DOI:
10.1039/D0RA09359A
(Paper)
RSC Adv., 2020,
10, 45059-45066
pH-sensitive zwitterionic polycarboxybetaine as a potential non-viral vector for small interfering RNA delivery
Received
3rd November 2020
, Accepted 1st December 2020
First published on 21st December 2020
Abstract
Small interfering RNA (siRNA) has great potential for the treatment of various diseases. However, its intrinsic deficiencies seriously limit its application. Herein, pH-sensitive zwitterionic polymer polycarboxybetaine (PCB) was developed as a non-viral vector for siRNA. The PCB could be protonated in an acidic environment and become positively charged from a cancer site. After protonation, PCB could complex siRNA via electrostatic interaction, and its loading ability was enhanced with a decrease of pH value. Compared with the PEI 10k, PCB50 with a similar molecular weight had comparable siRNA loading ability and lower cytotoxicity. Besides, siRNA loaded by PCB50 could escape from endosomes and reduce the loss of drugs, and based on the excellent uptake and obvious apoptotic effect on HeLa cells, the pH-sensitive PCB with low cytotoxicity could be used as a non-viral vector for safe siRNA delivery for cancer treatment.
1. Introduction
Small interfering RNA (siRNA) is mainly involved in the phenomenon of RNA interference (RNAi) and regulates the expression of genes.1–3 SiRNA has great value and potential in the treatment of various diseases. However, siRNA in blood is easily degraded by enzymes and has a short plasma half-life.4–6 In addition, its intrinsic properties such as large size (about 13 kDa) and negative surface charge limit entry permeability of cell membranes.7–10 Therefore, the therapeutic application of siRNA is limited and based on the development of efficient and safe carriers.
Nowadays, researchers have been vigorously developing safe and effective vectors for siRNA delivery. The vectors can be divided into viral vectors and non-viral vectors.6,11,12 However, the shortcomings of viral vectors such as immunogenic and inflammatory responses restrict their application.13,14 Among non-viral vectors, polyethylenimine (PEI) is widely used for siRNA delivery due to its excellent loading ability.15–19 PEI is positively charged under physiological condition, and can electrostatically interact with negatively charged siRNA to form PEI/siRNA polyplexes.20 However, the high cytotoxicity seriously limits its practical application.21
Zwitterionic polymer polycarboxybetaine (PCB) has positive quaternary ammonium group and negative carboxyl acid group alone, which is neutral under physiological environment.22–25 PCB can form a strong hydrated layer through electrostatic interaction and can be highly resistant to the adsorption of non-specific proteins in body.26–28 Our previous research has proved that PCB is pH-sensitive and its negative carboxyl acid group can be protonated in acidic environment, which is beneficial for the endosomal/lysosomal escape of siRNA.29 After protonation, PCB is positively charged due to their quaternary ammonium group, which might electrostatically interact with negatively charged siRNA as PEI. Therefore, zwitterionic PCB has the potential to be a non-viral vector for siRNA delivery.
Herein, to confirm this, we used PCB to complex siRNA, and evaluated its loading ability and cytotoxicity. The experimental results at the cellular level showed that PCB/siRNA polyplex can be endocytosed by cells in an acidic environment. Endosomal/lysosomal escape occurred to reduce the loss of therapeutic siRNA, and further cell therapy showed that the polyplex of PCB with siPLK1 had excellent value in inducing cancer cell apoptosis.
2. Experimental
2.1 Materials
2-(N,N′-Dimethylamino)ethyl methacrylate (DMAEMA, 98%) was obtained from Alfa Aesar. β-Propiolactone (98%) was purchased from J&K Scientific Ltd. 2,2′-Azobis(2-methylpropionitrile) (AIBN), 2-cyanopropan-2-yl benzodithioate and (3-(4,5-dimethylthiazol-2-yl)-2,5-diphenyltetrazolium bromide) (MTT) were obtained from Sigma Aldrich. HBSS solutions were obtained from Gibco, Lipofectamine 3000 was purchased from Invitrogen. Cy5-siRNA was purchased from Jill Biochemical Reagent Company. Antibodies against human polo-like kinase 1 (Plk1) was obtained from Affinity. Goat anti-rabbit, BCA Protein Assay Kit, Bradford Protein Assay and lysis buffer (RIPA) were purchased from Beyotime (China). siRNAs targeting human Plk1 (siPlk1) (sense strand, 5′-UGAAGAAGAUCACCCUCCUUAdTdT-3′; antisense strand, 5′-UAAGGAGGGUGAUCUUCUUCAdTdT-3′) and scrambled siRNA (siNonsense) (sense strand, 5′-UUCUCCGAACGUGUCACGUdTdT-3′; antisense strand, 5′-ACGUGACACGUUCGGAGAAdTdT-3′) were supplied by Su Zhou Ribo Life Science Co. Ltd. (Suzhou, China). ANNEXIN V-FITC/PI Apoptosis Detection Kit was obtained from Solarbio (Beijing, China). Dulbecco's Modified Eagle Medium (DMEM), penicillin (10
000 U mL−1), streptomycin (10 mg mL−1), trypsin–EDTA and fetal bovine serum (FBS) were purchased from Thermo. All other reagents used were obtained commercially at analytical grade.
2.2 Synthesis of CB monomer
Carboxybetaine (CB) monomer was synthesized by the method reported previously in our lab. Briefly, β-propiolactone (0.43 g, 6 mmol) in 5 mL of dried methylene chloride was added to 25 mL of dried dichloromethane containing DMAEMA (0.79 g, 5 mmol). The reaction mixture was stirred under nitrogen protection at 10 °C for 12 h. The white precipitate was washed with 50 mL of dried methylene chloride and 50 mL of anhydrous acetone. The product was then dried under reduced pressure to obtain the CB monomer and the yield could reach 82.0%.
2.3 Synthesis of PCB
The synthesis of PCB was completed by reversible addition–fragmentation chain transfer polymerization (RAFT). The following used PCB20 as an example. The chain transfer agent 2-cyanopropan-2-yl benzodithioate (10.62 mg, 0.05 mmol), CB monomer (343.91 mg, 1.5 mmol) and AIBN (2.66 mg) were dissolved in 5 mL of anhydrous methanol and the mixture was added into Schlenk bottle. After three cycles of freezing, degassing and thawing, the reaction system was stirred under nitrogen protection at 60 °C for 24 h. The final product was dialyzed and lyophilized to obtain PCB20, and the yield of PCB was 75.0%. PCB with other degree of polymerization was obtained by the same method with different weight of CB monomer.
2.4 The buffering capacity of PCB
The buffering capacity of PCB was detected using acid–base titration. The PCB was dissolved in 0.01 M NaCl solution. The pH of the solution was gradually adjusted to 10 with 1 M NaOH solution. 0.1 M HCl solution with a volume of 10 μL was then added dropwise to this solution. At each time it was added, the pH value was measured with a pH meter and recorded.
2.5 Preparation and characterization of PCB/siRNA polyplex
PCB was dissolved in citrate buffer with different pH values or phosphate buffer saline (PBS). PCB and siRNA were added together at various N/P (N: nitrogen portion of PCB; P: phosphorus portion of siRNA) ratios for 0.5 h at room temperature to prepare PCB/siRNA polyplexes. The average particle size and zeta potential of polyplexes were determined by dynamic light scattering (Zetasizer Nano ZS instrument, Malvern Instruments). The morphological analysis of the polyplexes was further performed by transmission electron microscopy (JEM-2100 electron microscope).
2.6 Agarose gel electrophoresis
Agarose gel electrophoresis was used to investigate the complexing ability of PCB and PEI to siRNA. PCB/siRNA polyplexes and PEI/siRNA polyplexes with different N/P ratios were mixed with loading buffer. The mixtures were then added to the agarose gel well. The electrophoresis was carried out at an electrophoresis voltage of 100 V for 10 min at room temperature. The results were observed with a gel imager.
2.7 Determination of cytotoxicity by MTT method
In order to measure the cytotoxicity of PCB/siRNA polyplexes and PEI/siRNA polyplexes, MTT cell viability assay was performed on HeLa cells. HeLa cells were seeded in 96-well plates at a concentration of 4 × 103 cells per well. To determine the cytotoxicity of the polyplexes, the polyplexes with 0.1 μg siRNA at various N/P ratios were added to culture medium with total volume of 100 μL. After incubating for 24 h, 20 μL of MTT solution (5 mg mL−1 in PBS) was added to each well and incubated at 37 °C for another 4 h. The medium and MTT were then replaced with 100 μL of DMSO. The samples were incubated at 37 °C for 5 minutes to dissolve MTT formazan. The plate was shaken gently for 10 minutes to ensure dissolution of formazan. The absorbance was measured at 490 nm using a Tecan microplate reader (Tecan, Switzerland).
2.8 Cellular uptake of PCB/siRNA polyplex
The method for evaluating the degree of endocytosis of PCB/siRNA polyplex by HeLa cells is as follows. PCB/siRNA polyplex (including 4 μg Cy5-siRNA per well) and HeLa cells were co-cultured in 24-well plate in HBSS solution of pH 6.5 and 7.4 respectively. After 1, 2, 4 and 6 h, cells were washed with PBS and the collected cells were then assessed with BD Calibur flow cytometry to test the fluorescence intensity of Cy5 in HeLa cell.
2.9 Endosomal/lysosomal escape of PCB/siRNA polyplex
2 × 105 HeLa cells were seeded in 35 mm Petri dishes (Cellvis) for 24 h. PCB/siRNA polyplex containing 4 μg Cy5-siRNA was added to the Petri dishes, and incubated at 37 °C for 2 and 6 h, respectively. The cells were washed three times with PBS and followed by staining with LysoTracker Red for 30 min at 37 °C. The cells were then washed three times with PBS and fixed with 4% paraformaldehyde for 10 min at 4 °C. Finally, the nuclei were stained with DAPI for 15 min at 37 °C after washing three times with PBS. The fluorescence images were taken by CLSM (Zeiss Co., Germany).
2.10 Western blot analysis
Briefly, HeLa cells were seeded in a 6-well plate at 2 × 105 cells per well at 37 °C and cultured for 24 h. The medium was replaced with a fresh medium containing the PCB/siRNA polyplex (siPLK1) (4 μg siRNA per well). After culturing at 37 °C for 48 h, the sample buffer (8% SDS, 0.25 M Tris–HCl (pH 6.8), 40% glycerol, 5% 2-mercaptoethanol and 0.04% bromophenol blue), was boiled at 95 °C for 10 minutes. The proteins were resolved by SDS-polyacrylamide gel electrophoresis (SDS-PAGE), transferred to polyvinylidene fluoride membranes, blocked in 5% non-fat powdered milk in PBS-T (0.5% Tween-20), and probed with antibodies. They were incubated with antibodies (Affinity) and detected by X-ray film after incubated with enhanced chemiluminescence reagent (ECL).
2.11 Apoptosis assay
To assess the induction of the apoptotic cells after treatment with PCB/siRNA polyplex (siPLK1), the HeLa cells were treated with FITC-Annexin V and propidium iodide (PI) (Solarbio) according to the manufacturer's protocol after 48 h of incubation. The stained cells were immediately acquired using a flow cytometer.
2.12 Statistical analysis
Statistical analysis was performed by using the Student's t-test with p < 0.05 as significant difference. The experimental results were given in the format of mean ± SD in the figures.
3. Results and discussion
3.1 The synthesis of PCB polymers
As shown in Fig. 1a, PCB was synthesized by RAFT using 2-cyanopropan-2-yl benzodithioate as the chain transfer agent and CB as the monomer. The final PCB product obtained was characterized by 1H NMR, which confirmed its successful synthesis (Fig. 1b). The gel permeation chromatography (GPC) curves of the purified PCB20 and PCB50 confirmed the successful polymerization (Fig. 1c).
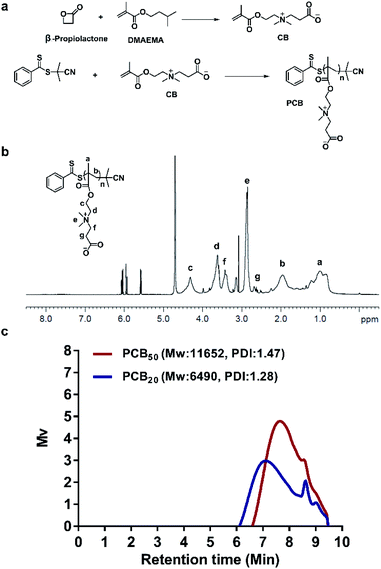 |
| Fig. 1 (a) The synthesis routes of PCB. (b) 1H NMR spectrum of PCB. (c) The GPC curves of PCB20 and PCB50. | |
3.2 Preparation and characterization of PCB/siRNA polyplex
The buffering capacity of PCB with degree of polymerization of 20 and 50 (PCB20 and PCB50) was investigated by acid–base titration in 0.01 M NaCl aqueous solution. As shown in Fig. 2a, PCB had a good buffering capacity over the pH range of 7.4 to 3.5, and the buffering capacity of PCB50 was better than that of PCB20. Next, the siRNA complexing ability of PCB20 in different pH values was detected by gel retardation assay. PCB20 was dissolved in citrate buffers with pH 3, 4, 5, 6 and PBS with pH 7.4, respectively. As shown in Fig. 2b, the siRNA loading ability of PCB20 was enhanced with the decrease of pH values. PCB20 could not completely complex siRNA at pH 7.4 even at N/P ratio of 40. In comparison, it could completely complex siRNA at N/P ratio of 20 at pH 4.0 and at N/P ratio of 10 at pH 3.0. However, the low pH value might affect the stability of siRNA. Therefore, pH 4.0 was chosen for the following experiments.
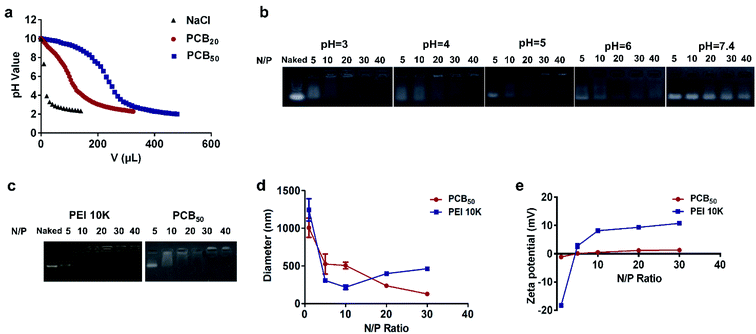 |
| Fig. 2 (a) The buffering capacity of PCB20 and PCB50. (b) Agarose gel electrophoresis retardation assay of PCB20 under different pH conditions. (c) Agarose gel electrophoresis retardation assay of PCB50 and PEI 10k. (d) The average particle diameter of polyplexes at different N/P ratios. (e) The average zeta potential of polyplexes at different N/P ratios. The mean ± SD was shown (n = 3). | |
In order to more reliably prove the performance of PCB, PCB50 with molecular weight roughly equivalent to that of the commercially available product PEI 10k was used to study its effect on the siRNA compounding ability. As shown in Fig. 2c, the results showed that the siRNA compounding ability of PCB50 at pH 4.0 was comparable to that of PEI 10k. PCB50 could completely complex siRNA at N/P ratio of 5, and its compounding ability was better than PCB20 at the same pH value. Subsequently, the average particle diameter and zeta potential of the both polyplexes were determined by dynamic light scattering (DLS). As shown in Fig. 2d, the diameter of PCB50/siRNA complexes were decreased with the increasing of N/P ratios. It was 128.2 nm at N/P ratio of 30. Their zeta potential was nearly zero. In comparison, the zeta potential of PEI/siRNA polyplexes was increased with the increase of N/P ratios. It was 10.7 mV at N/P ratio of 30 (Fig. 2e). The high surface charge might lead to high cytotoxicity.
In order to further determine the morphology of the PCB50/siRNA polyplexes at N/P ratio of 30, the morphological analysis was further performed by transmission electron microscopy. As shown in Fig. 3a, the PCB50/siRNA polyplexes had a spherical structure. Therefore, these results confirmed that the protonated PCB under acidic environment could complex siRNA to form a nano-scale spherical structure (Fig. 3b).
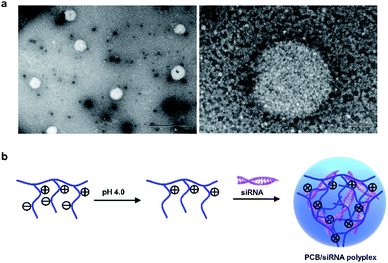 |
| Fig. 3 (a) TEM images of PCB50/siRNA polyplex at N/P ratio of 30. Scale bar (left): 1 μm. Scale bar (right): 100 nm. (b) Schematic diagram of the construction of PCB/siRNA polyplex. | |
3.3 Cytotoxicity and cellular uptake of the PCB/siRNA polyplex
Furthermore, the cytotoxicity of both polyplexes was detected by MTT assay. As shown in Fig. 4, PCB50/siRNA polyplexes almost did not change the cell viability with the increase of N/P ratios. In comparison, the cell viability was decreased for PEI 10k/siRNA polyplexes with the increase of N/P ratios. It was obviously that the cell viability of PCB50/siRNA polyplexes groups were better than that of PEI 10k/siRNA polyplexes at the same higher N/P ratios. The result indicated that PCB50/siRNA polyplexes were good biocompatible.
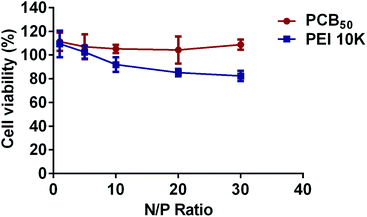 |
| Fig. 4 The cytotoxicity of polyplexes at different N/P ratios determined by MTT assay. The mean ± SD was shown (n = 3). | |
In HBSS solutions with pH of 6.5 and 7.4, respectively, HeLa cells were used to further determine the endocytosis effect of PCB50/Cy5-siRNA polyplex. As shown in Fig. 5, PCB50/siRNA polyplex showed more cellular uptake under the condition of pH 6.5, PCB50/siRNA polyplex exhibited the characteristics of easier entry into cells under slightly acidic conditions, which made the polyplex more suitable for tumor therapy. PCB50/siRNA polyplex was significantly more endocytosed by cells than free siRNA. Compared with PEI 10k under the environment of pH 6.5, the cell uptake rate of these two was comparable at 1 and 2 h. However, after 4 h, the endocytosis of PCB50/siRNA polyplex was weaker than that of PEI 10k/siRNA due to its lower surface positive charge.
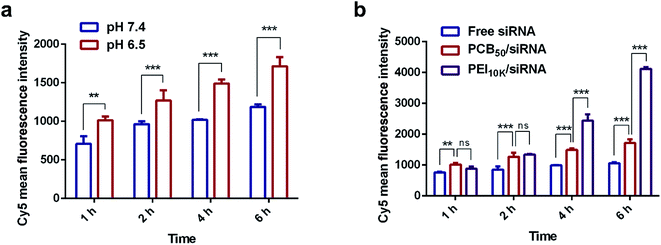 |
| Fig. 5 (a) The cellular uptake of PCB50/Cy5-siRNA by HeLa cells under the conditions of pH 6.5 and pH 7.4. (b) Comparison of uptake of PCB50/Cy5-siRNA, free Cy5-siRNA and PEI 10k/Cy5-siRNA by HeLa cells under the condition of pH 6.5. The mean ± SD was shown (n = 3) (*P < 0.05, **P < 0.01, ***P < 0.001). | |
3.4 Endosomal/lysosomal escape of the PCB/siRNA polyplex
The localization of polyplex in cells was observed by staining endosomes and early lysosomes with LysoTracker Red. HeLa cells were incubated with PCB50/Cy5-siRNA polyplex for 2 and 6 h, respectively. The CLSM analysis (Fig. 6) showed that red spots were observed within the HeLa cells and polyplex were mainly colocalized with the LysoTracker Red stained organelles after 2 h of incubation. Subsequently, to investigate whether PCB50/Cy5-siRNA polyplex could efficiently escape from the endosomes/lysosome, PCB50/Cy5-siRNA polyplex were incubated with HeLa cells for 6 h. As shown in Fig. 6, the separation of the green and red fluorescence spots was more significant, suggesting that PCB50/Cy5-siRNA polyplex could efficiently escape from the endosomes or early lysosomes to cytoplasm.
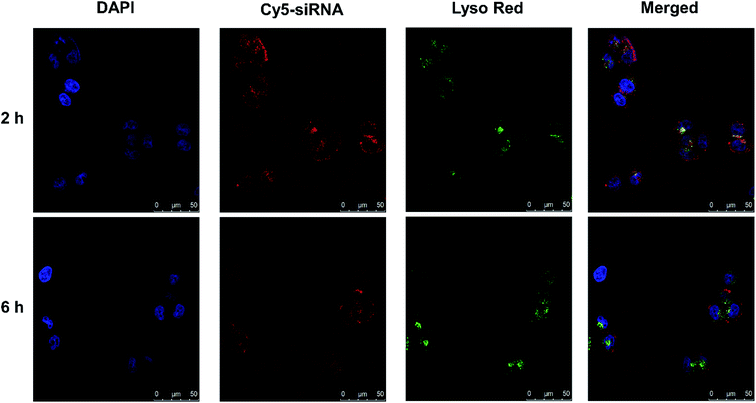 |
| Fig. 6 CLSM images of endosomal/lysosomal escape of Cy5-siRNA in HeLa cells for 2 h and 6 h of incubation. For each row, the images from left to right were cell nuclei stained by DAPI (blue), Cy5-siRNA (red), lysosome stained by LysoTracker Red (green), and overlays of the three images. The bar represents 50 μm. | |
3.5 Gene silencing ability
In order to further reveal the gene silencing activity of the polyplex, HeLa cells were incubated with the PCB50/siPLK1 complex for 48 h. Western blot analysis in Fig. 7a and b confirmed that PCB50/siPLK1 polyplexes could enhance the efficiency of siPLK1 and reduce the level of PLK1 protein.
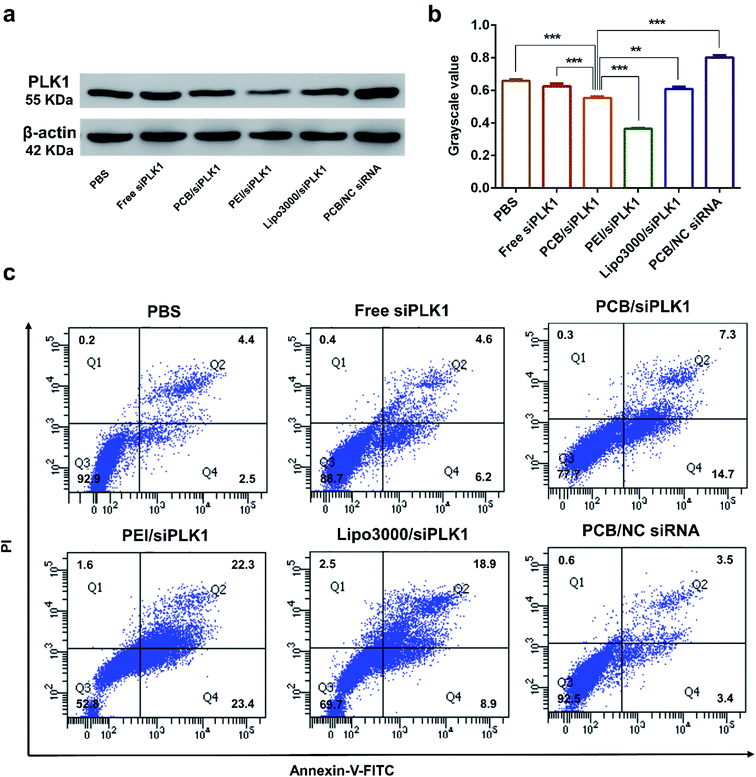 |
| Fig. 7 Gene silencing effect. (a) Western blot assay of PBS, free siPLK1, PCB/siPLK1, PEI/siPLK1, Lipofectamine 3000/siPLK1 and PCB/NC siRNA. (b) Relative quantitative value of gray value of western blot results. (c) Apoptosis assay of HeLa cells after 48 h incubation with PBS, free siPLK1, PCB/siPLK1, PEI/siPLK1, Lipofectamine 3000/siPLK1 and PCB/NC siRNA. The mean ± SD was shown (n = 3) (*P < 0.05, **P < 0.01, ***P < 0.001). | |
PLK1 protein is a key player in mitosis and its down-regulation can increase the apoptosis of tumor cells. As shown in Fig. 7c, the apoptosis study using FITC-Annexin V and PI double staining showed that PCB50/siPLK1 complex induced significant apoptosis of HeLa cells (≈22.0%). Although it only reached half of the commonly used PEI 10k/siPLK1 (≈45.7%) apoptosis rate, it was compared to the transfection reagent Lipofectamine 3000/siPLK1 (≈27.8%). In comparison, only a small amount of apoptosis was observed in the control group with free siPLK1, PCB50/nonsense siRNA and PBS.
4. Conclusions
In summary, with the gradual broadening of siRNA medical application, a lot of research has been conducted on the safe siRNA delivery materials. The safety issue is essential for clinical application. Here, we showed that PCB had good siRNA loading ability in low pH environment. Compared with PEI with the similar molecular weight, PCB had comparable siRNA loading ability and lower cytotoxicity. In addition, at the cellular level, it has also been shown that PCB can be used as a simple carrier to load siRNA into the cell in a slightly acidic environment, and can effectively escape from endosomal/lysosomal into the cytoplasm, preventing the loss of siRNA before gene silencing is achieved. Compared with PEI and Lipofectamine 3000, PCB with siPLK1 also has significant and excellent potential in inducing apoptosis of HeLa cells. Therefore, the pH-sensitive PCB with lower cytotoxicity could be used as a non-viral vector for safe siRNA delivery, and it could be guessed that it has further research significance for cancer treatment.
Conflicts of interest
There are no conflicts to declare.
Acknowledgements
This work was financially supported by the Beijing Natural Science Foundation (L172046, 2192057), Beijing Nova Program (Z201100006820140), the National High Technology Research and Development Program (2016YFA0200303), the National Natural Science Foundation of China (31771095, 21875254 and 21905283).
Notes and references
- N. J. Caplen and S. Mousses, Short interfering RNA (siRNA)-mediated RNA interference (RNAi) in human cells, Ann. N. Y. Acad. Sci., 2004, 1002, 56–62 Search PubMed.
- A. Reynolds, D. Leake, Q. Boese, S. Scaringe, W. S. Marshall and A. Khvorova, Rational siRNA design for RNA interference, Nat. Biotechnol., 2004, 22, 326–330 Search PubMed.
- Y. Yan, K. Zhou, H. Xiong, J. B. Miller, E. A. Motea, D. A. Boothman and D. J. Siegwart, Aerosol delivery of stabilized polyester-siRNA nanoparticles to silence gene expression in orthotopic lung tumors, Biomaterials, 2016, 118, 84–93 Search PubMed.
- E. Koutsilieri, A. Rethwilm and C. Scheller, The therapeutic potential of siRNA in gene therapy of neurodegenerative disorders, J. Neural Transm., Suppl., 2007, 72, 43–49 Search PubMed.
- M. Creixell and N. A. Peppas, Co-delivery of siRNA and therapeutic agents using nanocarriers to overcome cancer resistance, Nano Today, 2012, 7, 367–379 Search PubMed.
- Y. Higuchi, S. Kawakami and M. Hashida, Strategies for in vivo delivery of siRNAs: recent progress, Biodrugs, 2010, 24, 195–205 Search PubMed.
- K. A. Whitehead, R. Langer and D. G. Anderson, Knocking down barriers: advances in siRNA delivery, Nat. Rev. Drug Discovery, 2009, 8, 129–138 Search PubMed.
- R. Kanasty, J. R. Dorkin, A. Vegas and D. Anderson, Delivery materials for siRNA therapeutics, Nat. Mater., 2013, 12, 967–977 Search PubMed.
- H. Yin, R. L. Kanasty, A. A. Eltoukhy, A. J. Vegas, J. R. Dorkin and D. G. Anderson, Non-viral vectors for gene-based therapy, Nat. Rev. Genet., 2014, 15, 541–555 Search PubMed.
- R. L. Juliano, The delivery of therapeutic oligonucleotides, Nucleic Acids Res., 2016, 44, 6518–6548 Search PubMed.
- Y. Shen, Advances in the development of siRNA-based therapeutics for cancer, IDrugs, 2008, 11, 572–578 Search PubMed.
- S. Khormaee, Y. Choi, M. J. Shen, B. Xu, H. Wu, G. L. Griffiths, R. Chen, N. K. H. Slater and J. K. Park, Endosomolytic Anionic Polymer for the Cytoplasmic Delivery of siRNAs in Localized In Vivo Applications, Adv. Funct. Mater., 2013, 23, 565–574 Search PubMed.
- S. Zhang, B. Zhao, H. Jiang, B. Wang and B. Ma, Cationic lipids and polymers mediated vectors for delivery of siRNA, J. Controlled Release, 2007, 123, 1–10 Search PubMed.
- R. Ghavimi and M. Pourhossein, Review Article: Limitations of Clinical Application of Sirna Delivery Based on Non-Viral Vectors, J. Isfahan Med. Sch., 2014, 32, 34–48 Search PubMed.
- C. Ganas, A. Wei, M. Nazarenus, S. Rösler, T. Kissel, P. Rivera Gil and W. J. Parak, Biodegradable capsules as non-viral vectors for in vitro delivery of PEI/siRNA polyplexes for efficient gene silencing, J. Controlled Release, 2014, 196, 132–138 Search PubMed.
- B. Urban-Klein, S. Werth, S. Abuharbeid, F. Czubayko and A. Aigner, RNAi-mediated gene-targeting through systemic application of polyethylenimine (PEI)-complexed siRNA in vivo, Gene Ther., 2005, 12, 461–466 Search PubMed.
- A. Alshamsan, S. Hamdy, J. Samuel, A. O. S. El-Kadi, A. Lavasanifar and H. Uludag, The induction of tumor apoptosis in B16 melanoma following STAT3 siRNA delivery with a lipid-substituted polyethylenimine, Biomaterials, 2010, 31, 1420–1428 Search PubMed.
- J. L. Shen, H. C. Kim, H. Su, F. Wang, J. Wolfram, D. Kirui, J. Mai, C. Mu, L. Ji, Z. Mao and H. Shen, Cyclodextrin and Polyethylenimine Functionalized Mesoporous Silica
Nanoparticles for Delivery of siRNA Cancer Therapeutics, Theranostics, 2014, 4, 487–497 Search PubMed.
- N. Kim, D. Jiang, A. M. Jacobi, K. A. Lennox, S. D. Rose, M. A. Behlke and A. K. Salem, Synthesis and characterization of mannosylated pegylated polyethylenimine as a carrier for siRNA, Int. J. Pharm., 2012, 427, 123–133 Search PubMed.
- O. Boussif, F. Lezoualch, M. A. Zanta, M. D. Mergny, D. Scherman, B. Demeneix and J. P. Behr, A versatile vector for gene and oligonucleotide transfer into cells in culture and in vivo: polyethylenimine, Proc. Natl. Acad. Sci. U. S. A., 1995, 92, 7297–7301 Search PubMed.
- L. Zhao, Y. Li, D. Pei, Q. Huang, H. Zhang, Z. Yang, F. Li and T. Shi, Glycopolymers/PEI complexes as serum-tolerant vectors for enhanced gene delivery to hepatocytes, Carbohydr. Polym., 2019, 205, 167–175 Search PubMed.
- E. Kharlampieva, V. A. Izumrudov and S. A. Sukhishvili, Electrostatic Layer-by-Layer Self-Assembly of Poly(carboxybetaine)s: Role of Zwitterions in Film Growth, Macromolecules, 2007, 40, 3663–3668 Search PubMed.
- B. Cao, Q. Tang and G. Cheng, Recent advances of zwitterionic carboxybetaine materials and their derivatives, J. Biomater. Sci., Polym. Ed., 2014, 25, 1502–1513 Search PubMed.
- Y. Li, R. Y. Liu, Y. J. Shi, Z. Zhang and X. Zhang, Zwitterionic Poly(carboxybetaine)-based Cationic Liposomes for Effective Delivery of Small Interfering RNA Therapeutics without Accelerated Blood Clearance Phenomenon, Theranostics, 2015, 5, 583–596 Search PubMed.
- Y. Li, R. Liu, J. Yang, G. Ma, Z. Zhang and X. Zhang, Dual sensitive and temporally controlled camptothecin prodrug liposomes codelivery of siRNA for high efficiency tumor therapy, Biomaterials, 2014, 35, 9731–9745 Search PubMed.
- J. Ladd, Z. Zhang, S. Chen, J. C. Hower and S. Jiang, Zwitterionic Polymers Exhibiting High Resistance to Nonspecific Protein Adsorption from Human Serum and Plasma, Biomacromolecules, 2008, 9, 1357–1361 Search PubMed.
- J. Xu, J. Ji, W. Chen and J. Shen, Novel biomimetic polymersomes as polymer therapeutics for drug delivery, J. Controlled Release, 2005, 107, 502–512 Search PubMed.
- Y. Zhao, K. Wee and R. Bai, Highly hydrophilic and low-protein-fouling polypropylene membrane prepared by surface modification with sulfobetaine-based zwitterionic polymer through a combined surface polymerization method, J. Membr. Sci., 2010, 362, 326–333 Search PubMed.
- Y. Li, Q. Cheng, Q. Jiang, Y. Huang, H. Liu, Y. Zhao, W. Cao, G. Ma, F. Dai, X. Liang, Z. Liang and X. Zhang, Enhanced endosomal/lysosomal escape by distearoyl phosphoethanolamine-polycarboxybetaine lipid for systemic delivery of siRNA, J. Controlled Release, 2014, 176, 104–114 Search PubMed.
|
This journal is © The Royal Society of Chemistry 2020 |