DOI:
10.1039/D0RA08254F
(Paper)
RSC Adv., 2020,
10, 43882-43893
The detoxification mechanisms of low-accumulating and non-low-accumulating medicinal plants under Cd and Pb stress
Received
27th September 2020
, Accepted 17th November 2020
First published on 10th December 2020
Abstract
Recently, the levels of heavy metals in medicinal plants have aroused widespread concern because these elements usually enter the food chain through plants and are gradually passed to the final consumers, greatly threatening human health. To reduce heavy metal pollution, it is necessary to solve the problem from the source to ensure environmental quality during medicinal material production. We use low-accumulating and non-low-accumulating medicinal plants to remediate soil contaminated by Cd and Pb. This experiment aims to study the amino acid levels in root exudates, to study antioxidant enzymes and malondialdehyde (MDA) in leaves, and to discuss the detoxification mechanisms of low-accumulating and non-low-accumulating medicinal plants under Cd and Pb stress. In soil contaminated with Cd or Pb, catnip, thyme and Fineleaf Schizonepeta Herb were cultivated. Enrichment factor (EF) and translocation factor (TF) levels were calculated to determine which are low-accumulating medicinal plants with respect to Cd or Pb. The relationships between the amino acid levels in root exudates, the levels of antioxidant enzymes, the present heavy metal species, heavy metal concentrations, and plant species were discussed. Under Pb and Cd stress, the total amounts of amino acids secreted by plant roots and the level of each amino acid were associated with the heavy metal concentrations and plant species. Plants alleviate Pb and Cd stress via adding malondialdehyde (MDA) and antioxidant enzymes. Thyme can be used as a low-accumulating medicinal plant with any concentration of the heavy metal Pb. These results are of great significance for understanding the chemical behaviors of heavy metals at the root/soil interface under Cd and Pb stress and the detoxification mechanisms of medicinal plants.
1 Introduction
At present, heavy metal pollution in soil has attracted worldwide attention. Approximately 19.4% of arable land (2.6 × 107 hm2) in China suffers from heavy metal pollution.1 According to a 2014 National Soil Pollution Survey Bulletin reported by the Ministry of Environmental Protection of China, in 7% of cases, Cd levels in soil exceed the standard, making it the most polluting metal. Cd is known to be extremely toxic, and it can be easily taken up by plant roots and transferred to the aerial parts.2 A well-documented phenomenon is Itai-Itai disease, which arises due to the accumulation of Cd residue in rice.3 A typical heavy metal pollutant, with the strongest toxicity, that exist in many fertilizers is Pb, which is easily absorbed and eventually accumulated in the edible parts of plants. It can easily enter the human body from the food chain and can cause harm to human lives and health. According to Rodriguez et al.,4 due to the slow mobility of Pb, the migration of inorganic Pb and elemental Pb from the soil to underground water goes slowly, and Pb can be readily taken in by various plants. Even though the Pb content in the higher parts of plants (such as fruits, seeds, and leaves) is low, it remains toxic when consumed by humans due to the large accumulation of Pb in the roots.5
In the last quarter of the last century, people became more and more interested in using naturally sourced substances, particularly herbal medicines, for therapeutic purposes. The WHO estimates that herbal medicines are currently used by approximately 4 billion people for certain aspects of primary health care. However, the existence of heavy metals may have a strong impact on the safety, efficacy, and quality of natural products prepared from medicinal plants.6 Incidents of excessive heavy metals in traditional Chinese medicine (TCM) have occurred occasionally, seriously damaging the image of TCM and causing significant economic losses to the TCM industry.7 At present, heavy metal pollution is becoming increasingly serious. To reduce heavy metal pollution, in addition to taking effective control measures, it is also necessary to solve the problem from the source to ensure environmental quality during medicinal material production. Hence, phytoremediation has become more and more important. The phytoremediation of heavy metals is of great significance8–10 and it is considered to be one of the better measures to fix heavy-metal pollution due to its low cost, which can be 1000 times lower than traditional restoration methods such as excavation and reburying.11 A desired phytoremediation model would not only be tolerant to heavy metals, but would also have high efficiency in absorbing heavy metals effectively and show rapid growth, having good economic benefit.12 However, few plants are both hyperaccumulators and rapid-growth plants. Most so-called hyper-accumulators exhibit great potential for heavy metal accumulation, but their accumulation of biomass is very low. On the other hand, some low-accumulators show higher biomass production, even though they have lower uptake capacities than hyperaccumulators. Zhi et al.13 found that Chinese soybean (Tiefeng 29) was a low Pb-accumulator. Research by Manan et al.14 showed that C. asiatica is a low-accumulator of Zn and Pb. Research by Huang et al.15 indicated that Chinese cabbage (No. 12, No. 21) and cabbage (No. 6, No. 7) are low Cd-accumulating vegetables.
The “rhizosphere” is generally defined as an area of soil around the roots where microbes are highly active and affected by the secretion of a microbial community by the roots.16 Root exudates usually include amino acids, sugars, organic acids, high molecular weight compounds, and phenolic compounds.17,18 Low molecular weight compound (amino acids, sugars, organic acids, phenols) and high molecular weight compound (polysaccharides and proteins) root exudates play critical roles in rhizospheric processes.19 In addition, the root exudates of wheat and rice also showed a certain degree of toxicity under Pb and Cd stress, unlike plants that were not treated with Pb and/or Cd.20 Salt et al.21 found that citrate- and Ni-chelated histidine accumulated in plant root exudates that were not hyperaccumulating; therefore, they can assist in Ni detoxification strategies by helping to reduce the uptake of Ni. Therefore, root exudates have an influence on the distribution and absorption of Pb and Cd in plants.22
Reactive oxygen species (ROS) accumulate and damage cell membranes under heavy-metal stress, leading to increased membrane lipid peroxidation products. The malondialdehyde (MDA) content is a lipid peroxidation indicator, representing the extent of membrane lipid peroxidation and the intensity of a plant's reaction to stress conditions.23 To resist the negative effects of ROS accumulation and improve the survival rates of plants under these stresses, plants have to modulate the expression of related genes in complex antioxidant enzyme systems. Peroxidase (POD), superoxide dismutase (SOD), and catalase (CAT) are the main enzymes for scavenging ROS in plants.24 SOD is the first line of defense for plants when it comes to removing ROS, and it takes a core position in protecting enzyme systems. Its main function is to remove O2−, which can disproportionate O2− to generate H2O2. The main function of CAT and POD is to remove H2O2 in organisms.25
We use low-accumulating medicinal plants to remediate soil contaminated by Cd and Pb. A plant with low accumulation is one that can show reduced element absorption when the element concentration in the matrix is high or the net excretion of the element is high. Even though the concentration is high in the matrix, the element concentration in such plant tissue is still very low.26 The cultivation process of low-accumulating plants is simple and poses no ecological risk, and the selected crops can be directly promoted in local communities, so it is an ascendant approach to use low-accumulating plants to remediate polluted soil.
The purpose of this paper is to study the detoxification mechanisms of low- and non-low-accumulating plants under Cd and Pb stress. Via analyzing the amino acid content values of root exudates and the enzyme content values of leaves, the mechanisms of repair of low-accumulating plants and non-low-accumulating plants can be further analyzed.
2 Methods
2.1 The cultivation of medicinal plants
Soil samples were obtained from depths of 0–20 cm from the surface, passed through a 4 mm sieve, and then mixed with suitable amounts of CdCl2·2.5H2O or Pb(NO3)2 solution. Plastic flowerpots (20 cm diameter × 15 cm depth) were used, containing 2.5 kg of soil in each case. Five treatments were adopted: 1 CK treatment (control, no Cd or Pb); 2 Cd treatments, i.e., T1 (1.0 mg Cd kg−1 soil) and T2 (2.5 mg Cd kg−1 soil); and 2 Pb treatments, i.e., T1 (500 mg Pb kg−1 soil) and T2 (1500 mg Pb kg−1 soil). T1 and T2 indicate low and medium contamination levels. The heavy metal pollution classification standard and single pollution index methods were used to assess the soil heavy-metal pollution levels.27,28 500 g of each soil sample was accurately weighed and placed in a 300-mesh Nylon rhizosphere bag with a diameter of 15 cm. A rhizosphere bag was placed in each plastic flowerpot and the soil served as the plant rhizosphere soil.29 The soil was watered and the pots were then kept under constant conditions for a month, which is a sufficient time for various adsorption mechanisms in the soil to obtain a natural balance.
The catnip (Nepeta cataria), thyme (Thymus spp), and Fineleaf Schizonepeta Herb (Nepeta cataria L.) seeds used in this study were all obtained from a seed company. After sterilizing with 2% (v/v) hydrogen peroxide for 10 minutes, the seeds were washed with distilled water several times and sowed in each pot. No chemical fertilizers were used in these pots. During the experiments, soil moisture loss was compensated for to maintain a soil moisture content of 75–80%.
2.2 Determination of heavy metals in soil and plant samples
After harvesting, the plants were rinsed entirely with tap water and deionized water 3 times, and they were then divided into shoot and root groups. After drying at 105 °C for 5 minutes, the samples were completely dried in an oven at 70 °C, and then weighed and ground into powder. 0.50 g of soil sample and 0.50 g of plant sample were digested (v/v) in 12 ml of solution containing 13% concentrated HClO4 and 87% concentrated HNO3.30 ICP-AES (Spectro Arcos, Germany) was used to determine the Cd and Pb levels.29 The recoveries of these two elements were between 94% and 99%.
2.3 Determination of amino acids in root exudates
Approximately 2 g of rhizosphere soil was weighed and placed in a 10 ml centrifuge tube. To inhibit microbial activity, 4 ml of 0.1% H3PO4 solution was added to the root exudate components. In order to realize apparent equilibrium desorption, the tube was first shaken using a rotating shaker at a speed of 200 rpm in the dark, then the tube was centrifuged at 5000 rpm for 5 minutes to remove all microorganisms, and then it was centrifuged with a syringe filter (0.45 μm). 0.25 g of soil sample was accurately weighed, divided into three portions, and placed in a 100 ml hydrolysis bottle; 20 mL of 6 mol L−1 hydrochloric acid was then added, followed by hydrolysis at 105 °C for 12 h. The amino acids were determined via liquid chromatography (Thermo 3000).
2.4 Determination of leaf enzymes
Guaiacol oxidation was used to measure POD activities.31 The total SOD activity was measured using nitro-blue tetrazolium.32 The total CAT activity was determined via spectrophotometry.33 MDA was determined using the thiobarbituric acid method.34
2.5 Statistical analysis
All treatments were repeated three times and each sample was assayed three times in parallel. Excel 2018 and SPSS 18.0 were used to analyze data. Significant differences between means (P < 0.05) were tested using the least squares (LSD) method. All results are expressed as dry weights.
3 Results
3.1 Effects of Cd or Pb stress on medicinal plants
3.1.1 Effects on heights of medical plants.
3.1.1.1 Effects of Cd stress on plant heights.
Under low-concentration Cd stress, the plant heights of catnip, thyme, and Fineleaf Schizonepeta Herb were 1.46 times, 1.35 times, and 2.86 times those of the control groups, respectively (Fig. 1). Under medium-concentration Cd stress, the plant heights of catnip, thyme, and Fineleaf Schizonepeta Herb were 1.06 times, 1.18 times, and 1.86 times higher than those of the control groups, respectively. In general, the plant heights of the control groups were lower than those of the catnip, thyme, and Fineleaf Schizonepeta Herb groups under Cd stress, and the plant heights of the three plants reached maximum levels under low Cd stress. This shows that Cd stress can promote the growth of catnip, thyme, and Fineleaf Schizonepeta Herb.
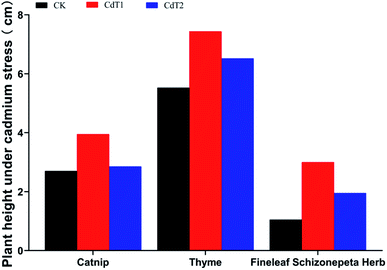 |
| Fig. 1 Plant heights under different levels of cadmium stress. | |
3.1.1.2 Effects of Pb stress on plant heights.
Under low-concentration Pb stress, the plant heights of catnip, thyme, and Fineleaf Schizonepeta Herb were 0.79 times, 0.58 times, and 1.35 times higher than those of the control groups (Fig. 2). Under high-concentration Pb stress, the plant heights of catnip, thyme and Fineleaf Schizonepeta Herb were 0.93 times, 0.52 times, and 0.81 times those of the control groups, respectively. The plant height changes of the three plants under Pb stress were different. Under Pb stress, the plant heights of the control groups were higher in the cases of catnip and thyme, and the changes to the thyme heights were more obvious. The plant heights of Fineleaf Schizonepeta Herb showed a trend of increasing first and then decreasing as Pb stress increased. This indicates that Pb stress can inhibit the growth of catnip and thyme, while it promotes the growth of Fineleaf Schizonepeta Herb at low concentrations and inhibits it at high concentrations.
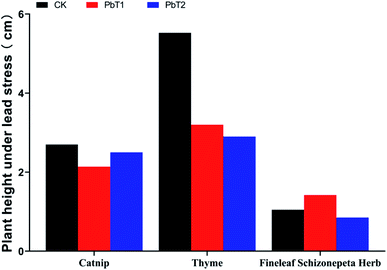 |
| Fig. 2 Plant heights under different levels of lead stress. | |
3.1.2 Effects on the dry weights of medical plants.
3.1.2.1 Effects of Cd stress on dry weights.
The dry shoot and root weights of catnip, thyme, and Fineleaf Schizonepeta Herb were affected by different concentrations of Cd (Fig. 3). In general, the dry weights of the roots and shoots of catnip, thyme, and Fineleaf Schizonepeta Herb showed a parabolic change as the concentration of Cd was increased. It can be seen that catnip, thyme, and Fineleaf Schizonepeta Herb are resistant to low Cd stress but weak under high-concentration Cd stress. In addition, under low Cd stress, the dry weights of the shoots and roots of the three plants were much higher compared with the control groups. It is speculated the growth of catnip, thyme and Fineleaf Schizonepeta Herb will be promoted under a certain concentration of Cd stress.
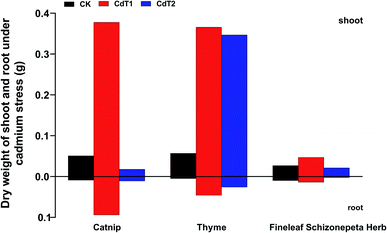 |
| Fig. 3 Dry weights of shoots and roots under cadmium stress. | |
3.1.2.2 Effects of Pb stress on dry weights.
Under low-concentration Pb stress, the catnip shoot and root dry weights have a tendency to decrease (Fig. 4), while under medium-concentration Pb stress, the catnip shoot and root dry weights were increased significantly, much higher than the control group. Under Pb stress, the shoot and root dry weights of Fineleaf Schizonepeta Herb decreased.
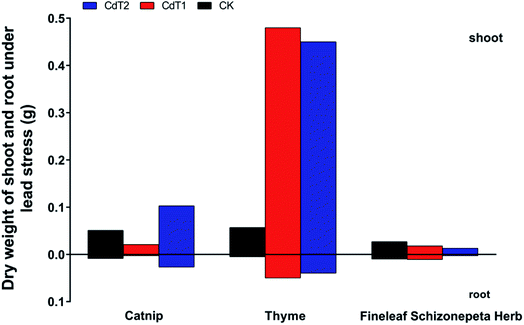 |
| Fig. 4 Dry weights of shoots and roots under lead stress. | |
3.1.3 The identification of medicinal plants with low heavy metal accumulation.
The heavy metal levels in the shoots and roots of catnip, thyme, and Fineleaf Schizonepeta Herb are shown in Table 1.
Table 1 Pb and Cd levels in roots and shoots of catnip, thyme, and Fineleaf Schizonepeta Herb
|
Catnip |
Thyme |
Fineleaf Schizonepeta Herb |
Shoots |
Roots |
Shoots |
Roots |
Shoots |
Roots |
CdT1 |
73.37% |
26.63% |
32.81% |
67.19% |
86.03% |
13.97% |
CdT2 |
54.17% |
45.83% |
42.99% |
57.01% |
82.00% |
18.00% |
PbT1 |
28.83% |
71.17% |
7.88% |
92.12% |
39.78% |
60.22% |
PbT2 |
26.16% |
73.84% |
1.31% |
98.69% |
38.91% |
61.09% |
Using translocation factor (TF) and enrichment factor (EF) values to identify low-accumulating medicinal plants,35 the following statements can be made (Table 2):
Table 2 Enrichment and translocation factors for the three medicinal plants
|
EF |
TF |
CdT1 |
CdT2 |
PbT1 |
PbT2 |
CdT1 |
CdT2 |
PbT1 |
PbT2 |
Catnip |
1.0 |
0.18 |
0.01 |
0.37 |
2.76 |
1.18 |
0.41 |
0.35 |
Thyme |
0.40 |
0.16 |
0.00 |
0.00 |
0.49 |
0.75 |
0.09 |
0.01 |
Fineleaf Schizonepeta herb |
1.94 |
1.22 |
0.01 |
0.01 |
6.16 |
4.56 |
0.66 |
0.64 |
(1) The heavy metal levels in the aboveground parts are lower than the maximum allowable doses for medicinal plants when the Cd content is ≤ 0.3 mg kg−1 and the Pb content is ≤ 5.0 mg kg−1 (referring to Chinese Pharmacopoeia, 2010 edition);
(2) when the translocation factor (TF) is <1.0, a plant is low accumulating:
where Cshoot is the concentration of Cd or Pb in the aerial parts of the medicinal plant and Croot is the concentration of Cd or Pb in the medicinal plant root;
(3) when the enrichment factor (EF) is <1.0, a plant is low accumulating:
where Cshoot is the Cd or Pb concentration in the overground parts of the medicinal plant and Csoil denotes the total Cd or Pb concentration in the corresponding soil sample; and
(4) In the case of high heavy metal tolerance, the biomass of soil polluted by heavy metals does not decrease remarkably.
In a low-concentration Pb environment, the Pb levels in the aerial parts of catnip and Fineleaf Schizonepeta Herb were less than 5.0 mg kg−1 and 2.68 mg kg−1, respectively. The enrichment factors of catnip and Fineleaf Schizonepeta Herb are in line with the standards of low-accumulating medicinal plants, being 0.01 and 0.01, respectively. Their translocation factors are 0.35 and 0.64, respectively. In a medium-concentration Pb environment, the Pb content of thyme above ground is 2.39 mg kg−1, and the enrichment factor and translocation factor values are 0.00 and 0.01, respectively. In a low-concentration Pb environment, the Pb content above ground is 2.18 mg kg−1, the enrichment factor is 0.00, and the transport factor is 0.09. It can be seen that thyme is a low-accumulating plant with respect to Pb.
3.1.4 Effects on amino acids in root exudates.
3.1.4.1 Effects of heavy-metal stress on the total amino acid content.
Under Cd stress of 1.0 mg kg−1 and 2.5 mg kg−1, the total amino acid levels secreted by catnip roots were 1.08 times and 1.01 times more than the control groups (Fig. 5), the total amino acids levels secreted by thyme roots were 1.02 times and 1.09 times higher than those of the control groups, and the total amino acid levels secreted by Fineleaf Schizonepeta Herb roots were 1.34 times and 1.13 times higher than those of the control groups.
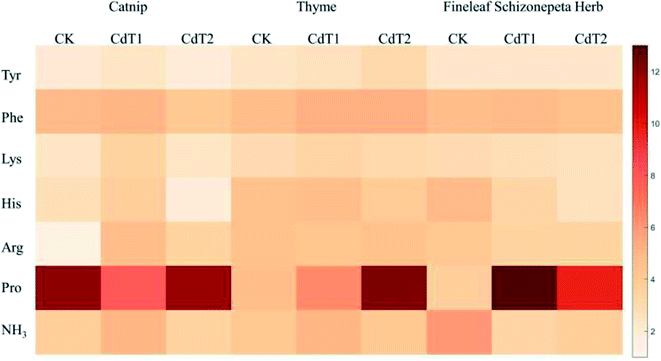 |
| Fig. 5 Amino acid levels in root exudates of medicinal plants under Cd stress. | |
Under 500 mg kg−1 and 1500 mg kg−1 Pb stress, the total amino acid levels secreted by catnip roots were 1.22 times and 1.00 times more than the control groups (Fig. 6), the total amino acid levels secreted by thyme roots were 1.02 times and 1.09 times more than the control groups, and the total amino acid levels secreted by Fineleaf Schizonepeta Herb roots were 1.21 times and 0.91 times those of the control groups.
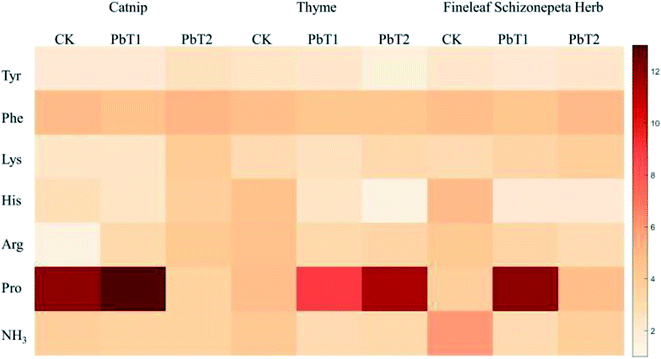 |
| Fig. 6 Amino acid levels in root exudates of medicinal plants under Pb stress. | |
3.1.4.2 Effects of heavy-metal stress on the types of amino acids.
Under Cd stress, the roots of catnip, thyme, and Fineleaf Schizonepeta Herb secrete six main amino acids (Fig. 5): Tyr, Phe, Lys, Pro, His, and Arg. Under both medium- and low-concentration Cd stress, the amount of proline secreted by the root systems is the highest, followed by phenylalanine, arginine, histidine, lysine, and tyrosine.
Under Pb stress, the roots of catnip, thyme, and Fineleaf Schizonepeta Herb secrete six main amino acids (Fig. 6): Tyr, Phe, Lys, Pro, His, and Arg. As shown in the figure, under any concentration of Pb stress, the roots produce proline in the highest amount, followed by phenylalanine, arginine, lysine, histidine, and tyrosine.
3.1.4.3 Effects on Pro under heavy-metal stress.
Changes in the proline content are often regarded as an important indicator of whether the amino acid metabolism of a plant is being impaired. When plants are exposed to unfavorable conditions, such as high temperature, low temperature, drought, salting, and air pollution, the free proline content in plant tissue increases significantly, which can give rise to a remarkable increase in the amino-acid levels secreted by the root system. This is because the molecular structure and physical and chemical properties of proline can protect the spatial structures of enzymes, stabilize the membrane system, participate in the synthesis of chlorophyll, eliminate NH3 toxicity, and reduce the acidity of cells. A rise in the plant proline content is a critical adaptive mechanism for the remediation of heavy metal stress.36 According to a report by Islam et al.,37 under heavy-metal stress, in tobacco plants, the integrity of cellular membranes was restored by proline, and enzymes in the AsA–GSH cycle were elevated due to proline. This indicates an efficient antioxidative defense system, which can defend plants against stress via improving the antioxidant activities of non-enzymes and enzymes. Through eliminating ROS toxins, increasing the levels of GSH and ASA, and increasing the activities of GR, APX, POX, CAT, and SOD, this mechanism decreases the toxicity of heavy metals. These tasks all reduce the transcription level and/or translation and endogenous proline levels.38 Furthermore, proline reacts with Cd2+ in plants to form the non-toxic compound Cd2+-proline.39 In addition, it supplies plants with energy needed to grow and survive, improves their tolerance to stress, defends membranes in plants against ion-leakage caused by Cd2+ and degradation,40 and improves the water potential of leaf tissue through protecting cellular membranes from heavy metal oxidation.41
Under low concentration Pb stress, the amount of proline secreted by catnip roots was 1.10 times higher than that of the control group, while the amount of proline was 0.28 times higher than that of the control group with medium-concentration Pb stress (Fig. 6). Under low-concentration Pb stress, the amount of proline secreted by the root of Fineleaf Schizonepeta Herb reached its maximum value, which was 3.29 times higher than that of the control group, and then decreased. Under the stress of low-concentration Cd, the amount of proline the root of Fineleaf Schizonepeta Herb secreted reached the maximum, which was 3.61 times higher than that of the control group. Under the stress of low-concentration Pb, the amount of proline secreted by the thyme root system was 1.91 times higher compared with the control group, while it was 2.41 times higher compared with the group under medium-concentration Pb stress. Under low-concentration Cd stress, the amount was 1.33 times higher compared with the control group, and it was 2.57 times higher compared with the group under medium-concentration Cd stress. In other words, under the heavy metal stress of Cd and Pb, as the concentration of Cd and Pb increases, the proline amount secreted by the root system also increases. Except for catnip under low-concentration Cd and medium-concentration Pb stress, compared with the control group, the proline secretion amount was reduced. In general, it was higher than the control group in other cases. Moreover, the secretion of proline is the largest compared with the secretion of other amino acids. This shows that proline makes a valuable contribution towards the heavy metal stress resistance mechanism of catnip, thyme, and Fineleaf Schizonepeta Herb, and the amount of proline secreted by the root of thyme correlates well with the heavy metal concentration.
3.1.4.4 Catnip amino acid secretion under heavy-metal stress.
At different concentrations of Pb and Cd, catnip roots secrete high levels of lysine and arginine in addition to proline. Under low-concentration Pb stress, the amount of lysine secreted by the root system was 1.05 times higher than that of the control group. Under medium-concentration Pb stress, the amount of lysine secreted is 1.62 times that of the control. When catnip is subjected to different concentrations of Cd stress, catnip roots secrete more arginine, lysine, and histidine. The secreted amounts of the three amino acid reach maximum values and then decrease. Among them, the amount of arginine secreted was 3.03 times that of the control, which means that the level of arginine secreted by the root system increased significantly under low-concentration Cd stress. Catnip secretes more lysine and arginine at different Pb and Cd stress concentrations, which may be one of the important mechanisms explaining how catnip resists Pb and Cd stress.
3.1.4.5 Thyme amino acid secretion under heavy-metal stress.
At different levels of Pb stress, the histidine levels secreted by thyme decreased sharply. The secretion levels of histidine under low-concentration and medium-concentration Pb stress were 0.54 and 0.33 times that of the control group, respectively. The sharp reduction in histidine may be one of the important mechanisms explaining thyme's resistance to Pb stress. At different levels of Cd stress, its root system will secrete more tyrosine in addition to more proline. Under low-concentration Cd stress, the amount of tyrosine secreted by the root system was 1.09 times higher compared with the control group, and the secreted amount was 1.30 times that of the control group under high-concentration Cd stress.
3.1.4.6 Fineleaf Schizonepeta Herb amino acid secretion under heavy-metal stress.
Under different levels of Pb and Cd stress, Fineleaf Schizonepeta Herb secretes more lysine in addition to more proline. Under low-concentration Pb stress, the lysine secretion was 1.09 times higher compared with the control group, while it was 1.20 times higher compared with the control group under-high concentration Pb stress. Fineleaf Schizonepeta Herb secretes more lysine at different levels of Pb stress, which may be one of the important mechanisms explaining its resistance to Pb stress.
3.1.5 Effects on NH3 content.
The NH3 content is related to the secretion of proline. The molecular structure and physical and chemical properties of proline can protect the spatial structure of enzymes, stabilize the membrane system, participate in the synthesis of chlorophyll, eliminate NH3 toxicity, and reduce the acidity of cells. Under low-concentration Cd stress, the NH3 content of Fineleaf Schizonepeta Herb was 0.58 times that of the control group, while it was 0.62 times that of the control group under medium-concentration Cd stress. Under low-concentration Pb stress, the NH3 content secreted by Fineleaf Schizonepeta Herb was 0.51 times that of the control group, while it was 0.63 times that of the control group under medium-concentration Pb stress. When the content of proline secreted by Fineleaf Schizonepeta Herb increased, the NH3 content decreased significantly.
3.1.6 Effects of enzymes on the stress resistance of plants.
3.1.6.1 Effects on enzymes under Cd stress.
On the 10th day under Cd stress, the MDA content levels in the leaves of the three plants increased as the Cd concentration increased, and the MDA content levels in the three plants at different stress concentrations were almost the same (Fig. 7). The CAT content in the leaves of the three plants and the SOD content in the leaves of the three plants showed increasing trends with an increase in the Cd concentration. Among the studied plants, the SOD secretion by thyme and Fineleaf Schizonepeta Herb under low-concentration stress and under medium-concentration stress were very alike. The POD content levels in the leaves of the three plants presented an upward trend as the Cd concentration increased. POD secretion under low-concentration stress increased significantly compared with the control. POD secretion under medium-concentration stress was comparable with that under low-concentration stress. There is little difference in the levels. In addition, the POD content levels in the three plants are almost the same at each stress concentration.
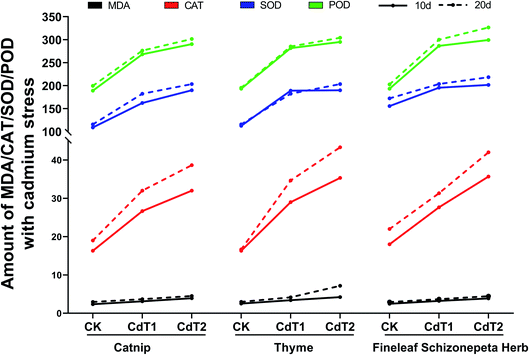 |
| Fig. 7 MDA, CAT, SOD, and POD amounts under different levels of cadmium stress. | |
On the 20th day under Cd stress, the MDA content levels in the leaves of the three plants went up as the Cd concentration increased; the MDA content of thyme increased significantly when the Cd concentration was at a medium level, and it was much higher than in the other two plants. The CAT content levels in the leaves of the three plants presented an upward trend. As the Cd concentration increased, the SOD content levels in the leaves of the three plants also presented an upward trend. Under stress at each concentration, SOD secretion by Fineleaf Schizonepeta Herb was higher than by the other two plants. The POD content levels in the leaves of the three plants showed an increasing trend along with increasing Cd concentration, and the amounts of POD secreted by the three plants under stress at each concentration were almost the same.
3.1.6.2 Effects on enzymes under Pb stress.
On the 10th day under Pb stress, the MDA content levels in the leaves of the three plants went up along with increasing Pb concentration, and the content levels of MDA in the three plants at each concentration of Pb stress were almost the same (Fig. 8). As the Pb concentration increased, the CAT levels in the three plants changed in different ways. CAT secretion by catnip and thyme increased as the Pb concentration increased, while CAT secretion by Fineleaf Schizonepeta Herb first decreased and then increased. SOD secretion by the three plants increased along with increasing Pb concentration, and the SOD secretion levels of Fineleaf Schizonepeta Herb under various Pb concentrations were much higher than the other two plants. The POD secretion levels of the three plants all increased as the Pb concentration increased, and POD secretion by Fineleaf Schizonepeta Herb at various Pb concentrations was slightly higher than those of the other two plants.
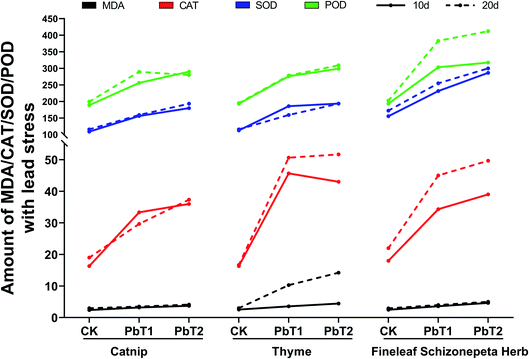 |
| Fig. 8 MDA, CAT, SOD, and POD amounts under different levels of lead stress. | |
On the 20th day under Pb stress, the MDA content levels in the leaves of catnip and Fineleaf Schizonepeta Herb went up along with increasing Pb concentration, while the MDA content decreased and then increased for thyme. The MDA secretion by thyme was much higher than those of the other two plants. As the Pb concentration increased, CAT secretion levels increased for all plants, except thyme (which first decreased and then increased), and the CAT secretion levels of thyme were much higher than those of catnip and Fineleaf Schizonepeta Herb. With an increase in Pb concentration, the SOD secretion levels of all three plants increased, and SOD secretion by Fineleaf Schizonepeta Herb was much higher than the other two plants. The secretion of POD by the three plants showed different trends as the Pb concentration increased. The secretion of POD by catnip first increased and then decreased, POD secretion by thyme increased, and POD secretion by Fineleaf Schizonepeta Herb first decreased and then increased. POD secretion by Fineleaf Schizonepeta Herb is higher than the other two plants.
In this study, the MDA content levels in the leaves of the three plants increased slightly with increases in Pb and Cd concentration, but no significant differences were found compared with the control group.
Our experiments showed that as the Pb and Cd concentrations increased, the levels of POD, CAT, and SOD in the leaves of the three plants generally increased compared with the control groups over the two time periods. Experiments by Hao et al.25 showed that Cu, Mg, and Fe can increase the MDA levels over 3 time periods, and they can increase the SOD and POD activities.
4 Discussion
4.1 Differences in plant heights and dry weights under heavy-metal stress
Under low-concentration Cd stress, the shoot and root dry weights of the three plants were much higher compared with the control groups, indicating that certain concentrations of Cd stress may promote the growth of catnip, thyme, and Fineleaf Schizonepeta Herb.
Under low-concentration Pb stress, the shoot and root dry weights of catnip tended to decrease. Thyme is a low-accumulating plant with respect to Pb. It can be clearly found that at different concentrations of Pb stress, the plant heights and dry weights of thyme are higher than those of the other two medical plants.
According to a study by Hammami et al.42 on the phytoremediation abilities of weeds (S. nigrum, Portulaca oleracea, Taraxacum officinale, and Abutilon theophrasti) in Cd-contaminated soil, the fresh weights and dry weights of the shoots and roots of each plant decreased as the Cd level in the soil increased. Manan et al.35 found that O. stamineus appeared to grow well in contaminated soil and did not seem to be any different visually from plants grown in controlled soil. O. stamineus roots in contaminated soil were 30% longer than the control group, which indicated that contaminated soil treatment did not have any adverse effects on O. stamineus root elongation. This shows the species-specific adaptation of different plants towards heavy-metal stress.
4.2 Changes in root amino acids
4.2.1 Total amounts of amino acids secreted by plant roots.
The current results show that under the stress at any concentration of Pb, the roots of catnip, thyme, and Fineleaf Schizonepeta Herb produce proline in the highest amounts, followed by phenylalanine, arginine, lysine, histidine, and tyrosine. Under stress at different Cd concentrations, the amounts of proline secreted by the root systems are still the highest, followed by phenylalanine, arginine, histidine, lysine, and tyrosine.
The trends of total amino acid secretion shown by catnip and Fineleaf Schizonepeta Herb are consistent. When the heavy-metal concentration is low, the amounts of amino acid secreted by the roots are high, while the amounts of amino acid secreted at higher heavy-metal concentrations are low. The reason may be that some of the proteins in the plants decompose when the heavy-metal stress concentration is low; however, when the heavy-metal stress concentration is higher, the plant's own anti-stress mechanisms play a role, synthesizing a large number of enzymes to resist adverse stress. The trend of amino acid secretion by thyme is that as the concentration of heavy metal increases, the total amount of amino acids secreted by the root system also increases; this is probably because at higher concentrations of Pb stress, the plasma membranes of thyme root cells are destroyed and root-cell amino acids are released. Past research has centered on the influence of amino acids on the abilities of plants to tolerate Cd. Experiments by Tang examining how Cd stress changes the amino acids secreted by different rice roots showed that a low concentration of Cd can promote the total amino acid secretion and a high concentration of Cd can inhibit amino acid secretion. Moreover, Cd stress had almost no effect on the types of amino acids secreted by the root system, but it had a great influence on the amounts of secreted amino acids;43 this is consistent with the conclusions of these experiments. Experiments by Liao et al.44 showed that the total amount of amino acids in sugarcane decreased under Cd treatment at low concentrations, but it increased as the Cd concentration was increased.
4.2.2 Types of amino acids secreted by plant roots under Pb stress.
Under Pb stress, the amino acid content levels of catnip changed, as the amounts of Tyr, Lys, and Arg increased. While the levels of Phe and His decreased first and then increased, the amount of Pro showed an opposite trend. In thyme, the amounts of Phe, Lys, and Arg decreased at first and then increased, Tyr and His decreased as the Pb concentration increased, and the amount of Pro increased with an increase in the Pb concentration. In Fineleaf Schizonepeta Herb, the amounts of Tyr and Phe decreased first and then increased. As the concentration of Pb increased, the levels of His and Arg decreased, but the amount of Lys increased. The amount of Pro showed a trend of increasing first and then decreasing. The amino acids secreted by rice roots include Phe, Arg, His, and Tyr. What is different in our experiments is that there is no secretion of Lys at various levels of Cd stress.
4.2.3 Types of amino acids secreted by plant roots under Cd stress.
The amino acid levels of catnip changed under Cd stress; the amounts of Tyr, Phe, Lys, His, and Arg increased at first and then decreased, and the Pro level decreased first and then increased. In thyme, the amounts of Phe, Lys, and His showed a trend of increasing first and then decreasing, the amounts of Tyr and Pro increased, and the level of Arg first decreased and then increased. In Fineleaf Schizonepeta Herb, the levels of Tyr, Phe, and Pro showed a trend of increasing first and then decreasing. The amounts of Lys, His, and Arg decreased as the Cd concentration increased. Experiments by Liao et al.44 found that amino acids such as Arg, Lys, Phe, His, Lie, Met, and Cys accumulate in sugarcane tissue under Cd stress. Among these, the levels of Arg and Lys increased as the concentration of Cd treatment increased, while the amounts of Tyr and His decreased under Cd25 treatment, increased under Cd50 treatment, and increased significantly under Cd100 treatment. The levels of Phe under Cd25 and Cd50 stress decreased compared with the control group and increased under Cd100 stress. As the Cd stress concentration increased, the amount of Pro decreased, and while it increased under Cd100 stress, it was still lower than that of the control group. Experiments by Tang et al.43 showed that the amounts of various amino acids secreted by rice root systems presented a trend of first increasing and then decreasing as the Cd concentration increased. Research by Zoghlami et al.45 showed that in tomato roots that were exposed to Cd, asparagine, glutamine and branched-chain amino acids (tryptophane, isoleucine, valine, and phenylalanine) accumulated significantly. Zemanová46 found that in the process of two Noccaea metallophyte species reacting to Cd stress, phenylalanine, threonine, tryptophan, and ornithine levels increased, while alanine and glycine levels decreased.
4.2.4 Changes in the amounts of proline secreted by plant roots under Cd stress.
The most common adaptive response when plants are exposed to a variety of metal ions, such as Al3+, Zn2+, Cd2+, and Cu2+, is the accumulation of proline.47 In our experiments, Pro was the amino acid that the three medical plant roots secreted in the highest amounts. It played a crucial role in the mechanism of catnip, thyme, and Fineleaf Schizonepeta Herb resistance to heavy metal stress, and the secretion levels could be correlated to the concentration of heavy metal. Except for the case of catnip under low-concentration Cd stress, where proline secretion was slightly reduced compared with the control, proline secretion in all other cases was higher than the control. Records have shown that the accumulation of Cd leads to proline accumulation in wheat, barley, and mung.48–50 However, Costa and Morel51 found that Cd did not induce proline accumulation in lettuce, but it induced particular increases in the levels of lysine, asparagine, and methionine. Experiments by Yin52 showed that the free proline content in coreopsis leaves is lower than that of a control, showing a general downward trend. The amount of proline in the roots of Gaillardia increases as the Pb concentration increases.
In addition, the increase in Pro levels proved a relationship between antioxidant enzymes and proline. Under heavy-metal stress, proline restored the cellular membrane integrity and increased the enzyme levels in the AsA–GSH cycle. This also shows that catnip, thyme, and Fineleaf Schizonepeta Herb have good tolerance to Pb and Cd.
Based on the existing literature, genotypic differences, differences in plant species and heavy-metal treatments, and even different parts of the plant result in different effects on amino-acid metabolisms. Generally speaking, these results suggest that amino acid accumulation is beneficial for Pb and Cd stress resistance.
4.2.5 Differences in amino acid levels between thyme and the other two medical plants.
It can be clearly found that the amounts of Arg, His, Lys, Phe, and Tyr secreted by the roots of thyme are lower than those of the other two plants under Pb stress. However, the levels of Pro are much higher than the other two plants. This reflects the important role of Pro in phytoremediation at the same time.
4.3 Changes in leaf enzyme levels under Cd stress
4.3.1 Changes in MDA secreted by plant leaves.
In this experiment, as the concentration of Cd was increased, the MDA levels in the leaves of the three plants increased, but compared with the control group, no remarkable differences were found. This may be because the increase in antioxidant enzyme activity partially eliminated the accumulation of H2O2 and ·O2−, making the increase in MDA content insignificant. If the Cd concentration is further increased, the MDA content may increase significantly.
4.3.2 Changes in SOD secreted by plant leaves.
SOD is the first line of defense for plants when it comes to eliminating ROS and it is of great importance in protective enzyme systems. Its main function is to separate O2 and to disproportionate O2− to generate H2O2. In this experiment, SOD levels showed a tendency to increase when the concentration of exogenous Cd in the soil was increased, which indicated that the induced expression of heavy metal Cd was higher and the ability to remove superoxide anions was enhanced.
4.3.3 Changes in CAT and POD secreted by plant leaves.
The main function of CAT and POD is to remove H2O2 in organisms but, because of their low affinity for substrates, the efficiency of H2O2 removal by CAT is not as efficient as by POD. In these experiments, it is obvious that the secretion of POD was much higher. In terms of CAT and POD secretion, as the Cd concentration increased, the secretion of both increased gradually, reflecting the stress response of plants to adversity.
4.4 Changes in leaf enzyme levels under Pb stress
On the 10th day of Pb stress, the levels of MDA, CAT, SOD, and POD in the three plants all increased, and the CAT level of the low-accumulation plant thyme was much higher than the other two plants. On the 20th day of Pb stress, the levels of MDA, CAT, SOD, and POD of the three plants were still generally high compared with the control group, and the levels of MDA and CAT in the low-accumulating plant thyme were much higher than the other two plants. It is inferred from this that MDA and CAT play decisive roles in the anti-stress response of thyme.
Experiments by Michalak et al.53 found that the MDA and SOD levels of cyanobacteria increased under heavy-metal stress. Zhang et al.54 found that compared with a control group, the activities of POD and SOD in plant leaves under heavy metal stress exhibited a fluctuating trend, while the activity of CAT increased along with the level of stress in K. candel but stayed the same in B. gymnorrhiza leaves. Studies by Choudhary et al.55 showed that the levels of MDA and SOD in cyanobacterium Spirulina platensis-S5 increased under heavy-metal stress. Hao et al.26 found that Cu, Mg, and Fe can increase MDA levels over 3 time periods, also increasing the activities of SOD and POD. Experiments by Radić et al.47 showed that antioxidant responses were observed under Zn and Al stress. Both SOD and POD levels increased significantly. This is similar to our experiments. In addition, the secretion levels of the three enzymes were higher under Pb stress than under Cd stress, indicating that the effects of Pb stress on the plants were stronger than those of Cd stress.
5 Conclusions
Under Pb and Cd stress, the total levels of amino acids secreted by plant roots and the levels of each amino acid were studied and associated with heavy metal concentrations and plant species. Pb and Cd stress has little effect on the types of amino acids secreted by the root systems, but it has a greater impact on the amounts of the various types of amino acids that are secreted. Proline is essential in the resistance of plants to heavy metal stress. Plants alleviate Pb and Cd stress via increasing MDA and antioxidant enzyme (CAT, POD, and SOD) levels. Thyme is a low-accumulating plant with respect to Pb. These results are of great significance for understanding the chemical behaviors of heavy metals at root/soil interfaces under Cd and Pb stress and the detoxification mechanisms of medicinal plants.
Author contributions
Yang Zhi and Qixing Zhou conceived and designed the study; Mo Zhou conducted the research and wrote the initial paper; Yang Zhi and Mo Zhou revised the paper; Yueying Dai and Jialun Lv collected data; Yajun Li and Zehua Wu had primary responsibility for the final content.
Conflicts of interest
There are no conflicts to declare.
Acknowledgements
This work was financially supported by the National Natural Science Foundation of China (No. 31600415).
References
- F. J. Zhao, Y. Ma and Y. G. Zhu, Soil Contamination in China: Current Status and Mitigation Strategies, Environ. Sci. Technol., 2015, 49(2), 750–759, DOI:10.1021/es5047099.
- Y. X. Cai, S. H. Zhang and K. Z. Cai, Cd accumulation, biomass and yield of rice are varied with silicon application at different growth phases under high concentration Cd-contaminated soil, Chemosphere, 2020, 242, 125128, DOI:10.1016/j.chemosphere.2019.125128.
- S. M. El-Bahi and N. W. El-Dine, A El-Shershaby, Elemental analysis of egyptian phosphate fertilizer components, Health Phys., 2004, 86(3), 303–307 CrossRef CAS PubMed.
- E. Rodriguez, R. Azevedo, P. Fernandes and ão. S. Conceic, Cr(VI) Induces DNA Damage, Cell Cycle Arrest and Polyploidization: A Flow Cytometric and Comet Assay Study in Pisum sativum, Chem. Res. Toxicol., 2011, 24(7), 1040–1047, DOI:10.1021/tx2001465.
-
M. Dikilitas, S. Karakas, P. Ahmad. Effect of lead on plant and human DNA damages and its impact on the environment, in Plant Metal Interaction Emerging Remediation Techniques, ed. Parvaiz Ahmad, Elsevier; Amsterdam, 2016pp. 41–67, DOI:10.1016/B978-0-12-803158-2.00003-5.
- S. J. Murch, K. Haq, H. P. V. Rupasinghe and P. K. Saxena, Nickel contamination affects growth and secondary metabolite composition of St. John's wort (Hypericum perforatum L.), Environ. Exp. Bot., 2003, 49, 251–257, DOI:10.1016/S0098-8472(02)00090-4.
-
L. H. Zhao, Y. H. Yang, Y. C. Hu, Current situation analysis and countermeasures on contamination of heavy metal in traditional Chinese medicinal materials in China, Zhong Cao Yao, 2014, DOI:10.7501/j.issn.0253-2670.2014.09.001.
-
R. L. Chaney, S. L. Brown, Y. M. Li, J. S. Angle, T. I. Stuczynski, W. L. Daniels, et al., Progress in risk assessment for soil metals, and in situ remediation and phytoextraction of metals from hazardous contaminated soils, Proc. US-EPA Phytorem: State Sci, Boston, MA. 2000 Search PubMed.
- S. Cheng, W. Grosse, F. Karrenbrock and M. Thoennessen, Efficiency of constructed wetlands in decontamination of water polluted by heavy metals, Ecol. Eng., 2002, 18(3), 317–325, DOI:10.1016/S0925-8574(01)00091-X.
- M. M. Lasat, Phytoextraction of toxic metals: a review of biological mechanisms, J. Environ. Qual., 2002, 31(1), 109–120, DOI:10.2134/jeq2002.1090.
-
S. Fahimirad and M. Hatami, Heavy Metal-Mediated Changes in Growth and Phytochemicals of Edible and Medicinal Plants, in: Medicinal Plants and Environmental Challenges,ed Mansour Ghorbanpour Ajit Varma, Springer, Switzerland, Cham; 2017. pp. 189–214 Search PubMed.
- G. Wieshammer, R. Unterbrunner, T. B. García, M. F. Zivkovic, M. Puschenreiter and W. W. Wenzel, Phytoextraction of Cd and Zn from agricultural soils by Salix ssp. and intercropping of Salix caprea and Arabidopsis halleri, Plant Soil, 2007, 298, 255–264, DOI:10.1007/s11104-007-9363-9.
- Y. Zhi, T. Sun and Q. X. Zhou, Assessment of Pb tolerance in 23 Chinese soybean cultivars and the effect of Pb on their mineral ion complement, Environ. Sci. Pollut. Res., 2014, 21(22), 12909–12921, DOI:10.1007/s11356-014-3181-4.
- F. A. Manan, T. T. Chai, A. A. Samad and D. D. Mamat, Evaluation of the Phytoremediation Potential of Two Medicinal Plants, Sains Malays., 2015, 44(4), 503–509, DOI:10.17576/jsm-2015-4404-04.
-
Z. L. Huang, Screening of Cd-accumulation Vegetable Cultivars and Research on it's Properties of Cd-accumulation and Physiology, dissertation of Master Degree, Huazhong Agricultural University, 2012.
- A. D. Kent and E. W. Triplett, Microbial communities and their interactions in soil and rhizosphere ecosystems, Annu. Rev. Microbiol., 2002, 56, 211–236, DOI:10.1146/annurev.micro.56.012302.161120.
- O. S. Olanrewaju, A. S. Ayangbenro, B. R. Glick and O. O. Babalola, Plant health: feedback effect of root exudates-rhizobiome interactions, Appl. Microbiol. Biotechnol., 2018 DOI:10.1007/s00253-018-9556-6.
- Y. Wang, W. Ren, Y. Li, Y. Xu, Y. Teng and P. Christie,
et al, Nontargeted metabolic
analysis to unravel the impact of di (2-ethylhexyl) phthalate stress on root exudates of alfalfa (Medicago sativa), Sci. Total Environ., 2019, 646, 212–219, DOI:10.1016/j.scitotenv.2018.07.247.
- H. Bais, T. Weir, L. Perry, S. Gilroy and J. Vivanco, The role of root exudates inrhizosphere interactions with plants and other organisms, Annu. Rev. Plant Biol., 2006, 57, 233–266, DOI:10.1146/annurev.arplant.57.032905.105159.
- Q. Lin, Y. X. Chen, H. M. Chen and C. R. Zheng, Study on chemical behavior of root exudates with heavy metals, Plant. Nut. Fertilizing Sci., 2003, 9(4), 425–431 Search PubMed.
-
D. E. Salt, N. Kato, U. Krämer, R. D. Smith, I. Raskin, The role of root exudates in nickel hyperaccumulation and tolerance in accumulator and non-accumulator species of Thlaspi, in Phytoremediation of contaminated soil and water, ed N. Terry and G. Banuelos, Lewis publishers inc, Boca raton, 2000. pp.189–200 Search PubMed.
- J. Dong, W. H. Mao, G. P. Zhang, F. B. Wu and Y. Cai, Root excretion and plant tolerance to cadmium toxicity-a review, Plant. Soil. Environ., 2007, 53(5), 193, DOI:10.17221/2205-PSE.
- X. F. Mao, J. J. Hen and Z. K. Han, Effects of Pb Stress on Growth and Physiological Metabolism and Accumulation Characteristics of Lonicera Japonica, J. Northeast. Ag. Sci., 2019, 44(5), 69–75, DOI:10.16423/j.cnki.1003-8701.2019.05.016.
- J. You and Z. Chan, ROS regulation during abiotic stress responses in crop plants, Front. Plant Sci., 2015 DOI:10.3389/fpls.2015.01092.
- X. Y. Hao, J. Liang and J. Chen, Effects of Soaking and Spraying of Cu2 +, Mg2 + and Fe2 + on Yield and Some Physiological Indexes of Mung Bean Leaves, J. Northeast. Ag. Sci., 2017, 042(005), 25–29, DOI:10.16423/j.cnki.1003-8701.2017.05.005.
- E. Stoltz and M. Greger, Accumulation properties of As, Cd, Cu, Pb and Zn by four wetland plant species growing on submerged mine tailings, Environ. Exp. Bot., 2002, 47(3), 271–280, DOI:10.1016/S0098-8472(02)00002-3.
- S. W. Chai, Y. M. Wen, Y. L. Zhang and J. F. Zhao, Evaluation on the pollution of agricultural soil heavy metal in Guangzhou City, Res. J. Environ. Sci., 2006, 19, 138–142, DOI:10.13198/j.res.2006.04.140.chaishw.027.
- W. T. Liu, Q. X. Zhou, Y. L. Zhang and S. H. Wei, Lead accumulation in different Chinese cabbage cultivars and screening for pollution-safe cultivars, J. Environ. Manag., 2010, 91, 781–788, DOI:10.1016/j.jenvman.2009.10.009.
- Y. Zhi, Q. Zhou, X. Leng and C. Zhao, Mechanism of Remediation of Cadmium-Contaminated Soil With Low-Energy Plant Snapdragon, Front. Chem., 2020, 8, 222, DOI:10.3389/fchem.2020.00222.
- S. Wei, Q. Zhou and X. Wang, Identification of weed plants excluding the uptake of heavy metals, Environ. Int., 2005, 31(6), 829–834, DOI:10.1016/j.envint.2005.05.045.
- A. C. Maehly and B. Chance, [136] The assay of catalases and peroxidases, Methods Biochem. Anal., 1954, 1, 357–424 CAS.
-
Y. Zhi, Cadmium and Lead Low Accumulation among Chinese Soybean Cultivars and the Evaluation of Quality Difference and Correlation, PhD thesis, Northeastern University, 2015.
-
H. Aebi, [13] Catalase in vitro, Methods Enzymol, Acad. Press, vol. 105, 1984, pp. 121–126. DOI:10.1016/S0076-6879(84)05016-3.
- C. Sun, T. Lv, L. Huang, X. Liu, C. Jin and X. Lin, Melatonin ameliorates aluminum toxicity through enhancing aluminum exclusion and reestablishing redox homeostasis in roots of wheat, J. Pineal Res., 2020, 68(4), e12642, DOI:10.1111/jpi.12642.
- F. Abd Manan, T. T. Chai, A. Abd Samad and D. D. Mamat, Evaluation of the phytoremediation potential of two medicinal plants, Sains Malays., 2015, 44(4), 503–509, DOI:10.17576/jsm-2015-4404-04.
- F. K. Zengin and O. Munzuroglu, Effects of some heavy metals on content of chlorophyll, proline and some antioxidant chemicals in bean (Phaseolus vulgaris L.) seedlings, Acta Biol. Cracov., Ser. Bot., 2005, 47(2), 157–164 Search PubMed.
- M. M. Islam, M. A. Hoque, E. Okuma, M. N. A. Banu, Y. Nakamura, Y. Shimoishi and Y. Murata, Exogenous proline and glycinebetaine increase antioxidant enzyme activities and confer tolerance to cadmium stress in cultured tobacco cells, J. Plant Physiol., 2009, 166(15), 1587–1597, DOI:10.1016/j.jplph.2009.04.002.
- S. Hayat, Q. Hayat, M. N. Alyemeni, A. S. Wani, J. Pichtel and A. Ahmad, Role of proline under changing environments: a review, Plant Signaling Behav., 2012, 7(11), 1456–1466, DOI:10.4161/psb.21949.
- S. S. Sharma, H. Schat and R. Vooijs, In vitro alleviation of heavy metal-induced enzyme inhibition by proline, Phytochem, 1998, 49(6), 1531–1535, DOI:10.1016/S0031-9422(98)00282-9.
- M. S. Wael, M. R. Mostafa, A. A. E. M. Taia, M. H. Saad and T. A. Magdi, Alleviation of cadmium toxicity in common bean (Phaseolus vulgaris L.) plants by the exogenous application of salicylic acid, J. Hortic. Sci. Biotechnol., 2015, 90(1), 83–91, DOI:10.1080/14620316.2015.11513157.
- E. Okuma, Y. Murakami, Y. Shimoishi, M. Tada and Y. Murata, Effects of exogenous application of proline and betaine on the growth of tobacco cultured cells under saline conditions, Soil Sci. Plant Nutr., 2004, 50(8), 1301–1305, DOI:10.1080/00380768.2004.10408608.
- H. Hammami, M. Parsa, M. H. R. Mohassel, S. Rahimi and S. Mijani, Weeds ability to phytoremediate cadmium-contaminated soil, Int. J. Phytorem., 2016, 18(1), 48–53, DOI:10.1080/15226514.2015.1058336.
- J. Tang, H. Y. Xu and C. Q. Wang, Effect of Cd stress on root growth and organic acids and amino acid secretion of three rice varieties, J. Hunan Agric. Univ., 2016 DOI:10.13331/j.cnkI.jhau.2016.02.002.
- J. Liao and Mo L. Jie He, Effects of Cd Stress on Cd and Free Amino Acids Content in Sugarcane [J], Southwest China J. Agric. Sci., 2018, 031(002), 296–299 Search PubMed.
- L. B. Zoghlami, W. Djebali, Z. Abbes, H. Hediji, M. Maucourt and A. Moing, Metabolite modifications in Solanum lycopersicum roots and leaves under Cd stress, Afr. J. Biotechnol., 2011, 10(4), 567–579 CAS.
- V. Zemanová, M. Pavlík and D. Pavlíková, Cd toxicity induced contrasting patterns of concentrations of free sarcosine, specific amino acids and selected microelements in two Noccaea species, PloS One, 2017, 12(5) DOI:10.1371/journal.pone.0177963.
- S. Radić, M. Babić, D. Škobić, V. Roje and B. Pevalek-Kozlina, Ecotoxicological effects of aluminum and zinc on growth and antioxidants in Lemna minor L, Ecotoxicol. Environ. Saf., 2010, 73(3), 336–342, DOI:10.1016/j.ecoenv.2009.10.014.
- K. Leskó and L. Simon-Sarkadi, Effect of Cd stress on amino acid and polyamine content of wheat seedlings, Period. Polytech., Chem. Eng., 2002, 46(1), 65–71 Search PubMed.
- A. Vassilev and F. Lidon, Cd-induced membrane damages and changes in soluble protein and free amino acid contents in young barley plants, Emir. J. Food Agric., 2011, 23(1), 130, DOI:10.9755/ejfa.v23i2.6347.
- F. Zhang, X. Li, C. Wang and Z. Shen, Effect of Cd on autoxidation rate of tissue and inducing accumulation of free proline in seedlings of mung bean, J. Plant Nutr., 2000, 23(3), 357–368, DOI:10.1080/01904160009382022.
- G. Costa and J. L. Morel, Water relations, gas-exchange and amino-acid content in Cd-treated lettuce[J], Plant Physiol. Biochem., 1994, 32(4), 561–570 CAS.
-
S. Yin, The physiology of two compositae plants tolerant to Pb stress and the environmental remediation of Pb pollution [D]. Jiangxi University of Finance and Economics. ( 2014) Search PubMed.
- A. Michalak, Phenolic compounds and their antioxidant activity in plants growing under heavy metal stress, Pol. J. Environ. Stud., 2006, 15(4), 523–530 CAS.
- F. Q. Zhang, Y. S. Wang, Z. P. Lou and J. D. Dong, Effect of heavy metal stress on antioxidative enzymes and lipid peroxidation in leaves and roots of two mangrove plant seedlings (Kandelia candel and Bruguiera gymnorrhiza), Chemosphere, 2007, 67(1), 44–50, DOI:10.1016/j.chemosphere.2006.10.007.
- M. Choudhary, U. K. Jetley, M. A. Khan, S. Zutshi and T. Fatma, Effect of heavy metal stress on proline, malondialdehyde, and superoxide dismutase activity in the cyanobacterium Spirulina platensis-S5, Ecotoxicol. Environ. Saf., 2007, 66(2), 204–209, DOI:10.1016/j.ecoenv.2006.02.002.
Footnote |
† Mo Zhou, Yueying Dai, Jialun Lv, Yajun Li and Zehua Wu are undergraduates of Shenyang Pharmaceutical University. |
|
This journal is © The Royal Society of Chemistry 2020 |
Click here to see how this site uses Cookies. View our privacy policy here.