DOI:
10.1039/D0FO02688C
(Paper)
Food Funct., 2021,
12, 191-202
Effect of Auricularia auricula fermentation broth on the liver and stomach of mice with acute alcoholism†
Received
14th October 2020
, Accepted 19th November 2020
First published on 20th November 2020
Abstract
In this paper, the protective effect of Auricularia auricula (A. auricula) fermentation broth on the liver and stomach of mice with acute alcoholism was studied. The A. auricula fermentation broth was prepared by adding Bacillus subtilis, lactic acid bacteria, and Saccharomyces cerevisiae to A. auricula solution. The changes of physical and chemical indexes during the fermentation of A. auricula were monitored, and the results showed the content of polysaccharides and protein in the two kinds of fermentation broth after the fermentation was completed. Furthermore, the characteristic structures of active substances such as proteins, polysaccharides and phenolics were found in the A. auricula fermentation by structural analysis. Antioxidant activity test results showed that the A. auricula fermentation broth had a strong ability to scavenge 1,1-diphenyl-2-picrylhydrazyl (DPPH) and hydroxyl radicals. Cell experiments showed that the fermentation broth of A. auricula could significantly enhance the activity of NRK cells and protect NRK cells from H2O2 damage. Animal experiments showed that the A. auricula fermentation broth had protective effects on the liver and stomach of mice with acute alcoholism, and significantly reduced the levels of alanine aminotransferase (ALT), aspartate aminotransferase (AST), total cholesterol (TC) and triglycerides (TG) in serum. These results indicated that the A. auricula fermentation broth had protective effects on the liver and stomach of mice with acute alcoholism, and could be used as a potential functional food to prevent liver and stomach damage caused by acute alcoholism.
Introduction
Alcohol is a psychoactive drug with dependence-producing potential and has been widely used in beverages as a recreational substance worldwide for centuries.1 Many people drink hard liquor chronically or acutely due to their addiction to alcohol. It is reported by the World Health Organization that there are 237 million men and 46 million women affected by harmful drinking.2 Alcohol abuse and related diseases have become a global public health problem, and the incidence rate is also increasing in recent years.3 As we all know, excessive drinking of alcohol usually induces a variety of organ disorders,4,5 including neurological, liver, gastrointestinal and cardiovascular diseases, and even cancer. When the level of alcohol in a person's blood is high, alcohol metabolism produces excessive reactive oxygen species (ROS), which can induce oxidative stress and lead to acute alcoholic injury.6 Therefore, the exploration of natural substances from food materials with antioxidant activity to alcohol is needed for preventing and treating damage caused by the oxidative stress of alcohol.
Auricularia auricula (A. auricula), also named “heimuer” in Chinese, is an important edible fungus with high carbohydrate and protein contents and has been recognized as a healthy food.7 Recently, there are many studies showing that the polysaccharides, melanin and protein in A. auricula have significant protective effects on acute alcoholic injury. For example, Wang et al.8 reported that the polysaccharides from A. auricula have protective effects on acute alcoholic liver injury through different metabolic pathways. Hou et al.9 extracted a natural melanin from A. auricula and examined its hepatoprotective effect on acute alcohol induced liver injury in mice. It is reported that the bioactive ingredients in A. auricula are not only found in its fruiting body and mycelium, but also in its fermentation broth and residues.10,11
It is generally known that fermentation is a metabolic process caused by microorganisms and is characterized by the anaerobic breakdown of carbohydrates. Microbial fermentation is often accompanied by a series of biochemical changes, which not only change the nutrient composition and proportion in food,12 but also produce many bioactive substances.13 Wang et al.14 reported that the antioxidant activity of rice and wheat bran was greatly improved after fermentation with Lactobacillus plantarum 423. Juan et al.15 found that the total phenolic and flavonoid contents were enhanced after fermentation of black soybean by Bacillus subtilis (B. subtilis) BCRC 14715 and the black soybean extract showed improved antioxidant activity. Therefore, the use of microbial fermentation to increase the bioactive substances in A. auricula may be a new treatment strategy for the treatment and prevention of acute alcoholic injuries.
In this study, A. auricula was treated with B. subtilis, lactic acid bacteria and Saccharomyces cerevisiae (S. cerevisiae) to prepare a fermentation broth. The use of multi-species bacteria to ferment A. auricula aims to destroy the cellular wall and allow the biologically active substances to be sufficiently released. Therefore, the fermentation products of A. auricula might be more useful in preventing alcoholic injury. The research strategy in Scheme 1 shows that two kinds of fermentation broth (FB-1 and FB-3) are prepared by adding B. subtilis and three species of B. subtilis, lactic acid bacteria, S. cerevisiae to A. auricula, respectively, using the water extract of A. auricula as a control. The active substance in the two kinds of A. auricula fermentation broth was inferred by the characteristic absorption peak of the active substance group appearing in the ultraviolet-visible absorption spectra and Fourier transform spectra. What's more, the antioxidation effect on living cells against hydrogen peroxide was studied. Lastly, the protective effects of the A. auricula fermentation broth on the liver and stomach of mice with acute alcoholism were evaluated. The results demonstrated that the A. auricula fermentation broth was effective in protecting the liver and stomach of mice with acute alcoholism.
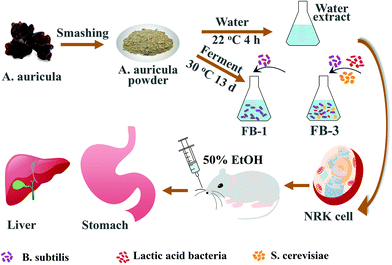 |
| Scheme 1 Preparation scheme of the A. auricula fermentation broth for cellular antioxidation and preventing alcoholic injury of the liver and stomach of mice. “WE” is the water extract of A. auricula; “FB-1” is the A. auricula fermentation broth with B. subtilis only; “FB-3” is the fermentation broth with B. subtilis, lactic acid bacteria and S. cerevisiae. | |
Experimental
Materials
The fruiting bodies of Auricularia auricula were purchased from Weiduobao food Co., Ltd in Suifenhe (Heilongjiang, China), which was identified (the certificate number was 150OP1800023) by Beijing Huace food certification service Co., Ltd, Beijing, China. The lactic acid bacteria powder was bought from Chuan Xiu Science and Technology Co., Ltd in Beijing, China. The combined B. subtilis and Enterococcus faecium granules with multivitamins were purchased from Han Mei Maceutical Co., Ltd in Beijing, China. S. cerevisiae was kindly provided by Quanran Technological Development Co., Ltd in Dalian, China. The NRK cells were a gift from Dr Li Yu's lab in Tsinghua University, China. The cell viability assay reagent 3-(4,5-dimethylthiazol-2-yl)-2,5-diphenyltetrazolium bromide (MTT) was procured from Aladdin Reagent Co., Ltd in Shanghai, China. Annexin VFITC/PI double staining apoptosis and cell cycle assay kits were purchased from Nanjing Jiancheng Bioengineering Institute in China. 1,1-Diphenyl-2-picrylhydrazyl (DPPH) was procured from Sigma-Aldrich LLC in Shanghai, China.
Isolation of B. subtilis
B. subtilis was cultured in beef extract peptone medium according to the previous method.16 After continuous gradient dilution, the medium containing B. subtilis was placed in a 60 °C incubator for 50 minutes, and then placed in a 37 °C incubator for 36 h. The colony morphology of the B. subtilis was examined with a microscope and relatively pure colonies of B. subtilis were identified and separated. The colonies of the obtained culture of B. subtilis were continuously sub-cultured twice, and were further used in the subsequent fermentation experiments.
Preparation of fermentation broth from A. auricula
The B. subtilis suspension was prepared by culturing the bacteria in beef extract peptone broth medium at 37 °C and 160 rpm for 1 day according to the method by Soufi et al.17 The lactic acid bacteria suspension was prepared by adding 1 g of lactic acid bacteria powder into deMan, Rogosa, and Sharpe (MRS) broth, and cultured in a constant temperature shaker at 37 °C and 160 rpm for 2 days. S. cerevisiae suspension was prepared by placing S. cerevisiae in yeast peptone dextrose (YPD) broth medium at 30 °C for 1 day.18
The A. auricula fermentation broths FB-1 (A. auricula fermentation broth with B. subtilis only) and FB-3 (fermentation broth with B. subtilis, lactic acid bacteria and S. cerevisiae) were prepared. After washing, the A. auricula was dried in an electric oven at 60 °C, and then pulverized into a powder with a disintegrator. The A. auricula solution was prepared in a sample to water ratio of 1
:
60 (wt/wt). After sterilization, 4 mL of B. subtilis suspension was added into 150 mL of A. auricula solution and the B. subtilis was cultured in a shaker for 13 days to prepare the A. auricula fermentation broth of FB-1. As for the preparation of FB-3, 4 mL of B. subtilis and 4 mL of lactic acid bacteria suspensions were added to A. auricula solution, and then cultured in a shaker at 30 °C. On the 7th day, 4 mL of S. cerevisiae suspension was added and the A. auricula solution was continued to culture until 13 days. After 13-day culture, the residue of A. auricula was removed by filtration at 4000 rpm for 10 min. The liquid supernatants of FB-1 and FB-2 were sterilized at 121 °C for 15 min, then freeze-dried and preserved for subsequent experiments.
The water extract (WE) of A. auricula was prepared by mixing 2.5 g of A. auricula powder into 150 mL of distilled water with a solid–liquid ratio of 1
:
60 (wt/wt), and stirring at 22 °C for 4 h with a magnetic stirrer.19 After extraction, the residue was removed by filtration at 4000 rpm for 10 min, and the liquid supernatant of WE was sterilized at 121 °C for 15 min, freeze-dried and stored for subsequent experiments as a control.
Fermentation process characterization
During the fermentation process, the physicochemical indexes of fermentation broth were recorded every day. The soluble solid content was determined with a RHB-10ATC hand-held refractometer (Loikaow, Shanghai, China). The pH was monitored with an FE28 pH meter (Mettler-Toledo, Shanghai, China). The total sugar and protein content was determined by the phenolsulphuric acid20 and Coomassie Brilliant Blue methods.21 The optical density was tested with a Lambda 35 UV-visible spectrophotometer (PerkinElmer, Waltham, MA, USA) at an absorbance of 600 nm.
Characterization
The FT-IR spectra of FB-1, FB-3 and WE of A. auricula were recorded with an infrared spectrometer (VECTOR 22, PerkinElmer, Norwalk, USA). Briefly, 1 mg of the FB-1, FB-3 or WE samples was mixed with 100 mg dry KBr, compressed into a tablet,22 and then analyzed with an FT-IR spectrometer in a scanning wavenumber from 4000 to 400 cm−1. The infrared spectra of FB-1, FB-3 and WE were analyzed and compared using the same method. As for the UV-visible spectra, 3 mL of WE, FB-1 and FB-3 with a concentration of 0.125 mg mL−1 were added into a quartz cuvette and scanned in the wavelength range of 200–700 nm, respectively.
Active substance analysis of fermentation broth
The total sugar content was measured by the phenolsulphuric acid method by mixing 1 mL of 0.5 mg mL−1 FB-1, FB-3 and WE solution with 1 mL of 80% phenol and 5 mL H2SO4, respectively.23 The sample tube was placed in a water bath at 30 °C for 20 min, and the absorbance was measured at 490 nm with a UV-vis spectrometer. The standard curve was drawn with the absorbance of 0.02, 0.04, 0.06, 0.08 and 0.1 mg mL−1 glucose solutions. The total sugar content in WE, FB-1 and FB-3 was calculated according to the standard curve of glucose.
The total protein content of the FB-1, FB-3 and WE samples was determined by the Coomassie Brilliant Blue G-250 method.24 A total of 1 mL of 10 mg mL−1 testing sample solution was added to 5 mL of 2 mg mL−1 Coomassie Brilliant Blue G-250 ethanol solution. After reaction for 2 min, the absorbance of the solution was determined at 595 nm. The standard curve was drawn with the absorbance of 3, 6, 12, 24 and 48 μg mL−1 bovine serum albumin (BSA) solution. The total protein content in the sample (WE, FB-1 and FB-3) was calculated according to the standard protein concentration curve.
The content of phenolics in the FB-1, FB-3 and WE of A. auricula was determined using the method of Folin–Ciocalteu colorimetry by Cilliers et al., with slight modifications.25 First, 1 mg of WE, FB-1 and FB-3 powder were mixed with 1 mL distilled water, respectively, followed by adding 5 mL 10% Folin–Ciocalteu reagent and reaction for 5 min. Second, 4 mL of 7.5% Na2CO3 was added to the mixture and placed at room temperature for 60 min. Finally, the absorbance of the solution was measured at 765 nm with a UV-visible spectrophotometer. The standard curve was drawn with the absorbance of 10, 20, 30, 40 and 50 μg mL−1 gallic acid standard solutions. The total phenolic content in WE, FB-1 and FB-3 was calculated by the following formula:
|  | (1) |
where
A is the absorbance of the tested sample (WE, FB-1 and FB-3),
V is the volume of the sample solution (1 mL),
K is the slope of the standard curve for gallic acid, and
m is the weight of the tested sample (0.001 g).
Antioxidant activity analysis
The test of DPPH radical scavenging ability of samples was slightly modified according to the method by Li et al.26 Briefly, 100 μL of freshly prepared DPPH ethanol solution (0.01 mmol L−1) was mixed with 100 μL of FB-1, FB-3 and WE of A. auricula at different concentrations (0, 0.4, 0.8, 1.2, 1.6, and 2.0 mg mL−1) in 96-well plates, respectively. The reaction solution was mixed and kept in the dark for 30 min. The optical density at 519 nm was measured to calculate the DPPH scavenging activity using ascorbic acid as a positive control. The DPPH scavenging abilities of FB-1, FB-3 and WE at concentrations of 0, 10, 20, 30, 40, and 50 μg mL−1 were calculated by the following formula: | Scavenging abilities (%) = [1 − (A1 − A2)/A0] × 100% | (2) |
where A0 represents the absorbance of DPPH ethanol solution, A1 represents the absorbance of FB-1, FB-3 or WE solution mixed with DPPH ethanol solution, and A2 is the absorbance of FB-1, FB-3 or WE solution.
The hydroxyl radical scavenging activities of FB-1, FB-3 and WE were measured according to a published method,27 with some modifications. A total of 1 mL of WE, FB-1 or FB-3 solution (0, 1, 2, 3, 4, and 5 mg mL−1) was added to 1 mL of 9 mmol L−1 FeSO4, 1 mL of 9 mmol L−1 salicylic acid and 1 mL of 2.4 mmol L−1 H2O2 and the mixture was incubated at 37 °C for 30 min. The mixture absorbance at 510 nm was measured using ascorbic acid (0.1, 0.2, 0.3, 0.4, and 0.5 mg mL−1) as a control. The hydroxyl radical scavenging activity was calculated using eqn (2).
Cell experiment
The cell activity of FB-1 and FB-3 A. auricula was determined with MTT assay using the water extract of A. auricula powder as a control.28 The NRK cells were seeded into 96-well plates and incubated at a cell density of 5 × 104 cells per well for 24 h. Then, the NRK cells were incubated with different concentrations (0, 0.31, 0.63, 1.25, 2.5, 5.0 and 10.0 mg mL−1) of FB-1, FB-3 and water extract of A. auricula for 24 h, respectively. After 20 μL of MTT (5 mg mL−1) solution was added to each well and incubated for 3 h, the supernatant was discarded and dissolved in dimethyl sulfoxide (DMSO). Finally, the absorbance at 490 nm was measured with an Infinite 200 multimode microplate reader to evaluate its effect on cell viability.
For the experiment on the protective effect on the damage of hydrogen peroxide (H2O2), the method was modified from previous reports.29 The NRK cells were seeded into 12-well plates at a cell density of 2 × 105 cells per well for 24 h and incubated with 0.5 mg mL−1 FB-1 and FB-3 for 24 h, respectively, using the water extract of A. auricula powder as a control. After treatment of NRK cells with 100 μL of 90 μmol mL−1 H2O2 solution for 2 h, the cells were collected after trypsinization and centrifuged at 1000 rpm for 5 min. The effects of A. auricula fermentation broth on the cell apoptosis of NRK cells by H2O2 were studied with a FACSVerse flow cytometer (BD, Franklin Lakes, NJ, USA) using an Annexin V-FITC/PI staining kit.
Animal experiment
The male BALB/c mice (20 ± 2 g, 7–8 weeks old) were purchased from Liaoning Changsheng Biotechnology Co., Ltd in Shenyang, China. The animal health license was issued by Xishan Biotechnology Co., Ltd in Suzhou, Jiangsu, China. All the experimental mice were grown in a specific-pathogen-free room with a temperature of 21–24 °C and a humidity of 60%–65% and were exposed to illumination for 12 hours a day, during which the mice were free to obtain sterile food and water. The experiments were carried out according to the regulations of the Experimental Animal Welfare and Ethics Committee of the Chinese Association for Experimental Animal Science.
After one week of acclimatization, all mice were randomly divided into 4 groups (5 mice in each group) according to Table 1, including control group, alcohol model group, A. auricula water extract (WE) group and A. auricula fermentation broth with three kinds of probiotics (FB-3) group, and the animal experiment administration method is shown in Fig. S1.† Before ethanol treatment, the mice in the WE and FB-3 groups were free to drink 2.67 mg mL−1 WE or FB-3 solution for 20 days, respectively. At the same time, the mice in the negative control and acute alcoholism groups drank distilled water freely. On the twentieth day, the mice in all groups except the control group were given 50% ethanol aqueous solution at a dose of 12 mL per kg body weight to establish an acute alcoholism model. After ethanol treatment, all mice were fasted for 12 hours. The mice were then sacrificed after blood extraction, and serum was collected by centrifugation at 4000 rpm for 10 min for further biochemical analysis with a Hitachi 3100 automatic biochemical analyzer (Hitachi, Tokyo, Japan). The organs of the livers and stomachs were weighed, and immersed in 10% neutral buffered formalin. The organs were embedded in paraffin, cut into 5 μm thick sections and stained with hematoxylin and eosin (HE) dyes. Each organ section was examined under a Nikon Ti–S fluorescence microscope (Nikon, Tokyo, Japan).
Table 1
In vivo experiments for testing FB-3 against mice with acute alcoholic injury
Group |
Distilled water |
50% ethanol (mL kg−1) |
Water extract (mg kg−1) |
FB-3 (mg kg−1) |
“—” the reagent is not applicable in this group. |
Negative control |
Drink freely |
— |
— |
— |
Positive control |
Drink freely |
12 |
— |
— |
Water extract |
— |
12 |
500 |
— |
FB-3 |
— |
12 |
— |
500 |
Results and discussion
Preparation of A. auricula fermentation broth
In this study, the fermentation broth (FB-1) of A. auricula was prepared by adding B. subtilis on the first day and fermentation for 13 days. The fermentation broth (FB-3) of A. auricula was prepared by adding B. subtilis and lactic acid bacteria on the first day and S. cerevisiae on the seventh day and fermentation for 13 days (Fig. 1a and b). The lactic acid bacteria and S. cerevisiae were introduced to compare the difference of one and three types of bacteria in the preparation of fermentation broth of A. auricula. First, the total number of B. subtilis colonies during the fermentation of FB-1 was monitored (Fig. 1c). The clump count significantly increased from 1 × 106 to 6 × 108 CFU mL−1 during the first six days and remained unchanged until the 13th day. This is because the A. auricula acting as the only carbon source for B. subtilis limits the probiotic reproduction, which was inconsistent with the growth pattern of B. subtilis with the microbial growth curve.30 Moreover, the content of soluble solids for the two kinds of fermentation broth decreased sharply on the second day, increased significantly on the third and fourth days, and then decreased on the sixth day (Fig. 1d). On the first day, the nutrients from soluble solids were consumed by the bacteria to produce metabolites through microbial fermentation. With the growth and reproduction of the microorganisms, the content of soluble solids began to increase on the second day, which was greater in FB-1 than that in FB-3 on the fourth and fifth days, possibly due to the competitive consumption by the two types of the microorganisms (B. subtilis and lactic acid bacteria). There was a significant decrease of the soluble solid content on the sixth day, which might be caused by the overconsumption of microorganisms. On the seventh day of fermentation, the S. cerevisiae was introduced into FB-3, and the soluble solid content increased to 1.39% on the eighth day. After dropping to 1.2% on the ninth day, the soluble solid content increased to 1.39% again and remained unchanged for FB-3 until the thirteenth day. However, the soluble solid content in FB-1 decreased significantly after 11-day fermentation. The optical density of FB-1 and FB-3 measured at a wavelength of 600 nm showed an overall increasing trend, indicating that the biomass of bacteria was increased during fermentation (Fig. 1e).31 Similarly, the pH of the two kinds of fermentation broth increased from 4.84 to 7.5 during the fermentation period (Fig. 1f). The pH value of FB-3 in the first four days of fermentation was lower than that of FB-1 because lactic acid bacteria produced lactic acid.32,33 After fermentation for seven days, the S. cerevisiae was added, and the pH of FB-3 was higher than that of FB-1. Moreover, the total sugar content in FB-1 and FB-3 decreased first and then increased, which was because the carbon source in the A. auricula was largely consumed,34 and the consumption rate was higher than the degradation rate of the A. auricula (Fig. 1g). In the period of 9–13 days, more and more nutrients were converted to sugar by microorganisms and the total sugar content increased to 7.05 (FB-1) and 8.85 (FB-3) mg mL−1, respectively. In addition, it is well known that B. subtilis belongs to Gram-positive aerobic bacteria and is a safe and beneficial microorganism for humans and animals. During the fermentation process, it can secrete extracellular enzymes such as protease, cellulase, chitinase, and amylase.35 The results in Fig. 1h show that the protein content of the two fermentation broths increased with the prolonged fermentation time. This might be due to the enzymes secreted by B. subtilis during fermentation, which broke down the insoluble proteins in the fruiting bodies of A. auricula into soluble proteins. At the end of the fermentation, the total protein content reached about 1.21 mg mL−1 for both FB-1 and FB-3.
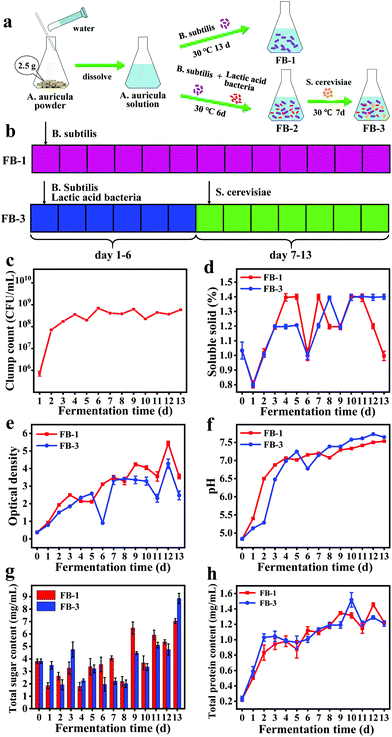 |
| Fig. 1 (a) Schematic illustration of the A. auricula fermentation broth (FB-1, A. auricula fermentation broth with B. subtilis only) and FB-3 (fermentation broth with B. subtilis, lactic acid bacteria and S. cerevisiae). (b) The B. subtilis was added on day 1 for FB-1, and the B. subtilis and lactic acid bacteria were added on day 1, and S. cerevisiae was added on day 7 for FB-2, (c) clump count, (d) soluble solid count, (e) optical density, (f) pH, (g) total sugar content, and (h) total protein content of FB-1 and FB-3. | |
Characterization
As shown in Fig. 2a, the UV-vis absorption spectrum of the WE was different from those of FB-1 and FB-3. The absorption peak at 260 nm for the WE was due to the n–π* transitions of aromatic C
O or C–N,36,37 and a shoulder peak was observed at 314 nm. However, the absorption peak at 260 nm red-shifted to 264 nm (FB-3) and 269 nm (FB-1). The presence of an absorption peak at 260–280 nm was found, indicating the possible presence of protein in the A. auricula fermentation broth.24 The FTIR results (Fig. 2b) indicated that the absorption profile is similar for the tested samples, the water extract, fermented FB-1, and FB-3. The absorption peaks of FB-1 and FB-3 at 3435 and 3445 cm−1 were attributed to the stretching vibration of the N–H and O–H bonds, while the peaks at 2928 and 2927 cm−1 were due to the stretching vibration of the C–H bond.38 Actually, the O–H bond was a characteristic peak of phenolic extracts.39 The stretching vibration of the C
O bond from COOH was found at 1734 cm−1 (FB-1) and 1729 cm−1 (FB-3), respectively.40 However, a very weak absorption peak was found at 1734 cm−1 in the FTIR spectrum of the WE. The absorption peaks near 1630 cm−1 were attributed to the stretching bands of C
O of amides.41 The absorption peaks at 1048, 1073 and 1077 cm−1 for the WE, FB-1 and FB-3 were due to the presence of C–O–C bonds in the sugar moiety, indicating that the polysaccharides present in the fermentation broth contain pyranoside bonds.42 In addition, the absorption peak in the range of 1400–1250 cm−1 was attributed to the stretching vibration of the C–N bond.43 The FTIR and UV-vis absorption spectra results both indicated that FB-1 and FB-3 might contain active substances such as proteins, polysaccharides and phenolics.
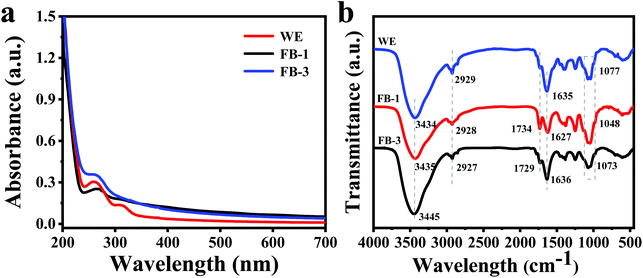 |
| Fig. 2 (a) UV-vis absorption and (b) FTIR spectra of the water extract (WE) of A. auricula, FB-1 (A. auricula fermentation broth with B. subtilis only) and FB-3 (fermentation broth with B. subtilis, lactic acid bacteria and S. cerevisiae). | |
Active substance analysis
Polysaccharides, protein and phenolics are the main components in A. auricula and have strong antioxidant activities.44 According to the results of UV-vis absorption and FITR spectra, the content of total sugar, protein and phenols in WE, FB-1 and FB-3 was measured based on the standard curves of total sugar, protein and phenolics in Fig. S2.† The results in Table 2 show that the total sugar and protein content of FB-1 and FB-3 after fermentation dramatically increased compared to that of the WE. That was due to the fermentation of microorganisms to produce more active substances.45 Huang et al.46 found that the reducing sugar content of longan pulp polysaccharides fermented with Lactobacillus fermentum increased significantly. Phenolics are known to be a natural plant metabolite that has antioxidant properties in some common plant-based foods such as tea, grain, vegetable, fruit and mushroom.47 In recent years, some studies have shown that fermented foods also contained antioxidants, including phenolics.13,48 As a natural antioxidant, phenolics can remove excess reactive oxygen species (ROS) and prevent oxidative damage.49 In this study, the total phenolic content in FB-1 and FB-3 was 8.347 and 11.139 mg g−1, respectively, and there was a decrease of the total phenolic content in the A. auricula fermentation broth. Microorganisms could hydrolyze complex phenolics into other substances with higher antioxidant activity.50 Similar results were also found by Oh et al.,51 that is, the decline of total phenolics in blueberry fermentation broth with Bacillus amyloliquefaciens, Lactobacillus brevis and Starmerella bombicola. Zhang et al.52 also found that the phenolic content in fermented Diospyros lotus decreased with the increase of fermentation time. The reduction of total phenolic content might be due to the bioconversion of the unstable phenolic compounds into other bioactive compounds during fermentation.53
Table 2 Total sugar, protein and phenolic content of FB-1, FB-3 and water extract of A. auricula
Components |
FB-1 |
FB-3 |
WE |
Sugar (mg g−1) |
511.45 (±13.94) |
671.91 (±31.58) |
297.03 (±14.00) |
Protein (mg g−1) |
88.83 (±1.02) |
91.87 (±2.73) |
18.04 (±2.57) |
Phenolics (mg g−1) |
8.35 (±0.04) |
11.14 (±0.01) |
15.24 (±0.01) |
Antioxidant activity characterization
It was reported that the fermentation of microorganisms could produce more bioactive substances, thus enhancing the antioxidant activity.54 In this research, DPPH and hydroxyl radical scavenging abilities were evaluated. The DPPH free radical scavenging ability was determined based on the hydrogen supply ability of antioxidants.55Fig. 3a shows the DPPH free-radical scavenging effect of FB-1, FB-3 and water extract, which exhibits a concentration-dependent behavior. The half-maximal effective concentration (EC50) of the water extract was 2.229 mg mL−1 due to the active ingredients from A. auricula, such as polysaccharides, protein and phenol, which have the ability to scavenge DPPH free radicals. However, compared with the water extract, the FB-1 (2.004 mg mL−1) and FB-3 (1.678 mg mL−1) showed stronger DPPH free radical scavenging ability. After fermentation with microorganisms, FB-1 and FB-3 contained more active ingredients with hydrogen supply capacity, which could scavenge DPPH free radicals.12 Furthermore, the antioxidant activity was related to the molecular weight of the active ingredient; the lower the molecular weight, the stronger the antioxidant activity it had.44,56
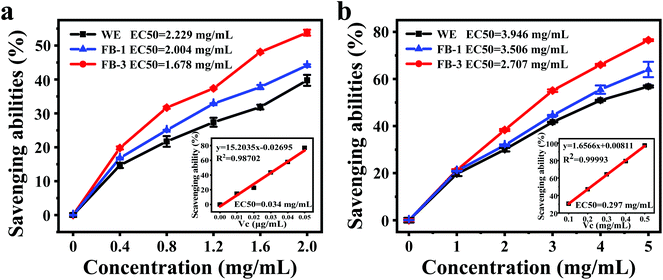 |
| Fig. 3 (a) DPPH radical scavenging ability of the water extract (WE), FB-1, FB-3 and vitamin C (inset), (b) hydroxyl radical scavenging ability of the water extract (WE), FB-1, FB-3 and vitamin C (inset). EC50: half maximal effective concentration. Vc: vitamin C. | |
Hydroxyl radicals are typical reactive oxygen species that can capture electrons, thus killing red blood cells, causing the degradation of DNA, cell membranes and organisms.27 Therefore, it is very important to remove the hydroxyl radicals to protect the body from oxidative damage. The hydroxyl radical scavenging ability analysis of FB-1, FB-3 and WE demonstrated that all the samples were capable of scavenging hydroxyl radicals (Fig. 3b). The A. auricula fermentation broth showed strong hydroxyl radical scavenging ability, although its scavenging ability was weaker than ascorbic acid, in which FB-3 had stronger hydroxyl radical scavenging ability than FB-1. The EC50 values of FB-1 and FB-3 were 3.642 and 3.037 mg mL−1, respectively. What's more, the WE without fermentation had the weakest ability to scavenge hydroxyl free radicals, and its EC50 was only 3.985 mg mL−1. These results proved that FB-3 had a better ability to scavenge hydroxyl radicals than the WE. Liu et al.57 showed that the fermented rice bran had improved hydroxyl radical scavenging activity after incubation with Grifola frondosa. Similarly, Wang et al.14 reported that rice and wheat bran fermented with L. plantarum 423 could scavenge hydroxyl radicals.
Cell viability and resistance to hydrogen peroxide damage
The viability of NRK cells treated with FB-1, FB-3 and WE for 24 h is shown in Fig. S3.† With the increase of concentration, the cell viability was significantly increased after FB-1 and FB-3 treatment. This indicated that the fermentation broth of A. auricula had a promoting effect on the growth of NRK cells, and the cell viability of FB-3 was greater than that of FB-1. However, the water extract had an inhibitory effect on cell growth when its concentration was more than 0.125 mg mL−1. Reactive oxygen species (ROS) are the main cause of cell death, which can lead to the peroxidation of polyunsaturated fatty acids in biofilms.58,59 Hydrogen peroxide is a kind of reactive oxygen species and a central redox signaling molecule.60 High concentration of H2O2 can initiate apoptosis and even necrosis,61 and lead to certain pathophysiological conditions. The protective effect of the A. auricula fermentation broth on hydrogen peroxide damage was analyzed by apoptosis or necrosis analysis of NRK cells (Fig. 4). There was no obvious death of the cells that were not treated with any samples (Fig. 4a), while the apoptosis rate of cells treated with 90 μM mL−1 H2O2 increased to 85.93% with a total death rate of 95.4% (Fig. 4b). After the cells were treated with 0.5 mg mL−1 WE, FB-1 and FB-3 upon exposure to H2O2, the sum of early and late apoptosis rates was 87.93%, 83.92% and 24.39%, respectively (Fig. 4f). The survival rates of NRK cells in Fig. 4c–e were 12.07%, 16.08%, and 75.61%, respectively. This demonstrated that, compared with the WE and FB-1, FB-3 could well protect NRK cells from H2O2 damage. Mushrooms have been regarded as functional foods with medicinal value for centuries that contain a variety of antioxidant compounds including polysaccharides, phenolics, proteins and melanin, with a protective effect on hydrogen peroxide damage. The polysaccharides isolated from Inonotus obliquus have protective effects on oxidative damage in RINm5F pancreatic β-cells caused by hydrogen peroxide.62 Actually, the phenolic components from the water extract of A. auricula can also reduce the cytotoxicity of H2O2 on baby hamster fibroblast cells.63 After fermentation, the antioxidant activities of proteins and polysaccharides in FB-1 and FB-3 were significantly increased, so that the cells could better prevent the damage of H2O2. Eun et al.64 found that Curcuma longa L. fermented with L. plantarum sp. was effective in preventing cell death induced by H2O2.
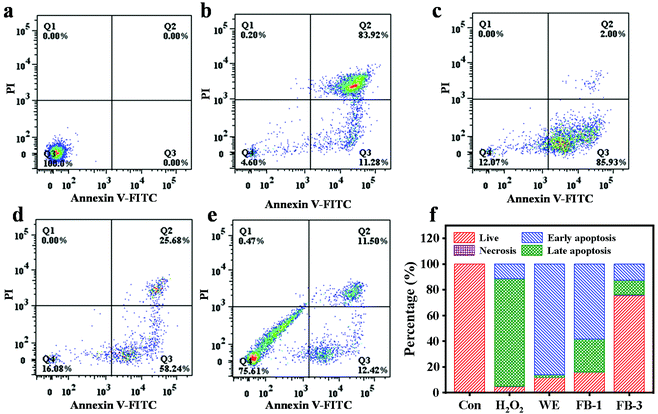 |
| Fig. 4 Flow cytometry analysis of NRK treated with (a) blank medium, (b) 90 μM mL−1 H2O2, (c) 0.5 mg mL−1 WE, (d) 0.5 mg mL−1 FB-1, and (e) 0.5 mg mL−1 FB-3 and (f) the statistical chart of flow cytometry analysis. | |
Analysis of serum biochemical indicators
Fig. 5 shows the change of main blood indexes in mice with acute alcoholism before and after treatment with WE, FB-1 and FB-3 of A. auricula. When the liver is injured, the levels of alanine aminotransferase (ALT) and aspartate aminotransferase (AST) in serum will increase, which can reflect the degree of liver damage.65 Compared to the ALT (33.88 ± 1.63 U L−1) and AST (140.94 ± 6.17 U L−1) in the negative control group, the acute alcohol intake led to elevated ALT (62.82 ± 3.80 U L−1) and AST (200.16 ± 18.10 U L−1) in the acute alcoholism group (Fig. 5a and b). This indicated that the model of acute alcoholism (AA) was successfully established, and the ALT and AST were released into the blood when the cell membranes of the liver and stomach were damaged. The increase in ALT and AST levels in serum could directly reflect cell necrosis.66 In contrast to the acute alcoholism group, the ALT (48.63 ± 2.56 U L−1) and AST (178.58 ± 7.00 U L−1) levels in the serum of the WE group were reduced. Meanwhile, the ALT (41.28 ± 3.18 U L−1) and AST (154.63 ± 6.07 U L−1) values of the FB-3 group also decreased significantly, and the decreased amount of the FB-3 group was even better than that of the WE group. The results showed that, compared with the A. auricula water extract, the A. auricula fermentation broth could inhibit the increase of ALT and AST levels in the serum of mice with acute alcoholism. This was closely related to the effect of microorganisms. A previous study also confirmed that, compared with unfermented ginseng, fermented ginseng could significantly reduce the levels of AST and ALT in serum, because microorganisms could hydrolyze ginsenosides into substances with stronger physiological activity.67 The phenolic compounds in the A. auricula fermentation broth could be decomposed by microorganisms into other active ingredients to reduce the levels of AST and ALT. The levels of total cholesterol (TC) and triglycerides (TG) in the serum of mice with acute alcoholism are shown in Fig. 5c and d which show that the levels of TC (3.52 ± 0.33 mmol g−1) and TG (2.87 ± 0.36 mmol g−1) in the serum of the acute alcoholism group were higher than those in the normal group (TC 2.26 ± 0.99 mmol g−1 and TG 1.40 ± 0.21 mmol g−1). Compared with the acute alcoholism group, the TC and TG contents in the serum of the FB-3 group were significantly reduced. This indicated that the A. auricula fermentation broth could inhibit the increase of endogenous lipid levels in serum, thereby slowing the process of alcohol damage.68
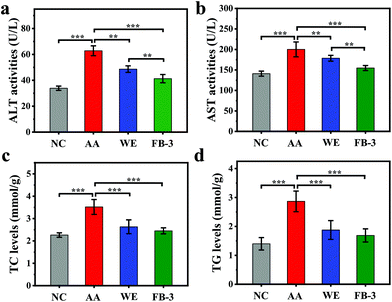 |
| Fig. 5 Effects of the WE and FB-3 on (a) ALT activities, (b) AST activities, (c) TC levels, and (d) TG levels in serum (*p < 0.05, **p < 0.01, and ***p < 0.001). NC, negative control; AA, acute alcoholism. | |
Histopathological analysis
The histopathological change in the stomach and liver is shown in Fig. 6. In the control group, the gastric mucosa glands were arranged orderly and the cells were evenly distributed (Fig. 6a). In contrast, the gastric wall in the acute alcoholism group was significantly thinned and the gastric mucosa was severely damaged (Fig. 6b), suggesting that the alcohol administration could cause damage to the stomachs of the mice.69 After treatment with the water extract or FB-3, the cells of gastric mucosa were more closely arranged with less cell loss and mucosal damage (Fig. 6c and d). This indicated that the water extract or FB-3 treatment can protect the stomach from alcohol damage. In addition, histopathological signs of alcoholic liver injury were found in the mice that received alcohol administration (Fig. 6f) as compared with the livers of mice without alcohol treatment (Fig. 6e). In the control group, the liver tissue morphology was polygonal, and the hepatocytes were arranged orderly with certain density. However, the mice fed with alcohol showed steatosis in the pericentral regions of hepatocytes and midzonal hepatic necrosis. The livers showed severe damage, such as significant cell swelling, cellular solute edema and balloon-like lesions. In contrast, the mice treated with the water extract and FB-3 did not show midzonal hepatic necrosis after exposure to alcohol (Fig. 6g and h), and steatosis remission was found in the livers of FB-3 treated mice (Fig. 6h).70 This result indicated that the FB-3 from A. auricula had a protective effect on the gastric mucosa and hepatocytes of mice with acute alcoholism, which is similar to or better than that of the water extract of A. auricula. The research of Cheng et al.71 found that Monascus-fermented red mold rice could reduce hepatic cell damage (steatosis).
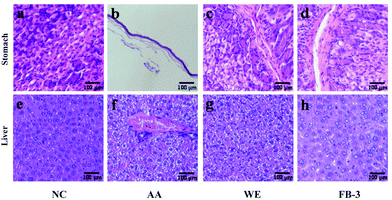 |
| Fig. 6 Effects of the WE and FB-3 on the cells in stomach tissue: (a) negative control (NC) group, (b) acute alcoholism (AA) group, (c) water extract (WE) group, and (d) FB-3 group; and in liver tissue: (e) negative control (NC) group, (f) acute alcoholism (AA) group, (g) water extract (WE) group, and (h) FB-3 group of alcohol-intoxicated mice. The scale was 100 μm. | |
Conclusion
In this study, FB-1 and FB-3 were prepared from A. auricula after fermentation for 13 days. The total sugar content was 511.45 ± 13.93 and 671.91 ± 31.58 mg g−1, respectively, for FB-1 and FB-3, with a total protein content of 88.83 ± 1.02 (FB-1) and 91.87 ± 2.73 mg g−1 (FB-3). The FTIR characterization showed that the FB-1 and FB-3 contained hydroxyl, carboxyl, amino and C–O–C groups. The total phenolic content of FB-1 and FB-3 was 8.35 and 11.14 mg g−1, respectively, and the FB-3 showed a stronger ability to scavenge DPPH and hydroxyl free radicals than FB-1. The FB-3 and FB-1 had a promoting effect on the growth of NRK cells, and the FB-3 had a better protection effect on NRK cells from H2O2 damage. In addition, in vivo animal experiments in mice showed that the FB-3 had a certain protective effect on the gastric mucosa and hepatocytes of mice with acute alcoholism.
Conflicts of interest
The authors declare no competing financial interest.
Acknowledgements
This work was supported by the National Science Fund for Distinguished Young Scholars of China (31925031) and the National Key Research and Development Program of China (2018YFD0901106).
Notes and references
- E. C. Prom-Wormley, J. Ebejer, D. M. Dick and M. S. Bowers, The genetic epidemiology of substance use disorder: A review, Drug Alcohol Depend., 2017, 180, 241–259 CrossRef.
-
Global status report on alcohol and health, ed. V. Poznyak and D. Rekve, WHO, Geneva, 2018 Search PubMed.
- S. F. Neong, J. Bhattacharyya, A. Leahy, I. R. Sargeant and D. L. Morris, PTU-259 Stent placement in palliation of oesophago-gastric cancer: the story so far, Gut, 2012, 61, 292–293 CrossRef.
- A. L. Klatsky, Alcohol, cardiovascular diseases and diabetes mellitus, Pharmacol. Res., 2007, 55, 237–247 CrossRef CAS.
- J. Rehm, G. Gmel, C. T. Sempos and M. Trevisan, Alcohol-related morbidity and mortality, Alcohol Res. Health, 2003, 27, 39–51 Search PubMed.
- T. M. Leung and N. Nieto, CYP2E1 and oxidant stress in alcoholic and non-alcoholic fatty liver disease, J. Hepatol., 2013, 58, 395–398 CrossRef CAS.
- Q. Wu, Z. Tan, H. Liu, L. Gao, S. Wu, J. Luo, W. Zhang, T. Zhao, J. Yu and X. Xu, Chemical characterization of Auricularia auricula polysaccharides and its pharmacological effect on heart antioxidant enzyme activities and left ventricular function in aged mice, Int. J. Biol. Macromol., 2010, 46, 284–288 CrossRef CAS.
- X. Wang, Y. Lan, Y. Zhu, S. Li, M. Liu, X. Song, H. Zhao, W. Liu, J. Zhang and S. Wang, Hepatoprotective effects of Auricularia cornea var. Li. polysaccharides against the alcoholic liver diseases through different metabolic pathways, Sci. Rep., 2018, 8, 1–12 CrossRef.
- R. Hou, X. Liu, J. Yan, K. Xiang, X. Wu, W. Lin, G. Chen, M. Zheng and J. Fu, Characterization of natural melanin from Auricularia auricula and its hepatoprotective effect on acute alcohol liver injury in mice, Food Funct., 2019, 10, 1017–1027 RSC.
- C. Gao, Y. Wang, C. Wang and Z. Wang, Antioxidant and immunological activity in vitro of polysaccharides from Gomphidius rutilus mycelium, Carbohydr. Polym., 2013, 92, 2187–2192 CrossRef CAS.
- J. Xu, R. Yue, J. Liu, H. Ho, T. Yi, H. Chen and Q. Han, Structural diversity requires individual optimization of ethanol concentration in polysaccharide precipitation, Int. J. Biol. Macromol., 2014, 67, 205–209 CrossRef CAS.
- H. Y. Shin, S. M. Kim, J. H. Lee and S. T. Lim, Solid-state fermentation of black rice bran with Aspergillus awamori and Aspergillus oryzae: Effects on phenolic acid composition and antioxidant activity of bran extracts, Food Chem., 2019, 272, 235–241 CrossRef CAS.
- T. Xia, B. Zhang, S. Li, B. Fang, W. Duan, J. Zhang, J. Song and M. Wang, Vinegar extract ameliorates alcohol-induced liver damage associated with the modulation of gut microbiota in mice, Food Funct., 2020, 11, 2898–2909 RSC.
- M. Wang, M. Lei, N. Samina, L. Chen, C. Liu, T. Yin, X. Yan, C. Wu, H. He and C. Yi, Impact of Lactobacillus plantarum 423 fermentation on the antioxidant activity and flavor properties of rice bran and wheat bran, Food Chem., 2020, 330, 1–27 Search PubMed.
- M. Y. Juan and C. C. Chou, Enhancement of antioxidant activity, total phenolic and flavonoid content of black soybeans by solid state fermentation with Bacillus subtilis BCRC 14715, Food Microbiol., 2010, 27, 586–591 CrossRef CAS.
- C. Huang, M. Sun, Y. Yang, F. Wang, X. Ma, J. Li, Y. Wang, Q. Ding, H. Ying, H. Song, Y. Wu, Y. Jiang, X. Jia, Q. Ba and H. Wang, Titanium dioxide nanoparticles prime a specific activation state of macrophages, Nanotoxicology, 2017, 11, 737–750 Search PubMed.
- B. Soufi, C. Kumar, F. Gnad, M. Mann, I. Mijakovic and B. Macek, Stable isotope labeling by amino acids in cell culture (SILAC) applied to quantitative proteomics of Bacillus subtilis, J. Proteome Res., 2010, 9, 3638–3646 CrossRef CAS.
- R. Kim, J. H. Jang, W. J. Park, H. K. Kim, H. S. Kwak and J. S. Lee, Production of Antihypertensive Angiotensin I-Converting Enzyme Inhibitor-Enriched Edible Yeast Using Gugija (Lycium chinesis Mill), Mycobiology, 2010, 38, 206–209 CrossRef.
- T. C. Finimundy, G. Gambato, R. Fontana, M. Camassola, M. Salvador, J. H. S. Mourad, J. A. P. Henriques, A. J. P. Dillon and M. Roesch-Ely, Aqueous extracts of Lentinula edodes and Pleurotus sajor-caju exhibit high antioxidant capability and promising in vitro antitumor activity, Nutr. Res., 2013, 33, 76–84 CrossRef CAS.
- X. Hou and W. Chen, Optimization of extraction process of crude polysaccharides from wild edible BaChu mushroom by response surface methodology, Carbohydr. Polym., 2008, 72, 67–74 CrossRef CAS.
- Q. Q. Liang and Y. S. Li, A rapid and accurate method for determining protein content in dairy products based on asynchronous-injection alternating merging zone flow-injection spectrophotometry, Food Chem., 2013, 141, 2479–2485 CrossRef CAS.
- J. Qiu, H. Zhang and Z. Wang, Auricularia auriculajudae polysaccharide-cisplatin complexes conjugated with folic acid as new tumor targeting agents, Int. J. Biol. Macromol., 2018, 120, 966–974 CrossRef CAS.
- Z. Ye, W. Wang, Q. Yuan, H. Ye, Y. Sun, H. Zhang and X. Zeng, Box-Behnken design for extraction optimization, characterization and in vitro antioxidant activity of Cicer arietinum L. hull polysaccharides, Carbohydr. Polym., 2016, 147, 354–364 CrossRef CAS.
- J. Xie, Z. Wang, M. Shen, S. Nie, B. Gong, H. Li, Q. Zhao, W. Li and M. Xie, Sulfated modification, characterization and antioxidant activities of polysaccharide from Cyclocarya paliurus, Food Hydrocolloids, 2016, 53, 7–15 CrossRef CAS.
- I. M. Valente, M. R. G. Maia, N. Malushi, H. M. Oliveira, L. Papa, J. A. Rodrigues, A. J. M. Fonseca and A. R. J. Cabrita, Profiling of phenolic compounds and antioxidant properties of European varieties and cultivars of Vicia faba L. pods, Phytochemistry, 2018, 152, 223–229 CrossRef CAS.
- D. Li, X. Na, H. Wang, Y. Xie, S. Cong, Y. Song, X. Xu, B. W. Zhu and M. Tan, Fluorescent carbon dots derived from Maillard reaction products: their properties, biodistribution, cytotoxicity, and antioxidant activity, J. Agric. Food Chem., 2018, 66, 1569–1575 CrossRef CAS.
- Y. Qu, C. Li, C. Zhang, R. Zeng and C. Fu, Optimization of infrared-assisted extraction of Bletilla striata polysaccharides based on response surface methodology and their antioxidant activities, Carbohydr. Polym., 2016, 148, 345–353 CrossRef CAS.
- J. Geng, X. Song, X. Zhang, S. Tie, L. Cao and M. Tan, Hydrophilic food-borne nanoparticles from beef broth as novel nanocarriers for zinc, J. Agric. Food Chem., 2019, 67, 6995–7004 CrossRef CAS.
- J. Wang, Y. Zhao, B. Zhang and C. Guo, Protective effect of total phenolic compounds from Inula helenium on hydrogen peroxide-induced oxidative stress in SH-SY5Y cells, Indian J. Pharm. Sci., 2015, 77, 163–169 CrossRef CAS.
- M. Peleg and M. G. Corradini, Microbial growth curves: what the models tell us and what they cannot, Crit. Rev. Food Sci. Nutr., 2011, 51, 917–945 CrossRef.
- I. Mytilinaios, M. Salih, H. K. Schofield and R. J. Lambert, Growth curve prediction from optical density data, Int. J. Food Microbiol., 2012, 154, 169–176 CrossRef CAS.
- F. Valerio, P. Lavermicocca, M. Pascale and A. Visconti, Production of phenyllactic acid by lactic acid bacteria: an approach to the selection of strains contributing to food quality and preservation, FEMS Microbiol. Lett., 2004, 233, 289–295 CrossRef CAS.
- P. H. Keen, N. K. Slater and A. F. Routh, Encapsulation of lactic acid bacteria in colloidosomes, Langmuir, 2012, 28, 16007–16014 CrossRef CAS.
- L. M. M. Ferreira, A. Mendes-Ferreira, C. M. J. Benevides, D. Melo, A. S. G. Costa, A. Mendes-Faia and M. Oliveira, Effect of controlled microbial fermentation on nutritional and functional characteristics of cowpea bean flours, Foods, 2019, 8, 1–15 CrossRef.
- H. T. M. Tran, B. Cheirsilp, B. Hodgson and K. Umsakul, Potential use of Bacillus subtilis in a co-culture with Clostridium butylicum for acetone–butanol–ethanol production from cassava starch, Biochem. Eng. J., 2010, 48, 260–267 CrossRef CAS.
- H. Wang, W. Su and M. Tan, Endogenous fluorescence carbon dots derived from food items, Innovation, 2020, 1, 57–67 Search PubMed.
- D. Li, X. Na, W. Zhou, C. Wang, Y. Li, B. W. Zhu and M. Tan, Adverse effects of fluorescent carbon dots from canned yellow croaker on cellular respiration and glycolysis, Food Funct., 2019, 10, 1123–1131 RSC.
- T. L. Nguyen, D. Wang, Y. Hu, Y. Fan, J. Wang, S. Abula, L. Guo, J. Zhang, S. K. khakame and B. K. Dang, Immuno-enhancing activity of sulfated Auricularia auricula polysaccharides, Carbohydr. Polym., 2012, 89, 1117–1122 CrossRef CAS.
- F. S. Grasel, M. F. Ferrao and C. R. Wolf, Development of methodology for identification the nature of the polyphenolic extracts by FTIR associated with multivariate analysis, Spectrochim. Acta, Part A, 2016, 153, 94–101 CrossRef CAS.
- G. Ma, B. M. Kimatu, L. Zhao, W. Yang, F. Pei and Q. Hu, In vivo fermentation of a Pleurotus eryngii polysaccharide and its effects on fecal microbiota composition and immune response, Food Funct., 2017, 8, 1810–1821 RSC.
- L. Rastogi and J. Arunachalam, Sunlight based irradiation strategy for rapid green synthesis of highly stable silver nanoparticles using aqueous garlic (Allium sativum) extract and their antibacterial potential, Mater. Chem. Phys., 2011, 129, 558–563 CrossRef CAS.
- D. Qiao, J. Liu, C. Ke, Y. Sun, H. Ye and X. Zeng, Structural characterization of polysaccharides from Hyriopsis cumingii, Carbohydr. Polym., 2010, 82, 1184–1190 CrossRef CAS.
- S. Song, S. Li, N. Su, J. Li, F. Shi and M. Ye, Structural characterization, molecular modification and hepatoprotective effect of melanin from Lachnum YM226 on acute alcohol-induced liver injury in mice, Food Funct., 2016, 7, 3617–3627 RSC.
- H. Chen, M. Zhang, Z. Qu and B. Xie, Antioxidant activities of different fractions of polysaccharide conjugates from green tea (Camellia Sinensis), Food Chem., 2008, 106, 559–563 CrossRef CAS.
- N. Zeybek, R. A. Rastall and A. O. Buyukkileci, Utilization of xylan-type polysaccharides in co-culture fermentations of Bifidobacterium and Bacteroides species, Carbohydr. Polym., 2020, 236, 116076 CrossRef CAS.
- F. Huang, R. Hong, Y. Yi, Y. Bai, L. Dong, X. Jia, R. Zhang, G. Wang, M. Zhang and J. Wu, In vitro digestion and human gut microbiota fermentation of longan pulp polysaccharides as affected by Lactobacillus fermentum fermentation, Int. J. Biol. Macromol., 2020, 147, 363–368 CrossRef CAS.
- J. M. Hussein, D. D. Tibuhwa, A. M. Mshandete and A. K. Kivaisi, Antioxidant properties of seven wild edible mushrooms from Tanzania, Afr. J. Food Sci., 2015, 9, 471–479 CrossRef.
- C. W. Ho, A. M. Lazim, S. Fazry, U. Zaki and S. J. Lim, Varieties, production, composition and health benefits of vinegars: A review, Food Chem., 2017, 221, 1621–1630 CrossRef CAS.
- F. Giampieri, J. M. Alvarez-Suarez, L. Mazzoni, T. Y. Forbes-Hernandez, M. Gasparrini, A. M. Gonzalez-Paramas, C. Santos-Buelga, J. L. Quiles, S. Bompadre, B. Mezzetti and M. Battino, Polyphenol-rich strawberry extract protects human dermal fibroblasts against hydrogen peroxide oxidative damage and improves mitochondrial functionality, Molecules, 2014, 19, 7798–7816 CrossRef.
- M. Dueñas, D. Fernández, T. Hernández, I. Estrella and R. Muñoz, Bioactive phenolic compounds of cowpeas (Vigna sinensisL). Modifications by fermentation with natural microflora and withLactobacillus plantarumATCC 14917, J. Sci. Food Agric., 2005, 85, 297–304 CrossRef.
- B. T. Oh, S. Y. Jeong, P. Velmurugan, J. H. Park and D. Y. Jeong, Probiotic-mediated blueberry (Vaccinium corymbosum L.) fruit fermentation to yield functionalized products for augmented antibacterial and antioxidant activity, J. Biosci. Bioeng., 2017, 124, 542–550 CrossRef CAS.
- Z. P. Zhang, J. Ma, Y. Y. He, J. Lu and D. F. Ren, Antioxidant and hypoglycemic effects of Diospyros lotus fruit fermented with Microbacterium flavum and Lactobacillus plantarum, J. Biosci. Bioeng., 2018, 125, 682–687 CrossRef CAS.
- R. Bhat, L. C. Suryanarayana, K. A. Chandrashekara, P. Krishnan, A. Kush and P. Ravikumar, Lactobacillus plantarum mediated fermentation of Psidium guajava L. fruit extract, J. Biosci. Bioeng., 2015, 119, 430–432 CrossRef CAS.
- T. Chen, M. Piao, S. M. Ehsanur Rahman, L. Zhang and Y. Deng, Influence of fermentation on antioxidant and hypolipidemic properties of maifanite mineral water-cultured common buckwheat sprouts, Food Chem., 2020, 321, 126741 CrossRef CAS.
- L. Liang, X. Wu, T. Zhao, J. Zhao, F. Li, Y. Zou, G. Mao and L. Yang, In vitro bioaccessibility and antioxidant activity of anthocyanins from mulberry (Morus atropurpurea Roxb.) following simulated gastro-intestinal digestion, Food Res. Int., 2012, 46, 76–82 CrossRef CAS.
- B. Ray, C. Hutterer, S. S. Bandyopadhyay, K. Ghosh, U. R. Chatterjee, S. Ray, I. Zeittrager, S. Wagner and M. Marschall, Chemically engineered sulfated glucans from rice bran exert strong antiviral activity at the stage of viral entry, J. Nat. Prod., 2013, 76, 2180–2188 CrossRef CAS.
- Q. Liu, X. Cao, X. Zhuang, W. Han, W. Guo, J. Xiong and X. Zhang, Rice bran polysaccharides and oligosaccharides modified by Grifola frondosa fermentation: Antioxidant activities and effects on the production of NO, Food Chem., 2017, 223, 49–53 CrossRef CAS.
- J. Kim, J. Kim, H. Kook and W. J. Park, PICOT alleviates myocardial ischemia-reperfusion injury by reducing intracellular levels of reactive oxygen species, Biochem. Biophys. Res. Commun., 2017, 485, 807–813 CrossRef CAS.
- M. M. Senchuk, D. J. Dues, C. E. Schaar, B. K. Johnson, Z. B. Madaj, M. J. Bowman, M. E. Winn and J. M. Van Raamsdonk, Activation of DAF-16/FOXO by reactive oxygen species contributes to longevity in long-lived mitochondrial mutants in Caenorhabditis elegans, PLoS Genet., 2018, 14, 1–27 Search PubMed.
- G. Bartosz, Reactive oxygen species: destroyers or messengers?, Biochem. Pharmacol., 2009, 77, 1303–1315 CrossRef CAS.
- S. Tie, X. Zhang, H. Wang, Y. Song and M. Tan, Procyanidins-loaded complex coacervates for improved stability by self-crosslinking and calcium ions chelation, J. Agric. Food Chem., 2020, 68, 3163–3170 CrossRef CAS.
- Y. C. Sim, J. S. Lee, S. Lee, Y. K. Son, J. E. Park, J. E. Song, S. J. Ha and E. K. Hong, Effects of polysaccharides isolated from Inonotus obliquus against hydrogen peroxide-induced oxidative damage in RINm5F pancreatic beta-cells, Mol. Med. Rep., 2016, 14, 4263–4270 CrossRef CAS.
- F. Oke and B. Aslim, Protective effect of two edible mushrooms against oxidative cell damage and their phenolic composition, Food Chem., 2011, 128, 613–619 CrossRef CAS.
- C. S. Eun, J. S. Lim, J. Lee, S. P. Lee and S. A. Yang, The protective effect of fermented Curcuma longa L. on memory dysfunction in oxidative stress-induced C6 gliomal cells, proinflammatory-activated BV2 microglial cells, and scopolamine-induced amnesia model in mice, BMC Complementary Altern. Med., 2017, 17, 367 CrossRef.
- J. Wang, Y. Zhang, Y. Zhang, Y. Cui, J. Liu and B. Zhang, Protective effect of Lysimachia christinae against acute alcoholinduced liver injury in mice, BioSci. Trends, 2012, 6, 89–97 CAS.
- M. Tahir, M. U. Rehman, A. Lateef, A. Q. Khan, R. Khan, W. Qamar, O. O'Hamiza, F. Ali, S. K. Hasan and S. Sultana, Diosmin abrogates chemically induced hepatocarcinogenesis via alleviation of oxidative stress, hyperproliferative and inflammatory markers in murine model, Toxicol. Lett., 2013, 220, 205–218 CrossRef CAS.
- J. Fan, Y. Wang, Y. You, Z. Ai, W. Dai, C. Piao, J. Liu and Y. Wang, Fermented ginseng improved alcohol liver injury in association with changes in the gut microbiota of mice, Food Funct., 2019, 10, 5566–5573 RSC.
- L. Zheng, G. Zhai, J. Zhang, L. Wang, Z. Ma, M. Jia and L. Jia, Antihyperlipidemic and hepatoprotective activities of mycelia zinc polysaccharide from Pholiota nameko SW-02, Int. J. Biol. Macromol., 2014, 70, 523–529 CrossRef CAS.
- H. Jeong, D. Kim, H. J. Song, S. Lee, M. Kim, K.-J. Kim and G. D. Han, β-Glucan-enriched fermented barley bran (Sigumjang meju) extracts attenuates gastric mucosal injury induced by acute alcohol intake in vivo, Food Biosci., 2019, 28, 20–26 CrossRef CAS.
- H. Kono, I. Rusyn, M. Yin, E. Gabele, S. Yamashina, A. Dikalova, M. B. Kadiiska, H. D. Connor, R. P. Mason, B. H. Segal, B. U. Bradford, S. M. Holland and R. G. Thurman, NADPH oxidase-derived free radicals are key oxidants in alcohol-induced liver disease, J. Clin. Invest., 2000, 106, 867–872 CrossRef CAS.
- C. f. Cheng and T. M. Pan, Protective Effect of monascus-fermented red mold rice against alcoholic liver disease by attenuating oxidative stress and inflammatory response, J. Agric. Food Chem., 2011, 59, 9950–9957 CrossRef CAS.
Footnote |
† Electronic supplementary information (ESI) available: Experimental design of animals; standard curves of total sugar, protein and phenolic contents of water extract, FB-1 and FB-3 of A. auricula; cell viability. See DOI: 10.1039/d0fo02688c |
|
This journal is © The Royal Society of Chemistry 2021 |
Click here to see how this site uses Cookies. View our privacy policy here.