 mmol), PQ (8 mol%) and CH3CN (8–10 mL) under white LED (12 W) irradiation and exposure to air atmosphere under ambient conditions for 6 h.
|
|
This reaction could also be performed on a gram scale. As shown in Scheme 3, treatment of 1.26 g (5 mmol) of 4-methyl-N-(2-(prop-1-en-2-yl)phenyl)benzamide (1b) with 1.17 g of trifluoromethylsulfinate under the optimized reaction conditions (Table 1, entry 20) afforded the desired 4-(2,2,2-trifluoroethyl)-4-methyl-2-p-tolyl-4H-benzo[d][1,3]oxazine (2b) in 87% (1.39 g) isolated yield. This clearly demonstrates the preparative practicality of this protocol.
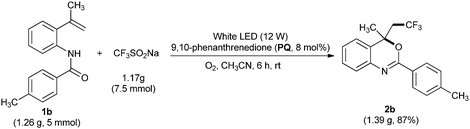 |
| Scheme 3 Gram-scale synthesis. | |
To understand the mechanism of this transformation, some control experiments were carried out as described in Scheme 4. The addition of 2 equiv. of 2,2,6,6-tetramethylpiperidine-1-oxyl (TEMPO, a typical radical scavenger) completely inhibited the reactions (Scheme 4), which suggested that the trifluoromethylation reaction proceeds via a radical process. The possible radical species involved in the reactions were further tested by electron paramagnetic resonance (EPR) spectroscopy. When PQ and CF3SO2Na in DCE were irradiated with a white LED in the presence of 2-methyl-2-nitrosopropane (MNP, a typical CF3-radical scavenger),15 a signal with six peaks corresponding to the radical adduct, i.e., CF3-MNP• (trifluoromethyl·tert-butyl nitroxide radical with g = 2.0069, aN = aF = 12.26 G),15 was clearly recorded by ESR. Nonetheless, in dark conditions, the same reaction mixture of MNP, PQ, and CF3SO2Na was shown to be EPR silent. These results confirmed the formation of the CF3-radical under the experimental conditions.
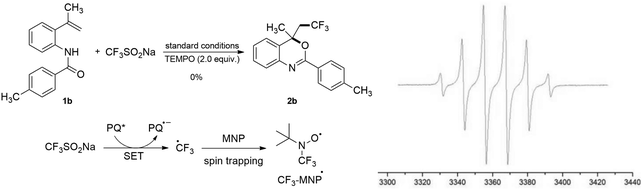 |
| Scheme 4 Control experiments with TEMPO and MNP are shown on the left, while the ESR spectrum of the trifluoromethyl tert-butylnitroxide radical is presented on the right. | |
On the basis of the above experimental evidence and our experience with visible-light photocatalysis,14 a rational reaction pathway for the difunctionalization of o-vinylanilides over 9,10-phenanthrenedione (PQ) is proposed in Scheme 5. PQ was excited when exposed to visible light, resulting in PQ* [Ered(PQ*/PQ˙−) = +1.6 V vs. SCE]. A single electron transfer from trifluoromethylsulfinate [Eox(CF3SO2−/CF3SO2˙) = +1.05 V vs. SCE] to PQ* afforded PQ˙− and the trifluoromethyl radical (CF3˙). Afterwards, the electron-deficient CF3˙ promptly attacks the electron-rich C
C bond of o-vinylanilides (1) to generate intermediate II. Following that, II is subjected to intramolecular radical cyclization, yielding the new radical intermediate III. Subsequently, a reaction between III and PQ˙− afforded the desired trifluoromethylated benzoxazines 2. During the process, PQ was reduced to PQH− and reoxidized by molecular oxygen to PQ.16
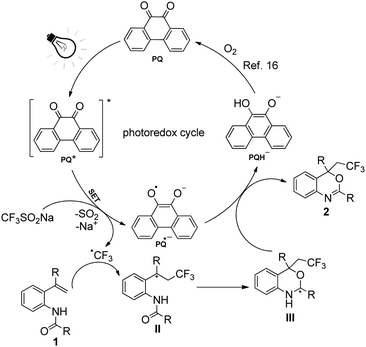 |
| Scheme 5 A plausible mechanism for the formation of trifluoromethylated benzoxazines from N-(2-vinylphenyl)amides and trifluoromethylsulfinate. | |
As mentioned at the beginning, in recent times, synthesis of trifluoromethylated benzoxazines (2) has drawn significant attention due to their anti-HIV activity (efavirenz) and lipophilicity. So far, only two synthesis methods have been described for their synthesis, both of which are limited to transition-metal catalysts and Umemoto's reagent (known to give diarylsulfide as a by-product and be expensive, i.e., 1 g@120 USD) as the –CF3 source. In contrast, the method reported here is based on the use of an organic dye, i.e., PQ, as the catalyst under visible light irradiation and trifluoromethylsulfinate (which yields only gaseous SO2 as the by-product and is cheap, i.e., 1 g@20 USD) as the CF3-source. Thus, we are certain that the present protocol may find a bright future, particularly in pharmaceutical industries where there is a necessity for final products to be totally free of traces of transition metals and inexpensive.
Conclusions
A novel, cost-efficient and metal-free protocol has been developed for the synthesis of trifluoromethylated benzoxazines from N-(2-vinylphenyl)amides and trifluoromethylsulfinate by employing PQ as the catalyst under visible-light irradiation. The facile formation of new C–C and C–O bonds takes place via a one-pot procedure. Moreover, the desired products in pure form are obtained in good yields by simple filtration using silica gel column chromatography. Further application of this strategy to other substrates is ongoing in our laboratory.
Author contributions
All of the authors contributed to the writing of the manuscript. The final version of the manuscript has received the approval of all authors. Deachen Chuskit and Priya are responsible for experimental design, execution, methodology, data analysis, interpretation, writing – original draft and review. Manjeet's responsibilities include conceptualization, inquiry, validation, and formal analysis. Palani Natarajan is responsible for research and supervision.
Conflicts of interest
The authors declare that they have no conflicts of interest.
Acknowledgements
The authors gratefully acknowledge the financial support from the Council of Industrial Scientific Research (CSIR), New Delhi, India, through an extramural research grant with project number 02(0380)/19/EMR-II. D. C. and Priya thank the CSIR, New Delhi for their senior research fellowships. Also, the authors are indebted to anonymous reviewers for their constructive comments and suggestions.
References
-
(a) J. Tanabe, M. Sue, A. Ishihara and H. Iwamura, Biosci., Biotechnol., Biochem., 1999, 63, 1614–1617 CrossRef CAS PubMed;
(b) A. A. A. Qahtani, T. M. A. Turki, A. A. Mousa, S. A. A. Mazroa, M. Khan and H. Z. Alkhathlan, Orient. J. Chem., 2012, 28, 287–295 CrossRef;
(c) B. V. S. Reddy, R. A. Babu, M. R. Reddy, B. J. M. Reddy and B. Sridhar, RSC Adv., 2014, 4, 44629–44633 RSC;
(d) J. Sheng, X. Su, C. Cao and C. Chen, Org. Chem. Front., 2016, 3, 501–504 RSC;
(e) N. Kumar, N. Yadav, N. Amarnath, V. Sharma, S. Shukla, A. Srivastava, P. Prasad, A. Kumar, S. Garg, S. Singh, S. Sehrawat and B. Lochab, Mol. Cell. Biochem., 2019, 454, 123–138 CrossRef CAS PubMed;
(f) K. Zhang, M. Han, Y. Liu and P. Froimowicz, ACS Sustainable Chem. Eng., 2019, 7, 9399–9407 CrossRef CAS;
(g) Y. Lyu and H. Ishida, Prog. Polym. Sci., 2019, 99, 101168 CrossRef CAS.
-
(a) K. Waisser, J. Gregor, L. Kubicová, V. Klimesová, J. Kunes, M. Machácek and J. Kaustová, Eur. J. Med. Chem., 2000, 35, 733–774 CrossRef CAS PubMed;
(b) A. Fensome, R. Bender, R. Chopra, J. Cohen, M. A. Collins, V. Hudak, K. Malakian, S. Lockhead, A. Olland, K. Svenson, E. A. Terefenko, R. J. Unwalla, J. M. Wilhelm, S. Wolfrom, Y. Zhu, Z. Zhang, P. Zhang, R. C. Winneker and J. Wrobel, J. Med. Chem., 2005, 48, 5092–5095 CrossRef CAS PubMed;
(c) N. Dias, J.-F. Goossens, B. Baldeyrou, A. Lansiaux, P. Colson, A. D. Salvo, J. Bernal, A. Turnbull, D. J. Mincher and C. Bailly, Bioconjugate Chem., 2005, 16, 949–958 CrossRef CAS PubMed;
(d) J. Ilas, Z. Jakopin, T. Borstnar, M. Stegnar and D. Kikelj, J. Med. Chem., 2008, 51, 5617–5629 CrossRef CAS PubMed;
(e) Z. Tang, W. Chen, Z. Zhu and H. Liu, J. Heterocycl. Chem., 2011, 48, 255–260 CrossRef CAS;
(f) W.-C. Lee, H.-C. Shen, W.-P. Hu, W.-S. Lo, C. Murali, J. K. Vandavasi and J.-J. Wang, Adv. Synth. Catal., 2012, 354, 2218–2228 CrossRef CAS.
-
(a) T. D. Penning, J. J. Talley, S. R. Bertenshaw, J. S. Carter, P. W. Collins, S. Docter, M. J. Graneto, L. F. Lee, J. W. Malecha, J. M. Miyashiro, R. S. Rogers, D. J. Rogier, S. S. Yu, G. D. Anderson, E. G. Burton, J. N. Cogburn, S. A. Gregory, C. M. Koboldt, W. E. Perkins, K. Seibert, A. W. Veenhuizen, Y. Y. Zhang and P. C. Isakson, J. Med. Chem., 1997, 40, 1347–1365 CrossRef CAS PubMed;
(b) A. Chiba, M. Mizuno, C. Tomi, R. Tajima, I. Alloza, A. Penta, T. Yamamura, K. Vandenbroeck and S. Miyake, Arthritis Res. Ther., 2012, 14, 1–9 CrossRef PubMed;
(c) Ş. G. Küçükgüzel, İ. Coşkun, S. Aydın, G. Aktay, Ş. Gürsoy, Ö. Çevik, Ö. B. Özakpınar, D. Özsavcı, A. Şener, N. K.-Basu, A. Basu and T. T. Talele, Molecules, 2013, 18, 3595–3614 CrossRef PubMed;
(d) A. D. Penta, A. Chiba, I. Alloza, A. Wyssenbach, T. Yamamura, P. Villoslada, S. Miyake and K. Vandenbroeck, PLoS One, 2013, 8, 83119 CrossRef PubMed;
(e) W. Zhu, J. Wang, S. Wang, Z. Gu, J. L. Aceñ, K. Izawa, H. Liu and V. A. Soloshonok, J. Fluorine Chem., 2014, 167, 37–54 CrossRef CAS;
(f) B. Chaudhary, N. Kulkarni, N. Saiyed, M. Chaurasia, S. Desai, S. Potkulea and S. Sharma, Adv. Synth. Catal., 2020, 362, 4794–4819 CrossRef CAS.
-
(a) O. Garcí, R. Sastre, D. Agua, A. Costela, I. G. Moreno and A. Roig, Chem. Phys. Lett., 2006, 427, 375–378 CrossRef;
(b) N. M. Cremaes, A. M. L. Periago, J. Saurina, A. Roiga and C. Domingo, J. Supercrit. Fluids, 2013, 73, 34–42 CrossRef;
(c) S. A. Lermontov, A. N. Malkova, N. A. Sipyagina, E. A. Straumal, A. E. Baranchikov, K. E. Yorov and V. K. Ivanov, J. Fluorine Chem., 2017, 193, 1–7 CrossRef CAS;
(d) T. Wu, J. Dong, F. Gan, Y. Fang, X. Zhao and Q. Zhang, Appl. Surf. Sci., 2018, 440, 595–605 CrossRef CAS.
-
(a) J. M. V. Nabais, A. Mouquinho, C. Galacho, P. J. M. Carrott and M. M. L. R. Carrott, Fuel Process. Technol., 2008, 89, 549–555 CrossRef CAS;
(b) J. Chang, W. Liu, M. Zhang, L. Cao, Q. Ge, H. Niu, G. Sui and D. Wu, RSC Adv., 2015, 5, 71425–71432 RSC;
(c) L. Servinis, L. C. Henderson, L. M. Andrighetto, M. G. Huson, T. R. Gengenbach and B. L. Fox, J. Mater. Chem. A, 2015, 3, 3360–3371 RSC;
(d) Ĺ. Szabó, S. Imanishi, N. Kawashima, R. Hoshino, K. Takada, D. Hirose, T. Tsukegi, K. Ninomiya and K. Takahashi, RSC Adv., 2018, 8, 22729–22736 RSC;
(e) H. Niu, M. Zhang, A. Li, Z. Wang, X. Wang, D. Wu and T. Su, High Perform. Polym., 2020, 32, 39–46 CrossRef CAS.
-
(a) D. Sriram, D. Banerjee and P. Yogeeswari, J. Enzyme Inhib. Med. Chem., 2009, 24, 1–5 CrossRef CAS PubMed;
(b) S. Mahapatra, T. S. Thakur, S. Joseph, S. Varughese and G. R. Desiraju, Cryst. Growth Des., 2010, 10, 3191–3202 CrossRef CAS;
(c) S. Mishra, P. Tandon and A. P. Ayala, Spectrochim. Acta, Part A, 2012, 88, 116–123 CrossRef CAS PubMed;
(d) C. Fandaruff, M. A. S. Silva, D. C. G. Bedor, D. P. de Santana, H. V. A. Rocha, L. Rebuffi, C. L. A. Ricardo, P. Scardi and S. L. Cuffini, Eur. J. Pharm. Biopharm., 2015, 91, 52–58 CrossRef CAS PubMed;
(e) M. M. Bastos, C. C. P. Costa, T. C. Bezerra, F. C. Silva and N. Boechat, Eur. J. Med. Chem., 2016, 108, 455–465 CrossRef CAS PubMed;
(f) Y. Zheng, L. Zhang and E. Meggers, Org. Process Res. Dev., 2018, 22, 103–107 CrossRef CAS.
- Q.-H. Deng, J.-R. Chen, Q. Wei, Q.-Q. Zhao, L.-Q. Lu and W.-J. Xiao, Chem. Commun., 2015, 51, 3537–3540 RSC.
- S. Jana, A. Ashokan, S. Kumar, A. Verma and S. Kumar, Org. Biomol. Chem., 2015, 13, 8411–8415 RSC.
-
(a) S. Xu, C. Fang, Y. Wu, W. Wu, Q. Guo, J. Zeng, X. Wang, Y. Liu and S. Cao, Dyes Pigm., 2017, 142, 8–16 CrossRef CAS;
(b) P. Y. Wang, H. S. Fang, W. B. Shao, J. Zhou, Z. Chen, B. A. Song and S. Yang, Bioorg. Med. Chem. Lett., 2017, 27, 4294–4297 CrossRef CAS PubMed;
(c) N. Yedukondalu, G. Gupta, J. R. Nadkarni, V. K. Gupta, S. H. Syed and A. Ali, RSC Adv., 2016, 6, 83069–83077 RSC.
-
(a) J. I. Bardagi, I. Ghosh, M. Schmalzbauer, T. Ghosh and B. König, Eur. J. Org. Chem., 2018, 34–40 CrossRef CAS;
(b) A. E. Wendlandt and S. S. Stahl, Angew. Chem., Int. Ed., 2015, 54, 14638–14658 CrossRef CAS PubMed;
(c) S. Lerch, L.-N. Unkel, P. Wienefeld and M. Brasholz, Synlett, 2014, 2673–2680 CAS;
(d) S. Lerch, L.-N. Unkel and M. Brasholz, Angew. Chem., Int. Ed., 2014, 53, 6558–6562 CrossRef CAS PubMed.
-
(a) D. Walker and J. D. Hiebert, Chem. Rev., 1967, 67, 153–195 CrossRef CAS PubMed;
(b) L. Liu and P. E. Floreancig, Org. Lett., 2010, 12, 4686–4689 CrossRef CAS PubMed;
(c) Z. Shen, J. Dai, J. Xiong, X. He, W. Mo, B. Hu, N. Sun and X. Hua, Adv. Synth. Catal., 2011, 353, 3031–3038 CrossRef CAS;
(d) K. Ohkubo, A. Fujimoto and S. Fukuzumi, J. Am. Chem. Soc., 2013, 135, 5368–5371 CrossRef CAS PubMed;
(e) K. Ohkubo, K. Hirose and S. Fukuzumi, Chem. – Eur. J., 2015, 21, 2855–2861 CrossRef CAS PubMed;
(f) F. Rusch, J.-C. Schober and M. Brasholz, ChemCatChem, 2016, 8, 2881–2884 CrossRef CAS;
(g) S. Das, P. Natarajan and B. König, Chem. – Eur. J., 2017, 23, 18161–18165 CrossRef CAS PubMed;
(h) D. Chuskit, R. Chaudhary, P. Venugopalan, B. König and P. Natarajan, Org. Chem. Front., 2018, 5, 3553–3556 RSC;
(i) Y. Zhang, W. Chen, L. Wang and P. Li, Org. Chem. Front., 2018, 5, 3562–3566 RSC;
(j) P. Natarajan and B. König, Eur. J. Org. Chem., 2021, 2145–2161 CrossRef CAS.
-
(a) M. P. Shurygina, Y. A. Kurskii, S. A. Chesnokov and G. A. Abakumov, Tetrahedron, 2008, 64, 1459–1466 CrossRef CAS;
(b) Y. Zhang, P. Ji, W. Hu, Y. Wei, H. Huang and W. Wang, Chem. – Eur. J., 2019, 25, 8225–8228 CrossRef CAS PubMed.
-
(a) S. Elkazaz and P. B. Jones, J. Org. Chem., 2010, 75, 412–416 CrossRef CAS PubMed;
(b) L. Cui, N. Tada, H. Okubo, T. Miura and A. Itoh, Green Chem., 2011, 13, 2347–2350 RSC;
(c) N. Tada, K. Hattori, T. Nobuta, T. Miura and A. Itoh, Green Chem., 2011, 13, 1669–1671 RSC;
(d) W. Zhang, J. Gacs, I. W. C. E. Arends and F. Hollmann, ChemCatChem, 2017, 9, 3821–3826 CrossRef CAS PubMed;
(e) J. Duval, V. Pecher, M. Poujol and E. Lesellier, Ind. Crops Prod., 2016, 94, 812–833 CrossRef CAS;
(f) W. Zhang, J. Gacs, I. W. C. E. Arends and F. Hollmann, Asian J. Org. Chem., 2019, 8, 1411–1414 CrossRef;
(g) R. Narobe, S. J. S. Düsel, J. Iskra and B. König, Adv. Synth. Catal., 2019, 361, 3998–4004 CrossRef CAS;
(h) J. C. González, D. A. Vosburg, S. E. M. Rodriguez, M. A. Vázquez, L. G. Zepeda, C. V. Gómez and S. L. Rivera, ChemCatChem, 2020, 12, 3811–3827 CrossRef.
-
(a) P. Natarajan, N. Kumar and Priya, ChemistrySelect, 2020, 5, 4862–4865 CrossRef CAS;
(b) P. Natarajan, D. Chuskit and Priya, Green Chem., 2019, 21, 4406–4411 RSC;
(c) P. Natarajan, Manjeet, Muskan, N. K. Brar and J. J. Kaur, Org. Chem. Front., 2018, 5, 1527–1531 RSC;
(d) R. Chaudhary and P. Natarajan, ChemistrySelect, 2017, 2, 6458–6479 CrossRef CAS;
(e) P. Natarajan, Manjeet, N. Kumar, S. Devi and K. Mer, Tetrahedron Lett., 2017, 58, 658–662 CrossRef CAS;
(f) P. Natarajan, A. Bala, S. K. Mehta and K. K. Bhasin, Tetrahedron, 2016, 72, 2521–2526 CrossRef CAS.
- Z. Hang, Z. Li and Z.-Q. Liu, Org. Lett., 2014, 16, 3648–3651 CrossRef CAS PubMed.
- M. Yang, H. Ahmed, W. Wu, B. Jiang and Z. Jia, BioMed Res. Int., 2018, 9523968 Search PubMed.
Footnote |
† Electronic supplementary information (ESI) available: General aspects, procedure, experimental characterization data and NMR spectra of some products associated with this article. See DOI: 10.1039/d1nj04548b |
|
This journal is © The Royal Society of Chemistry and the Centre National de la Recherche Scientifique 2022 |