DOI:
10.1039/C9SC04378K
(Edge Article)
Chem. Sci., 2020,
11, 403-408
Bidirectional enantioselective synthesis of bis-benzofuran atropisomeric oligoarenes featuring two distal C–C stereogenic axes†
Received
30th August 2019
, Accepted 11th November 2019
First published on 20th November 2019
Abstract
We report the bidirectional enantioselective synthesis of bis-benzofuran atropisomeric oligoarenes featuring two distal C–C stereogenic axes obtained by a two-fold central-to-axial chirality conversion upon oxidative aromatization. The key enantioenriched centrally chiral bis-dihydrobenzofuran precursors were synthesized via a bidirectional diastereo- and enantio-selective organocatalyzed domino reaction between simple achiral and easily accessible dihydroxylated aromatics and chloronitroalkenes. Moreover, the stereodivergent nature of the methodology was established by synthesizing both diastereomers of a non-symmetrically functionalized bis-axially chiral oligoarene.
Introduction
Oligoarenes are long pi-conjugated systems commonly used in a wide range of functional materials such as organic photovoltaics, dendrimers, and self-assembling polymers.1 They are also useful as precursors of more complex polyaromatic hydrocarbons and graphene nanoribbons.2 Over the past few decades, tremendous progress has been made in the development of protocols for the synthesis of oligoarenes and polyphenylenes.3 In this field, functionalized chiral oligoarenes bearing multiple stereogenic axes are particularly attractive for materials sciences, but their efficient enantioselective synthetic access remains a challenging task.4 In the few reported methods, proximal stereogenic axes are positioned on the same central aromatic core either on a 1,2- or on a 1,4-relationship as in teraryl or bis-styryl derivatives, respectively, or on a carbon–carbon double bond in a vicinal fashion (Scheme 1a). This is the case in the pioneering contributions of the groups of Tanaka and Shibata exploiting metal-catalyzed enantioselective [2 + 2 + 2] cycloadditions of achiral alkynes.5 More recently, Sparr's6 and Zhou's7 groups astutely combined enantioselective organocatalytic activation with chirality conversion8,9 to control oligo-1,2-naphthylenes or 2,3-diarylbenzoindoles, respectively. Complementarily, Yan10 and co-workers developed an enantioselective organocatalyzed addition of arylsulfinates to an in situ generated vinylidene o-quinone methide11 for the synthesis of vicinal atropisomeric styrenes. Quite surprisingly, very little attention has been paid to the enantioselective synthesis of oligoarenes featuring two C–C stereogenic axes positioned on two distal aromatic rings also found in naturally occurring michellamines (Scheme 1b).12 In this field, Hayashi's seminal contribution employed an enantioselective double Kumada cross-coupling leading to symmetrically substituted ternaphthalene derivatives.13,14 This lack of methodologies for the enantioselective synthesis of diversely functionalized chiral oligoarenes, especially in the heteroaromatic series, leaves space for a huge untapped potential. To fill this gap, we report a new bidirectional enantioselective synthesis of bis-benzofuran atropisomeric oligoarenes featuring two distal C–C stereogenic axes.
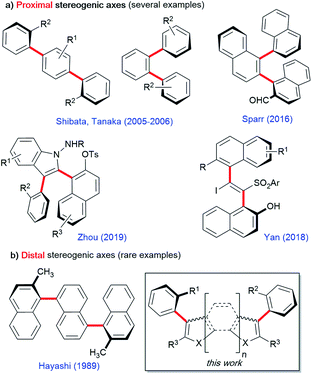 |
| Scheme 1 Enantioselective synthesis of bis-atropisomeric oligoarenes featuring either proximal (a) or distal (b) stereogenic axes. | |
Results and discussion
Our complementary bidirectional strategy starts from simple achiral and easily accessible distal dihydroxy arene derivatives 1 and chloronitroalkenes 2 (Scheme 2). Upon oxidative aromatization of bis-dihydrobenzofurans 3, a double central-to-axial chirality conversion would deliver the expected optically active S-shaped or E-shaped challenging atropisomeric bis-benzofurans 4 featuring two distal stereogenic axes. Moreover, the relatively large distance between both chiral axes of these new functional polyaromatic scaffolds will forge an interesting helically shaped molecular structure. The success of this approach lies in the combination of a two-fold diastereo- and enantio-selective organocatalyzed domino heterocyclization with double oxidative aromatization resulting in central-to-axial chirality conversion developed by our group.15 Moreover, it is expected that these two successive catalyst-controlled domino heterocyclizations would benefit a chiral amplification based on the Horeau principle,16 ensuring very high optical purities. Atropisomeric species built on a five-membered ring are quite difficult to construct in a stereoselective manner because of their lower configurational stability.17 Consequently, the synthesis of molecules displaying two fused benzofuran atropisomers would be even more rewarding.
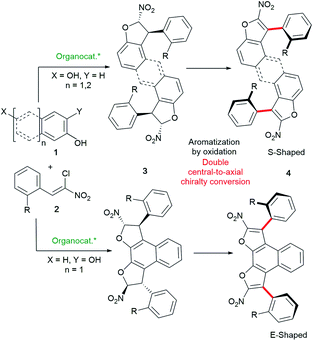 |
| Scheme 2 Bidirectional central-to-axial chirality conversion for the synthesis of S- and E-shaped bis-benzofuran atropisomeric oligoarenes 4. | |
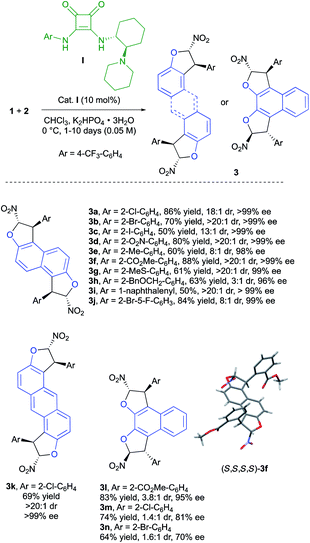 |
| Scheme 3 Control of the central chirality in bis-dihydrobenzofurans 3.19 | |
To validate these assumptions, we targeted first the synthesis of bis-dihydrobenzofurans 3 based on the enantioselective domino Friedel–Crafts-O-alkylation reaction between commercially available 2,6-dihydroxynaphthalene (1a) and various ortho-substituted (Z)-(2-chloro-2-nitroethenyl)benzenes (2).18 The use of the chiral bifunctional squaramide-tertiary amine organocatalyst (R,R)-I in combination with a weak inorganic base (K2HPO4) allowed the synthesis of the desired products 3a–k, with good to excellent diastereoselectivity and an exceptional level of enantiodiscrimination (96 to >99% ee), clearly indicating the beneficial role of the Horeau chiral amplification principle.
Importantly, the bidirectional domino bis-heterocyclization proceeds smoothly twice under catalyst control delivering mainly chiral diastereomers. However, in some cases, we observed the formation of minor meso diastereomers (3c, 3e, 3h and 3i) as a result of a competitive substrate-controlled reaction due to the bulkiness of the aryl substituent.20 Many different functional groups are well tolerated in this reaction such as electron-withdrawing substituents (3a–d, 3f, and 3j) and electron-donating ones (3e, 3g, and 3h). The introduction of a 1-naphthalenyl group in 3i was possible with excellent levels of diastereo- and enantio-selectivities, albeit in lower yield (50%). The use of anthracene-2,6-diol (1b) as the starting material gave the desired fused bis-dihydrobenzofuran 3k in good yield as virtually a single enantiomer (>20
:
1, >99% ee). To further investigate the scope of the reaction, 2,3-dihydroxynaphthalene (1c) was used as the tetra-nucleophilic partner, leading to products 3l–n. In these cases, the closer proximity of the reactive sites results in a deeper impact of the aryl moiety in 2 toward the steric control of the reaction. A rather small methyl ester function gave very good enantioselectivity (95% ee) but a lower diastereoselectivity (3.8
:
1 dr) arguing for a mismatch situation between catalyst I and the mono-dihydrobenzofuran intermediate during the second heterocyclization to 3l. A decrease of the enantioselectivity was observed when the size of the aryl substituent increased (81% ee and 70% ee for products 3m and 3n with 2-Cl-C6H4 and 2-Br-C6H4, respectively). In these two cases, the enantiodiscrimination of the first step is not as efficient, possibly due to the proximity of the two OH functions, which could disturb the hydrogen-bond network in the transition state. Despite the poor diastereoselectivity in these last few cases, both diastereomers could be easily separated by chromatography on silica gel to be engaged in the following oxidative chirality conversion step. The absolute configuration of 3f was determined to be (S,S,S,S) by single crystal X-ray crystallographic analysis.21 On this basis and with the literature precedent,22 a plausible transition state is depicted in Scheme 4. The Michael acceptor 2f is activated by the squaramide moiety of cat. I while a hydrogen bond is established between the tertiary amine of cat. I and the hydroxy function of 1a. In this rigid hydrogen bond network, a Michael addition of 1a on the Si face of nitroalkene 2f would afford the adduct A. Then, a base promoted O-cyclization would give the trans-dihydrobenzofuran B. A second organocatalyzed domino Michael/O-cyclization sequence from B delivered the observed (S,S,S,S)-3f.
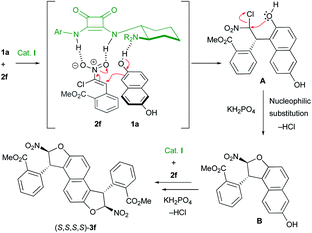 |
| Scheme 4 Proposed mechanism and transition state for the enantioselective synthesis of bis-dihydrobenzofurans 3. | |
Next the investigation of the double central-to-axial chirality conversion was carried out. The use of manganese dioxide in toluene at 0 °C allowed the clean formation of the desired S-shaped bis-benzofuran atropisomers in the naphthalene series with very good yields and good to excellent enantiomeric excesses (Scheme 5, 4a–i: 91 to >99% ee). Bis-benzofuran 4j featuring a sterically congested o,o′-disubstituted aryl moiety is nevertheless obtained albeit with reduced yield (41%) and diastereoselectivity (3.6
:
1 dr) but still an acceptable 90% ee. In contrast, the oxidation of 3k with an anthracenyl central core gave the desired bis-benzofuran 4k in good yield and almost perfect diastereo- and enantio-selectivities, positioning both stereogenic axes further away from each other. Finally, E-shaped bis-benzofuran atropisomers 4l–n with 2,3-dihydroxynaphthalene cores were obtained with similar efficiency and no erosion of the enantiomeric excess during the double conversion of chirality. The absolute configuration of product 4b was unambiguously determined to be (aS,aS) by X-ray diffraction techniques,23 accounting for a similar oxidation mechanism proposed in our previous work,15b following the Curtin–Hammett principle (Scheme 6). Hence, the two conformers 3A and 3A′ are in equilibrium with a preference for 3A′ where the sterically demanding group (blue ball) points outside from the furan subunit (see the X-ray result of 3f, Scheme 3). This most stable conformation 3A′ is apparently the least reactive under the oxidation conditions, because the hydride is shielded rendering the approach of the hydride acceptor (MnO2) unfavorable. The result is the fast generation of the oxocarbenium ion intermediate 3B. Subsequent deprotonation of the acidic hydrogen atom in 3B gives the enantioenriched 3C with efficient central-to-axial chirality conversion. Finally, iteration of this oxidative pathway from 3C gives the expected bis-furan atropisomer 4A. The meso compound 4B could come from unfavorable stereoselection in either the first or the second oxidation step, while the minor enantiomer 4C could be obtained by two successive unfavorable stereoselections.
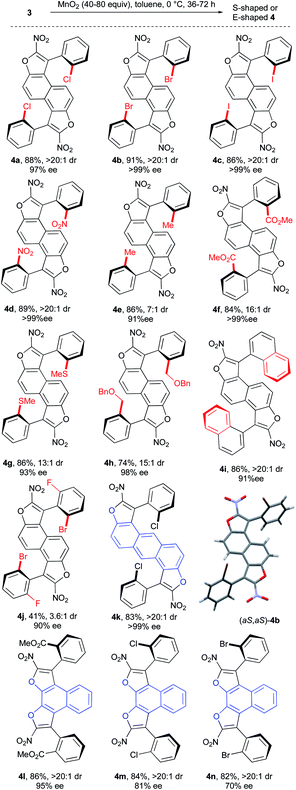 |
| Scheme 5 Scope of the double central-to-axial chirality conversion.19 | |
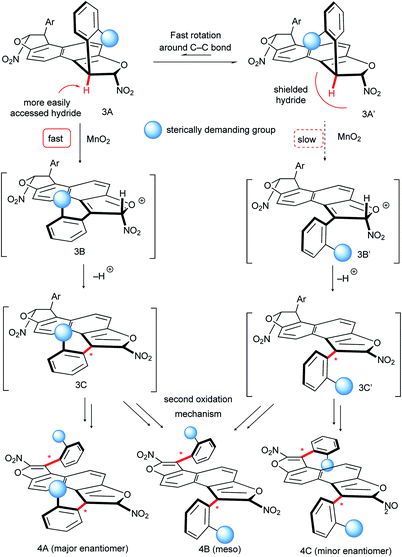 |
| Scheme 6 Mechanism of oxidation. | |
Based on the unique double catalyst-controlled bidirectionality of the process, we next target a stereodivergent approach leading to non-symmetrically functionalized S-shaped bis-atropisomeric oligoarenes (Scheme 7).24 This important feature has been addressed only once concerning molecules with multiple stereogenic axes.6b
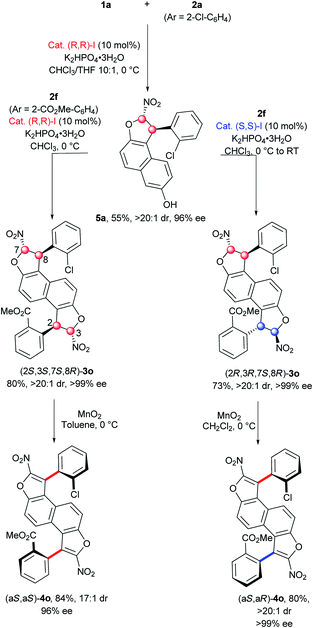 |
| Scheme 7 Stereodivergent synthesis of S-shaped bis-atropisomeric oligoarenes.19 | |
Accordingly, we investigated the possibility of incorporating two different aryl groups and access to both diastereomers. First, the mono-functionalization of 2,6-dihydroxynaphthalene (1a) with 1 equiv. of chloronitroolefin 2a (Ar = 2-Cl-C6H4) and catalyst (R,R)-I in a mixture of CHCl3/THF (10
:
1) allowed the formation of the expected mono-dihydrobenzofuran 5a bearing a 2-chlorophenyl group in 55% yield and with excellent diastereo- and enantio-selectivities (>20
:
1 dr, 96% ee). The second dihydrobenzofuran motif was constructed using the analogue 2f (Ar = 2-CO2Me-C6H4). The same catalyst gave the (2S,3S,7S,8R)-3o product in good yield (80%) and excellent stereoselectivity, while its enantiomer (S,S)-I produced the diastereomer (2R,3R,7S,8R)-3o in equally good yield (78%) and with perfect stereocontrol indicating absolutely no mismatch effect between 5a and the (S,S)-I catalyst. Then the oxidation step was accomplished on both diastereomers of 3o. Gratifyingly, the corresponding bis-benzofuran atropisomers (aS,aS)-4o and (aS,aR)-4o were obtained in good yields and excellent stereocontrol, with only a slight erosion of the diastereomeric ratio for (aS,aS)-4o (17
:
1 dr). Moreover, this non-symmetric synthesis shows the possibility of constructing original functionalized π-conjugated push–pull systems, with high potential in materials science.25
Finally, a step forward was taken by synthesizing more complex oligoarenes bearing two stereogenic axes even more distant form each other. This was possible starting from bis-naphthobenzofurandiols 1d and 1e. Compounds 3p and 3q were obtained in low yields (26% and 35%, see the ESI†) and still good to excellent enantioselectivity for the chiral diastereomer (88% and 98% ee) but with an unusual amount of the meso one (1
:
1.4 and 3
:
1 dr). Nevertheless, the chiral diastereomers could be separated by chromatography and engaged in the oxidative aromatization step (Scheme 8). Thus, the extended bis-benzofuran atropisomeric oligoarenes 4p and 4q were obtained in very good yields without erosion of the enantiomeric excess, accounting for a perfect double central-to-axial chirality conversion.
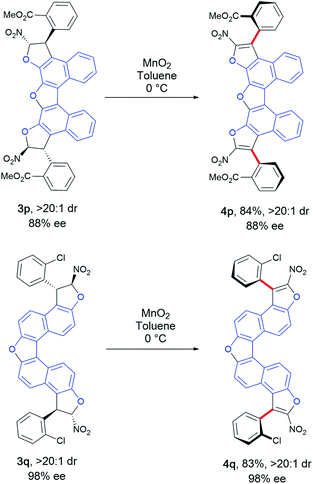 |
| Scheme 8 Synthesis of more complex bis-benzofuran atropisomeric oligoarenes 4p and 4q.19 | |
Conclusions
We have developed a unique bidirectional catalyst-controlled methodology allowing highly enantioselective access to hitherto unknown S- and E-shaped bis-benzofuran atropisomeric oligoarenes featuring two distal C–C stereogenic axes. The key feature lies in a successful double central-to-axial chirality conversion after organocatalyzed activation of simple and easily accessible achiral fused dihydroxyarenes and functionalized nitroolefins. This makes possible an unusual stereodivergent approach to these valuable new molecules featuring two different distal axes of chirality.
Conflicts of interest
There are no conflicts to declare.
Acknowledgements
Financial support from the Centre National de la Recherche Scientifique (CNRS), Aix-Marseille Université and Centrale Marseille is gratefully acknowledged. We thank Dr Daniel Dauzonne (Institut Curie) for the synthesis and generous gift of some initial chloronitroalkenes in multigram quantities and also for the know-how in the preparation of these species. We thank Dr Vivek S. Raut for preliminary studies on bidirectional dihydrobenzofuran synthesis. We also thank Dr Nicolas Vanthuyne and Ms Marion Jean (Aix-Marseille Université) for HPLC methods and Dr Michel Giorgi (Aix-Marseille Université) for the X-ray structural analysis.
Notes and references
- For reviews, see:
(a) C. Li, M. Liu, N. G. Pschirer, M. Baumgarten and K. Müllen, Chem. Rev., 2010, 110, 6817–6855 CrossRef CAS PubMed;
(b) Y. Lin, Y. Lia and X. Zhan, Chem. Soc. Rev., 2012, 41, 4245–4272 RSC;
(c) G. Franc and A. K. Kakkar, Chem. Soc. Rev., 2010, 39, 1536–1544 RSC;
(d) X. Zhang, T. Higashihara, M. Ueda and L. Wang, Polym. Chem., 2014, 5, 6121–6141 RSC.
-
(a) A. Narita, X.-Y. Wang, X. Feng and K. Müllen, Chem. Soc. Rev., 2015, 44, 6616–6643 RSC;
(b) A. Bohnen, K.-H. Koch, W. Lüttke and K. Müllen, Angew. Chem., Int. Ed. Engl., 1990, 29, 525–527 CrossRef.
-
(a) S. J. Hein, D. Lehnherr, H. Arslan, F. J. Uribe-Romo and W. R. Dichtel, Acc. Chem. Res., 2017, 50, 2776–2788 CrossRef CAS PubMed;
(b) M. Arseneault, C. Wafer and J.-F. Morin, Molecules, 2015, 20, 9263–9294 CrossRef CAS PubMed;
(c) Q. Fan, J. M. Gottfried and J. Zhu, Acc. Chem. Res., 2015, 48, 2484–2494 CrossRef CAS PubMed.
- For diastereoselective strategies to optically active derivatives, see:
(a) N.-X. Hu, S. Xie, Z. Popovic, B. Ong and A.-M. Hor, J. Am. Chem. Soc., 1999, 121, 5097–5098 CrossRef CAS;
(b) K. Takaishi, M. Kawamoto and K. Tsubaki, Org. Lett., 2010, 12, 1832–1835 CrossRef CAS PubMed;
(c) Q. Dherbassy, J.-P. Djukic, J. Wencel-Delord and F. Colobert, Angew. Chem., Int. Ed., 2018, 57, 4668–4672 CrossRef CAS PubMed;
(d) G. Beutner, R. Carrasquillo, P. Geng, Y. Hsiao, E. C. Huang, J. Janey, K. Katipally, S. Kolotuchin, T. La Porte, A. Lee, P. Lobben, F. Lora-Gonzalez, B. Mack, B. Mudryk, Y. Qiu, X. Qian, A. Ramirez, T. M. Razler, T. Rosner, Z. Shi, E. Simmons, J. Stevens, J. Wang, C. Wei, S. R. Wisniewski and Y. Zhu, Org. Lett., 2018, 20, 3736–3740 CrossRef CAS PubMed;
(e) T. Yanagi, K. Nogi and H. Yorimitsu, Synlett, 2019 DOI:10.1055/S-0037-1611767.
-
(a) T. Shibata, T. Fujimoto, K. Yokota and K. Takagi, J. Am. Chem. Soc., 2004, 126, 8382–8383 CrossRef CAS PubMed;
(b) T. Shibata and K. Tsuchikama, Chem. Commun., 2005, 6017–6019 RSC;
(c) T. Shibata, K. Tsuchikama and M. Otsuka, Tetrahedron: Asymmetry, 2006, 17, 614–619 CrossRef CAS;
(d) K. Tanaka, T. Suda, K. Noguchi and M. Hirano, J. Org. Chem., 2007, 72, 2243–2246 CrossRef CAS PubMed. For related molecules exhibiting both C–C and C–N vicinal axial chiralities, see:
(e) J. Oppenheimer, R. P. Hsung, R. Figueroa and W. L. Johnson, Org. Lett., 2007, 9, 3969–3972 CrossRef CAS PubMed.
-
(a) D. Lotter, M. Neuburger, M. Rickhaus, D. Häussinger and C. Sparr, Angew. Chem., Int. Ed., 2016, 55, 2920–2923 CrossRef CAS PubMed;
(b) D. Lotter, A. Castrogiovanni, M. Neuburger and C. Sparr, ACS Cent. Sci., 2018, 4, 656–660 CrossRef CAS PubMed.
- Y.-L. Hu, Z. Wang, H. Yang, J. Chen, Z.-B. Wu, Y. Lei and L. Zhou, Chem. Sci., 2019, 10, 6777–6784 RSC.
- For seminal studies, see:
(a) J. A. Berson and E. Brown, J. Am. Chem. Soc., 1955, 77, 450–453 CrossRef CAS;
(b) T. R. Hollands, P. de Mayo, M. Nisbet and P. Crabbé, Can. J. Chem., 1965, 43, 3008–3017 CrossRef CAS;
(c) E. W. Warnhoff and S. V. Lopez, Tetrahedron Lett., 1967, 28, 2723–2727 CrossRef;
(d) A. I. Meyers and D. G. Wettlaufer, J. Am. Chem. Soc., 1984, 106, 1135–1136 CrossRef CAS.
- For other recent examples of central-to-axial chirality conversion, see:
(a) F. Guo, L. C. Konkol and R. J. Thomson, J. Am. Chem. Soc., 2011, 133, 18–20 CrossRef CAS PubMed;
(b) L. C. Konkol, F. Guo, A. A. Sarjeant and R. J. Thomson, Angew. Chem., Int. Ed., 2011, 50, 9931–9934 CrossRef CAS PubMed;
(c) G.-Q. Li, H. Gao, C. Keene, M. Devonas, D. H. Ess and L. Kürti, J. Am. Chem. Soc., 2013, 135, 7414–7417 CrossRef CAS PubMed;
(d) Y.-H. Chen, D.-J. Cheng, J. Zhang, Y. Wang, X.-Y. Liu and B. Tan, J. Am. Chem. Soc., 2015, 137, 15062–15065 CrossRef CAS PubMed;
(e) J.-Z. Wang, J. Zhou, C. Xu, H. Sun, L. Kürti and Q.-L. Xu, J. Am. Chem. Soc., 2016, 138, 5202–5205 CrossRef CAS PubMed;
(f) M. Moliterno, R. Cari, A. Puglisi, A. Antenucci, C. Esperandio, E. Moretti, A. Di Sabato, R. Salvio and M. Bella, Angew. Chem., Int. Ed., 2016, 55, 6525–6529 CrossRef CAS PubMed;
(g) Y.-H. Chen, L.-W. Qi, F. Fang and B. Tan, Angew. Chem., Int. Ed., 2017, 56, 16308–16312 CrossRef CAS PubMed;
(h) H.-H. Zhang, C.-S. Wang, C. Li, G.-J. Mei, Y. Li and F. Shi, Angew. Chem., Int. Ed., 2017, 56, 116–121 CrossRef CAS PubMed;
(i) S. Maddox, G. A. Dawson, N. C. Rochester, A. B. Ayonon, C. E. Moore, A. L. Rheingold and J. L. Gustafson, ACS Catal., 2018, 8, 5443–5447 CrossRef CAS PubMed;
(j) L. W. Qi, J.-H. Mao, J. Zhang and B. Tan, Nat. Chem., 2018, 10, 58–64 CrossRef CAS PubMed.
-
(a) Y. Tan, S. Jia, F. Hu, Y. Liu, L. Peng, D. Li and H. Yan, J. Am. Chem. Soc., 2018, 140, 16893–16898 CrossRef CAS PubMed;
(b) During the proof reading of this article, Yan and co-workers reported the enantioselective synthesis of compounds bearing both helical and axial stereogenic elements: S. Jia, S. Li, Y. Liu, W. Qin and H. Yan, Angew. Chem., Int. Ed., 2019 DOI:10.1002/anie.201909214.
- J. Rodriguez and D. Bonne, Chem. Commun., 2019, 55, 11168–11170 RSC.
- G. Bringmann, R. Götz, S. Harmsen, J. Holenz and R. Walter, Liebigs Ann., 1996, 2045–2058 CrossRef CAS.
- T. Hayashi, K. Hayashizaki and Y. Ito, Tetrahedron
Lett., 1989, 30, 215–218 CrossRef CAS.
- A non-stereoselective double Suzuki reaction has also been employed for the total synthesis of naturally occurring racemic michellamines:
(a) G. Bringmann, R. Götz, P. A. Keller, R. Walter, M. R. Boyd, F. Lang, A. Garcia, J. J. Walsh, I. Tellitu, K. V. Bhaskar and T. R. Kelly, J. Org. Chem., 1998, 63, 1090–1097 CrossRef CAS;
(b) P. D. Hobbs, V. U. Upender and M. I. Dawson, Synlett, 1997, 965–967 CrossRef CAS.
-
(a) O. Quinonero, M. Jean, N. Vanthuyne, C. Roussel, D. Bonne, T. Constantieux, C. Bressy, X. Bugaut and J. Rodriguez, Angew. Chem., Int. Ed., 2016, 55, 1401–1405 CrossRef CAS PubMed;
(b) V. S. Raut, M. Jean, N. Vanthuyne, C. Roussel, T. Constantieux, C. Bressy, X. Bugaut, D. Bonne and J. Rodriguez, J. Am. Chem. Soc., 2017, 139, 2140–2143 CrossRef CAS.
- J. P. Vigneron, M. Dhaenens and A. Horeau, Tetrahedron, 1973, 29, 1055–1059 CrossRef CAS . For a recent review, see: A. M. Harned, Tetrahedron, 2018, 74, 3797–3841 CrossRef.
-
(a) D. Bonne and J. Rodriguez, Chem. Commun., 2017, 53, 12385–12393 RSC;
(b) D. Bonne and J. Rodriguez, Eur. J. Org. Chem., 2018, 2417–2431 CrossRef CAS. For recent contributions, see:
(c) S.-C. Zheng, Q. Wang and J. Zhu, Angew. Chem., Int. Ed., 2019, 58, 1494–1498 CrossRef CAS PubMed;
(d) Y. Kwon, J. Li, J. P. Reid, J. M. Crawford, R. Jacob, M. S. Sigman, F. D. Toste and S. J. Miller, J. Am. Chem. Soc., 2019, 141, 6698–6705 CrossRef CAS PubMed;
(e) H. Li, X. Yan, J. Zhang, W. Guo, J. Jiang and J. Wang, Angew. Chem., Int. Ed., 2019, 58, 6732–6736 CrossRef CAS PubMed;
(f) X.-L. He, H.-R. Zhao, X. Song, B. Jiang, W. Du and Y.-C. Chen, ACS Catal., 2019, 9, 4374–4381 CrossRef CAS.
-
(a) C. Jarava-Barrera, F. Esteban, C. Navarro-Ranninger, A. Parra and J. Alemán, Chem. Commun., 2013, 49, 2001–2003 RSC;
(b) M. Rueping, A. Parra, U. Uria, F. Besselièvre and E. Merino, Org. Lett., 2010, 12, 5680–5683 CrossRef CAS;
(c) D. Becerra, W. Raimondi, D. Dauzonne, T. Constantieux, D. Bonne and J. Rodriguez, Synthesis, 2017, 49, 195–201 CAS;
(d) For the synthesis of achiral furans from (Z)-(2-halo-2-nitroethenyl)benzenes, see: W. Raimondi, D. Dauzonne, T. Constantieux, D. Bonne and J. Rodriguez, Eur. J. Org. Chem., 2012, 6119–6123 CrossRef CAS.
- Yield of the isolated product. The diastereoselectivity was determined by 1H NMR spectroscopy of the crude reaction mixture. The enantiomeric excess was determined by HPLC analysis on a chiral stationary phase.
- See the ESI.†.
-
(a) X.-L. Zhang, K.-X. Feng, A.-B. Xia, Y.-Y. Zheng, C. Li, X.-H. Du and D.-Q. Xu, Eur. J. Org. Chem., 2018, 2918–2925 CrossRef CAS;
(b) J. Feng, L. Lin, K. Yu, X. Liu and X. Feng, Adv. Synth. Catal., 2015, 357, 1305–1310 CrossRef CAS.
- CCDC 1922303 (3f) contains the supplementary crystallographic data for this paper.†.
- CCDC 1921425 (4b) contains the supplementary crystallographic data for this paper.†.
- S. Krautwald and E. M. Carreira, J. Am. Chem. Soc., 2017, 139, 5627–5639 CrossRef CAS PubMed.
-
(a) F. Bures, RSC Adv., 2014, 4, 58826–58851 RSC;
(b) M. Kivala and F. Diederich, Acc. Chem. Res., 2009, 42, 235–248 CrossRef CAS PubMed.
Footnote |
† Electronic supplementary information (ESI) available. CCDC 1922303 and 1921425. For ESI and crystallographic data in CIF or other electronic format see DOI: 10.1039/c9sc04378k |
|
This journal is © The Royal Society of Chemistry 2020 |
Click here to see how this site uses Cookies. View our privacy policy here.