DOI:
10.1039/D0TB01628D
(Review Article)
J. Mater. Chem. B, 2021,
9, 53-79
Resorufin-based responsive probes for fluorescence and colorimetric analysis†
Received
2nd July 2020
, Accepted 12th October 2020
First published on 14th October 2020
Abstract
The fluorescence imaging technique has attracted increasing attention in the detection of various biological molecules in situ and in real-time owing to its inherent advantages including high selectivity and sensitivity, outstanding spatiotemporal resolution and fast feedback. In the past few decades, a number of fluorescent probes have been developed for bioassays and imaging by exploiting different fluorophores. Among various fluorophores, resorufin exhibits a high fluorescence quantum yield, long excitation/emission wavelength and pronounced ability in both fluorescence and colorimetric analysis. This fluorophore has been widely utilized in the design of responsive probes specific for various bioactive species. In this review, we summarize the advances in the development of resorufin-based fluorescent probes for detecting various analytes, such as cations, anions, reactive (redox-active) sulfur species, small molecules and biological macromolecules. The chemical structures of probes, response mechanisms, detection limits and practical applications are investigated, which is followed by the discussion of recent challenges and future research perspectives. This review article is expected to promote the further development of resorufin-based responsive fluorescent probes and their biological applications.
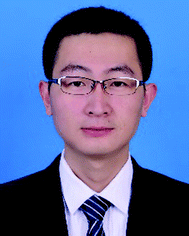
Zhichao Dai
| Zhichao Dai studied Analytical Chemistry at the Dalian University of Technology (China), where he received his PhD in 2015 for his contributions to the field of responsive probes for luminescence bioassay and imaging. He moved to the College of Chemistry and Chemical Engineering, Linyi University (China) immediately after his PhD to start an independent academic career as a lecturer. His current research focuses on the design, synthesis, and characterisation of luminescent molecular and nano-probes for biological and biomedical applications. |
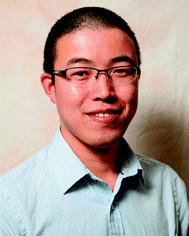
Run Zhang
| Run Zhang received his PhD from the Dalian University of Technology in 2012. He was a postdoctoral fellow and then a Macquarie University Research Fellow (MQRF) at MQ for three years from 2012 to 2015. He joined the Australian Institute for Bioengineering and Nanotechnology, The University of Queensland (AIBN, UQ) in 2016. Here, he was awarded an ARC DECRA Fellowship for 2017–2019 and is now an NHMRC Emerging Leadership Fellow. He is currently a leader of the biosensing and bioimaging team, working on the development of responsive molecules/nanomaterials for early disease diagnosis and treatment. |
1. Introduction
Rapid research developments in analytical chemistry are significantly contributing to biological and medical investigations by providing robust analytical methods for the detection of various biomarkers (biomolecules). Of the various biomolecules to be detected, substantial evidence has revealed that bioactive species such as reactive oxygen/nitrogen species (ROS/RNS), biothiols, anions, cations, and enzymes play important roles in diverse physiological processes. Alterations of their concentrations within the body are associated with various diseases.1–4 Therefore, the development of analytical techniques for the accurate determination of the levels of these analytes in-situ and in real-time is of great significance for better understanding the roles of these species in biological systems and for pre(clinical) diagnosis of various diseases.5–7 To date, a variety of analytical techniques including high-performance liquid chromatography, chromatography, electrochemical methods, mass spectrometry and flow injection analysis have been established for the detection of these target species.8–10 Of these methods, the fluorescence bioanalysis and imaging technique using uniquely tailored probes has been widely adopted in the fields of life science, biomedicine and pre(clinical) diagnostics owing to its high resolution, excellent selectivity and sensitivity, simplicity, and capability of real-time and noninvasive monitoring.11–14 Accordingly, great efforts have been devoted in recent years to developing fluorescent probes for the detection of target analytes, which has been achieved by recording the variation of probes’ spectroscopic characteristics post their interaction with analytes.15–18
Fluorescent probes are generally designed by incorporating analyte-recognition moieties with appropriate fluorophores. As a reporter, a variety of fluorophores with different excitation and emission wavelengths have been utilized for the design of fluorescent probes, examples of which use organic molecules,19,20 transition metal complexes,21–23 metal nanoclusters,24–26 and semiconductor quantum dots (QDS)27–29 as the fluorophores. Among these fluorophores, organic fluorescent dyes, such as coumarin, fluorescein, rhodamine, boron-dipyrromethenes (BODIPY), cyanine, etc., (Fig. S1, ESI†) have been carefully investigated for the development of fluorescent probes.30–32 For example, the BODIPY dye exhibits high fluorescence quantum yield, and sharp absorption and emission spectra, which enables probes to be developed for the detection of various biomolecules in vitro and in vivo.33,34 Many negatively charged dyes, such as fluorescein, have also been widely used for bioassay and imaging in live cells and organisms.33 For fluorescence imaging of biomolecules in tissues or small animals (e.g., mouse), the use of near infrared (NIR) fluorophores not only minimizes the disturbance from background auto-fluorescence in living systems and reduces photo-damage to biological samples, but also enables the biomolecules in deep tissue to be detected due to the larger penetration depth.35–37 For the development of NIR fluorescent probes, the most widely used fluorophores are cyanine derivatives,38 such as Cy5, Cy7, and Cy7.5.
Colorimetric analysis is a technique that is normally used to determine the analyte levels by comparing the color changes of the solution containing indicators (responsive probes). The concentration of the analyte can thus be determined by either Beer–Lambert's law or the standard curve after recording the changes of UV-vis absorption spectra. Different from fluorescent analysis, the color changes of the solution are able to be discerned with the naked eye.39 As a result, semi-quantitative determination of analyte levels could be achieved by colorimetric analysis without an equipped laboratory and spectrometers. To date, a variety of colorimetric probes have been developed by modifying well-defined chromophores, such as BODIPY, anthracene, rhodamine, fluorescein, coumarin, and resorufin.39–41
As an important organic fluorophore/chromophore, resorufin (Fig. 1A) has been frequently utilized in the development of responsive probes for fluorescence and colorimetric analysis. To date, more than 100 probes have been developed based on the resorufin scaffold and some of the probes have been commercialized. This is mainly because resorufin exhibits (i) unique photophysical properties, such as long excitation and emission wavelength (572/585 nm), high fluorescence quantum yield (ϕ = 0.74 in water), and large molar absorptivity (56
000 M−1 cm−1), (ii) favorable stability to light irradiation and pH changes (pH 6.0–10.0), (iii) high water solubility (1 mg mL−1) and biocompatibility, and (iv) pronounced capability in both fluorescence and colorimetric bioassay (Table S1, ESI†).42–45 The fluorescence intensity of the resorufin dye could be easily regulated by modification of its chemical structure, which facilitates the application of this fluorophore to the development of responsive fluorescent probes for bioassay. In most of the resorufin probes, the probes’ color can be changed from light yellow to pink after the response reaction with the analyte. This color change allows for colorimetric analysis of the analyte levels with the naked eye. More importantly, although the synthesis procedure of resorufin is not very clear from the recent literature, this fluorophore is commercially available at an affordable price. The responsive probes thus can be easily prepared by modifying the purchased resorufin fluorophore with an analyte-recognition moiety.
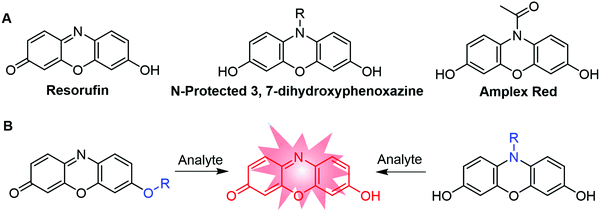 |
| Fig. 1 Chemical structures of resorufin, N-protected 3,7-dihydroxyphenoxazine, and Amplex Red (A) and the two general approaches for the design of resorufin-based responsive fluorescent probes (R = recognition moiety) (B). | |
Generally, there are two approaches to modify the resorufin dye for the development of responsive fluorescent probes (Fig. 1B). In the first approach, the probes are designed by the substitution (“protection”) of the 7-hydroxy group of resorufin. The intramolecular charge transfer (ICT) process of resorufin is thus weakened, resulting in the quenching of resorufin fluorescence. However, the analyte-triggered de-protection of the probes’ substituent groups allows for the recovery of the push–pull process in resorufin. The fluorescence signals are thus increased for the analyte detection with excellent signal-to-noise ratios. Based on this “protection–deprotection” approach, a variety of “OFF–ON” fluorescence responsive probes have been developed.46–48 Representative examples are nitrobenzenesulfonate–resorufin for fluoride ions,42 4-nitrobenzyl ether/carbonate-resorufin for nitroreductase,49 acrylate–resorufin for glutathione,50 ester–resorufin for hydrogen peroxide (H2O2),51 bromo-ester–resorufin for hydrazine,52etc. The second approach for the development of resorufin-based fluorescent probes relies on the N-protected 3,7-dihydroxyphenoxazine skeleton.43,53–55 As a result of N-protection, the π-conjugation system is interrupted, leading to the weak fluorescence of probes. Specific analyte-triggered deprotection reactions allow for the production of fluorescent resorufin, offering “OFF–ON” fluorescence responses to the analyte detection. In this approach, N-acetyl-3,7-dihydroxyphenoxazine (Amplex Red) has been widely used for the detection of various reactive biomolecules, such as H2O2.56 The almost non-fluorescent Amplex Red can react with H2O2 in the presence of specific enzymes, such as horseradish peroxidase (HRP). As a result of this specific reaction, Amplex Red could be converted to resorufin with obvious enhancement of fluorescence intensity for H2O2 detection. Based on a similar response mechanism, several methods for the detection of H2O2 has also been developed by using the nano-enzyme catalyzed oxidation reaction with N-modified resorufin derivates.57–59
In recent years, many excellent review articles have been published to summarize the progress in the development of responsive fluorescent probes by using the fluorophores of BODIPY, rhodamine, fluorescein, and cyanine.33,60–63 Reviews devoted to the resorufin-based fluorescent probes for the detection and imaging the levels of various analytes have not been found. In view of the significant contributions of resorufin fluorophores to the field of bioassay and imaging, it is quite worthy to comprehensively summarize the progress and achievements in the development of resorufin-based fluorescent probes. In this article, we summarize the recent studies on resorufin-based responsive fluorescent probes for the detection of various analytes, such as cations, anions, reactive (redox-active) sulfur species, small molecules, and biological macromolecules. The design strategies of the probes, sensing performances, response reaction mechanisms, and biological applications are discussed. The current challenges and future research directions in developing resorufin-based probes are then proposed. It is anticipated that this review article will facilitate the design and development of new fluorescent probes and promote the advancement of resorufin in future biological applications.
2. Progress of resorufin-based fluorescent probes
2.1 Fluorescent probes for the detection of cations
2.1.1 Fluorescent resorufin probes for the detection of lead and mercury ions (Pb2+/Hg2+).
Lead and mercury are toxic metals that have been widely used in chemical and petroleum industries.64,65 The discharge of these metals can cause serious environmental hazards and irreversible damage to animal and human health.66 By incorporating p-nitrophenol into a resorufin skeleton with a phosphodiester bond linker, a Pb2+-responsive fluorescent probe, NPPA, was developed by Sun and coworkers (Fig. 2).67NPPA showed weak fluorescence due to the intramolecular photoinduced electron transfer (PET) and the interruption of ICT processes. The reaction of NPPA with Pb2+ resulted in the cleavage of one phosphate ester bond, accompanied by the enhancement of fluorescence intensity at 498 nm (λex = 366 nm). The fluorescence variation of the probe was distinct from that of resorufin (λem = 585 nm), indicating the formation of phosphate ester substituted resorufin as the product (Fig. 2). The fluorescence response of NPPA to Pb2+ showed a good linearity in the Pb2+ concentration range of 50–125 nM in KH2PO4-NaOH buffer (50 mM, pH 8.0) with a detection limit of 22 nM. Furthermore, the probe exhibited good water solubility and high specificity for Pb2+ over other common metal ions.
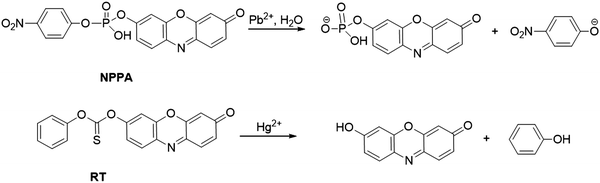 |
| Fig. 2 Chemical structures of probe NPPA and RT, and the response reaction mechanisms of probes for Pb2+ and Hg2+ detection, respectively. | |
On the basis of the high desulfurization of mercury ions, Choi et al. synthesized a Hg2+ probe, RT, by protecting the hydroxyl group of resorufin with the phenyl thionochloroformate group (Fig. 2).68 The Hg2+-induced cleavage reaction of the thionocarbonate group led to the formation of fluorescent resorufin, accompanied by a notable color change from yellow to pink. The absorbance of RT at 578 nm showed a good linearity with the concentration of Hg2+, which ensured low detection limits of RT for Hg2+ detection (6.3 × 10−8 M in CH3CN/citrate buffer (20 mM, 1
:
1, v/v, pH 6.2) and 5.8 × 10−8 M in simulated wastewater sample). RT was successfully applied in the construction of a microfluidic paper-based analytical device (μPAD) for Hg2+ detection in simulated wastewater samples (detection limit of 5.9 × 10−6 M).
Similar to activities with peroxidase (e.g., HRP), metal ion modified gold nanoparticles (Au NPs) are capable of serving as the nano-enzyme for various oxidation reactions. Based on AuNP nano-enzymes, two nano-platforms [Pt4+/Pb2+(AND)-Au NPPOX and Bi3+/Hg2+(INHIBIT)-Au NPPOX] were prepared by Lien and coworkers for the detection of Pb2+ and Hg2+.69 As shown in Fig. 3, due to the strong metallophilic interactions of Pt-Pb atoms/ions and aurophilic interactions of Au-Pb/Pt atoms/ions, the coexistence of Pt4+ and Pb2+ augments the deposition of Pt/Pb atoms/ions on the surface of Au NPs, resulting in the boosted peroxidase-like activity. In contrast, the coexistence of Bi3+ and Hg2+ led to the inhibition of Au NPs’ catalysis activity owing to the strong Hg–Au amalgamation. The catalysis activity variations of Au NPs can lead to a distinct color and fluorescence change in the presence of Amplex Red and H2O2, which allowed Pt4+/Pb2+(AND)-Au NPPOX and Bi3+/Hg2+(INHIBIT)-Au NPPOX for the detection of Pb2+ and Hg2+, respectively. The detection limits of the probes for Pb2+ and Hg2+ were 1.6 nM and 1.2 nM in a Tris–borate buffer (5.0 mM, pH 9.0), respectively.
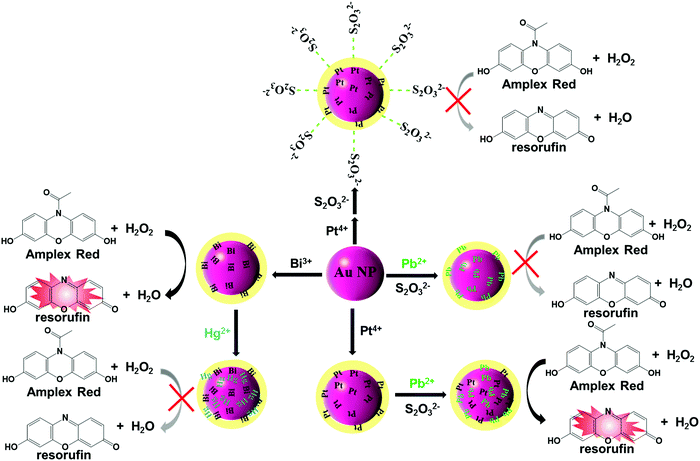 |
| Fig. 3 Schematic illustration of Pt4+/Pb2+(AND)-Au NPPOX and Bi3+/Hg2+(INHIBIT)-Au NPPOX for Pb2+ and Hg2+ detection. | |
2.1.2 Fluorescent resorufin probes for the detection of palladium species.
Palladium has been frequently used in various fields such as fine chemicals, jewellery and automobile catalytic converters. Residual palladium in food and water is a threat to human health.70,71 Ren and coworkers developed a Pd2+ fluorescent probe, 1 (Fig. 4) by using the thiophenemethyl amine modified O-proparyl group as the recognition unit.72 The strong affinity of the O-proparyl moiety towards Pd2+ resulted in the cleavage of the recognition moiety to form fluorescent resorufin. Over 24-fold enhancement of fluorescence intensity was observed in MeOH/PBS solution (10 mM, 8
:
2, v/v, pH 7.4). The probe showed an excellent linearity for Pd2+ detection (0–5 μM) with a detection limit of 0.05 μM. Using allyl chloroformate as the recognition unit, Yan group recently reported a probe, Res-1, for Pd detection (Fig. 4).73 The Tsuji–Trost reaction between the probe and Pd0 restored the fluorescence of resorufin with a typical color change from pale yellow to pink. The probe exhibited excellent selectivity and selectivity for Pd0 detection with a detection limit of 2.1 nM in PBS buffer (10 mM, pH 7.4). The probe was then applied for fluorescence imaging of palladium in HeLa cells. Similarly, Nieberding et al. reported another probe, RAE, for the detection of Pd0 on the basis of Pd0-catalyzed allylic C–O bond cleavage of resorufin allyl ether, (Fig. 4).74
 |
| Fig. 4 Chemical structures of resorufin-based fluorescent probes for palladium species detection. | |
2.1.3 Fluorescent resorufin probes for the detection of zinc ion (Zn2+).
Zn2+ plays a crucial role in various physiological processes.75,76 The abnormality in Zn2+ homeostasis is associated with many diseases, such as Alzheimer's disease and diabetes.77 To detect Zn2+ in biological samples, Loas and coworkers developed two resorufin-based ditopic fluorescent probes, ZBR4 and ZR1 (Fig. 5), by using 2,2′-dipicolylamine (DPA) as the recognition moiety for Zn2+ binding.78 The fluorescence of both probes was quenched due to the photoinduced electron transfer (PET) process from the amine groups to resorufin. Upon binding to Zn2+ in aqueous buffer (1 mM EGTA, 100 mM KCl, 50 mM PIPES, pH 7.0), PET of probes was inhibited, which resulted in about 14- and 41-fold enhancement in the fluorescence quantum yields of ZBR4 and ZR1, respectively. The application of ZBR4 and ZR1 was then demonstrated for imaging of Zn2+ in live HeLa cells (Fig. 5). Interestingly, ZR1 is able to accumulate in endoplasmic reticulum and ZBR4 is capable of localizing in mitochondria after cellular internalization, which enabled Zn2+ imaging at subcellular levels.
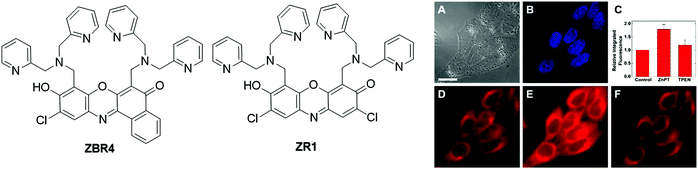 |
| Fig. 5 Chemical structures of fluorescent probes, ZBR4 and ZR1, and the fluorescence imaging of ZBR4 in HeLa cells (A: differential interference contrast image; B: incubated with Hoechst 33258; C: quantification of Zn2+-induced fluorescence response; D: fluorescence imaging before the addition of exogenous Zn2+; E: fluorescence imaging after treatment with ZnPT; F: fluorescence imaging after the addition of TPEN.). Adapted with permission from ref. 78. Copyright 2014 American Chemical Society. | |
2.1.4 Fluorescent resorufin probes for the detection of cobalt ions (Co2+) and cuprous ions (Cu+).
Cobalt is an essential trace element for humans and other living organisms. This metal, with both organic and inorganic forms, is involved in a variety of biological processes, such as the formation of myelin, carbohydrate metabolism and protein synthesis.79,80 High concentrations of Co2+ are also associated with diverse diseases such as cardiomyopathy and heart disease.81 Copper is essential for several crucial organism activities including the redox process and enzyme function. The primary state of copper is Cu+ rather than Cu2+ in cytosolic environments.82,83 The abnormal concentrations of copper can lead to various diseases, like Alzheimer's and Wilson's diseases.84 Resorufin-based fluorescent probes have also been developed recently for the detection of these two metal ions. Taking tetradentate ligands N3O and N4 as binding groups, Maity et al. developed two “OFF–ON” response probes, ResCo and ResCu (Fig. 6), for colorimetric and fluorometric detection of Co2+ and Cu+, respectively.85 In the presence of Co2+ and Cu+, both of the oxidative benzylic ether (C–O) bonds in probes were cleaved, resulting in a dramatic increase of the emission intensity with an obvious change of the solution color from light yellow to pink. The application of two probes for fluorescence imaging of Co2+ and Cu+ was realized in HEK293T cells.
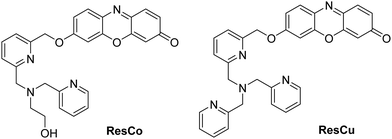 |
| Fig. 6 Chemical structures of fluorescent probes, ResCo and ResCu, for Co2+ and Cu+ detection, respectively. | |
2.2 Fluorescent probes for detection of anions
2.2.1 Fluorescent resorufin probes for the detection of fluoride ions (F−).
Fluoride ions, as the smallest and most electronegative anions, have been frequently utilized in toothpaste and treatment of osteoporosis.86 The abnormal level of fluoride has been implicated with many diseases, such as dental and skeletal fluorosis, osteosarcoma and nephrolithiasis.87–89 The resorufin fluorophore has also been involved in the development of responsive probes for F− detection. On the basis of the F−-triggered deprotection process of resorufin benzenesulfonate, three “OFF–ON” dual-signal probes, 2, 3 and 4, have been developed for F− detection by Im and coworkers (Fig. 7).42 Upon the addition of F−, the emission intensity of three probes at 591 nm was increased gradually, accompanied by a distinct color change from yellow to pink. In particular, the emission intensity of 2 showed a 58.9-fold enhancement in acetonitrile with higher sensitivity than other two probes (DoL, 1.9 × 10−6 M). Although the F− detection using probe 2 could be interfered by the sulfide ions (S2−), the addition of the masking agent (e.g., TPEN–Cu2+ complex) could effectively remove S2− from the samples to provide probe 2 with high specificity for F− detection.
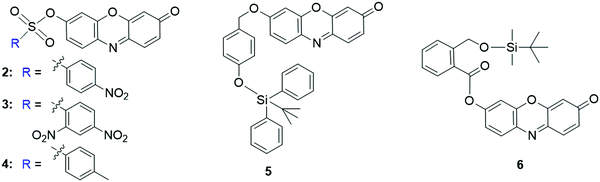 |
| Fig. 7 Chemical structures of resorufin-based fluorescent probes for the detection of F−. | |
The specific interaction of F− with silyl ethers was then exploited for the development of fluorescent probes for F− detection. For the probe 5 developed by Kim et al., the hydroxyl of resorufin was protected by Si–O bonds that can be cleaved by the F−-triggered Si–O bond cleavage reaction (Fig. 7).90 As a result, the fluorescence intensity of the probe was enhanced to be 500-fold and 200-fold in acetonitrile and CH3CN
:
HEPES buffer (10 mM, 1
:
1, v/v, pH 7.4), respectively. Additionally, the color of the solution changed from pale yellow to pink for the colorimetric analysis of F−. Similar probe 6 has also been reported by Roy and coworkers for the detection of F− (Fig. 7).91 In the presence of F−, the emission intensity was enhanced by 1820-fold in THF. The probe displayed a quick response towards F− (t1/2 = 1.96 min) and high sensitivity (DoL, 60 nM), enabling it for fluorescence imaging of F− in live HeLa cells.
2.2.2 Fluorescent resorufin probes for the detection of cyanide ions (CN−).
Of various anions, cyanide ions (CN−) are extremely poisonous and fatal to humans.92 The intake of CN− even at a very low amount will result in serious damage to the immune system and eventually leads to death in several minutes.93 Therefore, the methods for fast and accurate determination of CN− are highly demanded. Through coupling of 2′-formyl[1,1′-biphenyl]-2-carboxylic acid with resorufin, Lee et al. developed a “turn-on” fluorescent probe, IND-1 (Fig. 8), for the detection of CN− in aqueous environments.94 In the presence of CN−, the probe underwent an intramolecular crossed-benzoin reaction to yield highly fluorescent resorufin. The fluorescence intensity was increased to a maximum level within 10 s in PBS buffer. Probe IND-1 features high selectivity and sensitivity (DoL, 4 nM), allowing it to be used as a tool for the detection of CN− in blood serum and live HeLa cells.
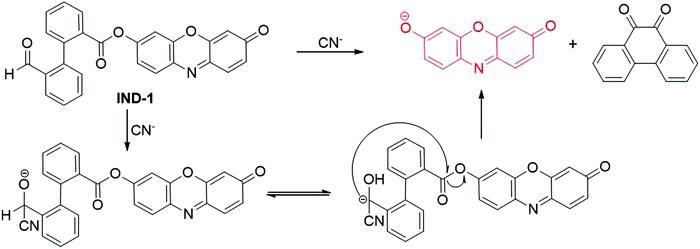 |
| Fig. 8 Chemical structure and the response reaction mechanism of fluorescent probe IND-1 for CN− detection. | |
Using a membrane-based nanozyme assay, the Lien group designed and prepared a nanoprobe for the detection of CN− in 2018.95 The cobalt hydroxide/oxide-modified graphene oxide (CoOxH-GO) exhibited excellent peroxidase-like catalytic properties, which could catalyze the oxidation reaction of Amplex Red to form resorufin with the assistance of H2O2. Owing to the high affinity of CN− to CoOxH-GO, the addition of CN− to the nanoparticles led to the formation of the Co–CN complex, thus restraining the electron transfer process between the CoOxH-GO and substrates. Therefore, the H2O2/AR-CoOxH-GO nanohybrid was used as a nanoprobe for the detection of CN−. The fluorescence intensity of the nanoprobe showed a good linearity to the concentration of CN− in the range of 75 nM–2.5 μM, and the detection limit was calculated to be 22 nM. Fluorescence detection of CN− in laboratory wastes and high salt sea water was carried out using the H2O2/AR-CoOxH-GO nanohybrid as a probe.
2.2.3 Fluorescent resorufin probes for the detection of phosphite.
Phosphite has been extensively used in agriculture and food industries, such as in fungicides, fertilizer, and organophosphorous pesticides. The discharge of phosphite-rich wastewaters would leading to serious environmental concerns such as eutrophication. Hence the detection of phosphite in water samples is an interesting topic in analytical chemistry and environmental analysis. Through recovery of the oxidase-like activity of NiO NPs (nickel oxide nanoparticles) suppressed by inhibitors (HEPES was selected), Chang et al. presented a fluorescent probe for the detection of phosphite.96 After the addition of phosphite to the NiO/HEPES/Amplex Red system of HEPES buffer solution of pH 7.5, the restoration of oxidase-like catalytic activity of NiO NPs led to the conversion of Amplex Red to resorufin. As a result, the fluorescence intensity at 580 nm increased during the phosphite analysis. The resulting mechanistic studies indicated that phosphite possesses stronger affinity towards NiO, which can replace HEPES from NiO and activate the catalyst. The detection limit for phosphite was deduced to be 1.46 μM. The probe was then used for the detection of phosphite in practical samples including lake water, cell culture medium and serum.
2.2.4 Fluorescent resorufin probes for the detection of perborate (BO3−).
Perborate (BO3−) has been frequently used in industry, such as for manufacturing bleaches, cosmetics and detergents.97 On the basis of the specific deprotection reaction of an acetate moiety triggered by perborate, Choi et al. developed a responsive fluorescent probe, 7 (Fig. 9), for BO3− detection.98 Probe 7 was designed with the protection of the hydroxyl group of resorufin by the acetate group. In the presence of BO3−, the cleavage of the acetate group led to the release of the free resorufin dye. As a result, a >520-fold change of the ratio between the absorbance at 572 and at 453 nm (A572/A453) and a dramatic “OFF–ON” fluorescence response in CH3CN-acetate solution (10 mM, 1
:
9, v/v, pH 4.8) were obtained. These changes of absorption and emission spectra enabled probe 7 to be used for BO3− detection with high sensitivity and selectivity.
 |
| Fig. 9 Chemical structure and response reaction mechanism of fluorescent probe 7 for BO3− detection. | |
2.3 Fluorescent probes for the detection of RSS, ROS, RNS, and other small molecules
2.3.1 Fluorescent resorufin probes for the detection of RSS.
2.3.1.1 Fluorescent resorufin probes for the detection of biothiols (GSH, Cys and Hcy).
As essential components of reactive sulfur species (RSS), low molecular weight biological thiols including glutathione (GSH), cysteine (Cys), and homocysteine (Hcy) are associated with diverse physiological processes.99–101 Owing to their excellent redox properties and nucleophilicity, biothiols have been recognized as significant antioxidants to protect cells and tissues against damage from ROS and other free radicals.99,102 Abnormal production of biothiols is closely related to various diseases, such as liver damage, cytotoxicity and cardiomyopathy.103 For the detection of biothiols, several resorufin-based fluorescent probes have been developed in recent years. For example, using 3 (Fig. 7) as a fluorescent probe, Zhao et al. reported a method for biothiol detection.104 In the presence of biothiols, the sulfonate ester linker of probe 3 was cleaved after the nucleophilic reaction, affording resorufin for colorimetric and fluorescence detection of biothiols. The detection limits for Cys, Hcy and GSH were determined to be 128, 148, and 183 nM, respectively. The probe was used for imaging of biothiols in rat cerebellum paraflocculus during salicylate-induced tinnitus. Using thiophenecarboxylate as the sensing moiety, the Zhou group developed a probe, 8 (Fig. 10), for selective detection of GSH over Hcy and Cys.105 Upon reaction with GSH in PBS buffer (10 mM, pH 7.4), an about 10-fold enhancement in fluorescence was observed. The detection limit was determined to be 8.9 × 10−7 M. The probe 8 was then used for the visualization of GSH in A549 cells.
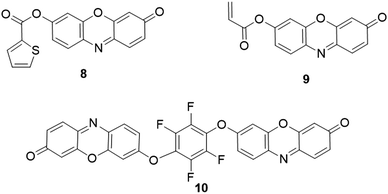 |
| Fig. 10 Chemical structures of fluorescent probes, 8–10, for biothiol detection. | |
Guo and coworkers reported another acrylate protected resorufin as the fluorescent probe (9) specific for GSH detection (Fig. 10).50 In PBS buffer (50 mM, pH 7.4) with cetyltrimethylammonium bromide (CTAB), the GSH-triggered nucleophilic reaction led to the liberation of resorufin, accompanied by the increase of emission intensity within 2 min. Interestingly, negligible changes of fluorescence spectra were observed upon the addition of Cys and Hcy. The presence of CTAB allowed the formation of micelles, which facilitated the reaction between probe 9 and GSH. The probe was then used for the detection of GSH in human plasma. Furthermore, probe 9 was successfully applied for the selective determination of GSH in whole blood deposited on filter paper. The recoveries for the paper-based detection were determined to be between 94% and 108.6%.106
By substituting hexafluorobenzene with two resorufin dyes at the para position, a fluorescent probe, 10 (Fig. 10), was presented by Ma and coworkers for the detection of thiols and the evaluation of redox homeostasis.107 Upon reaction with Cys and NaHS in MOPS buffer (0.2 M, pH 7.0), the cleavage of the ether bond resulted in the obvious enhancement of the emission intensity (∼28-fold within 160 s for HS− and ∼24-fold within 20 min for Cys, respectively). The different reaction rates were attributed to the diverse nucleophilic properties of NaHS and Cys. Selectivity studies revealed that other amino acids including GSH showed a negligible effect on the fluorescence response of probe 10 to Cys and HS−. In addition, resorufin could be reversibly reduced to nonfluorescent dihydroresorufin, which was treated as a redox indicator for investigating the redox variations induced by glucose oxidase (GOx)/glucose solution and dissolved oxygen. The reduced resorufin (dihydroresorufin) can also be used for tracing the dissolved oxygen in some biological processes. Probe 10 was then successfully employed as a tool for imaging thiol and redox homeostasis in macrophages. Nevertheless, the results implied that the responsive resorufin probes are not suitable for fluorescence and colorimetric analysis in oxygen-free solutions.
Gao et al. designed a fluorescent probe, 11 (Fig. 11), for simultaneous determination of Cys and GSH.108 Although two fluorophores were coupled (i.e., resorufin and 7-nitrobenzofurazan (NBD)), probe 11 was weakly fluorescent due to: (i) quenching of resorufin fluorescence by the substitution of hydroxyl, and (ii) quenching of NBD fluorescence by the “O” ether bond.109 The 7-nitrobenzofurazan (NBD) moiety of probe 11 exhibited a distinct property of nucleophilic aromatic substitution-rearrangement to Cys, leading to the formation of fluorescent resorufin and NBD-N-Cys. Only the nucleophilic substitution reaction could occur for GSH to produce fluorescent resorufin and non-fluorescent NBD-S-GSH, which enabled probe 11 to be used for the discrimination of Cys from GSH. With the concentrations of GSH/Cys increased from 0 to 500 μM, the fluorescence intensity increased by 43-fold (λem = 585 nm) for GSH, and 86-fold (λem = 540 nm) and 41-fold (λem = 585 nm) for Cys, respectively. The detection limits for Cys and GSH at 585 nm were calculated to be 0.13 μM and 0.07 μM, respectively. The probe was then used for the detection of Cys and GSH in human plasmas. Based on a similar strategy, Zhang recently developed a probe, RC (Fig. 11), for simultaneous discrimination of Cys, Hcy, GSH and H2S by integrating 7-diethylcoumarin with resorufin.110 In the presence of H2S, Cys, Hcy, and GSH, the ether linker between two dyes was cleaved, resulting in the generation of resorufin and diverse coumarin derivates with different absorption and emission wavelengths. Upon reacting with various sulfur species, multiple fluorescence signals with different emission intensity variations were obtained in DMF/PBS buffer (25 mM, 3
:
7, v/v, pH 7.4), i.e., a red emission channel for H2S (λem = 602 nm, 11.6-fold enhancement), blue–red emission channels for Cys (λem = 473 nm, 34-fold enhancement; λem = 602 nm, 3.9-fold enhancement), blue–green–red emission channels for Hcy (λem = 473 nm, 27-fold enhancement; λem = 537 nm; λem = 602 nm, 2.5-fold enhancement), and green–red emission channels for GSH (λem = 537 nm, 13-fold enhancement; λem = 602 nm, 4.9-fold enhancement). These distinct fluorescence responses allowed for the discrimination of these sulfur species in buffer and HeLa cells.
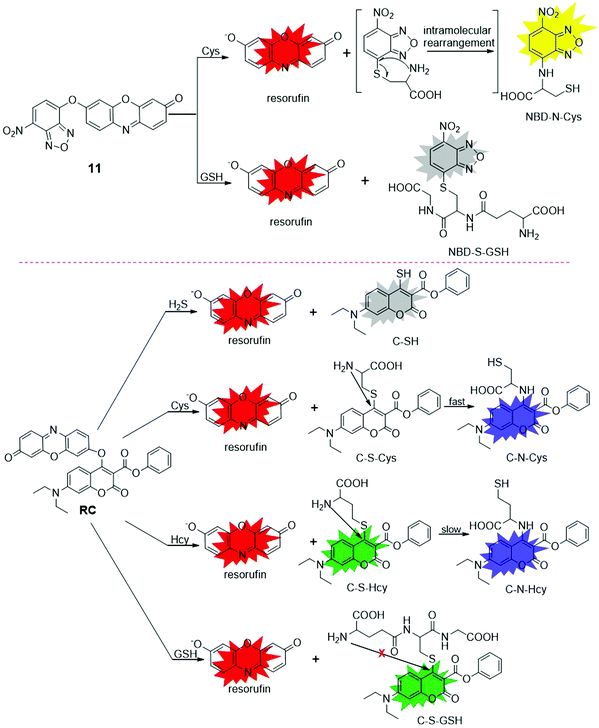 |
| Fig. 11 Chemical structures and response reaction mechanisms of fluorescent probes, 11 and RC, for selective detection of biothiols. | |
2.3.1.2 Fluorescent resorufin probes for the detection of hydrogen sulfide (H2S).
H2S is a colorless and flammable gas that has been widely investigated as a toxic gas,111 while recent research revealed that endogenous H2S produced by enzymatic reactions could serve as one of the gasotransmitters in living organisms.112,113 This gaseous molecule is involved in a variety of physiological processes and associated with various diseases.114 Saha et al. developed two resorufin-based fluorescent probes, Reso-N3 and Reso-Br, for the detection of H2S (Fig. 12).115 In the presence of H2S, resorufin was released after the specific azide-to-amine reduction and cyclization reaction with Reso-N3. For Reso-Br, a bromide-to-thiol nucleophilic substitution and a subsequent cyclization process occurred to release the resorufin. After reaction with H2S in PBS buffer (5 mM, pH 7.4), 614- and 414-fold emission enhancements were obtained for Reso-N3 and Reso-Br, respectively. Compared with Reso-Br, Reso-N3 showed high stability under physiological conditions and was applied for the imaging of intracellular H2S. Taking advantage of the tunable property of H2S on the peroxidase-like activity of enzyme mimics, Hsu and coworkers reported a FeOxH-rGO nanocomposite (iron hydroxide/oxide loaded on reduced graphene oxide) for H2S detection.116 In the presence of H2S, the formation of FeS resulted in the inhibition of its catalytic activity and the transformation of Amplex Red to resorufin was inhibited. This H2O2/Amplex Red-FeOxH-rGO nanosystem was then used for the detection of H2S sensitively (DoL, 50 nM). The application of this probe was also demonstrated for H2S detection in hot spring samples.
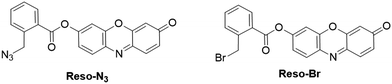 |
| Fig. 12 Chemical structures of fluorescent probes, Reso-N3 and Reso-Br, for H2S detection. | |
2.3.1.3 Fluorescent resorufin probes for the detection of sulfite (SO32−).
Sulfite (SO32−), is generally recognized as safe (GRAS) by the Food and Drug Administration (FDA). This anion has been widely used in the food industry as an additive in various foods and beverages, and an antimicrobial agent and antioxidant in wines.117,118 Recent studies also revealed that sulfur dioxide produced endogenously (presented as sulfite/bisulfite in biological fluids) can serve as a new messenger in the cardiovascular system for vasodilating, antihypertensive, and antiatherogenic effects.119 To detect this anion using a resorufin fluorescent probe, Choi et al. developed a chromogenic and fluorogenic probe, 12 (Fig. 13), by protecting the hydroxyl group of resorufin with a SO32− responsive levulinate group.120 The specific reaction of probe 12 with SO32− in CH3CN
:
HEPES solution (10 mM, 2
:
98, v/v, pH 7.0) led to the cleavage of the levulinate group to afford fluorescent resorufin. A 57-fold increase in the emission intensity was obtained after the response reaction. Meanwhile, the solution displayed a remarkable color change from dark to pink, enabling colorimetric analysis of SO32−. The probe showed excellent selectivity for SO32− and a detection limit of 4.9 × 10−5 M.
 |
| Fig. 13 Chemical structures of fluorescent probes, 12–14, for SO32−, H2Sn and aromatic thiols detection, respectively. | |
2.3.1.4 Fluorescent resorufin probes for the detection of polysulfides (H2Sn).
Hydrogen polysulfides (H2Sn, n > 1) belong to the group of RSS that are produced mainly by the reaction of H2S and ROS/RNS.121,122 In living organisms, H2Sn have been recognized as signaling molecules that play important roles in biological systems.123 For example, H2Sn were found to be more effective in protein S-sulfhydration than H2S owing to their favorable nucleophilicity and reducibility.124 Using nitro-activated fluorobenzonate as the recognition moiety, Liu et al. developed a responsive probe 13 for fluorescence detection of H2Sn (Fig. 13).125 The fluorescence of the probe was quenched through the effective PET process. Upon the addition of H2Sn (Na2S2 and Na2S4 as the donor of H2Sn), the sequential nucleophilic and an intramolecular cyclization reaction between H2Sn and probe led to the production of resorufin, accompanied by about 40-fold enhancement of emission intensity in PBS/CH3CN solution (10 mM, 3
:
7, v/v, pH = 7.4). The fluorescence increase showed a good linearity in the H2Sn concentration range from 0 to 50 μM with a detection limit of 24 nM. The probe showed low cytotoxicity and was used for imaging of endogenous H2Sn produced by lipopolysaccharide (LPS) stimulation of RAW 264.7 macrophage cells.
2.3.1.5 Fluorescent resorufin probes for the detection of aromatic thiols.
Aromatic thiols, such as thiophenol derivatives, belong to a class of thiols that have been widely used in pharmaceutical drugs, pesticides, and polymer materials.126 These thiols are highly toxic to living organisms, causing damage to the central nervous system after exposure under either liquid or vapor conditions.127,128 On the basis of nucleophilic reactions between dinitrophenyl ether and aromatic thiols, a fluorescent probe, 14 (Fig. 13), was reported by the Shiraishi group for aromatic thiol detection.48 The reaction of benzenethiol with probe 14 resulted in the cleavage of the dinitrophenyl group, accompanied by a dramatic increase of the fluorescence quantum yield from <0.001 to 0.38 in CH3CN/CHES buffer (100 mM, 1
:
1, v/v, pH 10.0). The selectivity study revealed that probe 14 is highly selective to benzenethiol over other nucleophiles such as aniline, phenol, I−, CN− and aliphatic thiols. The detection limit of probe 14 for benzenethiol was determined to be 0.07 mM.
2.3.2 Fluorescent resorufin probes for the detection of ROS.
2.3.2.1 Fluorescent resorufin probes for the detection of hydrogen peroxide (H2O2).
Hydrogen peroxide (H2O2) is the most stable ROS in living organisms. This biomolecule has been found to be implicated in various biological processes including cell proliferation, migration, and differentiation.129,130 An abnormal level of H2O2 is associated with many diseases, such as cancer and neurodegenerative diseases.130 As a result, recent research has been largely devoted to developing fluorescent probes for the detection of H2O2 in living systems,131–133 and H2O2-mediated boronate oxidation has been frequently used for the design of H2O2-responsive fluorescent probes.134 Takagi et al. developed two H2O2-reactive and photoactivatable theranostic agents, 15 and 16, by conjugating a photosensitizer group with resorufin using para-tolylboronic acid pinacol ester linkers (Fig. 14).51 Upon reaction with H2O2 in DMSO/PBS buffer (10 mM, 1
:
9, v/v, pH 7.4), the boronate group was oxidized, followed by an intramolecular cascade reaction of para-hydroxybenzylether derivative to afford resorufin. As a result, about 13- and 9-fold enhancements in fluorescence intensity were obtained for 15 and 16, respectively. Using boronate as the H2O2 recognition unit, the Odyniec group reported a dual-responsive (“AND”)-based fluorescent probe, Pinkment-OAc (Fig. 14), for the determination of H2O2 and esterase.135 The dual responsive fluorescent probes normally require the presence of both analytes under specific conditions for a fluorescence response.22 For Pinkment-OAc, prominent fluorescence enhancement was observed in the presence of both H2O2 and esterase.
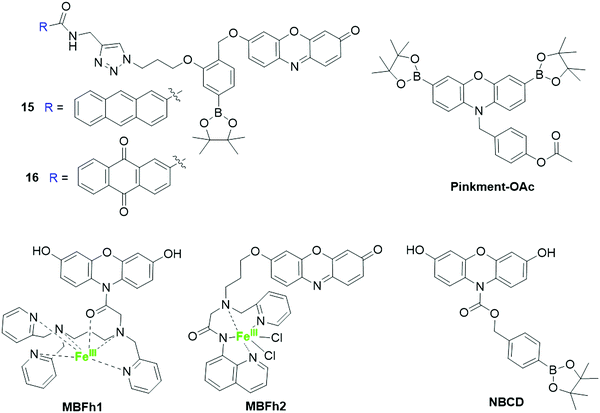 |
| Fig. 14 Chemical structures of resorufin-based fluorescent probes for the detection of H2O2. | |
N-Protected 3,7-dihydroxyphenoxazine-derived probes were recently developed for H2O2 detection. Using an iron complex as the recognition unit, Hitomi et al. presented a metal-based probe, MBFh1 (Fig. 14), for the detection of H2O2.53 The quick reaction between the iron complex of MBFh1 and H2O2 led to the formation of oxidants that can covert the 3,7-dihydroxyphenoxazine to resorufin in an intramolecular fashion. The detection limit for H2O2 was determined to be 3.2 μM in MOPS buffer (20 mM, 2.5% DMSO, pH 7.2). Moreover, the application of MBFh1 was then demonstrated to detect the enzymatically generated H2O2 in MOPS buffer. MBFh1 is not stable and can be decomposed under cell culture conditions through hydrolysis of the amide moiety. The hydrolysis prevents the application of MBFh1 in the analysis of intracellular H2O2. To address this issue, an improved metal-based fluorescent probe, MBFh2 (Fig. 14), containing an O-alkyl resorufin derivative and a nonheme iron-based peroxidase mimetic was developed by the same group.43 The reaction of MBFh2 with H2O2 resulted in the liberation of resorufin via a cascade reaction involving β-elimination. As a result, a 20-fold enhancement in fluorescence intensity was observed in HEPES buffer (20 mM, 2.5% DMSO, pH 7.2). MBFh2 showed high stability in buffer and cell culture media, allowing it to be used for the imaging of H2O2 in HeLa and A431 cells. Recently, Han and coworkers reported another N-borylbenzyloxycarbonyl-3,7-dihydroxyphenoxazine fluorescent probe, NBCD (Fig. 14), for the determination of intracellular H2O2.59 The oxidization of H2O2 led to the cleavage of the borate-based carbamate group and a gradual increase of bright red fluorescence in HEPES buffer (20 mM, pH 7.3). Different from MBFh1, the NBCD probe is stable in buffer and cell culture media, allowing it to be used as a tool for the detection of exogenous H2O2 in HeLa cells and endogenous H2O2 in epidermal growth factor (EGF)-stimulated A431 cells.
A combination of Amplex Red and peroxidase formed another approach for indirect detection of H2O2. The enzyme catalytic oxidation of Amplex Red to resorufin led to the recovery of the fluorescence.136 Using the combination of Amplex Red and horseradish peroxidase (HRP), Zhou et al. reported an approach for the selective detection of H2O2.56 This approach allowed H2O2 detection with high sensitivity (DoL, 50 nM) and selectivity. In addition to the natural enzymes, nanomaterials with excellent enzyme-mimicking activity could promote the generation of ˙OH from H2O2,137 followed by the oxidation of Amplex Red to resorufin. Hence, coupling of Amplex Red and peroxidase-like activity mimics is a potential approach for H2O2 determination.57,58
2.3.2.2 Fluorescent probes for the detection of hypochlorous acid (HOCl).
HOCl is produced in live cells and tissues from the enzyme catalytic reaction of H2O2 with chloride ions (Cl−). The enzyme, myeloperoxidase (MPO), is mainly localized in azurophilic granules of leukocytes, including neutrophils, macrophages/monocytes, and microglia in the brain.15 HOCl is an important ROS that plays vital roles in various physiological and pathological processes.138 Therefore, detection of HOCl in living organisms is of great significance for investigating its physiological functions and disease diagnosis. Based on the protection–deprotection approach, Wang et al. developed a probe, RF1, for HOCl detection. This probe was designed by coupling of N,N-dimethylthiocarbamate recognition moieties with resorufin fluorophores (Fig. 15).139 In CH3CN/PBS solution (10 mM, 1
:
19, v/v, pH 7.4), the electrophilic addition of Cl+ of HOCl to the sulfide of the thiocarbamate group allowed the generation of resorufin, resulting in a dramatic increase of the emission intensity (∼400-fold) within 4 s. The fluorescence intensity of RF1 exhibited excellent linearity with the HOCl concentration in the range of 0–10 μM and the detection limit was determined to be 1.4 nM. The application of RF1 was demonstrated by the imaging exogenous and endogenous HOCl in HeLa and RAW 264.7 cells, respectively. Similarly a response reaction mechanism was then adopted by Choi and coworkers for the development of another carbonothioate derivative based HOCl-responsive fluorescent probe, RT-1 (Fig. 15).140 The RT-1 showed high sensitivity with a similar detection limit to RF1 (DoL, 2.18 nM).
 |
| Fig. 15 Chemical structures of resorufin-based fluorescent probes for HOCl detection. | |
2.3.3 Fluorescent resorufin probes for the detection of peroxynitrite (OONO−).
Peroxynitrite (OONO−), as one of the RNS,141–144 is associated with various diseases due to its capability in protein malfunction, lipid peroxidation and DNA damage.145 In 2012, Zhang et al. developed a coumarin derivative-based fluorescent probe, PN600 (Fig. 16), for selective detection of OONO−.146 Upon reacting with OONO−, the consumption of PN600 and the formation of the intermediate product led to the decrease and increase of the excitation intensities at 350 nm and 467 nm, respectively. On further addition of OONO− (>15 equiv. to PN600), excitation at 575 nm was observed and the intensity was increased due to the production of the resorufin derivative. Although PN600 could also respond to ClO− to form intermediate products, the second step in the oxidation process to form resorufin derivatives did not occur, which allowed PN600 to discriminate OONO− from ClO−. The application of probe PN600 was then demonstrated by fluorescence imaging of OONO− in human glioma cells (Fig. 16). By virtue of the specific reaction between boronates with OONO−,147 Odyniec et al. reported boronate-modified resorufin as the fluorescent probe (Pinkment) for selective detection of OONO− (Fig. 16).135 The probe exhibited excellent selectivity for OONO− over other ROS/RNS. On the basis of the successful development of Pinkment, a dual-responsive (AND-logic probe) fluorescent probe, Pinkment-OTBS (Fig. 16), was sequentially developed for synergistic detection of OONO− and F−.
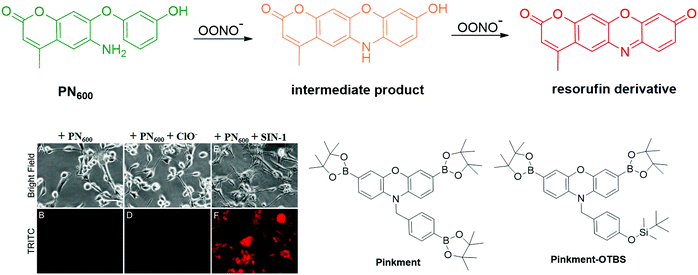 |
| Fig. 16 Chemical structures of resorufin-based fluorescent probes for the detection of OONO− and the fluorescence images of the PN600 probe for ONOO− detection in human glioma cells. Adapted with permission from ref. 146. Copyright 2012 American Chemical Society. | |
2.3.4 Fluorescent resorufin probes for the detection of other small molecules.
2.3.4.1 Fluorescent resorufin probes for the detection of hydrazine (N2H4).
Hydrazine (N2H4) is an important high-energy propellant that is widely used as a fuel in missiles and rockets. Recent investigations have revealed that N2H4 is one of the neurotoxins that cause severe mutagenic effects that damage the liver, lungs, kidneys, and the human central nervous system.148,149 Therefore, much attention has been focused on the detection of N2H4,150,151 including that using resorufin-based fluorescent probes. Using 4-bromobutyric ester as the recognition moiety, Qian et al. developed an “OFF–ON” probe, HFP, for N2H4 detection (Fig. 17).52 In CH3CN-HEPES solution (20 mM, 1
:
9, v/v, pH 7.4), the substitution–cyclization–elimination reaction between HFP and N2H4 led to the cleavage of ester bonds. A distinct color change of the solution from pale yellow to pink and a 16-fold enhancement of the emission intensity at 584 nm were observed for colorimetric and fluorescence detection of N2H4. The application of HFP was then demonstrated by fluorescence imaging of N2H4 in Chinese hamster ovary (CHO) cells. Tang and coworkers found that probe 13 is able to respond to N2H4 through a nucleophilic addition–elimination reaction between N2H4 and 2-fluoro-5-nitrobenzoic ester moieties (Fig. 13).152 The fluorescence of probe 13 was recovered within 30 min after the addition of N2H4 in CH3CN-PBS buffer (10 mM, 3
:
7, v/v, pH 7.4). In addition, probe 7 was also found to be able to detect N2H4 in DMSO–Tris solution (10 mM, 1
:
1, v/v, pH 8.0) by Choi and coworkers (Fig. 9).153 Upon addition of N2H4, the hydrazinolysis of phenol acetates resulted in the formation of resorufin, accompanied by the fluorescence enhancement at 595 nm by 47-fold (DoL, 8.2 × 10−7 M). Recently, an upconversion nanoparticle (UCNP) based nanoprobe has been developed by Reddy's group for the detection of N2H4 in water.154 The probe was designed by loading resorufin propionate (RePr) onto the surface of UCNPs through the host–guest interaction.155 In this luminescence resonance energy transfer (LRET) process, the emission of UCNPs (donor) at 542 nm was decreased due to the energy transfer from UCNPs to resorufin that was produced after the reaction between N2H4 and RePr. Using the emission of UCNPs at 602 nm as the reference, the ratio of I542/I602 showed a good linearity to the N2H4 concentration in the range of 0–40 μM (DoL, 35.5 μM).
 |
| Fig. 17 Chemical structure of HPF and its response mechanism for N2H4 detection. | |
2.3.4.2 Fluorescent resorufin probes for the detection of formaldehyde (FA).
Formaldehyde (FA) has been primarily treated as a highly toxic compound and carcinogen to living organisms, while recent studies demonstrated that FA could be endogenously generated during many essential biological processes.156 FA has also been recognized as one of the key hallmarks for various diseases, such as cancer.22,157,158 By protecting the hydroxyl group of resorufin with a homoallylamine aza-Cope trigger and a self-immolative linker, Bruemmer et al. designed and synthesized a responsive probe, FAP573 (Fig. 18), for fluorescence detection of FA.159 The reaction between the O-alkylated resorufin and FA via the 2-aza-Cope rearrangement resulted in the rearranged imine, followed by the elimination of the two carbon linkers through a β-elimination process.157 After the reaction of FAP573 with FA in PBS buffer (20 mM, pH 7.4) for 2 h, the fluorescence intensity at 585 nm showed about 4-fold enhancement. The probe was then used as a tool for the monitoring of the FA metabolism regulated by alcohol dehydrogenase 5 (ADH5) in ADH5 KO cells (Fig. 18).
 |
| Fig. 18 Chemical structure of FAP573 and its confocal microscopy images in response to FA metabolism in ADH5 KO (a and b) or wild-type WT (c and d) HAP1 cells. Cells were treated with FAP573, followed by vehicle (b and d) or FA (a and c). Adapted with permission from ref. 159. Copyright 2017 American Chemical Society. | |
2.3.4.3 Fluorescent resorufin probes for the detection of nitroxyl (HNO).
Nitroxyl (HNO), as one of the RNS, is the one-electron reduced derivative of NO.160 This biomolecule is produced either by the thiol-mediated reduction of NO or enzymatic reduction of L-arginine by nitric oxide synthase (NOS).161,162 It has been reported that HNO plays important roles in early diagnosis and treatment of various diseases, such as cardiovascular disorder and myocardial ischemia-reperfusion injury.163 To detect this biomolecule, several responsive fluorescent probes have been reported,130,162 including those of resorufin-based fluorescent probes. For example, on the basis of the widely used reaction (analogous to the Staudinger reaction) between HNO and phosphine, Bobba et al. reported a resorufin fluorophore based probe, 17 (Fig. 19), for the detection of HNO.164 After incubation of probe 17 with HNO for 30 min, the phosphorus group of the probe could be readily cleaved to form resorufin. As a result, dramatic increases of UV-vis (18-fold) and fluorescence intensity (30-fold) were obtained for colorimetric and fluorescence detection of HNO. The probe with a low detection limit (DoL, 20 nM) was then utilized as a tool for the imaging of HNO in CHO cells and nematodes.
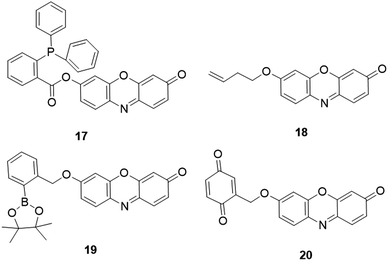 |
| Fig. 19 Chemical structures of resorufin-based fluorescent probes for HNO, O3, BPO and glucose detection, respectively. | |
2.3.4.4 Fluorescent resorufin probes for the detection of ozone (O3).
Ozone (O3) is one of the highly reactive oxygen species (hROS) that has a very short half-life of 66 s and has a very low concentration in living organisms.165,166 Recent research revealed that cholesterol ozonolysis products were present in clinical brain samples, which might induce misfolding of proteins in amyloid disease.167 Using but-3-enyl as a recognition group, Zhang et al. developed a resorufin-based probe, 18 (Fig. 19), for selective sensing of O3.168 Upon the specific reaction between O3 and but-3-enyl moiety for 40 min, the probe was converted to resorufin. The probe exhibited a high signal-to-noise ratio (207-fold) and a low detection limit (5.9 nM) for O3 detection in PBS buffer (50 mM, pH 7.4). The practical application of the probe was then demonstrated for the detection of O3 in real ambient air samples and HeLa cells.
2.3.4.5 Fluorescent resorufin probes for the detection of benzoyl peroxide (BPO).
Benzoyl peroxide (BPO) is a highly reactive oxidizing agent that has been widely used in various fields, such as a food additive to flour for bleaching and antisepsis.169–171 This oxidizing agent has also attracted increasing attention in life science due to its genotoxic effects on human peripheral lymphocytes.172 To detect this oxidizing agent, Chen et al. developed a fluorescent probe, 19 (Fig. 19), by coupling boronate (recognition moiety) with resorufin (reporter).173 In the presence of BPO, the deboronation of BPO led to the formation of resorufin, which resulted in a dramatic increase of the fluorescence intensity within 15 min. In ethanol-PBS buffer (20 mM, 1
:
9, v/v, pH 7.4), the enhancement of fluorescence intensity showed a good linearity to the concentration of BPO in the range of 0.5–26 μM with a low detection limit (DoL, 23 nM) for BPO detection.
2.3.4.6 Fluorescent resorufin probes for the detection of glucose.
Quantitate determination of glucose is of importance for the diagnosis of diabetes mellitus. Taking advantage of the enzyme-coupling reaction of DT-diaphorase (DTD), Gao and coworkers reported a fluorescent probe, 20 (Fig. 19), for the determination of glucose.174 In the presence of glucose dehydrogenase, the glucose was oxidized to NAD+ and a sequent proton was obtained to form nicotinamide adenine dinucleotide (NADH). The reaction between NADH and O-alkylated resorufin resulted in the cleavage of the benzoquinone group to yield the resorufin dye. The reaction led to a 16-fold enhancement of fluorescence at 585 nm. Using 20 as a probe, a detection limit of 0.2 μM was obtained in PBS buffer (100 mM, pH 7.4). The probe was then successfully utilized for the detection of glucose in human urine samples. Chih et al. reported a fluorescent glucose probe using the mixture of montmorillonite K10 clay mineral (Mont. K10), Amplex Red, and glucose oxidase (GOx).175 Upon the addition of glucose, the intermediate H2O2 was generated in the GOx region and further transformed to superoxide anion radicals by clay, which could react with Amplex Red to afford resorufin. The detection limit of this probe for glucose was determined to be 150 μM.
2.4 Fluorescent resorufin probes for detection of biomacromolecules
2.4.1 Fluorescent resorufin probes for the detection of DNA/RNA.
Nucleic acid (DNA/RNA) detection has been recognized as one of the most widely used tools in disease diagnosis and treatment monitoring. As an important reporter, fluorescent resorufin has also been used for the development of responsive probes for nucleic acid detection. For example, using the morpholino-modified magnetic microparticles, Hu et al. designed and prepared a probe for sequence-specific determination of DNA (Fig. 20).176 The biotin-labeled signal DNA could hybridize with the target DNA hybridized on the surface of morpholino microparticles. After the binding of streptavidin-alkaline phosphatase (SA-ALP) through specific SA-biotin binding, the formed nanoparticle could catalyze the hydrolyzation of L-ascorbic acid-2-phosphate (AAP) to produce L-ascorbic acid. The redox process between L-ascorbic acid and resazurin triggered the generation of resorufin, accompanied by a prominent fluorescence enhancement. The enhancement of fluorescence intensity of the probe exhibited a good linearity with the increase of DNA concentration from 0.1 pM to 0.1 nM and the detection limit was estimated to be 9.32 fM. The practical application of the probe was demonstrated in the detection of DNA in serum. Wang et al. designed and prepared two noncanonical DNA structure based Amplex Red oxidases, which could convert Amplex Red to resorufin without the aid of H2O2.177 The probe was used for the determination of microRNA relying on the amplification of the fluorescence signal derived from the oxidation of Amplex Red. The detection limit was calculated to be 2.0 pM.
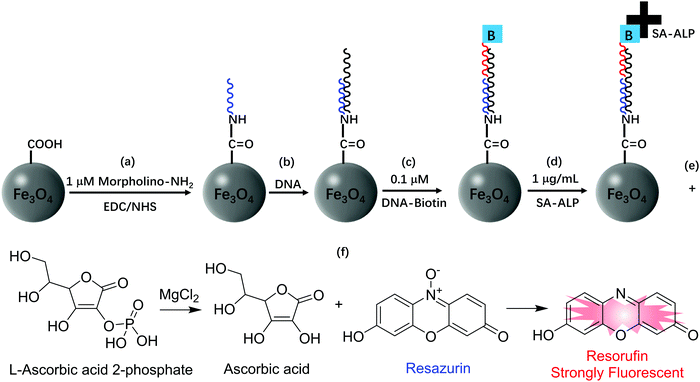 |
| Fig. 20 Schematic diagram of the magnetic microsphere functionalization and fluorescence turn-on response for DNA detection. (a) Modification of magnetic microspheres with morpholino, (b) hybridization of DNA with the morpholino probe, (c) hybridization of biotin-labeled signal probe DNA with the NDA nearby the 5′ end of sequence, (d) through biotin–streptavidin interaction, the SA-ALP was conjugated with signal probe DNA, (e) AAP was hydrolyzed by ALP to generate L-ascorbic acid, (f) L-ascorbic acid reduced resazurin to resorufin which is strongly fluorescent. | |
2.4.2 Fluorescent resorufin probes for the detection of enzyme activity.
The biotransformation of numerous chemicals and biomolecules in living organisms is catalyzed by various enzymes with diverse structures, such as oxidoreductases, transferases, hydrolases, lyases, isomerases, and ligases.178 For example, as one kind of hydrolase, carboxylesterases in endoplasmic reticulum and cytosol of various cells can catalyze the hydrolysis of carboxylic esters for the detoxification and metabolism of many drugs.179 Alterations of enzyme activity can lead to a number of different diseases. Detection of enzyme activity in organisms is thus of great importance for investigating their physiological function and disease diagnosis. In the past few decades, increasing attention has been devoted to developing fluorescent probes for the detection and imaging of enzymes in living systems,180–182 including resorufin-based probes.
Based on the protection–deprotection approach of 7-hydroxy groups, various resorufin-based fluorescent probes have been reported for enzyme analysis. For example, the Hakamata group developed a probe, 21 (Fig. 21), for selective detection and imaging of carboxylesterase activity.183 In the presence of carboxylesterase in PBS buffer of pH 8.0 at 37 °C, the carboxylic ester bond was cleaved via the hydrolysis reaction, followed by the spontaneous cleavage of quinone methide moieties to afford resorufin. The probe was then used for carboxylesterase activity imaging and inhibitor screening at the cellular level. Similarly, Zhang and coworkers reported another carboxylesterase probe, 22 (Fig. 21), by coupling the p-acetoxyphenylmethyloxy into resorufin.184 Upon addition of carboxylesterase, the carboxylesterase-mediated hydrolysis of the carboxylic ester led to the cleavage of the p-acetoxyphenylmethyloxy group and formation of resorufin, accompanied by a dramatic increase of the fluorescence intensity (321-fold). The probe with a detection limit of 8.6 × 10−5 U mL−1 was then used as a tool for the detection and imaging of carboxylesterase activity in HeLa cells.
 |
| Fig. 21 Chemical structures of fluorescent probes, 21 and 22, for carboxylesterase activity analysis. | |
Taking advantage of 3-hydroxyphenyl as the recognition group, Wu and coworkers designed and synthesized a probe, 23 (Fig. 22), for tyrosinase analysis.185 The tyrosinase-catalyzed m-hydroxyphenyl group in the probe promoted the hydroxylation at the adjacent 4-position, followed by the oxidative 1,6-rearrangement–elimination to yield resorufin. The probe exhibited high sensitivity and selectivity towards tyrosinase with a detection limit of 0.04 U mL−1. Practical application was investigated for monitoring endogenous tyrosinase activity in B16, HepG2 and MCF-7 cells. Li et al. developed another tyrosinase probe, 24 (Fig. 22), using m-tolylboronic acid pinacol ester as the recognition group.186 In the presence of tyrosinase, a similar response reaction occurred to switch “ON” the fluorescence for tyrosinase analysis. The emission intensity of 24 presented a good linear response towards tyrosinase in the range of 1–100 U mL−1, and the detection limit was determined to be 0.5 U mL−1. The fluorescence imaging of tyrosinase was evaluated in B16 cells. A remote lighted controlled fluorescent probe, PTFP (Fig. 22), was recently developed by Yang's group for more accurate and precise detection of tyrosinase in buffer and live cells.187 The FTFP probe was developed by protecting the free hydroxyl group of probe 23 with a photoactivatable 2-nitrobenzyl group.188 Interestingly, FTFP can be activated by UV light irradiation to afford probe 23 for tyrosinase detection. FTFP showed high selectivity and sensitivity (DoL, 0.08 U mL−1), allowing it to be used for photo-controlled imaging of tyrosinase in live B16 cells.
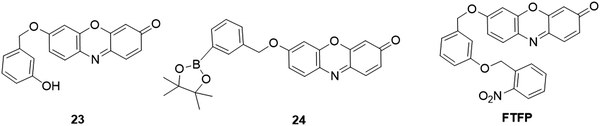 |
| Fig. 22 Chemical structures of fluorescent probes, 23, 24, and FTFP, for the analysis of tyrosinase activity. | |
Shen et al. reported a fluorescent probe, probe R (Fig. 23), for the detection of microsomal epoxide hydrolase.189 In the presence of epoxide hydrolase, the hydrolysis of the epoxide unit in the probe afforded a diol product. The diol product was able to be further converted to aldehyde with the aid of sodium periodate. Through a fast β-elimination process, resorufin was produced with 56- (pH 6.0), 80- (pH 8.4), and 55-fold (pH 10.0) enhancement in fluorescence, respectively. Smith et al. reported a fluorescent probe, RS (Fig. 23), for the detection of the sulfatase activity.190 The sulfatase hydrolysis resulted in the formation of resorufin and prominent increase of the emission quantum yield (from ≤0.01 to 0.74). Such fluorescence increases allowed for the detection of sulfatase activity. Yoshiya et al. developed a fluorescent probe, LISA-101 (Fig. 23), for the detection of γ-glutamyl cyclotransferase (GGCT) activity.191 Upon GGCT treatment, the hydroxylation of amino acids (Xaa) resulted in the intramolecular chemical cyclization reaction to release resorufin. The probe was used to investigate the GGCT activities in MCF-7 and NHDF cells in Tris buffer of pH 8 at 37 °C. Using resorufin glycosides as substrates, Ibatullin et al. developed an approach for the detection of glycoside hydrolase (GH) activity.192 To highlight the capacity of these substrates in cell wall morphological studies, a xylogluco-oligosaccharide (XGO) resorufin β-glycoside, XXXG-β-Res (Fig. 23), was prepared and successfully applied for in vivo fluorescence imaging of xyloglucan endohydrolase (XEH) activity in plant tissues from nasturtium (Tropaeolum majus) and Arabidopsis (Arabidopsis thaliana).
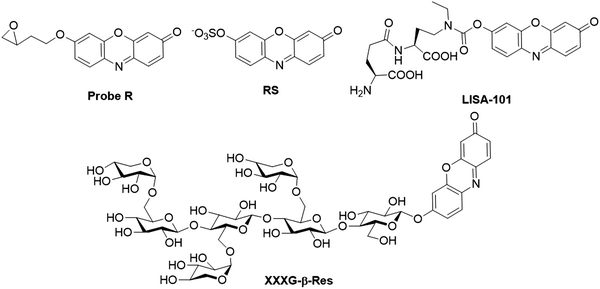 |
| Fig. 23 Chemical structures of resorufin-based fluorescent probes, Probe R, RS, LISA-101, and XXXG-β-Res, for the analysis of microsomal epoxide hydrolase, sulfatase, γ-glutamyl cyclotransferase and glycoside hydrolase activity, respectively. | |
By coupling 5-nitrofuran to resorufin via an ether bond, Li et al. designed and synthesized a fluorescent probe, 25 (Fig. 24), for the analysis of nitroreductase activity.47 Upon the addition of nitroreductase, the nitro moiety of the probe was reduced with the assistance of nicotinamide adenine dinucleotide (NADH). Through a sequent spontaneous 1,6-rearrangement elimination process, the resorufin was produced, accompanied by a distinct enhancement of the emission intensity (100-fold). The fluorescence response showed a good linearity with a concentration of nitroreductase in the range of 15–300 ng mL−1 and the detection limit for nitroreductase was determined to be 0.27 ng mL−1. The probe was successfully applied for the monitoring of the hypoxic status in HeLa and A549 cells via indirect detection of nitroreductase. Later, replacing the furan with thiophene, another nitroreductase probe, 26 (Fig. 24), was developed by the same group.193 Owing to the stronger electron-donating property of the S atom, the probe exhibited lower fluorescence at 585 nm with a quantum yield of ∼0.01, four times smaller than that of 25. After the reaction of probe 26 with nitroreductase at 37 °C for 20 min in PBS buffer (10 mM, pH 7.4, containing 50 mM NADH), significant enhancement in fluorescence of resorufin was obtained. The detection limit for nitroreductase was estimated to be 0.1 ng mL−1. Similarly, Yoon and coworkers reported two “OFF–ON” nitroreductase probes, 27 and 28, using p-nitrobenzyl as the recognition unit and ether and carbonate as linkers, respectively (Fig. 24).49 In comparison with 28, 27 showed higher selectivity and faster response time, which allowed it to be further applied for nitroreductase activity detection in bacteria.
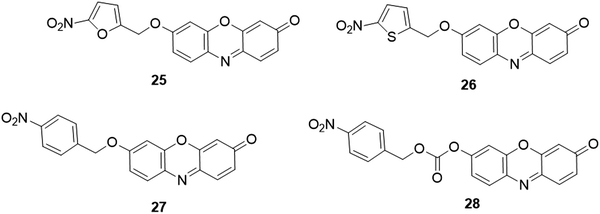 |
| Fig. 24 Chemical structures of fluorescent probes, 25–28, for the analysis of nitroreductase activity. | |
Wang and coworkers reported a fluorescent probe, Resazurin (Fig. 25), for the analysis of alkaline phosphatase (ALP) activity.194 In the presence of ALP, L-ascorbic acid 2-phosphate (AAP) was converted to L-ascorbic acid, which could reduce Resazurin to resorufin. As a result, a 7-fold increase of the emission intensity was obtained for the ALP analysis. The probe presented a low detection limit (0.12 mU mL−1), facilitating it to be utilized for monitoring ALP activity in biological samples. Another ALP probe, 29 (Fig. 25), was developed by the Zhang group using a phosphate group as the recognition unit and a reactive p-hydroxybenzyl group as the linker.195 The probe exhibited weak fluorescence (quantum yield ∼0.0023) due to the effective quenching effect of 7-hydroxy substitution through PET. In the presence of ALP, the phosphate group was eliminated through the dephosphorylation reaction catalysed by ALP. Then, a 1,6-elimination of the p-hydroxybenzylether derivative occurred to form the fluorescent resorufin. After reaction of the probe with ALP for 20 min, the fluorescence intensity was notably enhanced (DoL, 1.09 U L−1). Using this ALP-responsive probe, endogenous ALP activity in HeLa cells was then investigated. Using a similar response reaction mechanism, Biswas et al. developed two phosphorylated resorufin based fluorescent probes, pRes and F2pRes (Fig. 25), for sensing tyrosine phosphatase (PTP) activity.196 Upon dephosphorylation of PTP at acidic and neutral pH, both of the probe exhibited a prominent increase in the fluorescence intensity and a distinct color change from pale orange to pink. The practical application of the probes was then demonstrated for the analysis of PTP activity in HeLa cells.
 |
| Fig. 25 Chemical structures of fluorescent probes, Resazurin, 29, and pRes and F2pRes for the analysis of phosphatase activity. | |
As discussed above, probe 7 (Fig. 9) was designed for fluorescence and colorimetric detection of BO3−. Recent studies showed that the acylation of the hydroxyl group in probe 7 is sensitive to esterases, where the acetate group could be cleaved to yield fluorescent resorufin. As a result, 7 was used for the determination of horse serum cholinesterase by the Kramer group.197 However, the weak stability to hydrolysis severely restricted its practical applications. Using acetoxymethyl ether instead of the acylating group as the recognition moiety, Lavis and coworkers then reported three resorufin-based probes, 30, 31, and 32 for the analysis of esterase (Fig. 26).198 Compared with probe 30, probe 31 possessed good cell membrane permeability, which enabled probe 31 for fluorescence imaging of esterase in HeLa cells. Through introducing arachidonic acid (AA) onto 3,7-dihydroxyphenoxazine, Yadav and coworkers reported the isoform-selective activity-based fluorescent probe, CoxFluor (Fig. 26), for detection of cyclooxygenase-2 (COX-2).199 After reaction with the cyclooxygenase active moiety, the hydrogen atom in the probe was abstracted by enzyme's Tyr385 residue, and subsequent dioxygenation and cyclization to form the CoxFluor-PGG2, which could be oxidized by the π cationic porphyrin radical or oxyferryl to induce a labile oxygen-centered resorufin radical. Then, the fluorescent resorufin was formed with the dismutation and amide cleavage process. The fluorescence intensity was enhanced by 41-fold upon response to recombinant human COX-2. Fluorescence imaging studies of the probe were conducted to monitor the endogenous COX-2 activity in RAW 264.7 cells.
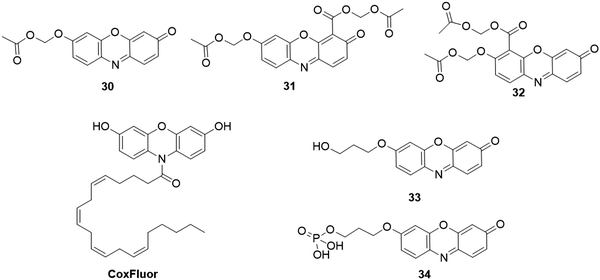 |
| Fig. 26 Chemical structures of resorufin-based fluorescent probes for esterases, cyclooxygenase-2, acetylcholinesterase, alcohol dehydrogenases and protein serine/threonine phosphatase activity analysis. | |
Using 3 (Fig. 7) as the probe, Cui et al. reported a method for assessing the acetylcholinesterase (AChE) activity.200 AChE-mediated hydrolysis of acetylthiocholine iodide (ATC, a substrate of AChE) afforded thiocholine, which could subsequently react with probe 3 to remove the DBS moiety. The fluorescence intensity at 585 nm was increased by 7-fold and the color of the solution was obviously changed from colorless to pink after the incubation of 3 and AChE for 30 min in PBS buffer (10 mM, 0.5% DMSO, pH 8.0). Using a primary alcohol and a phosphate moiety as the sensing group, Goncalves and coworkers designed and synthesized two fluorescent probes, 33 and 34 (Fig. 26), for the detection of alcohol dehydrogenases (ADHs) and protein serine/threonine phosphatase (PSTP) activity, respectively.201In vitro enzymatic analysis results revealed that the analogous alcohols in 33 could be oxidized by different commercial ADHs to release the resorufin dye. The calcineurin-catalyzed phosphate hydrolysis reaction of probe 34 induced the generation of probe 33, which was confirmed with the malachite green reagent. Furthermore, the multi-enzymatic cascade associated with phosphatase and alcohol dehydrogenase was investigated by probe 34. The practical application was conducted to evaluate ADH and phosphatase activity in normal and cancer cells via flow cytometry and confocal microscopy experiments.
β-Lactamases (Bla) belong to the family of bacterial enzymes that play crucial role in catalyzing the cleavage of penicillins and cephalosporins. Hence the accurate and rapid determination of Bla activity in biological samples is essential in clinical treatment.202 Gao et al. reported a fluorescent probe, CR2 (Fig. 27), for the detection and imaging of Bla.203 The probe exhibited good stability in PBS buffer with a half-life of ∼182 h. Upon reaction with Bla, a 42-fold enhancement in fluorescence was observed, which was recorded for fluorescence analysis of Bla. After modification with bromomethyl acetate, a membrane-permeable acetoxymethyl ester of CR2 (CR2/AM) was used as a tool for investigating the Bla activity in C6 glioma cells (Fig. 27).
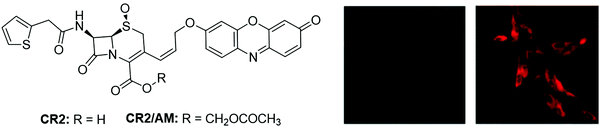 |
| Fig. 27 Chemical structures of CR2 and CR2/AM and the fluorescence images of wild-type (left) and Bla-stably transfected (right) C6 glioma cells stained with CR2/AM. Adapted with permission from ref. 203. Copyright 2003 American Chemical Society. | |
Cytochrome P450 (CYP450) is a significant enzyme that is located in the inner mitochondrial membrane. This enzyme is mainly involved in the metabolism of various xenobiotics.204 For CYP450 activity analysis, many resorufin-based fluorescent probes have been reported. The Marrone group developed a fluorescent probe, 35 (Fig. 28), for the detection of CYP450scc.46,205 This probe was designed by incorporating a cholene-based steroid structure to the hydroxyl of resorufin. The reaction between 35 and CYP450scc resulted in the cleavage of ether side chains to yield resorufin. The probe was applied for the determination of CYP450scc activity in mitochondrial preparations from chicken granulosa and MA-10 cells. Using resorufin benzyl ether 36 (Fig. 28) as a fluorescent enzyme substrate, Nuchtavorn et al. developed a paper-based sol–gel-derived device for the detection of CYP3A4 (CYP450 isozymes) activity.206 Quantitative fluorescence signals could be obtained through a handheld digital microscope and an image analysis software. This portable paper-based approach featured a low detection limit (0.35 μM) and favourable precision (RSDs < 4.1%) for CYP3A4 detection. Further application of the platform for evaluating the CYP3A4 activity was carried out in several plant extracts. Using cholesterol-resorufin (3b-hydroxy-22,23-bisnor-5-cholenyl ether) as a fluorescent probe, Homma et al. investigated the diverse cytochrome P450scc activity stimulated by NADPH, ACTH and ATP in adrenocortical cells.207 The scanning laser cytometry technique was exploited by Sidhu et al. for direct sensing P4501A1 (CYP450 isozymes)-mediated O-dealkylation activity.208 Enzyme activities of P-4501A1 and NADPH DT-diaphorase were evaluated in human hepatoma (Hep G2) cells, mouse (Hepa 1clc7 wt) and rat (H4-II-E) hepatomas.
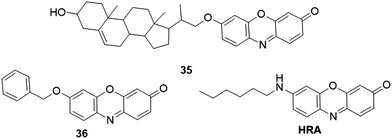 |
| Fig. 28 Chemical structures of resorufin-based fluorescent probes for cytochrome P450 activity analysis and ER imaging. | |
2.4.3 Fluorescent resorufin probes for the detection of protein.
As mentioned above, nanomaterials endowed with peroxidase-like activity can convert Amplex Red to resorufin in the presence of H2O2.57,58,137 Nevertheless, the catalytic activities of nanomaterials can be regulated reversibly by target analytes, which could be treated as a promising approach for the detection of target analytes involved in the related processes. By assembling aptamer-modified gold nanoparticles (Apt-AuNPs) on bismuth oxychloride (BiOCl) nanosheets, Hsu et al. reported Apt-AuNP/BiOCl nanocomposites equipped with tunable peroxidase-like activity for protein detection.57 The interaction between the aptamer units (Del-5-1 and v7t-1) and vascular endothelial growth factor-A165 (VEGF-A165) molecules inhibited the catalytic activity of Apt-AuNPs/BiOCl nanocomposites. Therefore, the system of AR/H2O2-Apt-AuNPs/BiOCl nanocomposites could be employed as a probe for the detection of VEGF-A165. The nanosystem exhibited excellent selectivity (>1000-fold over interferences) and sensitivity (detection limit ∼0.5 nM) for VEGF-A165, allowing the probe for the detection of VEGF in biological samples. Similarly, Wu and coworkers reported another aptamer-modified copper oxidenanorods (Apt-Cu NPs) with tunable peroxidase catalytic activity for thrombin recognition.58 The detection limit of the probe was determined to be 0.5 nM in serum samples and was applied for the detection of Mucin1-overexpressing tumor cells.
2.5 Other applications of resorufin-based probes
Fluorescent proteins are widely used fluorophores in biosensing and the bioimaging research field. The PRIME (Probe Incorporation Mediated by Enzymes) technique is considered as a promising method for realizing the labeling specificity comparable to fluorescent proteins.209,210 Liu and coworkers used resorufin as the fluorophore for engineering red ligase for the site-specific protein, which was applied in super-resolution imaging of the intermediate filament protein vimentin.34 As a significant subcellular organelle, endoplasmic reticulum (ER) is involved in the processing of secreted and transmembrane proteins. Using an amino analogue of resorufin, Phaniraj et al. developed a hydrophobic fluorescent probe, HRA (Fig. 28), for specific imaging of ER.211 The probe showed strong fluorescence in organic solvents (ϕ = 0.70) and could selectively localize in the ER of HeLa cells at low concentration (100 nM). The probe was applied for monitoring small molecule-regulated release of a GFP-GPI fusion protein from ER to the secretory pathway.
3. Conclusion
This review article outlined the recent advances in the development of resorufin-based probes for the detection of various analytes, such as metal ions, anions, small biomolecules, and biomacromolecules. It is clear that the fluorescence properties of resorufin could be finely regulated through the protection–deprotection process of 7-hydroxy group and substituent of N atoms of resorufin. These two approaches have thus been widely adopted for the construction of responsive probes for fluorescence and colorimetric detection of diverse analytes highlighted above. Owing to the excellent spectroscopic properties of resorufin and the corresponding responsive probes, including high photostability, good sensitivity and selectivity, applications of the majority of these fluorescent probes have been well demonstrated by detecting the levels of various target analytes in practical samples, such as environmental and biological systems.
Despite the rapid progress in the development of resorufin-based responsive probes, continuous efforts are still expected in the near future to develop new fluorescent probes for biological and environmental analysis. First, most of the resorufin-based responsive probes were developed using the protection–deprotection mechanism of 7-hydroxy group (first approach, Fig. 1B). Until now the second approach of N atom substitution has rarely been used in the design of responsive resorufin probes but it has been proved to be a very powerful approach by the development of Amplex Red,56 Pinkment-OAc,135 NBCD,59 Pinkment,135 Pinkment-OTBS,135 and CoxFluor.199 Second, although resorufin has high water solubility (1 mg mL−1), most of the resorufin-based responsive fluorescent probes have low water solubility. As a result, organic solvents are required as a co-solvent for the fluorescence and colorimetric analysis. The use of organic solvents severely restricted the wide usage in analysis, especially for the analysis required in situ in biological systems. Third, most of the resorufin-based probes showed typical “OFF–ON” fluorescence and a yellow to pink color response to target analytes, while few resorufin probes could be used for ratiometric analysis. Ratiometric fluorescence resorufin probes can be developed by exploiting the mechanism of Förster resonance energy transfer (FRET), where resorufin could serve as either an energy donor or an acceptor. As another result of this FRET mechanism-based resorufin probe, the difference between the probe's excitation and emission wavelengths could be enlarged, addressing the limitation of the small Stokes shift of resorufin (13 nm). Lastly, despite resorufin being widely used in the development of responsive probes, probes that can detect other biological species, such as ROS/RNS (e.g. ˙OH, 1O2, O2˙−, NO), have rarely been reported. In summary, fluorescent probes that selectively respond to diverse biological analytes with good water solubility, high accuracy and sensitivity are highly needed. It is expected that this article will contribute to the future development of new responsive probes for target analyte detection and facilitate their practical application in biological and environmental samples.
Conflicts of interest
There are no conflicts to declare.
Acknowledgements
Financial support from the National Natural Science Foundation of China (Grant No. 21804067 and 21806066), Australian Research Council (DE170100092), National Health and Medical Research Council (APP1175808), Shandong Province Natural Science Foundation (No. ZR2017BB070) and the Primary Research and Development Plan of Shandong Province (No. 2019GSF108093) is gratefully acknowledged.
References
- C. Nathan, Specificity of a third kind: reactive oxygen and nitrogen intermediates in cell signaling, J. Clin. Invest., 2003, 111(6), 769–778 CrossRef CAS
.
- S. H. Park, N. Kwon, J. H. Lee, J. Yoon and I. Shin, Synthetic ratiometric fluorescent probes for detection of ions, Chem. Soc. Rev., 2020, 49(1), 143–179 RSC
.
- X. Chen, Y. Zhou, X. Peng and J. Yoon, Fluorescent and colorimetric probes for detection of thiols, Chem. Soc. Rev., 2010, 39(6), 2120–2135 RSC
.
- K. Brieger, S. Schiavone, F. J. Miller Jr and K. H. Krause, Reactive oxygen species: from health to disease, Swiss Med. Wkly., 2012, 142, w13659 CAS
.
- J. Chan, S. C. Dodani and C. J. Chang, Reaction-based small-molecule fluorescent probes for chemoselective bioimaging, Nat. Chem., 2012, 4(12), 973–984 CrossRef CAS
.
- X. P. He, X. L. Hu, T. D. James, J. Yoon and H. Tian, Multiplexed photoluminescent sensors: towards improved disease diagnostics, Chem. Soc. Rev., 2017, 46(22), 6687–6696 RSC
.
- X. P. He, Y. Zang, T. D. James, J. Li and G. R. Chen, Probing disease-related proteins with fluorogenic composite materials, Chem. Soc. Rev., 2015, 44(13), 4239–4248 RSC
.
- J. Yin, Y. Hu and J. Yoon, Fluorescent probes and bioimaging: alkali metals, alkaline earth metals and pH, Chem. Soc. Rev., 2015, 44(14), 4619–4644 RSC
.
- J. Pranczk, D. Jacewicz, D. Wyrzykowski and L. Chmurzynski, Analytical methods for determination of reactive oxygen species, Curr. Pharm. Anal., 2014, 10(4), 293–304 CrossRef CAS
.
- N. Burford, M. D. Eelman, D. E. Mahony and M. Morash, Definitive identification of cysteine and glutathione complexes of bismuth by mass spectrometry: assessing the biochemical fate of bismuth pharmaceutical agents, Chem. Commun., 2003, 146–147 RSC
.
- H. Kobayashi, M. R. Longmire, M. Ogawa and P. L. Choyke, Rational chemical design of the next generation of molecular imaging probes based on physics and biology: mixing modalities, colors and signals, Chem. Soc. Rev., 2011, 40(9), 4626–4648 RSC
.
- R. Zhang, J. Yong, J. Yuan and Z. P. Xu, Recent advances in the development of responsive probes for selective detection of cysteine, Coord. Chem. Rev., 2020, 408, 213182 CrossRef CAS
.
- C. J. Chang, T. Gunnlaugsson and T. D. James, Imaging agents, Chem. Soc. Rev., 2015, 44(14), 4484–4486 RSC
.
- L. Tian, H. Ma, B. Song, Z. Dai, X. Zheng, R. Zhang, K. Chen and J. Yuan, Time-gated luminescence probe for ratiometric and luminescence lifetime detection of hypochorous acid in lysosomes of live cells, Talanta, 2020, 212, 120760 CrossRef CAS
.
- R. Zhang, B. Song and J. Yuan, Bioanalytical methods for hypochlorous acid detection: recent advances and challenges, Trends Anal. Chem., 2018, 99, 1–33 CrossRef CAS
.
- M. Kaur and D. H. Choi, Diketopyrrolopyrrole: brilliant red pigment dye-based fluorescent probes and their applications, Chem. Soc. Rev., 2015, 44(1), 58–77 RSC
.
- Z. Tang, H. Chen, H. He and C. Ma, Assays for alkaline phosphatase activity: progress and prospects, Trends Anal. Chem., 2019, 113, 32–43 CrossRef CAS
.
- H. Xiao, W. Zhang, P. Li, W. Zhang, X. Wang and B. Tang, Versatile fluorescent probes for imaging the superoxide anion in living cells and in vivo, Angew. Chem., Int. Ed., 2020, 59(11), 4216–4230 CrossRef CAS
.
- A. L. Berhanu, I. Mohiuddin, A. K. Malik, J. S. Aulakh, V. Kumar and K.-H. Kim, A review of the applications of Schiff bases as optical chemical sensors, Trends Anal. Chem., 2019, 116, 74–91 CrossRef CAS
.
- L. Wang, W. Du, Z. Hu, K. Uvdal, L. Li and W. Huang, Hybrid rhodamine fluorophores in the visible/NIR region for biological imaging, Angew. Chem., Int. Ed., 2019, 58(40), 14026–14043 CrossRef CAS
.
- D.-L. Ma, V. P.-Y. Ma, D. S.-H. Chan, K.-H. Leung, H.-Z. He and C.-H. Leung, Recent advances in luminescent heavy metal complexes for sensing, Coord. Chem. Rev., 2012, 256(23–24), 3087–3113 CrossRef CAS
.
- C. Liu, R. Zhang, W. Zhang, J. Liu, Y. L. Wang, Z. Du, B. Song, Z. P. Xu and J. Yuan, “Dual-key-and-lock” ruthenium complex probe for lysosomal formaldehyde in cancer cells and tumors, J. Am. Chem. Soc., 2019, 141(21), 8462–8472 CrossRef CAS
.
- Z. Du, B. Song, W. Zhang, C. Duan, Y. L. Wang, C. Liu, R. Zhang and J. Yuan, Quantitative monitoring and visualization of hydrogen sulfide in vivo using a luminescent probe based on a ruthenium(II) complex, Angew. Chem., Int. Ed., 2018, 57(15), 3999–4004 CrossRef CAS
.
- L. Shang, S. Dong and G. U. Nienhaus, Ultra-small fluorescent metal nanoclusters: synthesis and biological applications, Nano Today, 2011, 6(4), 401–418 CrossRef CAS
.
- Y. Zheng, L. Lai, W. Liu, H. Jiang and X. Wang, Recent advances in biomedical applications of fluorescent gold nanoclusters, Adv. Colloid Interface Sci., 2017, 242, 1–16 CrossRef CAS
.
- Q. Zhou, Y. Lin, M. Xu, Z. Gao, H. Yang and D. Tang, Facile synthesis of enhanced fluorescent gold–silver bimetallic nanocluster and its application for highly sensitive detection of inorganic pyrophosphatase activity, Anal. Chem., 2016, 88(17), 8886–8892 CrossRef CAS
.
- J. D. Schiffman and R. G. Balakrishna, Quantum dots as fluorescent probes: synthesis, surface chemistry, energy transfer mechanisms, and applications, Sens. Actuators, B, 2018, 258, 1191–1214 CrossRef
.
- S. Sarkar, P. Le, J. Geng, Y. Liu, Z. Han, M. U. Zahid, D. Nall, Y. Youn, P. R. Selvin and A. M. Smith, Short-wave infrared quantum dots with compact sizes as molecular probes for fluorescence microscopy, J. Am. Chem. Soc., 2020, 142(7), 3449–3462 CrossRef CAS
.
- S. Lv, F. Chen, C. Chen, X. Chen, H. Gong and C. Cai, A novel CdTe quantum dots probe amplified resonance light scattering signals to detect microRNA-122, Talanta, 2017, 165, 659–663 CrossRef CAS
.
- A. C. Sedgwick, L. Wu, H.-H. Han, S. D. Bull, X.-P. He, T. D. James, J. L. Sessler, B. Z. Tang, H. Tian and J. Yoon, Excited-state intramolecular proton-transfer (ESIPT) based fluorescence sensors and imaging agents, Chem. Soc. Rev., 2018, 47(23), 8842–8880 RSC
.
- Y. Tang, D. Lee, J. Wang, G. Li, J. Yu, W. Lin and J. Yoon, Development of fluorescent probes based on protection–deprotection of the key functional groups for biological imaging, Chem. Soc. Rev., 2015, 44(15), 5003–5015 RSC
.
- P. Gao, W. Pan, N. Li and B. Tang, Fluorescent probes for organelle-targeted bioactive species imaging, Chem. Sci., 2019, 10(24), 6035–6071 RSC
.
- T. Kowada, H. Maeda and K. Kikuchi, BODIPY-based probes for the fluorescence imaging of biomolecules in living cells, Chem. Soc. Rev., 2015, 44(14), 4953–4972 RSC
.
- D. S. Liu, L. G. Nivon, F. Richter, P. J. Goldman, T. J. Deerinck, J. Z. Yao, D. Richardson, W. S. Phipps, A. Z. Ye, M. H. Ellisman, C. L. Drennan, D. Baker and A. Y. Ting, Computational design of a red fluorophore ligase for site-specific protein labeling in living cells, Proc. Natl. Acad. Sci. U. S. A., 2014, 111(43), E4551–E4559 CrossRef CAS
.
- Y. Huang, F. Yu, J. Wang and L. Chen, Near-infrared fluorescence probe for in situ detection of superoxide anion and hydrogen polysulfides in mitochondrial oxidative stress, Anal. Chem., 2016, 88(7), 4122–4129 CrossRef CAS
.
- G. Niu, P. Zhang, W. Liu, M. Wang, H. Zhang, J. Wu, L. Zhang and P. Wang, Near-infrared probe based on rhodamine derivative for highly sensitive and selective lysosomal pH tracking, Anal. Chem., 2017, 89(3), 1922–1929 CrossRef CAS
.
- L. Yuan, W. Lin and H. Chen, Analogs of Changsha near-infrared dyes with large Stokes Shifts for bioimaging, Biomaterials, 2013, 34(37), 9566–9571 CrossRef CAS
.
- Z. Guo, S. Park, J. Yoon and I. Shin, Recent progress in the development of near-infrared fluorescent probes for bioimaging applications, Chem. Soc. Rev., 2014, 43(1), 16–29 RSC
.
- B. Kaur, N. Kaur and S. Kumar, Colorimetric metal ion sensors – A comprehensive review of the years 2011–2016, Coord. Chem. Rev., 2018, 358, 13–69 CrossRef CAS
.
- D. Maity and T. Govindaraju, Highly selective colorimetric chemosensor for Co2+, Inorg. Chem., 2011, 50(22), 11282–11284 CrossRef CAS
.
- R. M. Duke, E. B. Veale, F. M. Pfeffer, P. E. Kruger and T. Gunnlaugsson, Colorimetric and fluorescent anion sensors: an overview of recent developments in the use of 1,8-naphthalimide-based chemosensors, Chem. Soc. Rev., 2010, 39(10), 3936–3953 RSC
.
- H. G. Im, H. Y. Kim, M. G. Choi and S. K. Chang, Reaction-based dual signaling of fluoride ions by resorufin sulfonates, Org. Biomol. Chem., 2013, 11(18), 2966–2971 RSC
.
- Y. Hitomi, T. Takeyasu and M. Kodera, Iron complex-based fluorescent probes for intracellular hydrogen peroxide detection, Chem. Commun., 2013, 49(85), 9929–9931 RSC
.
- C. Bueno, M. Villegas, S. Bertolotti, C. Previtali, M. G. Neumann and A. V. Encinas, The excited-state interaction of resazurin and resorufin with aminesin aqueous solutions. Photophysics and photochemical reaction, Photochem. Photobiol., 2002, 76(4), 385–390 CrossRef CAS
.
- T. Wehner, J. Heck, C. Feldmann and K. Müller-Buschbaum, Reactivity of ZrO(MFP) and ZrO(RP) nanoparticles with LnCl3 for solvatochromic luminescence modification and pH-dependent optical sensing, Chem. – Eur. J., 2019, 25(72), 16630–16638 CrossRef CAS
.
- D. J. Simpson, C. J. Unkefer, T. W. Whaley and B. L. Marrone, A mechanism-based fluorogenic probe for the cytochrome P-450 cholesterol side chain cleavage enzyme, J. Org. Chem., 1991, 56(18), 5391–5396 CrossRef CAS
.
- Z. Li, X. Li, X. Gao, Y. Zhang, W. Shi and H. Ma, Nitroreductase detection and hypoxic tumor cell imaging by a designed sensitive and selective fluorescent probe, 7-[(5-nitrofuran-2-yl)methoxy]-3H-phenoxazin-3-one, Anal. Chem., 2013, 85(8), 3926–3932 CrossRef CAS
.
- Y. Shiraishi, K. Yamamoto, S. Sumiya and T. Hirai, Selective fluorometric detection of aromatic thiols by a chemosensor containing two electrophilic sites with different local softness, Chem. Commun., 2013, 49(99), 11680–11682 RSC
.
- J. W. Yoon, S. Kim, Y. Yoon and M. H. Lee, A resorufin-based fluorescent turn-on probe responsive to nitroreductase activity and its application to bacterial detection, Dyes Pigm., 2019, 171, 107779 CrossRef CAS
.
- Y. Guo, X. Yang, L. Hakuna, A. Barve, J. O. Escobedo, M. Lowry and R. M. Strongin, A fast response highly selective probe for the detection of glutathione in human blood plasma, Sensors, 2012, 12(5), 5940–5950 CrossRef CAS
.
- R. Takagi, A. Takeda, D. Takahashi and K. Toshima, Target-selective fluorescence imaging and photocytotoxicity against H2O2 high-expressing cancer cells using a photoactivatable theranostic agent, Chem. – Asian J., 2017, 12(20), 2656–2659 CrossRef CAS
.
- Y. Qian, J. Lin, L. Han, L. Lin and H. Zhu, A resorufin-based colorimetric and fluorescent probe for live-cell monitoring of hydrazine, Biosens. Bioelectron., 2014, 58, 282–286 CrossRef CAS
.
- Y. Hitomi, T. Takeyasu, T. Funabiki and M. Kodera, Detection of enzymatically generated hydrogen peroxide by metal-based fluorescent probe, Anal. Chem., 2011, 83(24), 9213–9216 CrossRef CAS
.
- P. Lefrancois, V. S. Vajrala, I. B. Arredondo, B. Goudeau, T. Doneux, L. Bouffier and S. Arbault, Direct oxidative pathway from amplex red to resorufin revealed by in situ confocal imaging, Phys. Chem. Chem. Phys., 2016, 18(37), 25817–25822 RSC
.
- G. V. Porcal, M. S. Altamirano, C. A. Glusko, S. G. Bertolotti and C. M. Previtali, Photophysical properties of the phenoxazin dyes resazurin and resorufin in soybean lecithin microemulsions, Dyes Pigm., 2011, 88(3), 240–246 CrossRef CAS
.
- M. Zhou, Z. Diwu, N. Panchuk-Voloshina and R. P. Haugland, A stable nonfluorescent derivative of resorufin for the fluorometric determination of trace hydrogen peroxide: applications in detecting the activity of phagocyte NADPH oxidase and other oxidases, Anal. Biochem., 1997, 253(2), 162–168 CrossRef CAS
.
- C. L. Hsu, C. W. Lien, C. W. Wang, S. G. Harroun, C. C. Huang and H. T. Chang, Immobilization of aptamer-modified gold nanoparticles on BiOCl nanosheets: tunable peroxidase-like activity by protein recognition, Biosens. Bioelectron., 2016, 75, 181–187 CrossRef CAS
.
- C.-W. Wu, S. G. Harroun, C.-W. Lien, H.-T. Chang, B. Unnikrishnan, I. P.-J. Lai, J.-Y. Chang and C.-C. Huang, Self-templated formation of aptamer-functionalized copper oxide nanorods with intrinsic peroxidase catalytic activity for protein and tumor cell detection, Sens. Actuators, B, 2016, 227, 100–107 CrossRef CAS
.
- Z. Han, X. Liang, X. Ren, L. Shang and Z. Yin, A 3,7-dihydroxyphenoxazine-based fluorescent probe for selective detection of intracellular hydrogen peroxide, Chem. – Asian J., 2016, 11(6), 818–822 CrossRef CAS
.
- F. Yan, K. Fan, Z. Bai, R. Zhang, F. Zu, J. Xu and X. Li, Fluorescein applications as fluorescent probes for the detection of analytes, Trends Anal. Chem., 2017, 97, 15–35 CrossRef CAS
.
- L. Yuan, W. Lin, K. Zheng, L. He and W. Huang, Far-red to near infrared analyte-responsive fluorescent probes based on organic fluorophore platforms for fluorescence imaging, Chem. Soc. Rev., 2013, 42(2), 622–661 RSC
.
- X. Chen, T. Pradhan, F. Wang, J. S. Kim and J. Yoon, Fluorescent chemosensors based on spiroring-opening of xanthenes and related derivatives, Chem. Rev., 2012, 112(3), 1910–1956 CrossRef CAS
.
- M. Beija, C. A. Afonso and J. M. Martinho, Synthesis and applications of Rhodamine derivatives as fluorescent probes, Chem. Soc. Rev., 2009, 38(8), 2410–2433 RSC
.
- H. N. Kim, W. X. Ren, J. S. Kim and J. Yoon, Fluorescent and colorimetric sensors for detection of lead, cadmium, and mercury ions, Chem. Soc. Rev., 2012, 41(8), 3210–3244 RSC
.
- Q. He, E. W. Miller, A. P. Wong and C. J. Chang, A selective fluorescent sensor for detecting lead in living cells, J.
Am. Chem. Soc., 2006, 128(29), 9316–9317 CrossRef CAS
.
- K. Sudhakar Gurpur, R. Verma and K. Vijaya Bhasker, Kumar Lalit. Heavy metals in environment, living systems and herbal preparations-An Overview, Int. Res. J. Pharm., 2012, 3(7), 128–130 Search PubMed
.
- M. Sun, D. Shangguan, H. Ma, L. Nie, X. Li, S. Xiong, G. Liu and W. Thiemann, Simple PbII fluorescent probe based on PbII-catalyzed hydrolysis of phosphodiester, Biopolymers, 2003, 72(6), 413–420 CrossRef CAS
.
- M. G. Choi, S. Y. Park, K. Y. Park and S. K. Chang, Novel Hg2+-selective signaling probe based on resorufin thionocarbonate and its μPAD application, Sci. Rep., 2019, 9(1), 3348 CrossRef
.
- C. W. Lien, Y. T. Tseng, C. C. Huang and H. T. Chang, Logic control of enzyme-like gold nanoparticles for selective detection of lead and mercury ions, Anal. Chem., 2014, 86(4), 2065–2072 CrossRef CAS
.
- W. Luo and W. Liu, A water-soluble colorimetric two-photon probe for discrimination of different palladium species and its application in bioimaging, Dalton Trans., 2016, 45(29), 11682–11687 RSC
.
- J. Jiang, H. Jiang, W. Liu, X. Tang, X. Zhou, W. Liu and R. Liu, A colorimetric and ratiometric fluorescent probe for palladium, Org. Lett., 2011, 13(18), 4922–4925 CrossRef CAS
.
- W. X. Ren, T. Pradhan, Z. Yang, Q.-Y. Cao and J. S. Kim, Rapid responsive palladium sensor under mild condition, Sens. Actuators, B, 2012, 171, 1277–1282 CrossRef
.
- J. W. Yan, X. L. Wang, Q. F. Tan, P. F. Yao, J. H. Tan and L. Zhang, A colorimetric and fluorescent dual probe for palladium in aqueous medium and live cell imaging, Analyst, 2016, 141(8), 2376–2379 RSC
.
- M. Nieberding, M. P. Tracey and K. Koide, Noneffervescent method for catalysis-based palladium detection with color or fluorescence, ACS Sens., 2017, 2(11), 1737–1743 CrossRef CAS
.
- S. Zhang, R. Adhikari, M. Fang, N. Dorh, C. Li, M. Jaishi, J. Zhang, A. Tiwari, R. Pati, F.-T. Luo and H. Liu, Near-infrared fluorescent probes with large Stokes shifts for sensing Zn(II) ions in living cells, ACS Sens., 2016, 1(12), 1408–1415 CrossRef CAS
.
- E. M. Nolan and S. J. Lippard, Small-molecule fluorescent sensors for investigating zinc metalloneurochemistry, Acc. Chem. Res., 2009, 42(1), 193–203 CrossRef CAS
.
- C. J. Frederickson, J.-Y. Koh and A. I. Bush, The neurobiology of zinc in health and disease, Nat. Rev. Neurosci., 2005, 6(6), 449–462 CrossRef CAS
.
- A. Loas, R. J. Radford and S. J. Lippard, Addition of a second binding site increases the dynamic range but alters the cellular localization of a red fluorescent probe for mobile zinc, Inorg. Chem., 2014, 53(13), 6491–6493 CrossRef CAS
.
- S. Okamoto and L. D. Eltis, The biological occurrence and trafficking of cobalt, Metallomics, 2011, 3(10), 963–970 RSC
.
- A. T. Aron, K. M. Ramos-Torres, J. A. Cotruvo and C. J. Chang, Recognition-and reactivity-based fluorescent probes for studying transition metal signaling in living systems, Acc. Chem. Res., 2015, 48(8), 2434–2442 CrossRef CAS
.
- D. G. Barceloux and D. Barceloux, Cobalt, Clin. Toxicol., 1999, 37(2), 201–216 CAS
.
- L. Zeng, E. W. Miller, A. Pralle, E. Y. Isacoff and C. J. Chang, A selective turn-on fluorescent sensor for imaging copper in living cells, J. Am. Chem. Soc., 2006, 128(1), 10–11 CrossRef CAS
.
- T. Hirayama, G. C. Van de Bittner, L. W. Gray, S. Lutsenko and C. J. Chang, Near-infrared fluorescent sensor for in vivo copper imaging in a murine Wilson disease model, Proc. Natl. Acad. Sci. U. S. A., 2012, 109(7), 2228–2233 CrossRef CAS
.
- B. E. Kim, T. Nevitt and D. J. Thiele, Mechanisms for copper acquisition, distribution regulation, Nat. Chem. Biol., 2008, 4, 176–185 CrossRef CAS
.
- D. Maity, A. Raj, D. Karthigeyan, T. K. Kundu and T. Govindaraju, Reaction-based probes for Co(II) and Cu(I) with dual output modes: fluorescence live cell imaging, RSC Adv., 2013, 3(37), 16788–16794 RSC
.
- B. Ke, W. Chen, N. Ni, Y. Cheng, C. Dai, H. Dinh and B. Wang, A fluorescent probe for rapid aqueous fluoride detection and cell imaging, Chem. Commun., 2013, 49(25), 2494–2496 RSC
.
- M. Kleerekoper, The role of fluoride in the prevention of osteoporosis, Endocrinol. Metab. Clin. North Am., 1998, 27(2), 441–452 CrossRef CAS
.
- Y. Wang, R. Zhang, R. Song, K. Guo, Q. Meng, H. Feng, C. Duan and Z. Zhang, Fluoride-specific fluorescence/MRI bimodal probe based on a gadolinium(III)–flavone complex: synthesis, mechanism and bioimaging application in vivo, J. Mater. Chem. B, 2016, 4(46), 7379–7386 RSC
.
- Y. Wang, R. Song, K. Guo, Q. Meng, R. Zhang, X. Kong and Z. Zhang, A gadolinium(III) complex based dual-modal probe for MRI and fluorescence sensing of fluoride ions in aqueous medium and in vivo, Dalton Trans., 2016, 45(44), 17616–17623 RSC
.
- S. Y. Kim and J.-I. Hong, Chromogenic and fluorescent chemodosimeter for detection of fluoride in aqueous solution, Org. Lett., 2007, 9(16), 3109–3112 CrossRef CAS
.
- A. Roy, D. Kand, T. Saha and P. Talukdar, Pink fluorescence emitting fluoride ion sensor: investigation of the cascade sensing mechanism and bioimaging applications, RSC Adv., 2014, 4(64), 33890–33896 RSC
.
- H. Agarwalla, M. Gangopadhyay, D. K. Sharma, S. K. Basu, S. Jadhav, A. Chowdhury and A. Das, Fluorescent probes for the detection of cyanide ions in aqueous medium: cellular uptake and assay for β-glucosidase and hydroxynitrile lyase, J. Mater. Chem. B, 2015, 3(47), 9148–9156 RSC
.
- J. Schubert and W. A. Brill, Antagonism of experimental cyanide toxicity in relation to the in vivo activity of cytochrome oxidase, J. Pharmacol. Exp. Ther., 1968, 162(2), 352–359 CAS
.
- J. H. Lee, J. H. Jang, N. Velusamy, H. S. Jung, S. Bhuniya and J. S. Kim, An intramolecular crossed-benzoin reaction based KCN fluorescent probe in aqueous and biological environments, Chem. Commun., 2015, 51(36), 7709–7712 RSC
.
- C. W. Lien, B. Unnikrishnan, S. G. Harroun, C. M. Wang, J. Y. Chang, H. T. Chang and C. C. Huang, Visual detection of cyanide ions by membrane-based nanozyme assay, Biosens. Bioelectron., 2018, 102, 510–517 CrossRef CAS
.
- Y. Chang, M. Liu and J. Liu, Highly selective fluorescent sensing of phosphite through recovery of poisoned nickel oxide nanozyme, Anal. Chem., 2020, 92(4), 3118–3124 CrossRef CAS
.
- J. Seiler, The mutagenic activity of sodium perborate, Mutat. Res., 1989, 224(2), 219–227 CAS
.
- M. G. Choi, S. Cha, J. E. Park, H. Lee, H. L. Jeon and S.-K. Chang, Selective perborate signaling by deprotection of fluorescein and resorufin acetates, Org. Lett., 2010, 12(7), 1468–1471 CrossRef CAS
.
- Z. Du, R. Zhang, B. Song, W. Zhang, Y.-L. Wang, J. Liu, C. Liu, Z. P. Xu and J. Yuan, Iridium(III) complex-based activatable probe for phosphorescent/time-gated luminescent sensing and imaging of cysteine in mitochondria of live cells and animals, Chem. – Eur. J., 2019, 25(6), 1498–1506 CrossRef CAS
.
- W. Zhang, R. Zhang, J. Zhang, Z. Ye, D. Jin and J. Yuan, Photoluminescent and electrochemiluminescent dual-signaling probe for bio-thiols based on a ruthenium(II) complex, Anal. Chim. Acta, 2012, 740, 80–87 CrossRef CAS
.
- R. Zhang, X. Yu, Z. Ye, G. Wang, W. Zhang and J. Yuan, Turn-on luminescent probe for cysteine/homocysteine based on a ruthenium(II) complex, Inorg. Chem., 2010, 49(17), 7898–7903 CrossRef CAS
.
- L. A. Herzenberg, S. C. De Rosa, J. G. Dubs, M. Roederer, M. T. Anderson, S. W. Ela, S. C. Deresinski and L. A. Herzenberg, Glutathione deficiency is associated with impaired survival in HIV disease, Proc. Natl. Acad. Sci. U. S. A., 1997, 94(5), 1967–1972 CrossRef CAS
.
- R. Franco, O. Schoneveld, A. Pappa and M. Panayiotidis, The central role of glutathione in the pathophysiology of human diseases, Arch. Physiol. Biochem., 2007, 113(4–5), 234–258 CrossRef CAS
.
- L. Zhao, Z. Li, L. Zhao and C. Zhang,
In vivo determination of reduced thiols in rat cerebellum paraflocculus following salicylate-induced tinnitus by fluorescence, Anal. Lett., 2017, 50(3), 530–543 CrossRef CAS
.
- H. Zhou, J. Tang, L. Lv, N. Sun, J. Zhang, B. Chen, J. Mao, W. Zhang, J. Zhang and J. Zhou, Intracellular endogenous glutathione detection and imaging by a simple and sensitive spectroscopic off-on probe, Analyst, 2018, 143(10), 2390–2396 RSC
.
- L. Hakuna, B. Doughan, J. O. Escobedo and R. M. Strongin, A simple assay for glutathione in whole blood, Analyst, 2015, 140(10), 3339–3342 RSC
.
- T. Ma, H. Ding, H. Xu, Y. Lv, H. Liu, H. Wang and Z. Tian, Dual-functional probes for sequential thiol and redox homeostasis sensing in live cells, Analyst, 2015, 140(1), 322–329 RSC
.
- X. Gao, X. Li, L. Li, J. Zhou and H. Ma, A simple fluorescent off-on probe for the discrimination of cysteine from glutathione, Chem. Commun., 2015, 51(45), 9388–9390 RSC
.
- C. Liu, J. Liu, W. Zhang, Y.-L. Wang, Q. Liu, B. Song, J. Yuan and R. Zhang, “Two birds with one stone” ruthenium(II) complex probe for biothiols discrimination and detection in vitro and in vivo, Adv. Sci., 2020, 2000458 CrossRef CAS
.
- H. Zhang, L. Xu, W. Chen, J. Huang, C. Huang, J. Sheng and X. Song, Simultaneous discrimination of cysteine, homocysteine, glutathione, and H2S in living cells through a multisignal combination strategy, Anal. Chem., 2019, 91(3), 1904–1911 CrossRef CAS
.
- R. Zhang, X. Yu, Y. Yin, Z. Ye, G. Wang and J. Yuan, Development of a heterobimetallic Ru(II)–Cu(II) complex for highly selective and sensitive luminescence sensing of sulfide anions, Anal. Chim. Acta, 2011, 691(1), 83–88 CrossRef CAS
.
- R. Kaushik and A. Ghosh, Amilan Jose, D., Recent progress in hydrogen sulphide (H2S) sensors by metal displacement approach, Coord. Chem. Rev., 2017, 347, 141–157 CrossRef CAS
.
- Q. Meng, Y. Shi, C. Wang, H. Jia, X. Gao, R. Zhang, Y. Wang and Z. Zhang, NBD-based fluorescent chemosensor for the selective quantification of copper and sulfide in an aqueous solution and living cells, Org. Biomol. Chem., 2015, 13(10), 2918–2926 RSC
.
- J. Liu, C. Duan, W. Zhang, H. T. Ta, J. Yuan, R. Zhang and Z. P. Xu, Responsive nanosensor for ratiometric luminescence detection of hydrogen sulfide in inflammatory cancer cells, Anal. Chim. Acta, 2020, 1103, 156–163 CrossRef CAS
.
- T. Saha, D. Kand and P. Talukdar, Performance comparison of two cascade reaction models in fluorescence off–on detection of hydrogen sulfide, RSC Adv., 2015, 5(2), 1438–1446 RSC
.
- K. I. Hsu, C. W. Lien, C. H. Lin, H. T. Chang and C. C. Huang, Immobilization of iron hydroxide/oxide on reduced graphene oxide: peroxidase-like activity and selective detection of sulfide ions, RSC Adv., 2014, 4(71), 37705–37713 RSC
.
- F. Zhou, Y. Sultanbawa, H. Feng, Y. L. Wang, Q. Meng, Y. Wang, Z. Zhang and R. Zhang, A new red-emitting fluorescence probe for rapid and effective visualization of bisulfite in food samples and live animals, J. Agric. Food Chem., 2019, 67(15), 4375–4383 CrossRef CAS
.
- W. Zhang, X. Xi, Y. L. Wang, Z. Du, C. Liu, J. Liu, B. Song, J. Yuan and R. Zhang, Responsive ruthenium complex probe for phosphorescence and time-gated luminescence detection of bisulfite, Dalton Trans., 2020, 49(17), 5531–5538 RSC
.
- Y. Wang, Q. Meng, R. Zhang, H. Jia, X. Zhang and Z. Zhang, A ratiometric fluorescence probe for imaging sulfur dioxide derivatives in the mitochondria of living cells, Org. Biomol. Chem., 2017, 15(13), 2734–2739 RSC
.
- M. G. Choi, J. Hwang, S. Eor and S. K. Chang, Chromogenic and fluorogenic signaling of sulfite by selective deprotection of resorufin levulinate, Org. Lett., 2010, 12(24), 5624–5627 CrossRef CAS
.
- N. Gupta, S. I. Reja, V. Bhalla and M. Kumar, Fluorescent probes for hydrogen polysulfides (H2Sn, n > 1): from design rationale to applications, Org. Biomol. Chem., 2017, 15(32), 6692–6701 RSC
.
- K. B. Li, F. Z. Chen, S. Zhang, W. Shi, D. M. Han, C. Cai and C. X. Chen, A nile red-based near-infrared fluorescent probe for endogenous hydrogen polysulfides in living cells, Anal. Methods, 2017, 9(45), 6443–6447 RSC
.
- C. Wang, J. Xu, Q. Ma, Y. Bai, M. Tian, J. Sun and Z. Zhang, A highly selective fluorescent probe for hydrogen polysulfides in living cells based on a naphthalene derivative, Spectrochim. Acta, Part A, 2020, 227, 117579 CrossRef CAS
.
- H. Kimura, Signaling molecules: hydrogen sulfide and polysulfide, Antioxid. Redox Signaling, 2015, 22(5), 362–376 CrossRef CAS
.
- J. Liu and Z. Yin, A resorufin-based fluorescent probe for imaging polysulfides in living cells, Analyst, 2019, 144(10), 3221–3225 RSC
.
- X. Liu, F. Qi, Y. Su, W. Chen, L. Yang and X. Song, A red emitting fluorescent probe for instantaneous sensing of thiophenol in both aqueous medium and living cells with a large Stokes shift, J. Mater. Chem. C, 2016, 4(19), 4320–4326 RSC
.
- R. Zhang, Z. Ye, Y. Yin, G. Wang, D. Jin, J. Yuan and J. A. Piper, Developing red-emissive ruthenium(II) complex-based luminescent probes for cellular imaging, Bioconjugate Chem., 2012, 23(4), 725–733 CrossRef CAS
.
- D. Kand, P. K. Mishra, T. Saha, M. Lahiri and P. Talukdar, BODIPY based colorimetric fluorescent probe for selective thiophenol detection: theoretical and experimental studies, Analyst, 2012, 137(17), 3921–3924 RSC
.
- Y. Chen, X. Shi, Z. Lu, X. Wang and Z. Wang, A fluorescent probe for hydrogen peroxide in vivo based on the modulation of intramolecular charge transfer, Anal. Chem., 2017, 89(10), 5278–5284 CrossRef CAS
.
- L. Wu, A. C. Sedgwick, X. Sun, S. D. Bull, X.-P. He and T. D. James, Reaction-based fluorescent probes for the detection and imaging of reactive oxygen, nitrogen, and sulfur species, Acc. Chem. Res., 2019, 52(9), 2582–2597 CrossRef CAS
.
- K. Żamojć, M. Zdrowowicz, D. Jacewicz, D. Wyrzykowski and L. Chmurzyński, Fluorescent probes used for detection of hydrogen peroxide under biological conditions, Crit. Rev. Anal. Chem., 2016, 46(3), 171–200 CrossRef
.
- M. Abo, Y. Urano, K. Hanaoka, T. Terai, T. Komatsu and T. Nagano, Development of a highly sensitive fluorescence probe for hydrogen peroxide, J. Am. Chem. Soc., 2011, 133(27), 10629–10637 CrossRef CAS
.
- D. J. Zheng, Y. S. Yang and H. L. Zhu, Recent progress in the development of small-molecule fluorescent probes for the detection of hydrogen peroxide, Trends Anal. Chem., 2019, 118, 625–651 CrossRef CAS
.
- E. W. Miller, A. E. Albers, A. Pralle, E. Y. Isacoff and C. J. Chang, Boronate-based fluorescent probes for imaging cellular hydrogen peroxide, J. Am. Chem. Soc., 2005, 127(47), 16652–16659 CrossRef CAS
.
- M. L. Odyniec, A. C. Sedgwick, A. H. Swan, M. Weber, T. M. S. Tang, J. E. Gardiner, M. Zhang, Y. B. Jiang, G. Kociok-Kohn, R. B. P. Elmes, S. D. Bull, X. P. He and T. D. James, ‘AND’-based fluorescence scaffold for the detection of ROS/RNS and a second analyte, Chem. Commun., 2018, 54(61), 8466–8469 RSC
.
- D. Debski, R. Smulik, J. Zielonka, B. Michalowski, M. Jakubowska, K. Debowska, J. Adamus, A. Marcinek, B. Kalyanaraman and A. Sikora, Mechanism of oxidative conversion of Amplex(R) Red to resorufin: Pulse radiolysis and enzymatic studies, Free Radical Biol. Med., 2016, 95, 323–332 CrossRef CAS
.
- W. Chen, J. Chen, Y. B. Feng, L. Hong, Q. Y. Chen, L. F. Wu, X. H. Lin and X. H. Xia, Peroxidase-like activity of water-soluble cupric oxide nanoparticles and its analytical application for detection of hydrogen peroxide and glucose, Analyst, 2012, 137(7), 1706–1712 RSC
.
- D. Wu, L. Chen, Q. Xu, X. Chen and J. Yoon, Design principles, sensing mechanisms, and applications of highly specific fluorescent probes for HOCl/OCl−, Acc. Chem. Res., 2019, 52(8), 2158–2168 CrossRef CAS
.
- J. Wang, Y. Men, L. Niu, Y. Luo, J. Zhang, W. Zhao and J. Wang, A reaction-based fluorescent probe for imaging of native hypochlorous acid, Chem. – Asian J., 2019, 14(21), 3893–3897 CrossRef CAS
.
- M. G. Choi, Y. J. Lee, K. M. Lee, K. Y. Park, T. J. Park and S. K. Chang, A simple hypochlorous acid signaling probe based on resorufin carbonodithioate and its biological application, Analyst, 2019, 144(24), 7263–7269 RSC
.
- D.-Y. Zhou, Y. Li, W.-L. Jiang, Y. Tian, J. Fei and C.-Y. Li, A ratiometric fluorescent probe for peroxynitrite prepared by de novo synthesis and its application in assessing the mitochondrial oxidative stress status in cells and in vivo, Chem. Commun., 2018, 54(82), 11590–11593 RSC
.
- S. Wang, L. Chen, P. Jangili, A. Sharma, W. Li, J.-T. Hou, C. Qin, J. Yoon and J. S. Kim, Design and applications of fluorescent detectors for peroxynitrite, Coord. Chem. Rev., 2018, 374, 36–54 CrossRef CAS
.
- Z. N. Sun, H. L. Wang, F. Q. Liu, Y. Chen, P. K. H. Tam and D. Yang, BODIPY-based fluorescent probe for peroxynitrite detection and imaging in living cells, Org. Lett., 2009, 11(9), 1887–1890 CrossRef CAS
.
- W. Zhang, Y. Liu, Q. Gao, C. Liu, B. Song, R. Zhang and J. Yuan, A ruthenium(II) complex–cyanine energy transfer scaffold based luminescence probe for ratiometric detection and imaging of mitochondrial peroxynitrite, Chem. Commun., 2018, 54(97), 13698–13701 RSC
.
- G. L. Squadrito and W. A. Pryor, Oxidative chemistry of nitric oxide: the roles of superoxide, peroxynitrite, and carbon dioxide, Free Radical Biol. Med., 1998, 25(4-5), 392–403 CrossRef CAS
.
- Q. Zhang, Z. Zhu, Y. Zheng, J. Cheng, N. Zhang, Y. T. Long, J. Zheng, X. Qian and Y. Yang, A three-channel fluorescent probe that distinguishes peroxynitrite from hypochlorite, J. Am. Chem. Soc., 2012, 134(45), 18479–18482 CrossRef CAS
.
- A. Sikora, J. Zielonka, M. Lopez, J. Joseph and B. Kalyanaraman, Direct oxidation of boronates by peroxynitrite: mechanism and implications in fluorescence imaging of peroxynitrite, Free Radical Biol. Med., 2009, 47(10), 1401–1407 CrossRef CAS
.
- C. Hu, W. Sun, J. Cao, P. Gao, J. Wang, J. Fan, F. Song, S. Sun and X. Peng, A ratiometric near-infrared fluorescent probe for hydrazine and its in vivo applications, Org. Lett., 2013, 15(15), 4022–4025 CrossRef CAS
.
- J. Zhang, L. Ning, J. Liu, J. Wang, B. Yu, X. Liu, X. Yao, Z. Zhang and H. Zhang, Naked-eye and near-infrared fluorescence probe for hydrazine and its applications in in vitro and in vivo bioimaging, Anal. Chem., 2015, 87(17), 9101–9107 CrossRef CAS
.
- R. Zhang, C. J. Zhang, Z. Song, J. Liang, R. T. K. Kwok, B. Z. Tang and B. Liu, AIEgens for real-time naked-eye sensing of hydrazine in solution and on a paper substrate: structure-dependent signal output and selectivity, J. Mater. Chem. C, 2016, 4(14), 2834–2842 RSC
.
- H. Xu, Z. Huang, Y. Li, B. Gu, Z. Zhou, R. Xie, X. Pang, H. Li and Y. Zhang, A highly sensitive naked-eye fluorescent probe for trace hydrazine based on ‘C-CN’ bond cleavage, Analyst, 2018, 143(18), 4354–4358 RSC
.
- T. Tang, Y. Q. Chen, B. S. Fu, Z. Y. He, H. Xiao, F. Wu, J. Q. Wang, S. R. Wang and X. Zhou, A novel resorufin based fluorescent “turn-on” probe for the selective detection of hydrazine and application in living cells, Chin. Chem. Lett., 2016, 27(4), 540–544 CrossRef CAS
.
- M. G. Choi, J. O. Moon, J. Bae, J. W. Lee and S. K. Chang, Dual signaling of hydrazine by selective deprotection of dichlorofluorescein and resorufin acetates, Org. Biomol. Chem., 2013, 11(18), 2961–2965 RSC
.
- K. L. Reddy, M. Venkateswarulu, K. R. Shankar, S. Ghosh and V. Krishnan, Upconversion luminescent material-based inorganic-organic hybrid sensing system for the selective detection of hydrazine in environmental samples, ChemistrySelect, 2018, 3(6), 1793–1800 CrossRef CAS
.
- R. Zhang, L. Liang, Q. Meng, J. Zhao, H. T. Ta, L. Li, Z. Zhang, Y. Sultanbawa and Z. P. Xu, Responsive upconversion nanoprobe for background-free hypochlorous acid detection and bioimaging, Small, 2019, 15(2), 1803712 Search PubMed
.
- R. He, J. Lu and J. Miao, Formaldehyde stress, Sci. China: Life Sci., 2010, 53(12), 1399–1404 CrossRef CAS
.
- J. Ohata, K. J. Bruemmer and C. J. Chang, Activity-based sensing methods for monitoring the reactive carbon species carbon monoxide and formaldehyde in living systems, Acc. Chem. Res., 2019, 52(10), 2841–2848 CrossRef CAS
.
- X. Xie, F. Tang, X. Shangguan, S. Che, J. Niu, Y. Xiao, X. Wang and B. Tang, Two-photon imaging of formaldehyde in live cells and animals utilizing a lysosome-targetable and acidic pH-activatable fluorescent probe, Chem. Commun., 2017, 53(48), 6520–6523 RSC
.
- K. J. Bruemmer, R. R. Walvoord, T. F. Brewer, G. Burgos-Barragan, N. Wit, L. B. Pontel, K. J. Patel and C. J. Chang, Development of a general aza-cope reaction trigger applied to fluorescence imaging of formaldehyde in living cells, J. Am. Chem. Soc., 2017, 139(15), 5338–5350 CrossRef CAS
.
- N. W. Pino, J. Davis, Z. Yu and J. Chan, NitroxylFluor: a thiol-based fluorescent probe for live-cell imaging of nitroxyl, J. Am. Chem. Soc., 2017, 139(51), 18476–18479 CrossRef CAS
.
- N. Paolocci, W. F. Saavedra, K. M. Miranda, C. Martignani, T. Isoda, J. M. Hare, M. G. Espey, J. M. Fukuto, M. Feelisch and D. A. Wink, Nitroxyl anion exerts redox-sensitive positive cardiac inotropy in vivo by calcitonin gene-related peptide signaling, Proc. Natl. Acad. Sci. U. S. A., 2001, 98(18), 10463–10468 CrossRef CAS
.
- R. Smulik-Izydorczyk, K. Dębowska, J. Pięta, R. Michalski, A. Marcinek and A. Sikora, Fluorescent probes for the detection of nitroxyl (HNO), Free Radical Biol. Med., 2018, 128, 69–83 CrossRef CAS
.
- K. Sunwoo, K. N. Bobba, J.-Y. Lim, T. Park, A. Podder, J. S. Heo, S. G. Lee, S. Bhuniya and J. S. Kim, A bioorthogonal ‘turn-on’ fluorescent probe for tracking mitochondrial nitroxyl formation, Chem. Commun., 2017, 53(10), 1723–1726 RSC
.
- K. N. Bobba, Y. Zhou, L. E. Guo, T. N. Zang, J. F. Zhang and S. Bhuniya, Resorufin based fluorescence ‘turn-on’chemodosimeter probe for nitroxyl (HNO), RSC Adv., 2015, 5(103), 84543–84546 RSC
.
- K. Xu, S. Sun, J. Li, L. Li, M. Qiang and B. Tang, A near-infrared fluorescent probe for monitoring ozone and imaging in living cells, Chem. Commun., 2012, 48(5), 684–686 RSC
.
- A. L. Garner, C. M. St Croix, B. R. Pitt, G. D. Leikauf, S. Ando and K. Koide, Specific fluorogenic probes for ozone in biological and atmospheric samples, Nat. Chem., 2009, 1(4), 316–321 CrossRef CAS
.
- T. Salthammer, Formaldehyde in the ambient atmosphere: from an indoor pollutant to an outdoor pollutant?, Angew. Chem., Int. Ed., 2013, 52(12), 3320–3327 CrossRef CAS
.
- Y. Zhang, W. Shi, X. Li and H. Ma, Sensitive detection of ozone by a practical resorufin-based spectroscopic probe with extremely low background signal, Sci. Rep., 2013, 3, 2830 CrossRef
.
- Q. Hu, W. Li, C. Qin, L. Zeng and J.-T. Hou, Rapid and visual detection of benzoyl peroxide in food by a colorimetric and ratiometric fluorescent probe, J. Agric. Food Chem., 2018, 66(41), 10913–10920 CrossRef CAS
.
- X. Tian, Z. Li, Y. Pang, D. Li and X. Yang, Benzoyl peroxide detection in real samples and zebrafish imaging by a designed near-infrared fluorescent probe, J. Agric. Food Chem., 2017, 65(43), 9553–9558 CrossRef CAS
.
- H. Ding, G. Yuan and L. Zhou, A new water-soluble two-photon fluorescent probe for detection of trace benzoyl peroxide in wheat flour and in living cell and tissue imaging, New J. Chem., 2019, 43(7), 3169–3173 RSC
.
- G. Bhasin, H. Kauser and M. Athar, Free radical generating agents lead to the rapid progression of benign skin tumors to carcinoma in iron-overloaded mice, Arch. Toxicol., 2004, 78(3), 139–146 CrossRef CAS
.
- W. Chen, Z. Li, W. Shi and H. Ma, A new resorufin-based spectroscopic probe for simple and sensitive detection of benzoyl peroxide via deboronation, Chem. Commun., 2012, 48(22), 2809–2811 RSC
.
- X. Gao, X. Li, Q. Wan, Z. Li and H. Ma, Detection of glucose via enzyme-coupling reaction based on a DT-diaphorase fluorescence probe, Talanta, 2014, 120, 456–461 CrossRef CAS
.
- T. Chih, H.-J. Jao and C. M. Wang, Glucose sensing based on an effective conversion of O2 and H2O2 into superoxide anion radical with clay minerals, J. Electroanal. Chem., 2005, 581(2), 159–166 CrossRef CAS
.
- W. Hu, Y. Ning, L. Li, J. Kong and X. Zhang, Highly sensitive detection of sequence-specific DNA with morpholino-functionalized magnetic microspheres, Anal. Methods, 2015, 7(16), 6712–6717 RSC
.
- S. Wang, B. Fu, J. Wang, Y. Long, X. Zhang, S. Peng, P. Guo, T. Tian and X. Zhou, Novel amplex red oxidases based on noncanonical DNA structures: property studies and applications in microRNA detection, Anal. Chem., 2014, 86(6), 2925–2930 CrossRef CAS
.
- X. Wu, W. Shi, X. Li and H. Ma, Recognition moieties of small molecular fluorescent probes for bioimaging of enzymes, Acc. Chem. Res., 2019, 52(7), 1892–1904 CrossRef CAS
.
- T. Satoh and M. Hosokawa, The mammalian carboxylesterases: from molecules to functions, Annu. Rev. Pharmacol., 1998, 38(1), 257–288 CrossRef CAS
.
- J. Zhang, X. Chai, X.-P. He, H.-J. Kim, J. Yoon and H. Tian, Fluorogenic probes for disease-relevant enzymes, Chem. Soc. Rev., 2019, 48(2), 683–722 RSC
.
- H.-W. Liu, L. Chen, C. Xu, Z. Li, H. Zhang, X.-B. Zhang and W. Tan, Recent progresses in small-molecule enzymatic fluorescent probes for cancer imaging, Chem. Soc. Rev., 2018, 47(18), 7140–7180 RSC
.
- H. Singh, K. Tiwari, R. Tiwari, S. K. Pramanik and A. Das, Small molecule as fluorescent probes for monitoring intracellular enzymatic transformations, Chem. Rev., 2019, 119(22), 11718–11760 CrossRef CAS
.
- W. Hakamata, A. Machida, T. Oku and T. Nishio, Design and synthesis of an ER-specific fluorescent probe based on carboxylesterase activity with quinone methide cleavage process, Bioorg. Med. Chem. Lett., 2011, 21(11), 3206–3209 CrossRef CAS
.
- Y. Zhang, W. Chen, D. Feng, W. Shi, X. Li and H. Ma, A spectroscopic off-on probe for simple and sensitive detection of carboxylesterase activity and its application to cell imaging, Analyst, 2012, 137(3), 716–721 RSC
.
- X. Wu, X. Li, H. Li, W. Shi and H. Ma, A highly sensitive and selective fluorescence off-on probe for the detection of intracellular endogenous tyrosinase activity, Chem. Commun., 2017, 53(16), 2443–2446 RSC
.
- H. Li, W. Liu, F. Zhang, X. Zhu, L. Huang and H. Zhang, Highly selective fluorescent probe based on hydroxylation of phenylboronic acid pinacol ester for detection of tyrosinase in cells, Anal. Chem., 2018, 90(1), 855–858 CrossRef CAS
.
- S. Yang, J. Jiang, A. Zhou, Y. Zhou, W. Ye, D.-S. Cao and R. Yang, Substrate-photocaged enzymatic fluorogenic probe enabling sequential activation for light-controllable monitoring of intracellular tyrosinase activity, Anal. Chem., 2020, 92(10), 7194–7199 CrossRef CAS
.
- W. Zhao, Y. Zhao, Q. Wang, T. Liu, J. Sun and R. Zhang, Remote light-responsive nanocarriers for controlled drug delivery: Advances and perspectives, Small, 2019, 15(45), 1903060 CrossRef CAS
.
- W. Shen, J. Zhang, G. Mao, K. Jiang and Q. Zhu, A long-wavelength, fluorogenic probe for epoxide hydrolase: 7-(2-(oxiran-2-yl) ethoxy) resorufin, Biol. Pharm. Bull., 2009, 32(9), 1496–1499 CrossRef CAS
.
- E. L. Smith, C. R. Bertozzi and K. E. Beatty, An expanded set of fluorogenic sulfatase activity probes, ChemBioChem, 2014, 15(8), 1101–1105 CrossRef CAS
.
- T. Yoshiya, H. Ii, S. Tsuda, S. Kageyama, T. Yoshiki and Y. Nishiuchi, A GGCT fluorogenic probe: design, synthesis and application to cancer-related cells, Org. Biomol. Chem., 2015, 13(11), 3182–3185 RSC
.
- F. M. Ibatullin, A. Banasiak, M. J. Baumann, L. Greffe, J. Takahashi, E. J. Mellerowicz and H. Brumer, A real-time fluorogenic assay for the visualization of glycoside hydrolase activity in planta, Plant Physiol., 2009, 151(4), 1741–1750 CrossRef CAS
.
- Z. Li, X. Gao, W. Shi, X. Li and H. Ma, 7-((5-Nitrothiophen-2-yl)methoxy)-3H-phenoxazin-3-one as a spectroscopic off-on probe for highly sensitive and selective detection of nitroreductase, Chem. Commun., 2013, 49(52), 5859–5861 RSC
.
- F. Wang, Y. Li, W. Li, Q. Zhang, J. Chen, H. Zhou and C. Yu, A facile method for detection of alkaline phosphatase activity based on the turn-on fluorescence of resorufin, Anal. Methods, 2014, 6(15), 6105–6109 RSC
.
- H. Zhang, C. Xu, J. Liu, X. Li, L. Guo and X. Li, An enzyme-activatable probe with a self-immolative linker for rapid and sensitive alkaline phosphatase detection and cell imaging through a cascade reaction, Chem. Commun., 2015, 51(32), 7031–7034 RSC
.
- S. Biswas, B. S. McCullough, E. S. Ma, D. LaJoie, C. W. Russell, D. Garrett Brown, J. L. Round, K. S. Ullman, M. A. Mulvey and A. M. Barrios, Dual colorimetric and fluorogenic probes for visualizing tyrosine phosphatase activity, Chem. Commun., 2017, 53(14), 2233–2236 RSC
.
- D. Kramer and G. Guilbault, Resorulin acetate as a substrate for determination of hydrolytic enzymes at low enzyme and substrate concentrations, Anal. Chem., 1964, 36(8), 1662–1663 CrossRef CAS
.
- L. D. Lavis, T. Y. Chao and R. T. Raines, Synthesis and utility of fluorogenic acetoxymethyl ethers, Chem. Sci., 2011, 2(3), 521–530 RSC
.
- A. K. Yadav, C. J. Reinhardt, A. S. Arango, H. C. Huff, L. Dong, M. G. Malkowski, A. Das, E. Tajkhorshid and J. Chan, An activity-based sensing approach for the detection of cyclooxygenase-2 in live cells, Angew. Chem., Int. Ed., 2020, 59(8), 3307–3314 CrossRef CAS
.
- K. Cui, Z. Chen, Z. Wang, G. Zhang and D. Zhang, A naked-eye visible and fluorescence “turn-on” probe for acetyl-cholinesterase assay and thiols as well as imaging of living cells, Analyst, 2011, 136(1), 191–195 RSC
.
- C. C. S. Gonçalves, B. Z. da Costa, M. L. S. O. Lima, G. F. Fiorito, A. L. T. G. Ruiz, S. B. P. de Oliveira, G. O. Barbosa, H. F. de Carvalho and A. J. Marsaioli, Enzymatic profiling in prostate and breast cancer cells: phosphate hydrolysis and alcohol oxidation, Tetrahedron, 2016, 72(46), 7235–7240 CrossRef
.
- A. Matagne, J. Lamotte-Brasseur and J.-M. Frère, Catalytic properties of class A β-lactamases: efficiency and diversity, Biochem. J., 1998, 330(2), 581–598 CrossRef CAS
.
- W. Gao, B. Xing, R. Y. Tsien and J. Rao, Novel fluorogenic substrates for imaging β-lactamase gene expression, J. Am. Chem. Soc., 2003, 125(37), 11146–11147 CrossRef CAS
.
- P. F. Hall, Role of cytochromes P-450 in the biosynthesis of steroid hormones, Vitam. Horm., 1985, 42, 315–368 CrossRef CAS
.
- B. L. Marrone, D. J. Simpson, T. M. Yoshida, C. J. Unkefer, T. W. Whaley and T. N. Buican, Single cell endocrinology: analysis of P-450scc activity by fluorescence detection methods, Endocrinology, 1991, 128(5), 2654–2656 CrossRef CAS
.
- N. Nuchtavorn, J. Leanpolchareanchai, L. Suntornsuk and M. Macka, Paper-based sol-gel thin films immobilized cytochrome P450 for enzyme activity measurement, Anal. Chim. Acta, 2020, 1098, 86–93 CrossRef CAS
.
- R. Homma, T. Kimoto, Y. Niimura, A. Krivosheev, T. Hara, Y. Ohta and S. Kawato, Real-time fluorescence analysis on molecular mechanisms for regulation of cytochrome P450scc activity upon steroidogenic stimulation in adrenocortical cells, J. Inorg. Biochem., 2000, 82(1-4), 171–180 CrossRef CAS
.
- J. S. Sidhu, T. J. Kavanagh, M. T. Reilly and C. J. Omiecinski, Direct determination of functional activity of cytochrome P-4501A1 and NADPH DT-diaphorase in hepatoma cell lines using noninvasive scanning laser cytometry, J. Toxicol. Environ. Health, 1993, 40(2-3), 177–194 CrossRef CAS
.
- C. Uttamapinant, K. A. White, H. Baruah, S. Thompson, M. Fernández-Suárez, S. Puthenveetil and A. Y. Ting, A fluorophore ligase for site-specific protein labeling inside living cells, Proc. Natl. Acad. Sci. U. S. A., 2010, 107(24), 10914–10919 CrossRef CAS
.
- X. Zhang, Y. Fu, G. Qian, R. Zhang and Z. P. Xu, An artificial protein-probe hybrid as a responsive probe for ratiometric detection and imaging of hydrogen peroxide in cells, J. Mater. Chem. B, 2020, 8, 5420–5424 RSC
.
- S. Phaniraj, Z. Gao, D. Rane and B. R. Peterson, Hydrophobic resorufamine derivatives: potent and selective red fluorescent probes of the endoplasmic reticulum of mammalian cells, Dyes Pigm., 2016, 135, 127–133 CrossRef CAS
.
Footnote |
† Electronic supplementary information (ESI) available. See DOI: 10.1039/d0tb01628d |
|
This journal is © The Royal Society of Chemistry 2021 |