DOI:
10.1039/D0FO02107E
(Paper)
Food Funct., 2021,
12, 387-396
Cyanidin-3-O-glucoside represses tumor growth and invasion in vivo by suppressing autophagy via inhibition of the JNK signaling pathways†
Received
10th August 2020
, Accepted 26th November 2020
First published on 26th November 2020
Abstract
Black bean seed coat extract (BBSCE) contains a high amount of bioactive compounds which can reduce the risk of cancers, but the underlying mechanism remains poorly understood in vivo. Here using a Drosophila model of a malignant tumor, wherein the activated oncogene Raf (RafGOF) cooperates with loss-of-function mutations in the conserved tumor suppressor scribble (scrib−/−), we investigated the antitumor mechanism of BBSCE and its main active component cyanidin-3-O-glucoside (C3G) in vivo. The results showed that supplementation of either BBSCE or C3G inhibited the tumor growth and invasion of RafGOFscrib−/− and extended their survival in a dose dependent manner. Strikingly, the activation of both autonomous and non-autonomous autophagy in tumor flies was significantly reduced by C3G treatment. A further study indicated that C3G exhibited an antitumor effect in vivo by blocking autophagy both in tumor cells and in its microenvironment by inhibiting the JNK pathway. Interestingly, the efficacy of chloroquine (CQ, an autophagy inhibitor used as an antitumor agent) combined with C3G is much better than either C3G or CQ treatment alone. C3G may be combined with CQ to treat cancers and to provide a theoretical basis for functional food or natural medicine development.
Introduction
Black bean (Phaseolus vulgaris L.) has been a common herbal material in oriental medicine for hundreds of years.1,2 Black bean seed coat extract (BBSCE) contains a high concentration of phenolic compounds, which exhibit numerous biological activities, such as eliminating free radicals, inhibiting oxidation and the growth of microbes, and reducing the risks of cardio- and cerebrovascular diseases and several types of cancers in vitro and in vivo.3–5 The primary phenolic compounds of BBSCE are flavonoids, predominantly anthocyanins and proanthocyanidins.6 Anthocyanins play a crucial role in cancer prevention and therapy because of their extensive sources, low cytotoxicity and safe consumption.7–9 Further studies showed that cyanidin-3-O-glucoside (C3G) accounted for 76.6% of anthocyanins in BBSCE.10 C3G is functional in multiple cancer cells and exerts antitumor activities.2 For example, C3G could induce cell cycle arrest and apoptosis of tumor cells in vitro by targeting the signaling pathways, such as JNK, PI3K/Akt, VEGF and so on.8,11,12 Tumorigenesis is a complicated process. Growing evidence suggests that the tumor microenvironment is a critical regulator of tumorigenesis, regulating tumor growth and invasion.13 The molecular mechanisms of the anticancer effect of C3G on cancer cells and a xenograft animal model have been previously explored,14,15 but the effect of C3G on the tumor microenvironment in vivo has been neglected.
Autophagy, a self-protection mechanism of cells, degrades the damaged organelles and maintains energy homeostasis.16 Recent studies have shown that autophagy in the tumor microenvironment promotes tumor growth by supplying nutrients and other factors.17 Anthocyanins have been shown to have antitumor properties including an impact on autophagy regulation in tumor development,18,19 but few studies have focused on the interaction between anthocyanins and the tumor microenvironment.
The fruit fly Drosophila melanogaster has been established as a versatile model organism in experimental food and nutrition research in the past few decades.20 Numerous studies investigate the effects of dietary factors on life span, anti-aging, reproduction, and development in Drosophila.21,22 Moreover, Drosophila is a critical model organism to explore the underlying mechanism of interaction between tumor cells and the microenvironment.23,24 It is well known that the distinguished Ras-Raf signaling pathway was first elucidated in Drosophila.25 The activation of this pathway is associated with 30% of human cancers.26 The activated oncogene Raf (RafGOF) cooperates with loss-of-function mutations in the conserved tumor suppressor scrib giving rise to metastatic tumors that show many behaviors observed in human cancers.27 In addition, the JNK, JAK/STAT, Wnt and Notch pathways are highly conserved from fly to human.23 All these make Drosophila an ideal model to study the interaction between active components and tumor progression.
In this study, we used a Drosophila malignant RafGOFscrib−/− model to explore the antitumor effect of BBSCE and its active monomer C3G in vivo. The results suggest that C3G supplementation can alleviate autonomous and non-autonomous autophagy in tumor flies. Furthermore, the relevant signaling pathway was investigated. Our study is of great significance for C3G development and application and this may improve the medicinal and nutritional value of C3G.
Materials and methods
Reagents
The following flies were used: w1118 (V#60000) was obtained from the Vienna Drosophila RNAi Center. Lamp1.CPIT-001775 (Lamp trapped YFP) (115517) was obtained from the Drosophila Genetics Resource Center. y,w,ey-flp; Act > y+ > GAL4 UAS-GFP; FRT82B tub-GAL80,28 w; Adv/Cyo; UAS-RafGOF FRT82B scrib1/TM6B;29 and Atg8a-mCherry30 were generously provided by Dr Jose C. Pastor-Pareja. BBSCE (containing at least 30% anthocyanins) was purchased from JF-Natural Company Limited (China). C3G (CAS: 7084-24-4) was purchased from Vicky biotechnology co. Ltd (China). Chloroquine (CQ) (Cat#6628) was purchased from Sigma-Aldrich Corporation (St Louis, MO).
Fly husbandry
Stocks and crosses were under normal conditions at 25 °C and 60% humidity with a 12 h light
:
12 h dark cycle and were reared on various media in this experiment. All fly crosses and larvae were maintained in vials containing traditional corn-yeast food or drug containing food. The corn-yeast standard food was prepared according to the traditional corn-yeast medium (100 g of corn, 10 g of soybean meal, 40 g of brown sugar, 14.5 g of sugar, 25 g of yeast, and 8.0 g of agar mixed with hot water to prepare 1000 mL of diet). BBSCE, C3G and CQ supplemented diets were dissolved in the standard medium. Drugs were added to standard food to obtain the following final concentrations: 2 mg mL−1 or 10 mg mL−1 BBSCE, 0.1 mg mL−1 or 0.4 mg mL−1 C3G, 0.5 mg mL−1 or 2.0 mg mL−1 CQ, and C3G and CQ simultaneously (0.4 mg mL−1 C3G + 0.5 mg mL−1 CQ). The concentration of CQ used in vivo was slightly adjusted according to Katheder's research.30 Crosses were flipped directly onto the drug-containing medium. After 3 days of mating, the parents were transferred to new vials in which they laid eggs.
Survival assays
To investigate the effect of BBSCE or C3G on the survival of tumor flies, the parents were reared on normal food, BBSCE containing food (2 mg mL−1 or 10 mg mL−1 BBSCE) and C3G containing food (0.1 mg mL−1 or 0.4 mg mL−1 C3G). Newly hatched larvae were collected and transferred to normal, BBSCE containing and C3G containing food vials, respectively. The density of each vial was controlled to 50 larvae. Food vials were changed every 2 days, and dead flies were counted at that time. Assays were performed with six replicates for each group. After 15 days, the number of dead individuals in the larval stage (N1) or in the pupal stage (N2), or eclosed tumor flies (N3) were recorded and the mortality rate at different stages and the eclosion rate were calculated using the following formulas: larval rate (%) = 100 × N1/50; pupal rate (%) = 100 × N2/50; and eclosion rate (%) = 100 × N3/50.
Eye defect analysis
To phenotype eye defects, flies were collected after eclosion at 25 °C and frozen to death at −80 °C overnight.31 Eyes were photographed using a Zeiss imager A1 stereomicroscope. More than six flies were scored per genotype, and each experiment was repeated three times.
Immunohistochemistry
The third-instar larvae were dissected in cold PBS, the dissected tissues were fixed in 4% paraformaldehyde in PBS for 10 min at room temperature, stained, and mounted following standard procedures.32 The following antibodies and dyes were used: mouse anti-MMP1 (1
:
100 Developmental Studies Hybridoma Bank, DSHB) and rabbit anti-phosphorylated c-Jun (pJNK) (1
:
200 Calbiochem, San Diego). The fluorescein-conjugated secondary antibodies used Cy3-conjugated goat anti-rat IgG (1
:
500 for pJNK) and anti-mouse IgG (1
:
500 for MMP1). Slices were mounted with 50% glycerol/PBS. Images were obtained using a Nikon ECLIPES Ti2-U microscope (Nikon, Tokyo, Japan).
Fluorescence microscopy
The whole bodies of larvae were placed on a microscope slide and observed with a Nikon ECLIPES Ti2-U microscope (Nikon, Tokyo, Japan). The whole bodies of tumor larvae were observed at 12 days after egg laying (AEL) while the whole bodies of Atg8a-mcherry larvae and Lamp1-YFP larvae were examined at the wandering stage.
For the dissected tumors, the eye imaginal discs and cephalic complexes, including eye imaginal discs, brain lobes and ventral nerve cord (VNC), were dissected in cold PBS within 20 min, fixed in 4% (vol/vol) paraformaldehyde for 10 min, washed once with PBS, and imaged immediately at day 7 AEL and at day 12 AEL, respectively. ImageJ was used to quantify the size and fluorescence intensity of tumors. Quantification of the data was presented in bar graphs created with GraphPad Prism 5. Three independent replications were carried out.
The autophagy was observed in the eye imaginal discs, cephalic complexes and other tissues of tumor larvae (Atg8a-mcherry) and guts of Atg8a-mcherry larvae and Lamp1-YFP larvae with a Nikon ECLIPES Ti2-U microscope (Nikon, Tokyo, Japan). More than 6 samples were tested each time, and the experiments were repeated at least three times.
Western blotting
Western blotting was carried out according to standard procedures.32 In total, 20–30 cephalic complexes were collected on ice in PBS and extracted with PBST containing protease inhibitors. The protein concentration was measured using a BCA protein assay kit (thermal). All experiments were repeated at least three times. The following primary antibodies were used: rabbit anti-pJNK (1
:
2000, Calbiochem) and rabbit anti-GABARAP + GABARAPL1 + GABARAPL2 (Atg8-family proteins, 1
:
2000, Abcam). A HRP-conjugated goat anti-rabbit IgG (Zhongshan Goldenbridge Biotechnology, China) was used as secondary antibody.
Statistical analysis
Data were analyzed by using Student's t-test to compare between groups and one way analysis of variance (ANOVA) for multiple groups. The statistical results were presented as mean ± SEM. Asterisks indicate the critical levels of significance (* P < 0.05, ** P < 0.01, and *** P < 0.001).
Results
BBSCE inhibits tumor growth and invasion in vivo
The survival rate of tumor flies reared on different doses of BBSCE was determined (Fig. 1). About 66% of the tumor flies died at the larval stage under normal conditions, while 34% of them died at the pupal stage, and thus no adult could eclose. Low dose BBSCE (2 mg mL−1) slightly reduced the proportion of flies that died at the larval stage from 65% to 60%, whereas high dose BBSCE (10 mg mL−1) showed a strong reduction in the proportion (down 28%). Magically, tumor flies reared on high dose BBSCE can survive to adults (about 4%). These data suggested that BBSCE treatment prolonged the survival of tumor flies in a dose dependent manner.
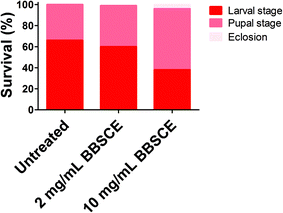 |
| Fig. 1 BBSCE (10 mg mL−1) prolonged survival of tumor flies. No significant difference in survival of tumor flies was found between control and low dose BBSCE (2 mg mL−1) group. | |
The imaginal eye discs of the Drosophila malignant RafGOFscrib−/− model have been used to explore the mechanism of tumor growth and invasion and screen functional substances.33 Herein, the imaginal eye discs carrying hyperplastic tumors were chosen to illustrate BBSCE's effect. As shown in Fig. 2A and B, BBSCE supplementation (10 mg mL−1) dramatically improved the hyperproliferated tumors in the eye-antennal imaginal discs at 7 or 12 days AEL. The BBSCE group showed a reduction of 26% at 7 days AEL (P < 0.05) and 42% at 12 days AEL (P < 0.001) in the tumor size (Fig. 2C). Besides, the fluorescence intensity of tumor tissues reflected the number of tumors. Compared with the control, the BBSCE group showed a significant reduction in the number of tumors at both 7 days AEL (down 66%, P < 0.001) and 12 days AEL (down 36%, P < 0.01) (Fig. 2D). These results suggest that BBSCE restrains tumor growth in vivo.
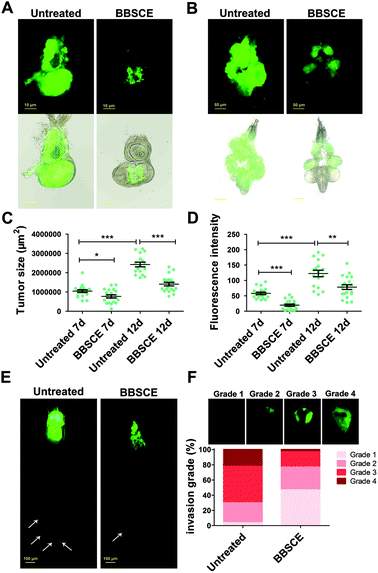 |
| Fig. 2 BBSCE (10 mg mL−1) inhibited tumor growth and invasion in Drosophila. (A and B) When flies were reared on BBSCE, tumor growth was strongly inhibited both at 7 days AEL (A) and 12 days AEL (B). (C and D) Quantification of tumor size and intensity showed that malignant flies reared on BBSCE media led to a reduction in tumor growth and number (12 days AEL; n ≥ 15). (E) BBSCE repressed the process of tumor migration. (F) The invasive behavior in tumor flies can be reduced by BBSCE supplementation (12 days AEL; n ≥ 50). Differences with *P < 0.05, **P < 0.01, or ***P < 0.001 were considered significant. | |
Tumor metastasis is the major cause of cancer mortality.34 Whole-body animal models may provide important evidence for clinical cancer treatment.35 To assess the effect of BBSCE on tumor metastasis, we observed the whole body of tumor flies at 12 days AEL using a fluorescence microscope. The results showed that tumor cells proliferated in the eye-antennal discs of the malignant RafGOFscrib−/− flies and migrated to several places, such as hemolymph, fat body and gut (white arrowheads) (Fig. 2E). The malignant phenotypes were strongly restrained by BBSCE treatment (Fig. 2E). Furthermore, based on the degree of tumor cell invasion into the VNC, we classified invasion into four grades. We found that the degree of invasion into the VNC of flies was strongly reduced when they were reared on BBSCE. The number of tumor flies with severe invasion (grade 4) showed a 19% decrease, while the number of flies with no invasion (grade 1) increased by 43% (Fig. 2F). Taken together, BBSCE inhibits tumor growth, invasion and distant migration, and prolongs the survival of malignant tumor flies. The inhibitory effect of BBSCE on tumor growth has also been found in carcinogen-treated F344 rats and human colon cancer Caco-2 cells.9,36 These findings suggested that the Drosophila malignant tumor model is suitable for use in functional factor studies as it shows many similarities with mammalian species.
C3G inhibits tumor growth and invasion in vivo
BBSCE shows a complex composition. To further illustrate the correlation between its components and tumor progression, purified C3G, one of the most abundant active components in anthocyanins of BBSCE,37 was used to investigate the effect on tumor development. The proportion of tumor flies dead at the larval stage fell 8% and 24% under low (0.1 mg mL−1) and high dose C3G (0.4 mg mL−1), respectively. The eclosion rate of tumor flies reared on C3G was 9% in the low dose group and 19% in the high dose group, whereas no fly could eclose under normal conditions (Fig. 3A). The eyes of the eclosed flies appeared wrinkled and necrotic (Fig. 3B). Obviously, tumor overgrowth in RafGOFscrib−/− flies was significantly suppressed when treated with high dose C3G, but was only partially improved upon low dose supplementation (Fig. 3C, 4A and B). These data indicated that C3G represses tumor progression in a dose dependent manner. The two doses of C3G did not affect the growth and development of normal flies (w1118) (data not shown). Statistical analysis indicated that C3G (0.4 mg mL−1) showed a significant attenuation in the tumor size at both 7 days (down 22%, P < 0.01) and 12 days (down 22%, P < 0.01), compared with the control group (Fig. 4C). The number of tumor cells (the value of the fluorescence intensity) dropped by 45% at 7 days (P < 0.001) and 41% at 12 days (P < 0.01) (Fig. 4D). These data suggest that C3G inhibits tumor growth in Drosophila.
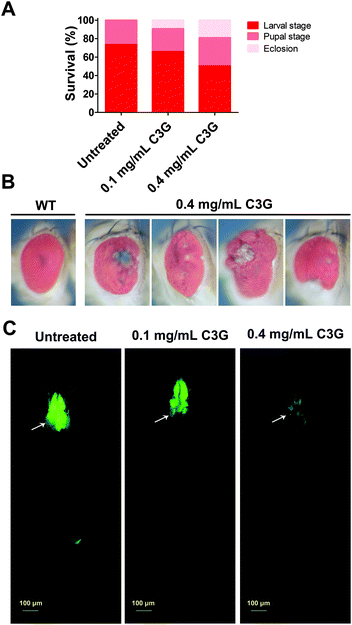 |
| Fig. 3 C3G prolonged the survival of tumor flies and repressed tumor growth. (A) Compared with the normal diet, C3G prolonged their survival in a dose dependent manner. (B) Eyes of eclosed tumor flies that were reared on C3G appeared wrinkled and necrotic. WT showed eyes of wild type flies. (C) C3G inhibited tumor growth and metastasis in a dose dependent manner. | |
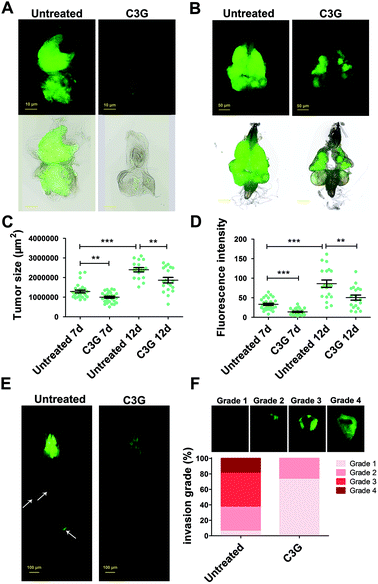 |
| Fig. 4 C3G inhibited tumor growth and invasion in Drosophila. (A and B) Oral administration of 0.4 mg mL−1 C3G led to a reduction in tumor growth either at 7 days AEL (A) or 12 days AEL (B). (C and D) Quantification of tumor size and number showed that malignant flies reared on C3G media led to a reduction in the tumor area and intensity (12 days AEL; n ≥ 15). (E) C3G inhibited the process of tumor migration. (F) The invasive behavior in tumor flies can be reduced when they were reared on C3G media (12 days AEL; n ≥ 50). Differences with *P < 0.05, **P < 0.01, or ***P < 0.001 were considered significant. | |
We then investigated the effects of C3G on tumor metastasis. Similar to BBSCE, C3G supplementation reduced the number of tumor cells metastasized into other tissues (Fig. 4E). Furthermore, the proportion of severe (grade 4) and moderate (grade 3) invasion into the VNC of flies was vanishingly low upon 0.4 mg mL−1 C3G supplementation, and most of the VNC were not invaded by tumor cells (grade 1, about 75%) (Fig. 4F). The inhibitory effect of C3G on invasive behaviors was stronger than that of the BBSCE group (Fig. 2F and 4F). Taken together, C3G inhibits tumor growth, invasion and distant migration in vivo and prolongs the survival of tumor flies.
C3G inhibits the JNK-mediated MMP1 pathway in malignant flies
Recent studies have revealed that JNK signaling is necessary for tumorigenesis and tumor invasion.38 The absence of the JNK activity completely abolished these malignant phenotypes.28 To investigate whether JNK signaling participates in C3G's antitumor mechanism, we examined the level of pJNK as an indicator of JNK activation.39 Compared with the control, C3G supplementation can reduce the pJNK level in the eye imaginal discs and cephalic complexes (Fig. 5A and Fig. S1A†). Together, C3G reduced the activity of JNK in tumor flies.
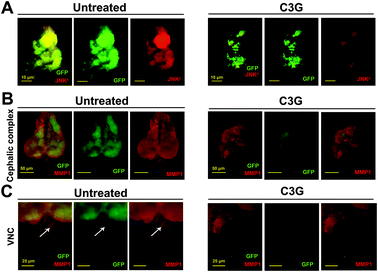 |
| Fig. 5 The JNK signaling pathway was responsible for the inhibitory effect of C3G on tumor growth and invasion. (A) Compared with normal conditions, C3G can reduce the expression of phosphorylated JNK at 7 days AEL. (B and C) Tumor flies induced massive growth and invasion into the VNC as indicated by MMP1 staining in both the cephalic complex (B) and VNC (C), which were strongly repressed by C3G supplementation (0.4 mg mL−1). | |
MMP1 serves as a transcriptional target of JNK signaling in the tumor invasion process.40 As shown in Fig. 5B and C, tumor cells activated massive MMP1 expression and migrated to the VNC at 12 days AEL. Obviously, tumor flies produced a low expression of MMP1 when reared on C3G media and no GFP-positive cells were observed in the VNC when compared to flies reared under normal conditions. These data suggested that C3G could inhibit tumor invasion by reducing the MMP1 activity. Taken together, these findings indicated that C3G may impede the invasive behavior of malignant flies by inhibiting the JNK pathway.
C3G restrains tumor progression by reducing the autonomous and non-autonomous autophagic activities
As reported earlier, the JNK-dependent autophagy pathway is required for tumor progression.30 In order to ascertain whether C3G participates in the regulation of autophagy in tumor progression, the GFP-labelled tumor cells carrying the autophagosome marker Atg8a tagged with mCherry (mChAtg8a)30 were used to assess the effects of C3G on autophagy in vivo. Interestingly, the particles of mChAtg8a accumulated in tumor cells and the surrounding normal cells of the eye-antennal discs at 7 days AEL in such a way that malignant clones induced autonomous autophagy (merged yellow signal, black arrowheads) and non-autonomous autophagy (red signal, white arrowheads) (Fig. 6A). C3G can break the accumulated mChAtg8a particles in both autonomous tumor cells and non-autonomous normal cells (Fig. 6A). About 12 days AEL, the puncta of autophagosomes were further enhanced in the cephalic complex, and along with GFP-labelled tumor clones invaded the VNC under normal conditions (Fig. 6B), whereas C3G supplementation dramatically attenuated the autophagosomes (Fig. 6C). To further investigate the effects of C3G on the autophagy level, the Atg8a level was assessed by using the western blot assay. Compared with the normal diet, C3G significantly reduced Atg8a expression in tumors (Fig. S1B†), which was consistent with the above results. These data suggested that C3G blocks the activation of autophagy in tumor flies. Interestingly, we found that C3G did not affect the autophagy levels of the whole body and gut of normal flies, as evidenced by two autophagic markers, the early autophagosome marker Atg8a and the late autolysosome marker Lamp141 (Fig. S2†). This suggests that C3G does not disturb autophagy homeostasis in normal organisms.
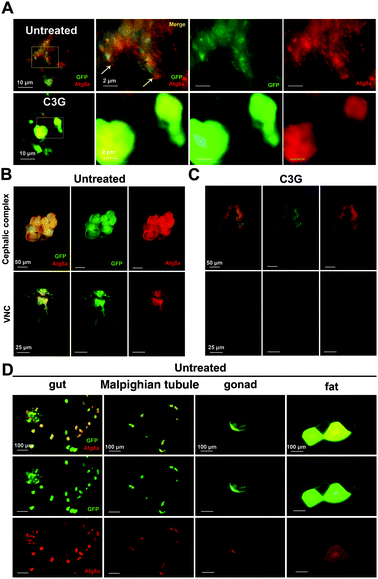 |
| Fig. 6 C3G suppressed autophagy in tumor flies. (A) C3G can inhibit autonomous and non-autonomous autophagy in tumor files. (B and C) Activation of autophagy in the VNC of flies can be reduced when they were reared on C3G media. (D) Tumor cells metastasized to distant organs, such as gut, Malpighian tubule, gonad and fat body. | |
Studies have shown that peripheral organs also responded to eye-antennal specific tumors.42 We found that eye disc-specific tumor cells migrated to distant organs, such as gut, Malpighian tubule, gonad and fat body, and that autophagosomes always accompanied them (Fig. 6D). Supposedly, tumor cells firstly migrate to other organs, and the established autophagy subsequently helps tumor growth and invasion to capture the surrounding normal cells. Interestingly, these metastasized cells were completely abolished in tumor flies reared on C3G media (Fig. 4E). These results indicate that C3G also alleviates autophagy established by metastasized tumor cells in other tissues. Altogether, C3G may effectively inhibit tumor progression via inhibition of both cell-autonomous and non-cell autonomous autophagy.
C3G enhances pharmacological efficacy of chloroquine in tumor treatment
CQ is an autophagy inhibitor that blocks fusion of autophagosomes with lysosomes, and can be extensively used to treat human cancers.43 To reduce the side effects of CQ, we investigated whether C3G is able to synergize with CQ in treating cancers.
At 12 days AEL, we found that both low dose (0.5 mg mL−1) and high dose (2.0 mg mL−1) CQ showed an inhibitory effect on tumor growth (a 34% and 56% decrease in the tumor size respectively) and invasion, and obviously the inhibitory effect of high dose CQ was more effective than that of low dose CQ. These findings suggested that a dose–effect relationship existed between the inhibitory effects and CQ concentrations (Fig. 7A–D). No significant difference in the inhibitory effect was observed between 2.0 mg mL−1 CQ and 0.4 mg mL−1 C3G supplementation (P > 0.05) (Fig. 7C and D). Interestingly, C3G (0.4 mg mL−1) synergized with low dose CQ (0.5 mg mL−1) can significantly repress tumor growth, showing a 66% decrease in the tumor size (Fig. 7B and C) and a 74% decrease in tumor numbers (Fig. 7D), and the inhibitory effects were stronger than those of either high dose CQ or C3G alone (Fig. 7A–D). Taken together, a combination of CQ and C3G exhibited a stronger antitumor effect in vivo and C3G may serve as an adjuvant drug in tumor therapy.
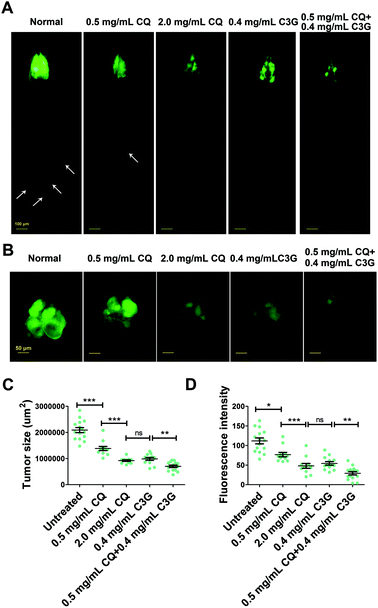 |
| Fig. 7 The combination of C3G and CQ showed a greater antitumor effect. (A and B) C3G synergized with low dose CQ showed a stronger antitumor effect than either CQ or C3G treatment alone at 12 days AEL. (C and D) Quantification of tumor size and fluorescence intensity (number of tumors) at day 12 AEL (n ≥ 12). Differences with *P < 0.05, **P < 0.01, or ***P < 0.001 were considered significant. | |
Discussion
Cancer undergoes a transformation from a fatal disease into a chronic disease and its cost imposes a heavy burden on our families.44 Chemotherapy is an effective treatment of cancers, but these drugs are quite toxic.45 Hence, it is imperative to discover new active substances. In vivo and in vitro experiments suggest that C3G, one of the most widely distributed components in black beans, displays anti-proliferative activities against cancer cells,46 but its antitumor mechanism is not fully illuminated. The results obtained in this study show that C3G plays a remarkable inhibitory role in tumor growth, invasion and distant metastasis in vivo by inhibiting both cell autonomous autophagy in tumor cells and non-autonomous autophagy in the tumor microenvironment which is dependent on JNK signaling. Our findings indicate that C3G may provide guidance on clinical drug development.
Tumorigenesis and tumor growth are inseparable from their microenvironment.47 Recent studies have suggested that natural active components play an important role in tumor progression by interacting with the tumor microenvironment.13 It has been reported that anthocyanins exert immunomodulatory effects on the tumor microenvironment and immunotherapy.48 C3G may activate the immune response in the tumor microenvironment and kill cancer cells.18 In our present study, we found that C3G could repress non-autonomous autophagy in the tumor microenvironment in vivo (Fig. 6A), which is required for tumor progression in Drosophila as confirmed by Katheder.30
In addition, cell-autonomous autophagy in tumor cells also plays a crucial role in tumorigenesis and tumor development.49 Cancers benefit from and require functional autophagy for their growth and progression.50 We found that the eye-antennal discs of malignant RafGOFscrib−/− flies showed massive autophagosomes in tumor cells (Fig. 6A), which promote tumor growth and invasion.50 Currently, numerous natural compounds are being involved in autophagy regulation.51 As a potential autophagy regulator, anthocyanins show an inhibitory effect on the proliferation of cancer cells in vitro by inducing autophagy.8 Contrary to their results, we found that C3G supplementation can break those autonomous autophagosomes in tumor flies, which may be attributed to the role of the tumor microenvironment.
Malignant tumor growth induces autophagy both in tumor cells and in its microenvironment.52 Based on these observations, we speculate C3G may inhibit tumor growth through two aspects: on the one hand, C3G inhibits autonomous autophagy of tumor cells that provides sustainable energy for tumor growth by degrading the dysfunctional components; on the other hand, C3G inhibits non-autonomous autophagy of the surrounding normal cells that provides recycled nutrients, including amino acid, fatty acid and other nutrient molecules, for tumor growth. The present study provides in vivo evidence for the inhibition of both autonomous and non-autonomous autophagy in tumor flies upon C3G treatment.
Emerging evidence indicates that excessive or a low level of autophagy in normal organisms can damage their health.53,54 We found that C3G has no effect on the autophagy level of normal flies (Fig. S2†), suggesting that C3G does not disturb autophagy homeostasis in normal organisms. Therefore, C3G supplementation is not harmful to human health, but can inhibit tumor progression by reducing the autonomous and non-autonomous autophagic activities.
As is known, the JNK signaling pathway plays a crucial role in tumor progression.55 The JNK signaling pathway is able to regulate autophagy in response to oxidative stress and oncogenic transformation.56 In the present study, JNK signaling is remarkably activated in the malignant RafGOFscrib−/− model, which was consistent with previous studies.57 C3G blocks the activation of JNK phosphorylation, showing an inhibitory effect on JNK signaling (Fig. 5A). These findings suggest that C3G may impede tumor progression via inhibition of the JNK-mediated autophagy pathway. In addition, transforming growth factor-β (TGF-β), secreted by cancer cells, activate autophagy by stimulating the expression of mRNA transcripts of several autophagy-related genes.58,59 Interestingly, autophagy also enhances TGF-β expression by inducing the activation of cyclic adenosine monophosphate (cAMP)/protein kinase A (PKA)/cAMP response element binding (CREB) signalling, leading to epithelial to mesenchymal transition (EMT).60 Not only that, the activated autophagy in tumors is also modulated through Smad and Wnt/β-catenin signaling.61,62 If C3G would affect these signaling pathways in tumor cells, the underlying mechanism needs further investigation.
JNK promotes the expression of MMPs, enzymes with clear links to tumor cell motility.63 Metastasis is a characteristic of malignant tumors and is one of the main causes of death in cancer patients, accounting for about 90% of cancer deaths.64 Therefore, targeting metastasis is the crucial point of treatment in cancer patients. The secretion of MMP1 helps tumor cells to invade the surrounding cells and metastasize to distant places.63,65 Obviously, massive MMP1 activation and invasive behaviors induced by RafGOFscrib−/− tumor cells can be significantly suppressed or nearly abolished upon C3G supplementation (Fig. 3B and C). These data indicate that C3G effectively inhibits JNK-mediated tumor metastasis.
Numerous clinical trials indicate that MMPs promote tumor invasion and metastasis, but most clinical trials of MMP inhibitors failed.66 Fortunately, pharmacological autophagy inhibitors serve as novel cancer therapeutic agents and have been used in cancer treatment.67,68 The autophagy inhibitor CQ suppresses autophagy by impairing the fusion of autophagosomes with lysosomes, showing a beneficial effect on cancer.43 CQ administration is effective at reducing tumor growth and metastasis in vivo.69,70 However, chronic CQ exposure leads to various diseases, such as irreversible retinal toxicity, dysfunction of liver and kidney, and cardiomyopathy.44,64 One of the new findings in this study is to implicate the roles of C3G in autophagy regulation in tumors. We found that a combination of C3G and CQ shows a greater antitumor effect than C3G or CQ treatment alone. This result led us to propose that C3G may be used clinically to replace or partially substitute for CQ in cancer treatment (Fig. 7). This is the first report to provide in vivo evidence for the adjuvant antitumor potential of the anthocyanin C3G combined with CQ, and the usefulness of this combined therapy needs to be assessed in clinical trials.
Conclusions
In summary, BBSCE and its active monomer C3G can inhibit tumor growth and invasion in vivo and prolong the survival of tumor flies. Further studies revealed that the inhibition of the cell-autonomous and non-cell autonomous autophagy pathways could be responsible for C3G's antitumor effect. Moreover, C3G combined with the antitumor agent CQ shows a stronger antitumor effect than either C3G or CQ treatment alone. These findings provide new evidence for further understanding of the molecular mechanisms by which C3G acts as a potential effective agent for cancer treatment.
Abbreviations
BBSCE | Black bean seed coat extract |
C3G | Cyanidin-3-O-glucoside |
AEL | After egg laying |
VNC | Ventral nerve cord |
CQ | Chloroquine |
TGF-β | Transforming growth factor-β |
cAMP | Cyclic adenosine monophosphate |
CREB | cAMP response element binding |
EMT | Epithelial to mesenchymal transition |
Conflicts of interest
The authors declare that they have no conflicts of interest.
Acknowledgements
This project was funded by the National Natural Science Foundation of China (31671284), the Fundamental Research Funds for the Central Universities (JZ2020HGPA0115 and PA2017GDQT0018), Youth Science and Technology Talents Support Program (2020) by Anhui Association for Science and Technology (RCTJ202001) and the National Postdoctoral Program for Innovative Talents (BX201600045). The authors appreciate the gifts of fly stocks from Dr Jose C. Pastor-Pareja (Tsinghua University, Beijing, China) and Dr Bing Zhou (Tsinghua University, Beijing, China). The authors are also grateful to the Bloomington Drosophila Stock Center, the Vienna Drosophila RNAi Center and Tsinghua Fly Center for fly stocks.
References
- X. Sun, Y. Zhang, J. Li, N. Aslam, H. Sun, J. Zhao, Z. Wu and S. He, Effects of particle size on physicochemical and functional properties of superfine black kidney bean (Phaseolus vulgaris L.) powder, PeerJ, 2019, 7, e6369 CrossRef PubMed.
- K. Ganesan and B. Xu, Polyphenol-Rich Dry Common Beans (Phaseolus vulgaris L.) and Their Health Benefits, Int. J. Mol. Sci., 2017, 18, 2331 CrossRef PubMed.
- J. Y. Wang, C. Zhu, T. W. Qian, H. Guo, D. D. Wang, F. Zhang and X. Yin, Extracts of black bean peel and pomegranate peel ameliorate oxidative stress-induced hyperglycemia in mice, Exp. Ther. Med., 2015, 9, 43–48 CrossRef PubMed.
- C. Huang, S. Y. Lee, C. L. Lin, T. H. Tu, L. H. Chen, Y. J. Chen and H. C. Huang, Co-treatment with quercetin and 1,2,3,4,6-penta-O-galloyl-beta-D-glucose causes cell cycle arrest and apoptosis in human breast cancer MDA-MB-231 and AU565 cells, J. Agric. Food Chem., 2013, 61, 6430–6445 CrossRef CAS PubMed.
- X. Hou, Y. Liu, L. Niu, L. Cui and M. Zhang, Enhancement of voltage-gated K+ channels and depression of voltage-gated Ca2+ channels are involved in quercetin-induced vasorelaxation in rat coronary artery, Planta Med., 2014, 80, 465–472 CAS.
- B. Xu and S. K. Chang, Antioxidant capacity of seed coat, dehulled bean, and whole black soybeans in relation to their distributions of total phenolics, phenolic acids, anthocyanins, and isoflavones, J. Agric. Food Chem., 2008, 56, 8365–8373 CrossRef CAS PubMed.
- L. F. de Sousa Moraes, X. Sun, M. Peluzio and M. J. Zhu, Anthocyanins/anthocyanidins and colorectal cancer: What is behind the scenes?, Crit. Rev. Food Sci. Nutr., 2019, 59, 59–71 CrossRef CAS PubMed.
- B. W. Lin, C. C. Gong, H. F. Song and Y. Y. Cui, Effects of anthocyanins on the prevention and treatment of cancer, Br. J. Pharmacol., 2017, 174, 1226–1243 CrossRef CAS PubMed.
- M. Dong, X. He and R. H. Liu, Phytochemicals of black bean seed coats: isolation, structure elucidation, and their antiproliferative and antioxidative activities, J. Agric. Food Chem., 2007, 55, 6044–6051 CrossRef CAS PubMed.
- J. K. Jhan, Y. C. Chung, G. H. Chen, C. H. Chang, Y. C. Lu and C. K. Hsu, Anthocyanin contents in the seed coat of black soya bean and their anti-human tyrosinase activity and antioxidative activity, Int. J. Cosmet. Sci., 2016, 38, 319–324 CrossRef CAS PubMed.
- M. Xu, K. A. Bower, S. Wang, J. A. Frank, G. Chen, M. Ding, X. Shi, Z. Ke and J. Luo, Cyanidin-3-glucoside inhibits ethanol-induced invasion of breast cancer cells overexpressing ErbB2, Mol. Cancer, 2010, 9, 285 CrossRef PubMed.
- L. Wang, H. Li, S. Yang, W. Ma, M. Liu, S. Guo, J. Zhan, H. Zhang, S. Y. Tsang, Z. Zhang, Z. Wang, X. Li and Y. D. Guo, Cyanidin-3-o-glucoside directly binds to ERalpha36 and inhibits EGFR-positive triple-negative breast cancer, Oncotarget, 2016, 7, 68864–68882 CrossRef PubMed.
- D. F. Quail and J. A. Joyce, Microenvironmental regulation of tumor progression and metastasis, Nat. Med., 2013, 19, 1423–1437 CrossRef CAS PubMed.
- M. Ding, R. Feng, S. Y. Wang, L. Bowman, Y. Lu, Y. Qian, V. Castranova, B. H. Jiang and X. Shi, Cyanidin-3-glucoside, a natural product derived from blackberry, exhibits chemopreventive and chemotherapeutic activity, J. Biol. Chem., 2006, 281, 17359–17368 CrossRef CAS PubMed.
- E. Cho, E. Y. Chung, H. Y. Jang, O. Y. Hong, H. S. Chae, Y. J. Jeong, S. Y. Kim, B. S. Kim, D. J. Yoo, J. S. Kim and K. H. Park, Anti-cancer Effect of Cyanidin-3-glucoside from Mulberry via
Caspase-3 Cleavage and DNA Fragmentation in vitro and in vivo, Anti-Cancer Agents Med. Chem., 2017, 17, 1519–1525 CrossRef CAS PubMed.
- S. W. Ryter, S. M. Cloonan and A. M. Choi, Autophagy: a critical regulator of cellular metabolism and homeostasis, Mol. Cells, 2013, 36, 7–16 CrossRef CAS PubMed.
- E. E. Mowers, M. N. Sharifi and K. F. Macleod, Functions of autophagy in the tumor microenvironment and cancer metastasis, FEBS J., 2018, 285, 1751–1766 CrossRef CAS PubMed.
- C. Mazewski, M. S. Kim and E. Gonzalez de Mejia, Anthocyanins, delphinidin-3-O-glucoside and cyanidin-3-O-glucoside, inhibit immune checkpoints in human colorectal cancer cells in vitro and in silico, Sci. Rep., 2019, 9, 11560 CrossRef PubMed.
- S. Wu, Y. Hu, W. Bai, J. Zhao, C. Huang, C. Wen, L. Deng and D. Lu, Cyanidin-3-o-glucoside inhibits UVA-induced human dermal fibroblast injury by upregulating autophagy, Photodermatol., Photoimmunol. Photomed., 2019, 35, 360–368 CrossRef CAS PubMed.
- S. Staats, K. Luersen, A. E. Wagner and G. Rimbach, Drosophila melanogaster as a Versatile Model Organism in Food and Nutrition Research, J. Agric. Food Chem., 2018, 66, 3737–3753 CrossRef CAS PubMed.
- R. Tang, X. Chen, T. Dang, Y. Deng, Z. Zou, Q. Liu, G. Gong, S. Song, F. Ma, L. Huang and Z. Wang, Lycium barbarum polysaccharides extend the mean lifespan of Drosophila melanogaster, Food Funct., 2019, 10, 4231–4241 RSC.
- S. Chen, Q. Yang, X. Chen, Y. Tian, Z. Liu and S. Wang, Bioactive peptides derived from crimson snapper and in vivo anti-aging effects on fat diet-induced high fat Drosophila melanogaster, Food Funct., 2020, 11, 524–533 RSC.
- Z. Mirzoyan, M. Sollazzo, M. Allocca, A. M. Valenza, D. Grifoni and P. Bellosta, Drosophila melanogaster: A Model Organism to Study Cancer, Front. Genet., 2019, 10, 51 CrossRef CAS PubMed.
- M. F. Wangler, S. Yamamoto and H. J. Bellen, Fruit flies in biomedical research, Genetics, 2015, 199, 639–653 CrossRef CAS PubMed.
- D. A. Wassarman, M. Therrien and G. M. Rubin, The Ras signaling pathway in Drosophila, Curr. Opin. Genet. Dev., 1995, 5, 44–50 CrossRef CAS PubMed.
- A. A. Samatar and P. I. Poulikakos, Targeting RAS-ERK signalling in cancer: promises and challenges, Nat. Rev. Drug Discovery, 2014, 13, 928–942 CrossRef CAS PubMed.
- R. A. Pagliarini and T. Xu, A genetic screen in Drosophila for metastatic behavior, Science, 2003, 302, 1227–1231 CrossRef CAS PubMed.
- M. Wu, J. C. Pastor-Pareja and T. Xu, Interaction between Ras(V12) and scribbled clones induces tumour growth and invasion, Nature, 2010, 463, 545–548 CrossRef CAS PubMed.
- K. Doggett, F. A. Grusche, H. E. Richardson and A. M. Brumby, Loss of the Drosophila cell polarity regulator Scribbled promotes epithelial tissue overgrowth and cooperation with oncogenic Ras-Raf through impaired Hippo pathway signaling, BMC Dev. Biol., 2011, 11, 57 CrossRef CAS PubMed.
- N. S. Katheder, R. Khezri, F. O'Farrell, S. W. Schultz, A. Jain, M. M. Rahman, K. O. Schink, T. A. Theodossiou, T. Johansen, G. Juhasz, D. Bilder, A. Brech, H. Stenmark and T. E. Rusten, Microenvironmental autophagy promotes tumour growth, Nature, 2017, 541, 417–420 CrossRef CAS PubMed.
- G. Xiao, Q. Fan, X. Wang and B. Zhou, Huntington disease arises from a combinatory toxicity of polyglutamine and copper binding, Proc. Natl. Acad. Sci. U. S. A., 2013, 110, 14995–15000 CrossRef CAS PubMed.
- G. Xiao, Z. H. Liu, M. Zhao, H. L. Wang and B. Zhou, Transferrin 1 Functions in Iron Trafficking and Genetically Interacts
with Ferritin in Drosophila melanogaster, Cell Rep., 2019, 26, 748–758 CrossRef CAS PubMed.
- M. Tipping and N. Perrimon, Drosophila as a model for context-dependent tumorigenesis, J. Cell. Physiol., 2014, 229, 27–33 CAS.
- X. Guan, Cancer metastases: challenges and opportunities, Acta Pharm. Sin. B, 2015, 5, 402–418 CrossRef PubMed.
- M. Sonoshita and R. L. Cagan, Modeling Human Cancers in Drosophila, Curr. Top. Dev. Biol., 2017, 121, 287–309 CAS.
- H. J. Kim, I. Tsoy, J. M. Park, J. I. Chung, S. C. Shin and K. C. Chang, Anthocyanins from soybean seed coat inhibit the expression of TNF-alpha-induced genes associated with ischemia/reperfusion in endothelial cell by NF-kappa B-dependent pathway and reduce rat myocardial damages incurred by ischemia and reperfusion in vivo, FEBS Lett., 2006, 580, 1391–1397 CrossRef CAS PubMed.
- J. Tan, Y. Li, D. X. Hou and S. Wu, The Effects and Mechanisms of Cyanidin-3-Glucoside and Its Phenolic Metabolites in Maintaining Intestinal Integrity, Antioxidants, 2019, 8, 479 CrossRef CAS PubMed.
- M. B. Hammouda, A. E. Ford, Y. Liu and J. Y. Zhang, The JNK Signaling Pathway in Inflammatory Skin Disorders and Cancer, Cells, 2020, 9, 857 CrossRef CAS PubMed.
- M. Das, D. S. Garlick, D. L. Greiner and R. J. Davis, The role of JNK in the development of hepatocellular carcinoma, Genes Dev., 2011, 25, 634–645 CrossRef CAS PubMed.
- A. Srivastava, J. C. Pastor-Pareja, T. Igaki, R. Pagliarini and T. Xu, Basement membrane remodeling is essential for Drosophila disc eversion and tumor invasion, Proc. Natl. Acad. Sci. U. S. A., 2007, 104, 2721–2726 CrossRef CAS PubMed.
- J. Dai, M. Ma, Z. Feng and J. C. Pastor-Pareja, Inter-adipocyte Adhesion and Signaling by Collagen IV Intercellular Concentrations in Drosophila, Curr. Biol., 2017, 27, 2729–2740 CrossRef CAS PubMed.
- W. O. Miles, N. J. Dyson and J. A. Walker, Modeling tumor invasion and metastasis in Drosophila, Dis. Models Mech., 2011, 4, 753–761 CrossRef CAS PubMed.
- M. Mauthe, I. Orhon, C. Rocchi, X. Zhou, M. Luhr, K. J. Hijlkema, R. P. Coppes, N. Engedal, M. Mari and F. Reggiori, Chloroquine inhibits autophagic flux by decreasing autophagosome-lysosome fusion, Autophagy, 2018, 14, 1435–1455 CrossRef CAS PubMed.
- K. D. Miller, L. Nogueira, A. B. Mariotto, J. H. Rowland, K. R. Yabroff, C. M. Alfano, A. Jemal, J. L. Kramer and R. L. Siegel, Cancer treatment and survivorship statistics, 2019, CA Cancer J. Clin., 2019, 69, 363–385 CrossRef PubMed.
- A. C. Cormier, L. Drapek, J. Fahey, B. Rowen, B. Burns-Britton, M. Lavadinho-Lemos and T. Hultman, When the Patient Seeks Cure: Challenging Chemotherapy and Radiation Side Effects Requiring Creative Solutions, Clin. J. Oncol. Nurs., 2016, 20, 117–120 CrossRef PubMed.
- T. S. Liang, R. F. Guan, Z. Wang, H. T. Shen, Q. L. Xia and M. Q. Liu, Comparison of anticancer activity and antioxidant activity between cyanidin-3-O-glucoside liposomes and cyanidin-3-O-glucoside in Caco-2 cells in vitro, RSC Adv., 2017, 7, 37359–37368 RSC.
- G. Deep and R. Agarwal, Targeting tumor microenvironment with silibinin: promise and potential for a translational cancer chemopreventive strategy, Curr. Cancer Drug Targets, 2013, 13, 486–499 CrossRef CAS PubMed.
- C. Focaccetti, V. Izzi, M. Benvenuto, S. Fazi, S. Ciuffa, M. G. Giganti, V. Potenza, V. Manzari, A. Modesti and R. Bei, Polyphenols as Immunomodulatory Compounds in the Tumor Microenvironment: Friends or Foes?, Int. J. Mol. Sci., 2019, 20, 1714 CrossRef CAS PubMed.
- R. Amaravadi, A. C. Kimmelman and E. White, Recent insights into the function of
autophagy in cancer, Genes Dev., 2016, 30, 1913–1930 CrossRef CAS PubMed.
- E. White, The role for autophagy in cancer, J. Clin. Invest., 2015, 125, 42–46 CrossRef PubMed.
- V. Naponelli, A. Modernelli, S. Bettuzzi and F. Rizzi, Roles of autophagy induced by natural compounds in prostate cancer, BioMed Res. Int., 2015, 2015, 121826 CAS.
- H. Folkerts, S. Hilgendorf, E. Vellenga, E. Bremer and V. R. Wiersma, The multifaceted role of autophagy in cancer and the microenvironment, Med. Res. Rev., 2019, 39, 517–560 CrossRef PubMed.
- B. Levine and G. Kroemer, Autophagy in the pathogenesis of disease, Cell, 2008, 132, 27–42 CrossRef CAS PubMed.
- S. J. Cherra 3rd and C. T. Chu, Autophagy in neuroprotection and neurodegeneration: A question of balance, Future Neurol., 2008, 3, 309–323 Search PubMed.
- C. Bubici and S. Papa, JNK signalling in cancer: in need of new, smarter therapeutic targets, Br. J. Pharmacol., 2014, 171, 24–37 CrossRef CAS PubMed.
- Y. Y. Zhou, Y. Li, W. Q. Jiang and L. F. Zhou, MAPK/JNK signalling: a potential autophagy regulation pathway, Biosci. Rep., 2015, 35, e00199 CrossRef PubMed.
- M. Uhlirova, H. Jasper and D. Bohmann, Non-cell-autonomous induction of tissue overgrowth by JNK/Ras cooperation in a Drosophila tumor model, Proc. Natl. Acad. Sci. U. S. A., 2005, 102, 13123–13128 CrossRef CAS PubMed.
- S. Dash, P. M. Sarashetti, B. Rajashekar, R. Chowdhury and S. Mukherjee, TGF-beta2-induced EMT is dampened by inhibition of autophagy and TNF-alpha treatment, Oncotarget, 2018, 9, 6433–6449 CrossRef PubMed.
- H. T. Chen, H. Liu, M. J. Mao, Y. Tan, X. Q. Mo, X. J. Meng, M. T. Cao, C. Y. Zhong, Y. Liu, H. Shan and G. M. Jiang, Crosstalk between autophagy and epithelial-mesenchymal transition and its application in cancer therapy, Mol. Cancer, 2019, 18, 101 CrossRef PubMed.
- S. Hu, L. Wang, X. Zhang, Y. Wu, J. Yang and J. Li, Autophagy induces transforming growth factor-beta-dependent epithelial-mesenchymal transition in hepatocarcinoma cells through cAMP response element binding signalling, J. Cell. Mol. Med., 2018, 22, 5518–5532 CrossRef CAS PubMed.
- J. Li, B. Yang, Q. Zhou, Y. Wu, D. Shang, Y. Guo, Z. Song, Q. Zheng and J. Xiong, Autophagy promotes hepatocellular carcinoma cell invasion through activation of epithelial-mesenchymal transition, Carcinogenesis, 2013, 34, 1343–1351 CrossRef CAS PubMed.
- L. Zhu, X. Fu, X. Chen, X. Han and P. Dong, M2 macrophages induce EMT through the TGF-beta/Smad2 signaling pathway, Cell Biol. Int., 2017, 41, 960–968 CrossRef CAS PubMed.
- M. Uhlirova and D. Bohmann, JNK- and Fos-regulated Mmp1 expression cooperates with Ras to induce invasive tumors in Drosophila, EMBO J., 2006, 25, 5294–5304 CrossRef CAS PubMed.
- M. F. Marmor, U. Kellner, T. Y. Lai, R. B. Melles and W. F. Mieler, Recommendations on Screening for Chloroquine and Hydroxychloroquine Retinopathy (2016 Revision), Ophthalmology, 2016, 123, 1386–1394 CrossRef PubMed.
- X. Ma, J. Y. Lu, Y. Dong, D. Li, J. N. Malagon and T. Xu, PP6 Disruption Synergizes with Oncogenic Ras to Promote JNK-Dependent Tumor Growth and Invasion, Cell Rep., 2017, 19, 2657–2664 CrossRef CAS PubMed.
- R. E. Vandenbroucke and C. Libert, Is there new hope for therapeutic matrix metalloproteinase inhibition?, Nat. Rev. Drug Discovery, 2014, 13, 904–927 CrossRef CAS PubMed.
- C. Wang, Q. Hu and H. M. Shen, Pharmacological inhibitors of autophagy as novel cancer therapeutic agents, Pharmacol. Res., 2016, 105, 164–175 CrossRef CAS PubMed.
- M. Cordani and A. Somoza, Targeting autophagy using metallic nanoparticles: a promising strategy for cancer treatment, Cell. Mol. Life Sci., 2019, 76, 1215–1242 CrossRef CAS PubMed.
- T. Hu, P. Li, Z. Luo, X. Chen, J. Zhang, C. Wang, P. Chen and Z. Dong, Chloroquine inhibits hepatocellular carcinoma cell growth in vitro and in vivo, Oncol. Rep., 2016, 35, 43–49 CrossRef CAS PubMed.
- K. Sun, X. L. Guo, Q. D. Zhao, Y. Y. Jing, X. R. Kou, X. Q. Xie, Y. Zhou, N. Cai, L. Gao, X. Zhao, S. S. Zhang, J. R. Song, D. Li, W. J. Deng, R. Li, M. C. Wu and L. X. Wei, Paradoxical role of autophagy in the dysplastic and tumor-forming stages of hepatocarcinoma development in rats, Cell Death Dis., 2013, 4, e501 CrossRef CAS PubMed.
Footnote |
† Electronic supplementary information (ESI) available: Fig. S1: C3G repressed the expression of pJNK and Atg8a in tumor flies. Fig. S2: The autophagy levels in the whole body and gut of normal flies were not affected by BBSCE (A) or C3G (B and C). See DOI: 10.1039/d0fo02107e |
|
This journal is © The Royal Society of Chemistry 2021 |