DOI:
10.1039/D0FO02653K
(Paper)
Food Funct., 2021,
12, 362-372
Taxifolin ameliorate high-fat-diet feeding plus acute ethanol binge-induced steatohepatitis through inhibiting inflammatory caspase-1-dependent pyroptosis
Received
10th October 2020
, Accepted 25th November 2020
First published on 26th November 2020
Abstract
Excessive alcohol drinking and a high-fat diet (HFD) promote steatohepatitis in the comorbidity of NAFLD and AFLD. Taxifolin (TAX) is a rich dihydroxyflavone compound found in onions, milk thistle and Douglas fir. We aimed to explore the intervention mechanism of TAX on chronic steatohepatitis induced by HFD feeding plus acute ethanol binge. We established an in vivo model by HFD feeding plus a single dose of ethanol binge, and established an in vitro model by oleic acid or palmitic acid on HepG2 cells to induce lipid accumulation. TAX regulated lipid synthesis by inhibiting the expression of SREBP1 and upregulating the PPARγ level. In addition, TAX inhibited the expression of P2X7R, IL-1β, and caspase-1. Moreover, TAX reduced the expression of caspase-1 activation; thereby inhibiting the recruitment of macrophages and neutrophils. TAX also improved the inflammatory response caused by caspase-1 activation in steatotic hepatocytes. TAX exhibited an inhibitory effect on lipid accumulation and caspase-1-related pyroptosis. Collectively, TAX has therapeutic potential as an intervention of steatohepatitis induced by alcohol combined with HFD and for preventing non-alcoholic fatty liver degeneration targeting caspase-1-dependent pyroptosis.
1. Introduction
Fatty liver (hepatosteatosis) refers to diffuse fatty infiltration of the liver, accompanied by inflammation, liver cell necrosis, and apoptosis. Clinically, it can be roughly divided into alcoholic fatty liver disease (AFLD) and non-alcoholic fatty liver disease (NAFLD). AFLD refers to chronic liver disease caused by long-term heavy drinking.1 NAFLD is a comprehensive disease of metabolic stress, without a history of drinking, and is closely related to obesity, type 2 diabetes, and other metabolic diseases.2–5 When unchecked, both NAFLD and AFLD develop to steatohepatitis, fibrosis, cirrhosis, and even hepatocellular carcinoma.6 In addition, clinical data showed that alcohol can promote the production of inflammation, fibrosis, and alcohol dehydrogenase genes,7 which in turn accelerates the development of NAFLD.8
Among these, the most common and important cause of NAFLD is obesity.9,10 The relationship between obesity and a fatty liver is more serious than the relationship between excessive alcohol consumption and a fatty liver. Drinking alcohol is a common habit in many human societies, but it is unclear how alcohol and NAFLD have a synergistic effect. Although, moderate amounts of alcohol have been shown to help cheer the spirit, excessive alcohol uptake has serious toxic effects on the liver, and alcohol abuse has become a serious public health problem in the world today. Obesity is an extremely important risk factor for alcoholic liver disease, and obese people who drink alcohol are more likely to suffer from liver damage.11,12 The reason for this may be that adipose tissue can promote monocytes to secrete inflammatory factors such as Interleukin-1β (IL-1β), which induce peroxidation and generate free radicals, which in turn aggravate the liver, potentially leading to damage.13 Comorbidities related to excessive drinking and a high-fat diet (HFD) will gradually promote steatohepatitis.14 Research and identification of the potential mechanisms and therapeutic targets of AFLD on the basis of NAFLD has become a hot spot.
Sterol-regulatory element binding protein 1 (SREBP1), a subtype of SREBP, is a nuclear transcription factor for lipid metabolism, and plays an important role in the synthesis of fatty acids and triglycerides.15,16 Alcohol stimulation can directly or indirectly upregulate SREBP1, increase lipid synthesis, and thereby promote lipid accumulation.17 SREBP1 is also closely related to NAFLD, and is an important target for the treatment of fatty liver. Peroxisome proliferation-activated receptor gamma (PPARγ) is one of the nuclear receptor superfamily of ligand-inducible transcription factors.18 Research has shown that following HFD feeding or chronic ethanol feeding, hepatic PPARγ was upregulated and activated in mice,19,20 but its upregulation and activation were attenuated by HFD plus acute ethanol binge.7 In addition, the pathogenesis of fatty liver is tightly related to anti-inflammatory effects.21 Pyroptosis is a means of programmed cell death accompanied by vigorous inflammatory response, and induces pathological inflammation of organs. After stimulation, pro-caspase-1 is cleaved to form the active cysteinyl aspartate specific proteinase-1 (caspase-1), which promotes the maturation and secretion of IL-1β, recruits inflammatory immune cells, and expands the inflammation reaction, thereby inducing a series of inflammatory reactions.22
Taxifolin (Pubchem CID: 439533, TAX, also known as dihydroquercetin) is a potent flavonoid found in onions,23 milk thistle,24 and Douglas fir.25 No significant toxic effects have been found in the use of TAX in large doses, and it possesses strong anti-inflammatory,21 hepatoprotective,26 anti-oxidation,27 anti-tumor,28 and other pharmacological effects. Theriault et al.29 reported that TAX could reduce the synthesis and storage of lipids in the liver by regulating the activity of enzymes, thereby affecting lipid metabolism. Also, our previous experimental results30 showed that in binge drinking both an alcoholic fatty liver model and acute alcoholic fatty liver model TAX could inhibit the accumulation of lipids in alcoholic fatty livers, and showed a good improvement on inflammation. We previously reported brief research illustrating that TAX remarkably reversed severe acute hepatosteatosis caused by alcohol binge combined with a short-term HFD fed model.31 However, how TAX regulates chronic hepatoseatotitis with a mixed factor of obesity and alcohol binge is still elusive.
Therefore, our current experiment explored the detailed mechanism TAX on alcohol-stimulated non-alcoholic fatty liver in mice exposed to alcohol combined with long-term HFD feeding.
2. Materials and methods
2.1 Materials
TAX (PubChem CID: 439533; purity ≥99.00%) was purchased from Chengdu Push Bio-technology. Metformin (PubChem CID: 14219) was used as a positive control and purchased from Beyotime Institute of Biotechnology (Haiman, Jiangsu, China). Anti-P2X7R (ab48871), anti-SREBP1 (ab28481), anti-glyceraldehyde-3-phosphate dehydrogenase (GAPDH) (ab8245), anti-F4/80 (ab6640), anti-neutrophil elastase (NE, ab68672), and anti-PPARγ (ab19481) antibodies were purchased from Abcam (Cambridge, MA, USA). Anti-caspase-1 antibody (SC-514) was obtained from Santa Cruz Biotechnology, Inc. (Santa Cruz, CA, USA). Anti-IL-1β (AF401NA) antibody was purchased from R&D Systems (Minneapolis, MN, USA). Horseradish peroxidase (HRP)-conjugated goat anti-mouse IgG (Abcam), HRP-conjugated goat anti-rabbit IgG (Abcam), HRP-conjugated rabbit anti-goat IgG (Bioss, Beijing, China), Alexa Fluor 488 goat anti-rat IgG (Abcam), Alexa Fluor 594 Goat anti-Rabbit IgG (H + L) (ThermoFisher Scientific), and Alexa Fluor 488 conjugated goat anti-rabbit IgG (H + L) (ThermoFisher Scientific) were used as secondary antibodies for detection. The BCA Protein Assay Kit was obtained from Beyotime (Jiangsu, China).
2.2 Animal experimental protocols
Male ICR mice (8–10 weeks old, 18–22 g) were purchased from Yisi Laboratory Animal Technology (Changchun, Jilin, China). All the animals were raised in the animal room of Yanbian University. All the animals were raised in a 12/12 h light/dark environment with a consist temperature of 20–25 °C and humidity of 40%–70%. All the procedures throughout the animal experiments were performed in strict accordance with the Guide for the Care and Use of Laboratory Animals (National Research Council, 1996) and approved by the Animal Research Committee of Yanbian University with permission number 2017102610.
After one week of adaptation, the mice were randomly divided into nine groups (n = 9), as follows: normal, LFD (low-fat-diet fed) group, HFD only (high-fat-diet fed) group, HFD/ETOH (HFD-fed plus acute ethanol binge) group, HFD/ETOH + TAX (1, 5, and 25 mg per kg, body weight) group, TAX only (25 mg kg−1) group, and HFD/ETOH + metformin (300 mg per kg, body weight) group. All the mice were fed with a standard diet, low-fat diet (as pair-fed diet, 10% kcal% fat, catalog no. TP23522, Trophic Animal Feed High-tech Co., Ltd, Haian, Jiangsu, China), or high-fat diet (60% kcal% fat; catalog no. TP23520, Trophic Animal Feed High-tech Co., Ltd) for 12 weeks and on the last day of HFD feeding, the mice were given a single dose of ethanol (5 g per kg body weight as a 31.25% solution in water) according to the method to establish a HFD plus ethanol binge feeding model as described by Chang et al.32 From the 9th week, all the mice except the normal, LFD, HFD, and HFD plus ethanol groups were given the corresponding dose of TAX or metformin for a continuous 4 weeks. Nine hours after gavage of a single dose of ethanol, all the mice were sacrificed under anesthetization with isoflurane. Blood was collected by direct cardiac puncture. The mice livers were isolated, and a portion of the liver was immersed in 10% neutral formalin buffer for histological analysis, then the rest of the livers were frozen and stored in OCT, and the remaining tissues were stored at −80 °C until the subsequent analysis experiments.
2.3 Cell culture
The human hepatoma cell line HepG2 was kindly provided by Dr Jung Joon Lee (Korea Institute of Biological Sciences and Biotechnology, Daejeon, South Korea). HepG2 cells were cultured in DMEM medium containing 10% fetal bovine serum (FBS), 100 μg ml−1 streptomycin, and 100 units per ml penicillin, and in a 5% CO2 humidified incubator at 37 °C. HepG2 cells were exposed to different concentrations of TAX (25, 50, 100, and 200 μM) or metformin (500 μM) for 1 h and then exposed with oleic acid (OA, 2 mM) for 18 h or palmitic acid (PA, 500 μM) for 12 h to mimic the steatotic hepatocytes.
2.4 Liver histological analysis
Liver tissues were fixed with 10% neutral formalin buffer and then embedded in paraffin. Next, 5 μm-thick sections of paraffin-embedded liver tissues were stained with hematoxylin and eosin (H&E). Additionally, cryosections (5 μm) of the mice livers were stained with Oil Red O, and then counterstained with hematoxylin. All the analyses was carried out in a blinded manner.
2.5 Measurement of the biochemical parameters
Blood samples were centrifuged at 3000 rpm and 4 °C for 30 min to separate the serum, and then stored at −80 °C for the further experiments. The levels of serum alanine transferase (ALT), serum alanine acid transferase (AST), serum high density lipoprotein (HDL-C), serum low-density lipoprotein (LDL-C), serum total cholesterol, serum, and hepatic triglyceride (TG) were measured using enzymatic colorimetric kits (Nanjing Jiancheng Biotechnology Research Institute, Nanjing, China) according to the manufactures’ instructions.
2.6 Immunohistochemistry (IHC)
Paraffin sections were deparaffinized with xylene and dehydrated with gradient ethanol. The sections were immersed in 10 mM sodium citrate buffer (pH 6.0) and heated in a microwave for performing antigen retrieval. After cooling to room temperature, those sections were treated with 3% hydrogen peroxide and then blocked with 5% goat serum and avidin/biotin blocking solution (Vector Laboratories, Inc., Burlingame, CA, USA), and incubated with prediluted SREBP1 or NE primary antibodies, followed by incubating with appropriate secondary antibodies. The bound antibodies were observed with the Lab Vision™ DAB Plus (Thermo Fisher Scientific, Fremont, California, USA) substrate staining system, and images were acquired by light microscopy (Nikon TI-E, Nikon, Tokyo, Japan).
2.7 Immunofluorescence staining (IF)
Frozen sections were fixed in acetone/methanol (1
:
1) and incubated with 5% goat serum at room temperature to reduce non-specific binding, and then incubated with anti-F4/80, caspase-1 or PPARγ primary antibodies at 4 °C overnight. HepG2 cells were fixed with 4% paraformaldehyde, and then 0.1% Triton X-100 permeate was added at room temperature, then blocked with 10% goat serum at room temperature, and then the procedure followed the previous description. The fluorescence was observed with a Ti-E inverted microscope (Nikon, Tokyo, Japan) or FV10i confocal laser scanning microscope from Olympus. Immunofluorescence intensity was analyzed with Image Pro-Plus 6.0. All these checks were performed blindly.
2.8 RT-PCR
Total RNA was isolated from the liver or cells using the SV Total RNA Isolation System (LS1040, Promega, Madison, WI, USA) according to the manufacturer's instructions. Isolated RNA was reverse-transcribed into complementary DNA (cDNA). Also, RT-PCR was performed by using primers specific for the genes, as described in Table 1. Gapdh was used as the housekeeping gene. The reaction conditions comprised: 2 min at 95 °C, and then 35 cycles of 30 s at 95 °C, 30 s at 55 °C, and 1 min at 72 °C. The final extension was done at 72 °C for 10 min. The PCR products were resolved in 2% agarose gel, and ethidium bromide stained special bands were visualized under UV light and photographed.
Table 1 Primer sequences for RT-PCR
Gene |
Sense (5′ to 3′) |
Anti-sense (5′ to 3′) |
mIl1b
|
GTACATCAGCACCTCACAAG |
CACAGGCTCTCTTTGAACAG |
mGapdh
|
CTTGTGCAGTGCCAGCC |
GCCCAATACGGCCAAATCC |
hCaspase1
|
TGCAGGACAACCCAGCTATC |
AGTCATGTCCGAAGCAGTGAG |
hP2rX7
|
AGCGGAAAGAGCCTGTCATC |
GCAGGATGTTTCTCGTGGTG |
hGapdh
|
GGCTCTCCAGAACATCATC |
CTCTTCCTCTTGTGCTCTTG |
2.9 Oil red staining on HepG2 cells
After the HepG2 cells were treated, the medium was discarded, and the cells were fixed with 4% paraformaldehyde solution, then permeated with 0.1% Triton X-100 at room temperature, and then washed with 60% isopropanol, and stained with Oil red O solution. The cells were washed with distilled water to remove any unbound dye. Next, the cells were counterstained with hematoxylin. The results were examined using an FV10i confocal laser scanning microscope from Olympus (Tokyo, Japan).
2.10 Western blot analysis
Total protein was extracted from the livers or cells with RIPA lysis buffer (Beyotime) supplemented with Complete Protease Inhibitor Cocktail (Roche). Equal amounts of protein were separated by 8%–12% sodium dodecyl sulfate–polyacrylamide gel electrophoresis (SDS-PAGE), and then transferred to PVDF membranes. Also, the membranes were blocked with 5% skim milk in PBS containing 0.1% Tween20 for 1 h at room temperature, and then incubated with the specific primary antibody at 4 °C overnight, and then incubated with the HRP-conjugated secondary antibody for 1 h at room temperature. Finally, the Clarity™ ECL Western blotting substrate (Bio-Rad, Hercules, CA, USA) was used to visualize the target protein, and Bio-Rad Quantity One software was used for quantitative optical density determination.
2.11 Statistical analysis
All the data are expressed herein as the mean ± SD. Statistical analysis was performed using the GraphPad Prism 5.0 program (GraphPad Software Inc., San Diego, California, USA), and statistical significance was determined using one-way analysis of variance (ANOVA) and Tukey multiple comparisons. Statistical significance (P value) between the groups was less than 0.05.
3. Results
3.1 TAX attenuated the liver injury and lipid metabolism related parameter index in mice fed with HFD and alcohol binge
There was no remarkable difference in the liver function and lipid metabolism parameters between the normal group and LFD group, indicating that LFD feeding did not cause any side effects. To explore the synergy effect of HFD plus ETOH on liver injury, mice were fed with HFD, followed by gavage with a single dose of ethanol. As demonstrated in Fig. 1A and B, the mice in the HFD alone group and HFD plus ETOH group both gained weight. The weight of the mice gradually reduced when the mice were administrated with TAX. The morphology of a normal liver appeared dark red, and the surface was smooth and delicate. After HFD feeding plus ETOH binge, the liver was swollen, white, and showed an erosive trend. TAX administration relieved the morphological change of the liver in a dose-dependent manner (Fig. 1C). Serum and liver AST, ALT, LDL-C, TC, and TG levels were markedly elevated in mice livers from the HFD plus ETOH group, and then showed a clear recovery tendency contributed by the TAX treatment (Fig. 1D–I). The serum HDL-C level of the mice in the HFD plus ETOH group was markedly reduced, but elevated in the TAX-administered groups (HFD plus ETOH and TAX1, 5, 25) as shown in Fig. 1J. The serum level of IL-1β in the HFD plus ETOH group mice was increased, while TAX decreased the level of IL-1β (Fig. 1K).
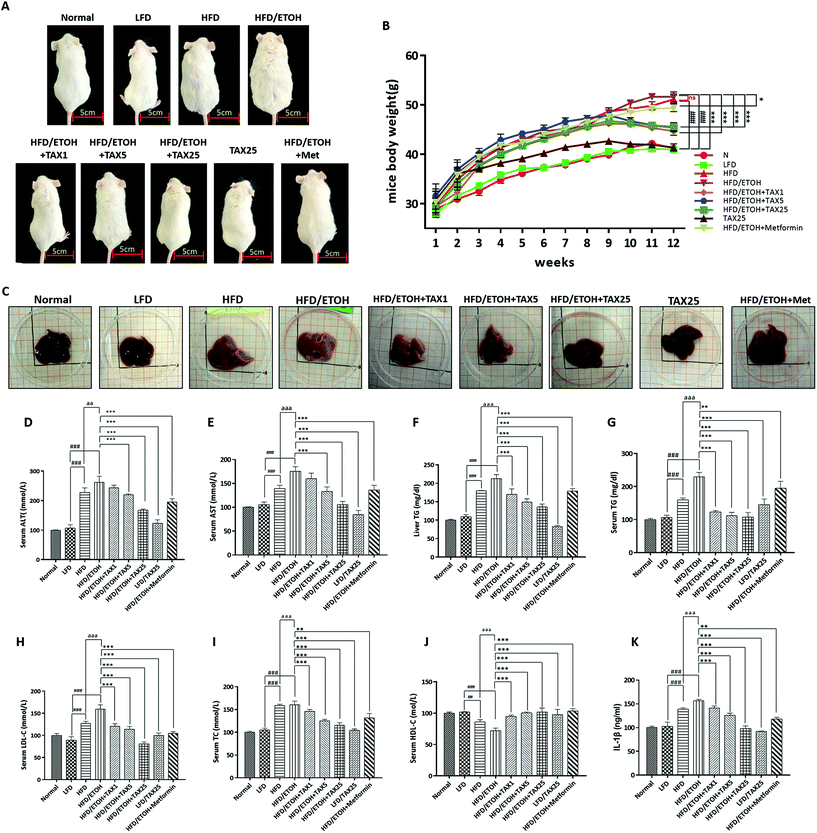 |
| Fig. 1 Effect of taxifolin on the mice morphology and biochemical indicators in mice serum and liver. (A) Morphological changes in the mice. (B) Body weight of the mice. (C) Morphological changes of the mice livers. (D) Serum ALT activity. (E) Serum AST activity. (F) Hepatic TG level. (G) Serum TG level. (H) Serum LDL-C level. (I) Serum TC level. (J) Serum HDL-C level. (K) IL-1β level in serum. Data are presented as the mean ± SD (n = 6). ##P < 0.01, ###P < 0.001, significantly different when compared with LFD-fed group; ** P < 0.01, *** P < 0.001, significantly different when compared with the HFD plus ETOH group; aaP < 0.01, aaaP < 0.001, significantly different when compared with the HFD-alone group. | |
3.2 TAX attenuated lipid accumulation and inflammation infiltration in the livers of the mice treated by HFD feeding plus acute ethanol binge
As shown in Fig. 2A and B, the liver histological changes in the HFD plus ETOH mice were examined by H&E and Oil Red O. Both HFD-alone feeding and HFD feeding plus ETOH binge caused obvious hepatic steatosis and lipid droplets, while there was no obvious change in the TAX alone group. A single dose of ethanol significantly exacerbated the degree of lipid accumulation caused by HFD. As expected, TAX treatment reduced hepatic lipid accumulation in the mice suffering from NAFLD and AFLD. The positive staining of SREBP1 around the central vein was elevated in the HFD-fed mice with or without ethanol binge stimulation (Fig. 2C). Also, a number of PPARγ positive-stained hepatocytes were found in the LFD-fed groups, while a HFD alone did not affect the intensity of PPAR in the mouse liver. However, with an extra dose of ethanol, the expression of PPARγ was remarkably decreased (Fig. 2D). TAX administration suppressed the SREBP1 level and restored the PPARγ level in the liver to almost a normal degree. We also examined the expression of NE, a marker of the activated neutrophil and F4/80, a marker of macrophages (Fig. 2E and F). Both NE and F4/80 were increased in mice livers with HFD feeding plus ETOH binge, while TAX treatment decreased the levels of NE and F4/80, indicating TAX notably blocked immune cells recruitment. Additionally, an extra dose of ethanol significantly improved the caspase-1 level in the HFD-fed mice (Fig. 2G), while TAX dose-dependently reduced the fluorescence intensity of caspase-1. Those data suggested that TAX exhibited an inhibitory effect on lipid accumulation and inflammation in a mixed hepatosteatotitis induced with long-term HFD feeding plus ETOH binge.
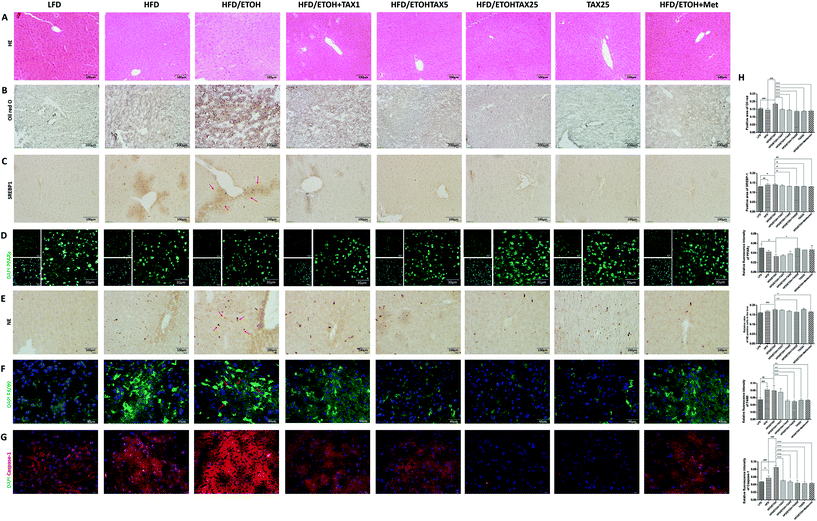 |
| Fig. 2 Taxifolin attenuated lipid accumulation and inflammation in the livers of obese mice exposed to alcohol. (A) HE staining (200× original magnification). (B) Oil Red O staining (100× original magnification). Immunohistochemical staining for SREBP1 (C, 200× original magnification) and NE (E, 400× original magnification). Immunofluorescence staining for PPARγ (D, green, 600× original magnification), F4/80 (F, green, 400× original magnification) and caspase-1 (G, red, 400× original magnification). Nuclei were stained with DAPI (blue). Representative images are shown. Red arrows indicate the positive staining areas. (G) Positive area of Oil Red O and SREBP1 staining, relative ratio of NE positive cells in the liver and relative fluorescence intensity of PPARγ, F4/80 and caspase-1 were analyzed with Image Pro-Plus 6.0. Data are presented as the mean ± SD (n = 5). #P < 0.05, ##P < 0.01, ###P < 0.001, significantly different when compared with the LFD-fed group; * P < 0.05, ** P < 0.01, *** P < 0.001, significantly different when compared with the HFD plus ETOH group; aP < 0.05, aaP < 0.01, aaaP < 0.001, significantly different when compared with the HFD-alone group. | |
3.3 TAX alleviated the inflammatory response in the livers of mice fed with HFD combined with alcohol binge by targeting pyroptosis
We next performed Western blot analysis to observe the expression of P2X7R and IL-1β in the mice livers. Compared with the LFD-fed group, the expressions of P2X7R and IL-1β in the HFD plus ETOH group were significantly increased, while TAX decreased the expressions of P2X7R and IL-1β (Fig. 3A–C). RT-PCR analysis of IL-1β in the HFD plus ETOH group was markedly increased compared with in the normal group, and it was improved after TAX (Fig. 3A and C). Combined with the data on caspase-1 (Fig. 2G), the results hinted that ethanol consumption more sensitively affected P2X7R-caspase-1-related pyroptotic inflammation on the basis of HFD-caused hepatosteatotitis, while TAX dose-dependently reduced the occurrence of pyroptosis in mice fed with HFD plus ETOH binge.
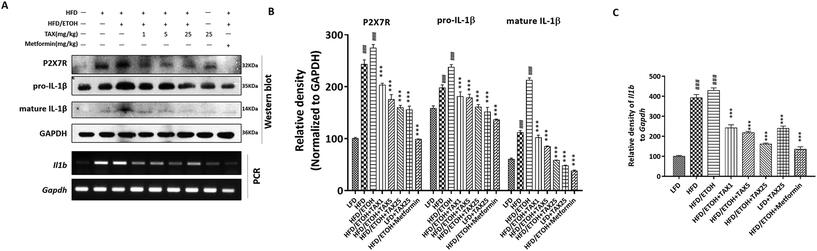 |
| Fig. 3 Taxifolin affected P2X7R and IL-1β expression in the livers of mice suffering from mixed hepatosteatotitis induced by a HFD plus ethanol binge. (A) Protein levels of P2X7R and IL-1β and mRNA levels of Il1b (encoding IL-1β) of the mice livers were determined by Western blot analysis and RT-PCR. (B and C) Each protein or mRNA expression was normalized to the GAPDH protein or Gapdh (encoding GAPDH) mRNA, respectively. Data are presented as the mean ± SD (n = 5). ###P < 0.001, significantly different when compared with the LFD-fed group; *** P < 0.001, significantly different when compared with the HFD plus ETOH group. | |
3.4 TAX suppressed lipid accumulation and caspase-1-related pyroptotic inflammation in steatotic HepG2 cells induced by oleic acid or palmitic acid
We applied Oil red O staining to demonstrate the changes in lipid content of HepG2 cells stimulated by OA or PA. The results showed that the untreated normal cells showed no obvious staining, but after PA or OA treatment, the Oil red O-stained area of the lipid deposition was significantly increased (Fig. 4A and 5A). The lipid accumulation induced by PA or OA gradually decreased with TAX pretreatment. We examined the effects of TAX on SREBP1 and caspase-1 by immunofluorescence staining on OA- or PA-stimulated HepG2 cells. The fluorescence intensity of SREBP1 was significantly enhanced by OA or PA stimulation, while TAX pretreatment decreased the fluorescence intensity of SREBP1 and caspase-1 (Fig. 4A and 5A). As the results of Western blot indicated, TAX also remarkably suppressed the protein expression of SREBP1 and P2X7R in OA- and PA-stimulated HepG2 cells (Fig. 4C and 5C). Also, the mRNA levels of P2rX7 and caspase-1 in HepG2 cells stimulated by OA or PA were determined. TAX inhibited the mRNA levels of caspase1 and P2rX7 in HepG2 cells stimulated by OA or PA, respectively (Fig. 4C and 5C), which was consistent with the in vivo results (Fig. 2G). The above results indicated that OA- or PA-stimulated lipid accumulation in hepatocytes was accompanied with caspase-1-dependent pyroptotic inflammation, and especially TAX effectively prevented pyroptosis occurring in steatotic hepatocytes, and eventually suppressed the lipid accumulation.
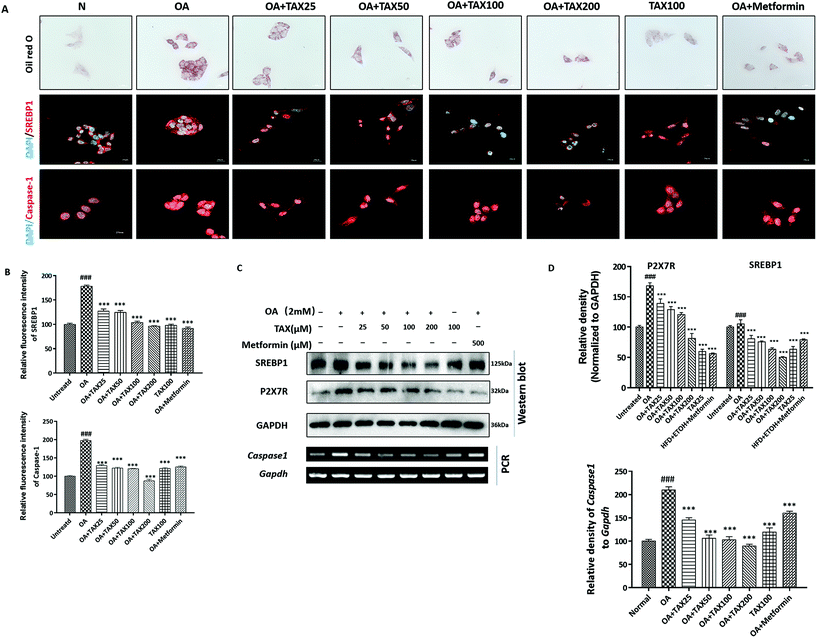 |
| Fig. 4 Taxifolin modulated lipid accumulation and inflammatory response in HepG2 cells stimulated by oleic acid. (A) Lipid droplets in the HepG2 cells were stained with Oil Red O (600× original magnification). Immunofluorescence staining for SREBP1 (red) and caspase-1 (red) in HepG2 cells (600× original magnification). Nuclei were stained with DAPI (blue). (B) Relative fluorescence intensity of SREBP1 and caspase-1 was analyzed with Image Pro-Plus 6.0. (C) Protein expression of P2X7R and SREBP1, and mRNA expression of caspase1 (encoding caspase-1) in HepG2 cells were determined by Western blot analysis and RT-PCR. (D) Each protein or mRNA expression was normalized to the GAPDH protein or Gapdh mRNA, respectively. Data are presented as the mean ± SD (n = 5). ###P < 0.001, significantly different when compared with untreated cells; *** P < 0.001, significantly different when compared with oleic acid-treated cells. | |
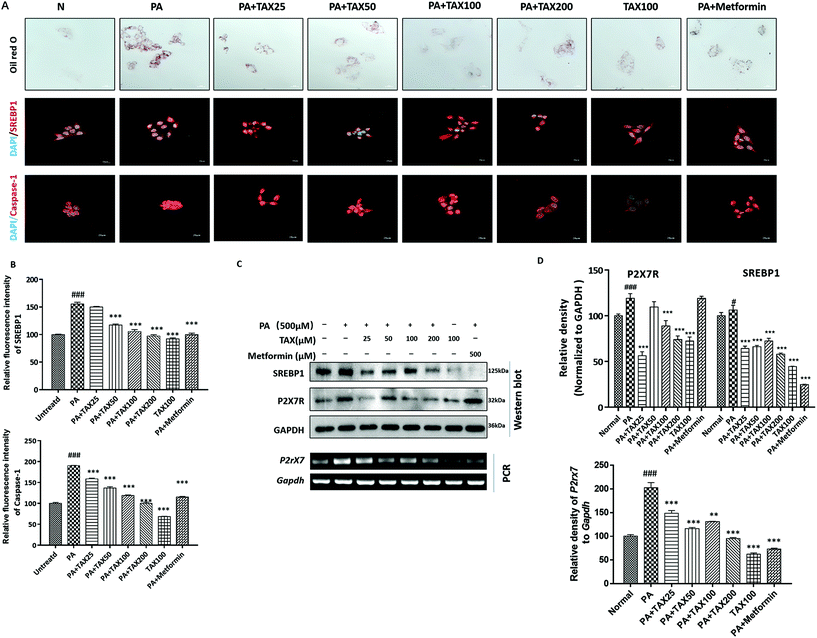 |
| Fig. 5 Taxifolin modulated lipid accumulation and inflammatory response in HepG2 cells stimulated by palmitic acid. (A) Lipid droplets in the HepG2 cells were stained with Oil Red O (600× original magnification). Immunofluorescence staining for SREBP1 (red) and caspase-1 (red) in HepG2 cells (600× original magnification). Nuclei were stained with DAPI (blue). (B) Relative fluorescence intensity of SREBP1 and caspase-1 was analyzed with Image Pro-Plus 6.0. (C) Protein expression of SREBP1 and P2X7R, and mRNA expression of P2rX7 (encoding P2X7R) in HepG2 cells were determined by Western blot analysis and RT-PCR. (D) Each protein or mRNA expression was normalized to the GAPDH protein or Gapdh mRNA, respectively. Data were presented as means ± SD (n = 5). #P < 0.05, ###P < 0.001, significantly different when compared with untreated cells; *** P < 0.001, significantly different when compared with palmitic acid-treated cells. | |
4. Discussion
It has been recognized for many years that many factors cause fatty liver, such as obesity, excessive amounts of alcohol consumption, metabolic disorders, drug effects, and malnutrition. Among those pathogenic factors, obesity and heavy drinking often coexist and work synergistically to promote the development and progression of alcoholic hepatosteatosis, alcoholic steatohepatitis, cirrhosis, and hepatocellular carcinoma.33–35 Drinking alcohol may be more likely to cause liver steatosis on the basis of obesity, while obesity exacerbates AFLD at all stages.36,37 To mimic the synergic effect of NAFLD and AFLD, we applied HFD feeding plus gavage of a single dose of ethanol to establish a mixed steatohepatitis, which causes much more severe steatohepatitis.38
Excessive alcohol drinking can aggravate the pathological process of steatosis and lipid metabolism disorders caused by NAFLD, and directly or indirectly regulate lipid metabolism-related transcription factors, such as SREBP1 and PPARγ, thereby increasing lipid synthesis and reducing fatty acid oxidation. Pathological features of bubble-like lipid droplets can be observed in the livers of mice fed with HFD combined with ethanol binge. Also, the comorbidities of NAFLD and AFLD also exhibited a typical inflammation infiltration, characterized by an increasing NE and F4/80, markers of activated neutrophil and macrophages, respectively. TAX remarkably alleviated SREBP1 and PPARγ-mediated steatosis and inflammation infiltration in the livers of obese mice exposed with alcohol binge.
In addition, TAX blocked the recruitment of inflammatory macrophages and neutrophils. NE is an inflammatory injury factor, a particularly prominent feature in animal models of HFD feeding plus acute ethanol binge stimulation with neutrophil infiltration in the liver, which is a mark of steatohepatitis and promotes various types of liver diseases.39–41 NE is released from activated neutrophils and accumulates at the inflammatory sites. NE regulates the formation of neutrophil extracellular traps (NETs) and these NETs are robustly deposited in liver sinusoids during systemic inflammation.38 Therefore, NE is considered as not merely a marker of the infiltration of neutrophil but also a marker of neutrophil activation. HFD and alcohol binge combination enhanced NE level in the mouse liver, indicating the inhibition of NE might prevent NET formation, and ultimately prevent inflammation induced by excessive alcohol and high fat diets. As expected, TAX notably suppressed NE levels in the livers of the mice. F4/80 is a marker of mature mouse macrophages, and during the maturation and activation of macrophages, the expression of F4/80 is significantly changed. Our data showed that TAX significantly reduced the expression of F4/80, indicating the alleviation of inflammatory infiltration.
Caspase-1 is produced after the activation of inflammasome, which not only can cut the precursor inflammatory factors, such as IL-1β, but also can directly induce programmed cell death. IL-1β is a cellular inflammatory factor that is significantly increased in alcoholic and non-alcoholic steatohepatitis.42,43 The pro-IL-1β is cleaved by caspase-1 to become mature-IL-1β via the activation of P2X7R-NLRP3 inflammation. We performed the detection of inflammatory factors, and the levels of P2X7R, caspase-1, and IL-1β in the liver of mice suffered by mixed hepatosteatotitis induced by alcohol and HFD were significantly increased. Interestingly, a single dose of alcohol notably increased the inflammatory factors in the obese mice fed with long-term HFD, but did not affect the lipid accumulation related factors, suggesting that targeting inflammation, especially pyroptotic inflammation, is a potent promising strategy for the therapy of the comorbidity of NAFLD and AFLD. As expected, TAX exhibited excellent inhibitory capacities on lipid accumulation, and particularly toward inflammation via caspase-1-mediated pyroptosis. We also applied an in vitro model of OA- or PA-stimulated HepG2 cells to induce lipid accumulation and inflammation. Using OA or PA to set up the model of steatotic hepatocytes is a classic method and is simple and easy to implement, and it well mimics the characteristics hepatocytes in human hepatosteatotitis. OA can cause the accumulation of triglycerides and induce excellent lipid accumulation,44 while PA has a strong apoptosis-stimulating effect.45 OA and PA lead to caspase-1-mediated pyroptosis in steatotic hepatocytes, accompanied with P2X7R activation. TAX expectedly restrained not merely lipid accumulation but also caspase-1 and P2X7R expression in steatotic hepatocytes, which was confirmed in vivo.
Collectively, TAX ameliorated steatohepatitis induced by long-term HFD feeding plus alcohol binge through the modulation of SREBP1 and PPARγ, targeting pyroptotic inflammation related IL-1β release and caspase-1 activation (Fig. 6).
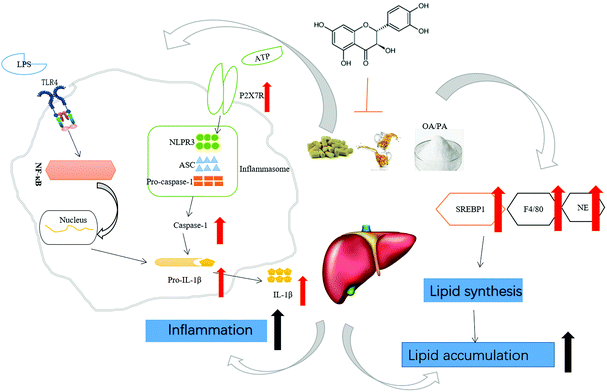 |
| Fig. 6 Underlying potential mechanism of the amelioration of HFD feeding plus acute ethanol binge-induced steatohepatitis by taxifolin. Taxifolin attenuated lipid accumulation and especially pyroptotic inflammation in steatohepatitis induced by long-term HFD feeding plus alcohol binge, suggesting a potent promising strategy for the therapy of the comorbidity of NAFLD and AFLD. | |
Author contributions
Ms. Zi-Ying Zhan and Ms. Mei Wu are the primary investigators in this study. Ms. Min Jiang, Ms. Yue Shang, Mr Yong-Ce Lin, Ms. Mei-Hua Piao and Ms. Rong-Hui Sun participated in part of in vivo experiments. Ms. Jian Liu, Ms. Chun-Ying Qiao, Ms. Huan Ye, Ms. Jing-Ya Jiao and Ms. Zhi-Hong Zhang participated in part of in vitro experiments. Prof. Yan-Ling Wu participated in part of statistical analysis. Prof. Li-Hua Lian and Prof. Ji-Xing Nan designed the whole study and wrote the manuscript.
Abbreviations
AFLD | Alcoholic fatty liver disease |
NAFLD | Non-alcoholic fatty liver disease |
SREBP1 | Sterol regulatory element binding protein-1 |
P2X7R | Purinergic receptor P2X ligand-gated ion channel 7 receptor |
IL-1β | Interleukin-1 beta |
Caspase-1 | Cysteinyl aspartate specific proteinase-1 |
PPARγ | Peroxisome proliferation–activated receptor gamma. |
Conflicts of interest
The authors declare that they have no conflict of interest.
Acknowledgements
This study was supported by a grant from the National Natural Science Foundation of China (82074095, 81960677 and 81660689), and partially by Science and Technology Planning Projects from the Science and Technology Department of Jilin Province (20180414048GH and 20180201065YY) and Science and Technology Planning Project of the Jilin Provincial Education Department (JJKH20191155KJ).
References
- J. B. Hoek, A. Cahill and J. G. Pastorino, Alcohol and mitochondria: a dysfunctional relationship, Gastroenterology, 2002, 122, 2049–2063 CrossRef CAS PubMed.
- A. J. Sanyal, C. Campbell-Sargent, F. Mirshahi, W. B. Rizzo, M. J. Contos, R. K. Sterling, V. A. Luketic, M. L. Shiffman and J. N. Clore, Nonalcoholic steatohepatitis: association of insulin resistance and mitochondrial abnormalities, Gastroenterology, 2001, 120, 1183–1192 CrossRef CAS PubMed.
- R. Blake and I. A. Trounce, Mitochondrial dysfunction and complications associated with diabetes, Biochim. Biophys. Acta, 2014, 1840, 1404–1412 CrossRef CAS PubMed.
- J. W. Chisholm, J. Hong, S. A. Mills and R. M. Lawn, The LXR ligand T0901317 induces severe lipogenesis in the db/db diabetic mouse, J. Lipid Res., 2003, 44, 2039–2048 CrossRef CAS PubMed.
- E. Han and Y. H. Lee, Non-Alcoholic Fatty Liver Disease: The Emerging Burden in Cardiometabolic and Renal Diseases, Diabetes Metab. J., 2017, 41, 430–437 CrossRef PubMed.
- R. Lakshman, R. Shah, K. Reyes-Gordillo and R. Varatharajalu, Synergy between NAFLD and AFLD and potential biomarkers, Clin. Res. Hepatol. Gastroenterol., 2015, S29–S34 CrossRef CAS PubMed.
- W. Wang, M. J. Xu, Y. Cai, Z. Zhou, H. Cao, P. Mukhopadhyay, P. Pacher, S. Zheng, F. J. Gonzalez and B. Gao, Inflammation is independent of steatosis in a murine model of steatohepatitis, Hepatology, 2017, 66, 108–123 CrossRef CAS PubMed.
- C. Gluud, S. S. Baker, R. D. Baker, W. Liu, N. J. Nowak and L. Zhu, Role of Alcohol Metabolism in Non-Alcoholic Steatohepatitis, PLoS One, 2010, 5, e9570 CrossRef PubMed.
- D. Issa, N. Alkhouri, C. Tsien, S. Shah, R. Lopez, A. McCullough and S. Dasarathy, Presence of sarcopenia (muscle wasting) in patients with nonalcoholic steatohepatitis, Hepatology, 2014, 60, 428–429 CrossRef PubMed.
- T. Karlas, J. Wiegand and T. Berg, Gastrointestinal complications of obesity: Non-alcoholic fatty liver disease (NAFLD) and its sequelae, Best Pract. Res., Clin. Endocrinol. Metab., 2013, 27, 195–208 CrossRef CAS PubMed.
-
M. Lazo and M. C. Mitchell, Epidemiology and Risk Factors for Alcoholic Liver Disease, Alcoholic and Non-Alcoholic Fatty Liver Disease, ed. N. Chalasani and G. Szabo, Springer, Cham, 2016, DOI:10.1007/978-3-319-20538-0_1.
- S. Zakhari, Bermuda Triangle for the liver: alcohol, obesity, and viral hepatitis, J. Gastroenterol. Hepatol., 2013, 28(Suppl 1), 18–25 CrossRef PubMed.
- M. A. Abdelmegeed, A. Banerjee, S. H. Yoo, S. Jang, F. J. Gonzalez and B. J. Song, Critical role of cytochrome P450 2E1 (CYP2E1) in the development of high fat-induced non-alcoholic steatohepatitis, J. Hepatol., 2012, 57, 860–866 CrossRef CAS PubMed.
- A. M. Diehl, Obesity and alcoholic liver disease, Alcohol, 2004, 34, 81–87 CrossRef CAS PubMed.
- L. J. Engelking, M. J. Cantoria, Y. Xu and G. Liang, Developmental and extrahepatic physiological functions of SREBP pathway genes in mice, Semin. Cell Dev. Biol., 2018, 81, 98–109 CrossRef CAS PubMed.
- J. D. Horton, J. L. Goldstein and M. S. Brown, SREBPs: activators of the complete program of cholesterol and fatty acid synthesis in the liver, J. Clin. Invest., 2002, 109, 1125–1131 CrossRef CAS.
- S. R. Commerford, L. Peng, J. J. Dube and R. M. O'Doherty, In vivo regulation of SREBP-1c in skeletal muscle: effects of nutritional status, glucose, insulin, and leptin, Am. J. Physiol.: Regul., Integr. Comp. Physiol., 2004, 287, R218–R227 CrossRef CAS PubMed.
- J. Berger and D. E. Moller, The mechanisms of action of PPARs, Annu. Rev. Med., 2002, 53, 409–435 CrossRef CAS PubMed.
- E. Moran-Salvador, M. Lopez-Parra, V. Garcia-Alonso, E. Titos, M. Martinez-Clemente, A. Gonzalez-Periz, C. Lopez-Vicario, Y. Barak, V. Arroyo and J. Claria, Role for PPARgamma in obesity-induced hepatic steatosis as determined by hepatocyte- and macrophage-specific conditional knockouts, FASEB J., 2011, 25, 2538–2550 CrossRef CAS PubMed.
- W. Zhang, Q. Sun, W. Zhong, Q. Sun, W. Zhong, X. Sun and Z. Zhou, Hepatic Peroxisome Proliferator–Activated Receptor Gamma Signaling Contributes to Alcohol–Induced Hepatic Steatosis and Inflammation in Mice, Alcohol Clin. Exp. Res., 2016, 40, 988–999 CrossRef CAS PubMed.
- M. Zhao, J. Chen, P. Zhu, M. Fujino, T. Takahara, S. Toyama, A. Tomita, L. Zhao, Z. Yang, M. Hei, L. Zhong, J. Zhuang, S. Kimura and X. K. Li, Dihydroquercetin (DHQ) ameliorated concanavalin A-induced mouse experimental fulminant hepatitis and enhanced HO-1 expression through MAPK/Nrf2 antioxidant pathway in RAW cells, Int. Immunopharmacol., 2015, 28, 938–944 CrossRef CAS PubMed.
- P. Broz, Immunology: Caspase target drives pyroptosis, Nature, 2015, 526, 642–643 CrossRef CAS PubMed.
- R. Slimestad, T. Fossen and I. M. Vågen, Onions: A Source of Unique Dietary Flavonoids, J. Agric. Food Chem., 2007, 55, 10067–10080 CrossRef CAS PubMed.
- S. N. Wallace, D. J. Carrier and E. C. Clausen, Batch solvent extraction of flavanolignans from milk thistle (Silybum marianum L. Gaertner), Phytochem Anal., 2010, 16, 7–16 CrossRef PubMed.
- A. E. Weidmann, Dihydroquercetin: More than just an impurity?, Eur. J. Pharmacol., 2012, 684, 19–26 CrossRef CAS PubMed.
- A. R. Tapas, D. M. Sakarkar and R. B. Kakde, Flavonoids as Nutraceuticals: A Review, Trop. J. Pharm. Res., 2008, 7, 1089–1099 Search PubMed.
- S. D. Marino, C. Festa, F. Zollo, A. Nini, L. Antenucci, G. Raimo and M. Iorizzi, Antioxidant Activity and Chemical Components as Potential Anticancer Agents in the Olive Leaf (Olea europaea L. cv Leccino.) Decoction, Anticancer Agents Med. Chem., 2014, 14, 1376–1385 CrossRef PubMed.
- Z. R. Zhang, M. A. Zaharna, N. M. K. Wong, S. K. Chiu and H. Y. Cheung, Taxifolin Enhances Andrographolide-Induced Mitotic Arrest and Apoptosis in Human Prostate Cancer Cells via Spindle Assembly Checkpoint Activation, PLoS One, 2013, 8, e54577 CrossRef CAS PubMed.
- A. Theriault, Q. Wang, S. C. V. Iderstine, B. Chen, A. A. Franke and K. Adeli, Modulation of hepatic lipoprotein synthesis and secretion by taxifolin, a plant flavonoid, J. Lipid Res., 2000, 41, 1969–1979 CrossRef CAS.
- Y. Zhang, Q. Jin, X. Li, J. Min, B. W. Cui, K. L. Xia, Y. L. Wu, L. H. Lian and J. X. Nan, Modulation of AMPK-dependent lipogenesis mediated by P2X7R-NLRP3 inflammasome activation contributes to the amelioration of alcoholic liver steatosis by dihydroquercetin, J. Agric. Food Chem., 2018, 16, 4862–4871 CrossRef PubMed.
- M. Wu, C. Y. Qiao, H. Ye, Y. L. Wu, J. X. Nan and L. H. Lian, Dihydroquercetin inhibits mouse hepatic steatosis induced by high fat diet feeding plus acute ethanol binge, Chin. Pharmacol. Bull., 2020,(01), 147–148 Search PubMed.
- B. Chang, M. J. Xu, Z. Zhou, Y. Cai, M. Li, W. Wang, D. Feng, A. Bertola, H. Wang and B. Gao, Short- or long-term high-fat diet feeding plus acute ethanol binge synergistically induce acute liver injury in mice: An important role for CXCL1, Hepatology, 2015, 62, 1070–1085 CrossRef CAS PubMed.
- H. Iturriaga, D. Bunout, S. Hirsch and G. Ugarte, Overweight as a risk factor or a predictive sign of histological liver damage in alcoholics, Am. J. Clin. Nutr., 1988, 47, 235–238 CrossRef CAS PubMed.
- S. Naveau, A. S. Dobrin, A. Balian, M. Njike-Nakseu, P. Nohra, A. Asnacios, S. Prevot and G. Perlemuter, Body fat distribution and risk factors for fibrosis in patients with alcoholic liver disease, Alcohol.: Clin. Exp. Res., 2013, 37, 332–338 CrossRef CAS PubMed.
- S. Naveau, V. Giraud, E. Borotto, A. Aubert, F. Capron and J. C. Chaput, Excess weight risk factor for alcoholic liver disease, Hepatology, 1997, 25, 108–111 CrossRef CAS PubMed.
- R. Parker, S. J. Kim, G. Y. Im, J. Nahas, B. Dhesi, N. Vergis, A. Sinha, A. Ghezzi, M. R. Rink, A. McCune, G. P. Aithal, P. N. Newsome, C. J. Weston, A. Holt and B. Gao, Obesity in acute alcoholic hepatitis increases morbidity and mortality, EBioMedicine, 2019, 45, 511–518 CrossRef PubMed.
- M. Thiele, V. Rausch, G. Fluhr, M. Kjaergaard, F. Piecha, J. Mueller, B. K. Straub, M. Lupsor-Platon, V. De-Ledinghen, H. K. Seitz, S. Detlefsen, B. Madsen, A. Krag and S. Mueller, Controlled attenuation parameter and alcoholic hepatic steatosis: Diagnostic accuracy and role of alcohol detoxification, J. Hepatol., 2018, 68, 1025–1032 CrossRef CAS PubMed.
- I. Cichon, W. Ortmann, A. Bednarz, M. Lenartowicz and E. Kolaczkowska, Reduced Neutrophil Extracellular Trap (NET) Formation During Systemic Inflammation in Mice With Menkes Disease and Wilson Disease: Copper Requirement for NET Release, Front Immunol., 2019, 10, 3021–3021 CrossRef CAS PubMed.
- R. Xu, H. Huang, Z. Zheng and F.-S. Wang, The role of neutrophils in the development of liver diseases, Cell Mol. Immunol., 2014, 011, 224–231 CrossRef CAS PubMed.
- A. Bertola, O. Park and B. Gao, Chronic plus binge ethanol feeding synergistically induces neutrophil infiltration and liver injury in mice: a critical role for E-selectin, Hepatology, 2013, 58, 1814–1823 CrossRef CAS PubMed.
- B. Gao and H. Tsukamoto, Inflammation in Alcoholic and Nonalcoholic Fatty Liver Disease: Friend or Foe?, Gastroenterology, 2016, 150, 1704–1709 CrossRef PubMed.
- J. Petrasek, S. Bala, T. Csak, D. Lippai, K. Kodys, V. Menashy, M. Barrieau, S. Y. Min, E. A. Kurt-Jones and G. Szabo, IL-1 receptor antagonist ameliorates inflammasome-dependent alcoholic steatohepatitis in mice, J. Clin. Invest., 2012, 122, 3476–3489 CrossRef CAS PubMed.
- K. A. Negrin, R. J. R. Flach, M. T. DiStefano, A. Matevossian, R. H. Friedline, D. Y. Jung, J. K. Kim and M. P. Czech, IL-1 signaling in obesity-induced hepatic lipogenesis and steatosis, PLoS One, 2014, 9, e107265 CrossRef PubMed.
- M. Cnop, J. C. Hannaert, A. Hoorens, D. L. Eizirik and D. G. Pipeleers, Inverse relationship between cytotoxicity of free fatty acids in pancreatic islet cells and cellular triglyceride accumulation, Diabetes, 2001, 50, 1771–1777 CrossRef CAS PubMed.
- M. Ricchi, M. R. Odoardi, L. Carulli, C. Anzivino, S. Ballestri, A. Pinetti, L. I. Fantoni, F. Marra, M. Bertolotti, S. Banni, A. Lonardo, N. Carulli and P. Loria, Differential effect of oleic and palmitic acid on lipid accumulation and apoptosis in cultured hepatocytes, J. Gastroenterol. Hepatol., 2009, 24, 830–840 CrossRef CAS PubMed.
Footnote |
† These authors contributed equally to this work. |
|
This journal is © The Royal Society of Chemistry 2021 |
Click here to see how this site uses Cookies. View our privacy policy here.