DOI:
10.1039/D0RA04807K
(Paper)
RSC Adv., 2020,
10, 43894-43903
In vitro kinetic release study, antimicrobial activity and in vivo toxicity profile of a kojic acid ester-based nanoemulsion for topical application
Received
31st May 2020
, Accepted 9th November 2020
First published on 9th December 2020
Abstract
Nanoemulsions have emerged as novel vehicles for drug delivery that allow sustained or controlled release for topical application. In this study, kojic acid ester-based nanoemulsion (KAE-NA) was analyzed for in vitro permeation evaluation, kinetic release study, in vitro antimicrobial activity and in vivo toxicity profile on embryonic zebrafish (Danio rerio). Based on KAE-NA in vitro permeation evaluation, the percentage of permeation was significantly improved from 4.94% at 1 h to 59.64% at 8 h of application. The permeation rate of KAE-NA at 8 h was 4659.50 μg cm−2 h−1 (initial concentration, C0 = 2000 μg mL−1) with a permeability coefficient (Kp) value of 0.48 cm h−1. The kinetic release analysis showed the Korsmeyer–Peppas model was the best fitted kinetic model with high linearity [R2 = 0.9964]. Antimicrobial activity of KAE-NA was studied against the skin pathogen bacteria Staphylococcus aureus ATCC 43300. The results indicated that the inhibition zone size of the KAE-NA (8.00 ± 0.0 mm) was slightly bigger than that of its active ingredient, kojic acid ester (6.5 ± 0.0 mm). The toxicity profile of KAE-NA on embryonic zebrafish revealed less toxicity with LC50 (50% lethal concentration) more than 500 μg mL−1. The survival rate of the embryonic zebrafish was more than 80% when treated at doses ranging from 7.81–250 μg mL−1 and showed normal development throughout the experiment without any observed deformation. Hence, KAE-NA proved to be less toxic on the embryonic zebrafish.
1. Introduction
Applying the new generation of nanotechnology for topical delivery systems offers numerous advantages in targeting active therapeutic ingredients to the desired site. This includes greater skin retention, improvement in the stability of cosmetic ingredients and long-lasting effect of sustained release of active ingredients that can be achieved. Nanotechnology-based novel carriers of cosmetics include nanoemulsions, nanocapsules, liposomes, niosomes, nanocrystals, solid lipid nanoparticles, carbon nanotubes, fullerenes and dendrimers.1 Basically, nanoemulsions are a kinetically stable system (liquids in the nanoemulsion mixture will be separated very slowly) with droplet size ranging from 20 to 200 nm. Besides, these nanoemulsions performed as a delivery system in transporting various functional lipophilic compounds for drugs, antioxidants, flavors, antimicrobial agents and nutraceuticals products. To date, they are considered to be the most advanced nanoparticulate system for cosmetic applications.2 Research done by Ahmad N. and co-workers, 2019 were successfully prepared a novel curcumin nanoemulsion by ultrasonication with 93.64 ± 6.48 nm droplet size for wound healing treatment. The in vitro drug release using a pre-treated dialysis membrane was 79.64 ± 5.34% after 24 h application.3 Besides, copper peptide in a nanoemulsion system (120.7 nm) was developed to prevent aging. The permeability test results using Franz diffusion cell on cellulose acetate membrane was 21.89 ± 0.53% after 8 h application. Results from this permeation study show that nanoemulsion has the potential to be used as a carrier vehicle for the delivery of copper peptide.4
The advantages of nanoemulsions over micro- and macro-counterparts are their high surface area allowing effective transport properties (the smaller the size of the emulsion, the higher the stability and better capability to carry active ingredients) and they do not have inherent creaming like macroemulsions making the longer shelf life of the products. In terms of delivering lipophilic compounds, nanoemulsions are superior to liposomes due to their lipophilic interior.5 In addition, a topical delivery system is considered to be the best route for transferring active ingredients compare to an oral intravenous pathway. This type of route enhances the bioavailability of drugs, a pain-free method, avoidance of the first-pass effect, patient compliance, steady-state plasma drug pass effect and ease of application.6
Considering these advantages of nanoemulsions as a carrier, kojic acid ester-based nanoemulsion (KAE-NA) can be used for the topical delivery system. Previous research reported kojic acid ester has therapeutic uses for melasma, antioxidant and preservative in foods, antibiotic, chemical intermediate, metal chelate, pesticide, and antimicrobial agents. In addition, kojic acid ester has a lower toxicity value and more stable in terms of storage stability, compatibility and oil-solubility compare to kojic acid itself.7,8 Marta and Jorge (2005) claimed that the composition for topical use of the present invention of kojic acid ester may be prepared in various forms such as emulsion, cream, and powder which can exhibit the melanin synthesis-inhibiting activity, whitening effect, and anti-suntan effect.9 Since emulsion, cream and powder are in micro and macro size, thus kojic acid ester was further study using a nanotechnology system (nanoemulsion) to give optimum stability and compatibility. In spite of that, the safety and efficacy of products are another part of the assessment that should be considered before providing to consumers. The latest research reported that the use of the zebrafish model (Danio rerio) has been accepted and validated for the in vivo toxicity and teratogenicity testing of colloidal nano-drug delivery systems.10
Its advantages as an intermediate model prior to in vivo studies in mammals are the high genetic homology with humans, the molecular and physiological similarities with mammals, the ex utero growth, the ability to perform high-throughput screenings due to the high number of fertilized eggs after spawning and its fast development that allows studying the establishment of the principal organ systems during the first week.11 Unpredicted human safety events in clinical trials for new drugs are costly in terms of human health and money. The drug discovery industry attempts to minimize those events with diligent preclinical safety testing. Current standard practices are good at preventing toxic compounds from being tested in the clinic; however, false-negative preclinical toxicity results are still a reality. Therefore, the continual improvement must be pursued in the preclinical realm. Higher-quality therapies can be brought forward with more information about potential toxicities and associated mechanisms. The embryonic zebrafish model is a bridge between in vitro assays and mammalian in vivo studies. This model is powerful in its breadth of application and tractability for research. In the past two decades, our understanding of disease biology and drug toxicity has grown significantly owing to thousands of studies on this tiny vertebrate.12
The zebrafish embryo model is already accepted as a validated alternative assay to assess fish acute toxicity (OECD, No. 236)13 and currently, the zebrafish embryo is also being explored as a potential replacement for one of the regulatory in vivo mammalian embryo fetal developmental toxicity studies in view of the upcoming third revision of the ICH S5 guideline on detection of the toxicity to reproduction for human pharmaceuticals.14 Moreover, the zebrafish model allows evaluating parameters such as lethal dose, acute toxicity, teratogenicity, and specific-organ toxicity, in particular of the heart and the nervous system.15,16 To add, the transparent body of zebrafish allows us to study the biodistribution of actives and drug delivery systems by diverse imaging techniques.17 This report focused on the safety and efficacy of kojic acid ester-based nanoemulsion significant for topical application.
2. Material and methods
2.1 Materials
Kojic acid ester-based nanoemulsion (110.01 ± 0.14 nm) was prepared using the method described by Syed et al., 2018.18 Roughly, the nanoemulsion was prepared under high and low energy emulsification technique consisting of kojic acid ester (10% w/w), Tween 80 (3.19% w/w), castor oil and lemon oil (3.74% w/w), xanthan gum (0.70% w/w) and deionized water (81.68% w/w). Synthetic membrane (cellulose acetate) was purchased at Advantec, Japan. Phosphate-buffer saline (PBS) was purchased at Sigma Aldrich. Embryonic zebrafish (Danio rerio) kit was obtained from Danio Assay Laboratories Sdn. Bhd, Universiti Putra Malaysia, Malaysia.
2.2 In vitro permeation study
The permeation and percentage release studies of KAE-NA were performed across cellulose acetate (Advantec, Japan) using Franz diffusion cells (Perme Gear, Hellertown, PA, USA) and analyzed using UV-visible spectrophotometer (Shimadzu 1650PC, Japan) at λmax = 306 nm. The Franz diffusion cells consist of two main compartments which were the donor and receptor medium where a cellulose acetate membrane (13 mm) was placed in between them. Both compartments were clipped tightly to avoid leaking of the sample. The area for the diffusion of nanoemulsion between the media was 0.64 cm2. The kojic acid ester based-nanoemulsion (2000 μg mL−1) was placed on top of the donor's compartment whereas the receptor compartment contained phosphate-buffered saline (PBS) (5 mL) with pH 7.4 was maintained at 37 °C and stirred at speed 600 rpm. Every hour, 3 mL was taken out from the receptor compartment and another 3 mL of PBS was replaced into the same compartment. The total volume of 5 mL PBS was maintained inside the receptor compartment throughout the process. A total of nine samples were collected at time t = 0, 1, 2, 3, 4, 5, 6, 7 and 8 h. The 8 h determination indicated nanoemulsion needs to be applied 2 to 4 times daily (or about 4 to 8 h) for topical application.19 The process was carried out in triplicate and samples were analyzed using a UV-visible spectrophotometer with respect to the percentage of cumulative kojic acid ester detected in the receptor compartment.
2.3 Kinetic release study
The kinetic measurement of KAE-NA was determined by the mathematical models; zero-order (cumulative amount of drug release against time, eqn (1)), first-order (log cumulative amount of drug remaining against time, eqn (2)), Higuchi (cumulative percentage of drug release against the square root of time, eqn (3)), Hixson–Crowell (cube root cumulative amount of drug remaining against time, eqn (4)) and Korsmeyer–Peppas (log cumulative percentage of drug release against log time, eqn (5)). The best model fitted the release data was evaluated based on the coefficient determination (R2) obtained from the plotted graph. The models were constructed based on the model's theoretical equation. |
log Mt = log M0 + K1t/2.303
| (2) |
where M0 is the initial amount of kojic acid ester in dissolution media, Mt is the amount of kojic acid ester released in time t, M∞ is the amount of drug released after time ∞, K0, K1, KH, KHC and Kkp are the release rate constants, Q is the cumulative amount of kojic acid ester released in time t per unit area, fraction of kojic acid ester release over time, n is the release exponent and t is the time.
2.4 In vitro antimicrobial activity
In vitro antimicrobial activity of kojic acid ester-based nanoemulsion and kojic acid ester against Staphylococcus aureus ATCC 43300 were studied by means of agar diffusion method. The test was carried out using 6 mm diameter of a paper disc containing antibiotics placed onto a plate for microbe's growth. The microbe culture was standardized to 0.5 McFarland standards (Mueller Hinton agar pH 7.3) with approximately 108 cells. Streptomycin standard was used for each bacterium. Plates were next inverted and incubated at 37 °C for 24 h. After incubation, each plate was examined. The diameters of the zones of complete inhibition were measured to the nearest whole millimeter (mm) using sliding calipers. The clear zone formation (inhibition zone) around the disc indicated antimicrobial activity. Positive (Streptomycin) and negative (deionized water) controls were performed to verify the testing conditions. All tested samples were carried out triplicate.
2.5 In vivo toxicology profile on embryonic zebrafish (Danio rerio)
The in vivo toxicity profile on embryonic zebrafish (Danio rerio) was performed in accordance with the guidelines for care and use of Animal Biochemistry & Biotechnology Laboratory, Faculty of Biotechnology & Biomolecular Sciences, Universiti Putra Malaysia and approved by the Institutional Animal Care and Use Committee, Universiti Putra Malaysia (UPM/IACUC/AUP No. R059/2018).
The collected synchronized embryonic zebrafish were arrayed by pipette into a 96-well plate, one embryo per well with a 200 mL embryo medium. The prepared inhibitor solutions (in 1% DMSO) were added to the embryo medium from 0 to 120 hpf (hours post-fertilization, total 120 h exposure). Stereomicroscope was employed for observing the effects on the pigmentation of zebrafish. Phenotype based evaluation of the body pigmentation was performed at 120 hpf. Embryos were deteriorated by forceps, anesthetized in tricaine methanesulfonate solution (Sigma-Aldrich), mounted in 1% methylcellulose on a depression slide (Aquatic Eco-Systems, Apopka, FL, USA) and photographed under the stereomicroscope Z16 (Leica Microsystems, Ernst-Leitz-Strasse, Germany) for observation. Images capturing and pixel measurement analyses were carried out.
2.6 Statistical analysis
Statistical analysis was carried out using GraphPad Prism 8.0.2. Each experiment was performed in triplicate and the data were expressed as mean ± standard deviation.
3. Results and discussion
3.1 In vitro permeation evaluation
Permeation studies aimed to achieve good skin penetration by assessing the relationship between the skin, active ingredient and formulation. In this study, cellulose acetate membrane was used in Franz cell diffusion to stimulate the skin instead of animal skin or human cadaver skin to perform the in vitro permeation study.20 As reported by Ng. et al., (2010), the porous synthetic membrane-like cellulose acetate membrane was suggested by the Food and Drug Administration (FDA) for topical assessment due to the non-rate limiting barrier.21 Usually, this assessment is used excised human or animal skin but when biological skin is not readily available, synthetic membranes are employed. The ultimate function of synthetic membrane employed in Franz cell drug diffusion studies are for simulation of the skin22,23 and quality control.24 The absorption of the active matter was indicated by transporting the active matter through the cellulose acetate membrane.25 Shah et al., 1989 from FDA used different microporous membranes, namely cellulose acetate, cellulose and polysulfone of similar pore sizes and thicknesses to examine the permeation of hydrocortisone (HC) from two commercial creams. They found that the HC flux was consistent irrespective of the types of synthetic membrane.26
Fig. 1 shows the calibration curve of kojic acid ester. The calibration showed excellent linearity over the concentration range from 62.5 to 1000 μg mL−1. The mean linear regression equation of the calibration curve is y = 0.0003x + 0.0015, with a correlation coefficient of 0.998. These results were used to find the concentration of kojic acid ester in the receptor cells of the Franz diffusion cell and calculate the amount of kojic acid ester transferred through the cellulose acetate membrane.
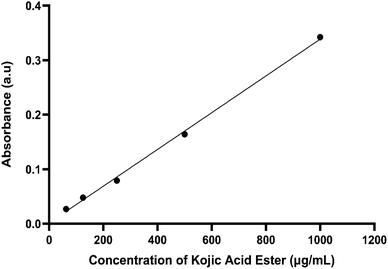 |
| Fig. 1 Calibration curve of kojic acid ester (μg mL−1). | |
Fig. 2 shows the percentage of cumulative permeation of KAE-NA. The permeability of the kojic acid ester was significantly improved and the release of the active matter increased from 4.94% at 1 h to 59.64% at 8 h. Based on the release study, it seems that kojic acid ester released faster particularly at the first 4 h and this may due to the smaller droplet size of the nanoemulsion which has a higher surface area, therefore made the initial release faster.
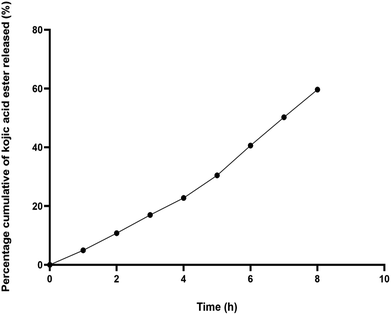 |
| Fig. 2 In vitro permeation study for kojic acid ester based-nanoemulsion (KAE-NA). | |
Fig. 3 demonstrates a permeation profile of KAE-NA. The permeation rate and permeation coefficient, Kp are shown in Table 1. Kojic acid ester based-nanoemulsion showed the highest permeation rate of kojic acid ester at 4659.50 μg cm−2 h−1 with Kp value of 0.48 cm h−1.
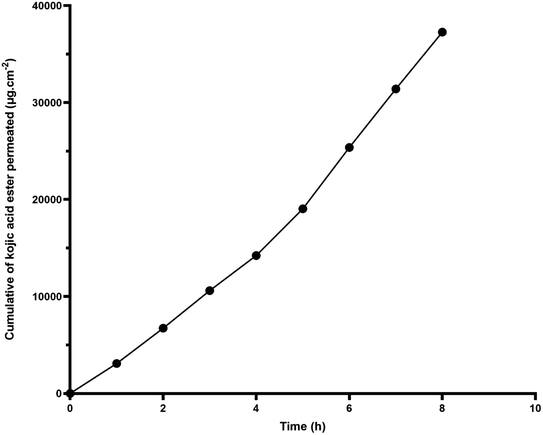 |
| Fig. 3 Cumulative kojic acid ester permeation from nanoemulsion (KAE-NA) through cellulose acetate membrane. | |
Table 1 The permeation parameters of kojic acid ester based-nanoemulsion
Sample |
Flux at 8 h (J, μg cm−2 h−1) |
Permeated amount at 8 h (%) |
Permeation coefficient, Kp (cm h−1) |
Kojic acid ester based-nanoemulsion |
4659.50 |
59.64 |
0.48 |
Apart from the small droplet size of nanoemulsion, the surfactant used in the system was able to compromise with the barrier function of the cellulose acetate membrane thus, facilitate the passage of kojic acid ester. In addition, according to Tsai et al., (2014) the nanoemulsions could change the surface electrical charge of the drug and enhance its' permeability. Based on the results, nanoemulsion can be used as a successful carrier vehicle for the delivery of kojic acid ester.27
3.2 Kinetic release analysis
In order to determine the kinetic release pattern of kojic acid ester-based nanoemulsion, the in vivo permeation data were substituted with five different kinetic models. The release rate was calculated from the slope of appropriate plots and the regression coefficient (R2) was determined as shown in Table 2. Based on Fig. 4, the kinetic release of kojic acid ester-based nanoemulsion was best fitted with the Korsmeyer–Peppas model with high linearity (R2 = 0.9964). Also, the model Korsmeyer–Peppas equation states the type of diffusion, which was evaluated by release exponent, n = 1.1194 which is higher than 0.89. This implies that the KAE-NA release from the system follows Super case II transport.
Table 2 The regression coefficient (R2) of five different kinetic models for kojic acid ester-based nanoemulsion
Kinetic model |
Regression coefficient (R2) |
Zero-order |
0.9865 |
First-order |
0.8210 |
Higuchi |
0.9390 |
Hixson–Crowell |
0.9009 |
Korsmeyer–Peppas |
0.9964 |
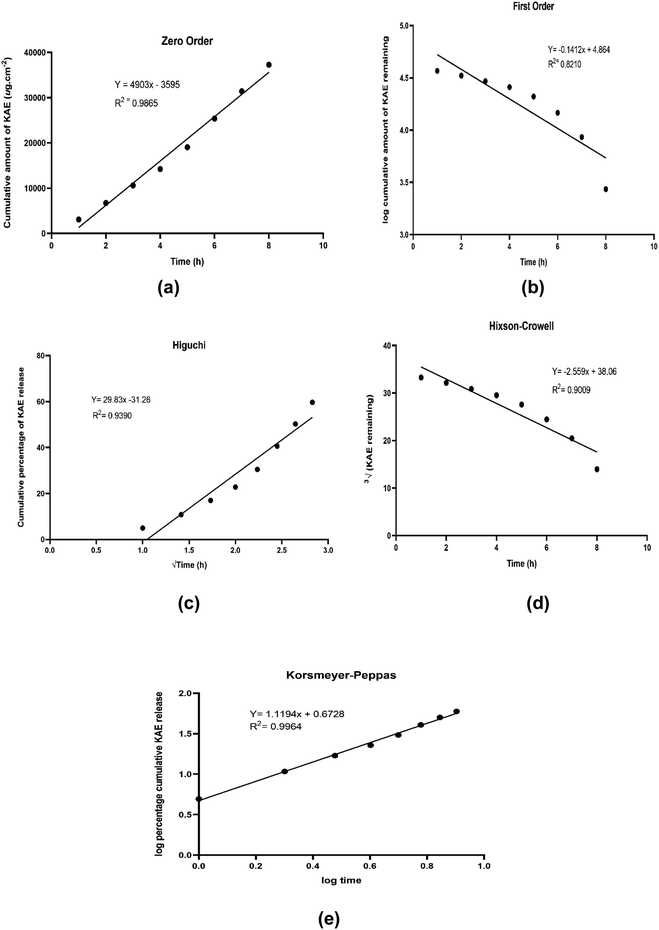 |
| Fig. 4 Graph of kinetic models (a) zero order (b) first-order (c) Higuchi (d) Hixson–Crowell (e) Korsmeyer–Peppas. | |
3.3 In vitro antimicrobial activity
The in vitro antimicrobial activity against the pathogen bacteria Staphylococcus aureus ATCC 43300 (S. aureus) on kojic acid ester-based nanoemulsion (KAE-NA) and kojic acid ester (KAE) were investigated. Based on the literature, Staphylococcus aureus (S. aureus) is a Gram-positive bacterium that capable of causing numerous diseases of the human skin. The incidence of S. aureus skin infections reflects the conflict between the host skin's immune defenses and the S. aureus' virulence elements. Besides, S. aureus is a common component of human skin microbiota and it colonizes the nasal mucosa of the world's population.28 Furthermore, S. aureus colonization has a negative impact on some chronic inflammatory dermatose such as atopic dermatitis (AD), a multifactorial complex disease that causes skin barrier dysfunction.29,30 It is also the primary cause of delayed healing and infection in both acute and chronic wounds and often produce invasive infections that can even cause sepsis.31,32 S. aureus represent the most common source of skin and soft tissue infections (SSTIs) for Gram-positive bacteria.33 Therefore, the antimicrobial assessment of Staphylococcus aureus on kojic acid ester-based nanoemulsion for topical application was studied.
The results of the inhibition zone size activity of Staphylococcus aureus ATCC 43300 on both samples were shown in Table 3 and Fig. 5 respectively. Based on the results, kojic acid ester-based nanoemulsion gave better inhibition size (8.0 mm) as compared to the active ingredient, kojic acid ester (6.5 mm). These findings may due to the composition of nanoemulsion which possesses high stability towards particle aggregation and gravitational separation showing improved and efficient activity such as antimicrobial activity.34 Even though the active ingredient in the nanoemulsion, kojic acid ester was reported to have antibacterial properties against bacteria and fungi,35,36 the encapsulation of kojic acid ester in nanoemulsion gave better inhibition results due to its improvement in stability, controlled particle size and utilization for topical delivery. Since kojic acid ester has low solubility in water, it needs to be encapsulated into a suitable delivery system, in order to be active in topical application, where microorganism are more likely to grow and proliferate.18
Table 3 The inhibition zone size effect of kojic acid ester-based nanoemulsion, kojic acid ester and controls towards Staphylococcus aureus ATCC 43300
Samples |
Diameter zone inhibition on bacteria Staphylococcus aureus ATCC 43300 (mm) |
Kojic acid ester |
6.5 ± 0.0 |
Kojic acid ester-based nanoemulsion |
8.0 ± 0.0 |
Positive control (Streptomycin) |
28.0 ± 0.1 |
Negative control (deionized water) |
No inhibition |
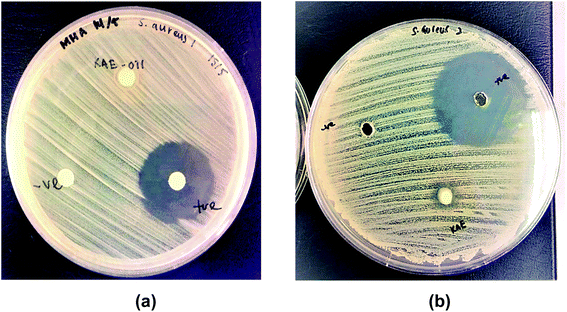 |
| Fig. 5 Inhibition zone of (a) kojic acid ester and (b) kojic acid ester-based nanoemulsion towards Staphylococcus aureus ATCC 43300. | |
Presumably, the mechanism action of the antimicrobial nanoemulsion against S. aureus involved by bringing the kojic acid ester into proximity with the cell membrane. The trapped kojic acid ester molecules in the inner phase of nanoemulsion may result in an extended drug release and a prolonged activity. Delivery systems at the nanoscale can potentially increase the passive cellular absorption mechanisms, thus reducing mass transfer resistances and increasing antimicrobial activity. Besides, the addition of lemon essential oil and potassium sorbate in the kojic acid ester-based nanoemulsion may also help in the inhibition growth of bacteria. The antimicrobial action of essential oil has been attributed to their phenolic compounds and their interaction with microbial cell membranes. They are known to penetrate through the microbial membrane and cause the leakage of ions and cytoplasmatic content thus leading to a cellular breakdown.37 Previous research by Pannu et al. 2011, developed novel NB-002 oil-in-water nanoemulsions with broad antifungal activity against dermatophytes and activity against propionibacterium acne for topical treatment of skin, hair and nail infections.38 Besides, Hamouda et al. 2001, have presented a novel nanoemulsion formulation with a unique topical antimicrobial activity against bacteria, enveloped viruses and fungi including H. influenzae, N. gonorrhoeae and V. cholera, P. aeruginosa.39
3.4 Toxicology evaluation using embryonic zebrafish (Danio rerio)
The exposure concentrations of kojic acid ester-based nanoemulsion (110.01 nm) for in vivo toxicity evaluation using embryonic zebrafish were ranging from 7.81–500 μg mL−1. Besides the survival rate, hatching rate and teratogenic defects were also studied as suggested by Rizzo et al., 2013.17 Table 4 shows the toxicity effect of kojic acid ester-based nanoemulsion expresses as survival and mortality of embryos after 96 hours, post-exposure is the concentration-dependent whereby the embryo mortality increased with increased concentration of sample. Based on the results, there were only a few embryos were coagulated after 96 hpf even at high concentration. The calculated LC50 values of kojic acid ester-based nanoemulsion showed less toxic effect with LC50 more than 500 μg mL−1. Fig. 6 demonstrates the survival rate of embryonic zebrafish treated with kojic acid ester-based nanoemulsion at 0 to 120 h. The survival rate of the embryonic zebrafish showed more than 80% when treated at doses ranging from 7.81–250 μg mL−1. Embryos that survived showed normal development normally throughout the experiment without any observed deformation. This indicates that the concentrations tested are less toxic to the embryos and may offer for further cosmeceutical application.
Table 4 Toxicity effect of kojic acid ester-based nanoemulsion on embryonic zebrafish after 96 hpf
Sample |
Concentration (μg mL−1) |
Total embryos tested |
Percentage (%) |
Percentage (%) |
LC50 (μg mL−1) |
Survival |
Death |
Normal |
Abnormal |
Kojic acid ester-based nanoemulsion |
500.00 |
36.00 |
75.00 |
25.00 |
66.67 |
33.33 |
>500 |
250.00 |
36.00 |
83.33 |
16.67 |
83.33 |
16.67 |
125.00 |
36.00 |
83.33 |
16.67 |
83.33 |
16.67 |
62.50 |
36.00 |
83.33 |
16.67 |
83.33 |
16.67 |
31.25 |
36.00 |
91.67 |
8.33 |
83.33 |
16.67 |
15.63 |
36.00 |
91.67 |
8.33 |
83.33 |
16.67 |
7.81 |
36.00 |
91.67 |
8.33 |
83.33 |
16.67 |
Embryo medium + 0.1% DMSO |
— |
36.00 |
100.00 |
0.00 |
100.00 |
0.00 |
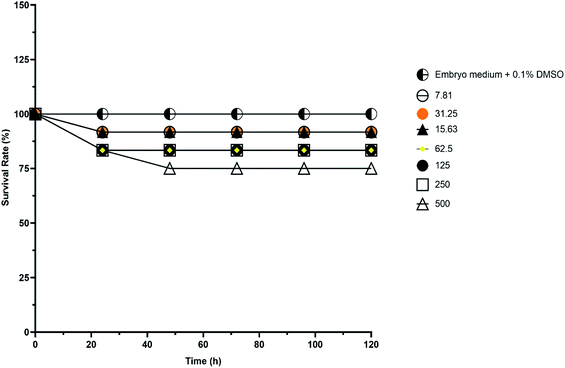 |
| Fig. 6 The survival rate of embryonic zebrafish treated with kojic acid ester-based nanoemulsion at 0 to 120 h at different sample concentrations. | |
Hatching rate of the embryonic zebrafish treated with kojic acid ester-based nanoemulsion at 24 to 120 h as shown in Table 5. Fig. 7 shows the healthy images of zebrafish embryogenesis showing stages of development at different hours of post-fertilization (hpf) captured using an inverted microscope at 10× digital magnification. According to previous studies, embryonic zebrafish is considered healthy if the embryos exhibit all the epical endpoint characteristics such as the full formation of somite (body segment), presence of a heartbeat, tails completely detached from the yolk sac and no coagulation inside the embryos.40–42
Table 5 Hatching rate of embryonic zebrafish treated with kojic acid ester-based nanoemulsion at 24 to 120 h
Sample Concentration (μg mL−1) |
Time of exposure (h) |
24 |
48 |
72 |
96 |
120 |
500.00 |
0.00 |
16.67 |
66.67 |
66.67 |
66.67 |
250.00 |
0.00 |
0.00 |
83.33 |
83.33 |
83.33 |
125.00 |
0.00 |
0.00 |
83.33 |
83.33 |
83.33 |
62.50 |
0.00 |
0.00 |
83.33 |
83.33 |
83.33 |
31.25 |
0.00 |
0.00 |
91.67 |
91.67 |
91.67 |
15.63 |
0.00 |
0.00 |
91.67 |
91.67 |
91.67 |
7.81 |
0.00 |
0.00 |
91.67 |
91.67 |
91.67 |
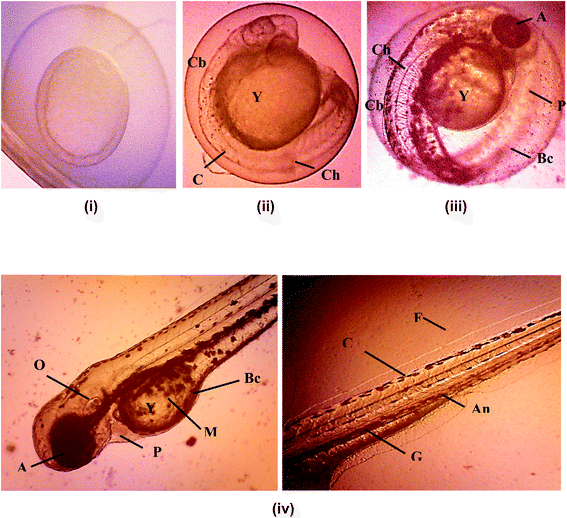 |
| Fig. 7 Healthy images of zebrafish embryogenesis showing 4 stages of development at different hours of post fertilization (hpf) captured using inverted microscope at 10× digital magnification. (i) Blastula period (4 hpf); (ii) segmentation period (24 hpf); (iii) pharyngula period (48 hpf); (iv) hatching period (72 hpf). A-eye anlarge; An-anus; Bc-blood cells; C-chorda; Ch-chorion; Cb-curved body; F-fin; G-gut; M-melanophores; O-ear bud; P-pericard; Y-yolk sac. Scale bar = 0.5 mm. | |
Apart from that, the advantages of using zebrafish is that the eggs from fertilization to pharyngulation stage are transparent where the tissues become dense and pigmentation started to appear. Thus, this enables monitoring of all of the organs and systems for any changes during earlier developmental stages. In addition, teratogenic zebrafish embryos with lacking specific organs or having abnormal organs function can survive well beyond the time where organs normally start to function in healthy individuals.43
Fig. 8 shows images of malformation defect in zebrafish embryo and larvae after 120 h of exposure to a 500 μg mL−1 of KAE-NA captured using microscope 100× digital magnification. Common teratogenic effects of the zebrafish embryo include the curved tail, short body length, curved body, pericardial edema. Wang, 2011 reported the fact that the induction of proteratogenic or procarcinogenic agents without the involvement of exogenous metabolic activation systems in the embryonic zebrafish can be promoted by activating phase I enzyme activities at very clear embryonic stages.44 Therefore, the presence of teratogenicity in the developing larvae was slightly increased with the increasing concentration of the sample. The toxicity effect on morphological features of the zebrafish embryo was not dosed dependent as evident by different teratogenic defects across all concentrations of KAE-NA.
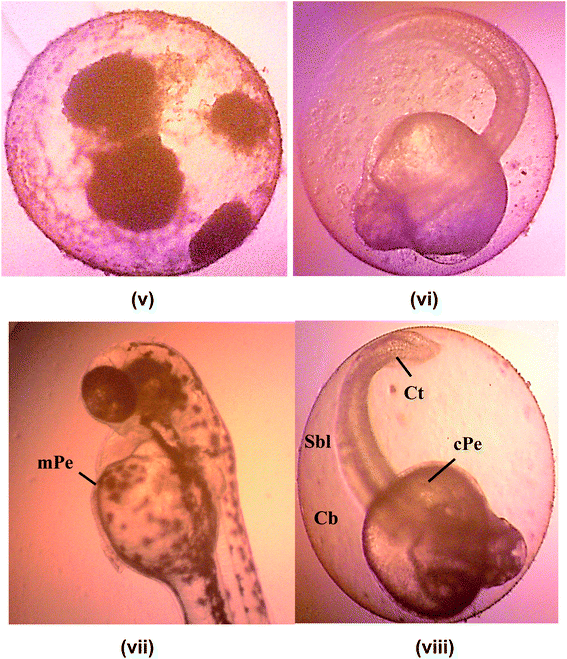 |
| Fig. 8 Images of malformation defect in zebrafish embryo and larvae after 120 hours of exposure to a different concentration of KAE-NA captured using microscope 100× digital magnification. (v) Coagulated embryo; (vi) unhatched embryo; (vii) mPe-mild pericardial edema; (viii) Sbl-short body length, Cb-curve body, Ct-curve tail, cPe-chronic pericardial. Scale bar = 0.5 mm. | |
This may be because the morphological defect was recorded at a low concentration of kojic acid ester based-nanoemulsion. These teratogenic effects arise concurrently and non-concurrently in individual zebrafish larvae. The previous researcher documented the fact that the presence of organic fatty acids may affect the metamorphosis and formation of the larval skin which predisposes to high risk of morphological defects.45 Besides, research had shown that injury, disease, parasite, stress-related spawning, unusual water quality conditions (high temperature, low dissolved oxygen, low pH), poor nutrition and toxic algal bloom are some of the factors that contribute to the teratogenic defects.46
4. Conclusion
The permeation studies of kojic acid ester based-nanoemulsion revealed that the permeability of the kojic acid ester was increased from 4.94% at 1 h to 59.64% at 8 h. The permeation rate of kojic acid ester based-nanoemulsion at 8 h was 4659.50 μg cm−2 h−1 (initial concentration, C0 = 2000 μg mL−1) with the permeability coefficient, Kp value of 0.48 cm h−1 shows nanoemulsion could be used as a vehicle carrier for the topical delivery system. The kinetic model suggested that Korsmeyer–Peppas model was the best fitted with high linearity of R2 = 0.9964 indicate that the kinetic release of KAE-NA followed the Super case II transport system. The LC50 of zebrafish embryos (Danio rerio) showed no toxicity effect with more than 500 μg mL−1. Antimicrobial activity of kojic acid ester based-nanoemulsion showed inhibition zone size of the kojic acid ester based-nanoemulsion (8.00 mm) was slightly bigger than kojic acid ester (6.5 mm). This study has revealed that KAE-NA has less toxicity and potentially used for further cosmeceutical applications.
Conflicts of interest
There are no conflicts to declare.
Acknowledgements
The authors would like to thank Universiti Putra Malaysia (UPM) for supporting this work under Universiti Putra Malaysia Grant No. UPM-IPS/9673600.
References
- S. Duarah, K. Pujari, R. D. Durai and V. H. B. Narayanan, Int. J. Appl. Pharm., 2016, 8(1), 8–12 CAS
. - N. Dasguptaa, S. Ranjanab, S. Mundraa, C. Ramalingama and A. Kumarc, Int. J. Food Prop., 2015, 1–21 Search PubMed
. - N. Ahmad, R. Ahmad, A. Al-Qudaihia, S. E. Alaseela, I. Z. Fitaa, M. S. Khalidd and F. H. Pottood, RSC Adv., 2019, 9, 20192–20206 RSC
. - S. Samson, M. Basri, H. R. F. Masoumi, R. A. Karjiban and E. A. Malek, RSC Adv., 2016, 6, 17845–17856 RSC
. - S. Sharma, J. Silva, W. Abebe, S. M. Sousa, V. G. Duarte, M. I. L. Machado and K. Sarangdevot, RSC Adv., 2012, 1(3), 408–415 Search PubMed
. - V. C. Jhawat, V. Saini, S. Kamboj and N. Maggon, Int. J. Pharm. Sci. Rev. Res., 2013, 20, 47–56 CAS
. - S. S. Kang, J. K. Hyoung, J. Changbae and Y. S. Lee, Bioorg. Med. Chem. Lett., 2009, 19, 188–191 CrossRef CAS
. - H. C. J. Kim, J. K. Cho, S. Y. Kim and Y. S. Lee, Bioorg. Med. Chem. Lett., 2004, 14, 2843–2846 CrossRef CAS
. - I. R. Marta and I. G. Jorge, Dermatol. Surg., 2005, 31, 886–889 Search PubMed
. - Y. Li, X. Miao, T. Chen, X. Yi, R. Wang, H. Zhao, S. M. Y. Lee, X. Wang and Y. Zheng, Colloids Surf., B, 2017, 156, 227–235 CrossRef CAS
. - K. Y. Lee, G. H. Jang, C. H. Byun, M. Jeun, P. C. Searson and K. H. Lee, Biosci. Rep., 2017, 37, 20170199 CrossRef
. - S. Cassar, I. Adatto, J. L. Freeman, J. T. Gamse, I. Iturria, C. Lawrence, A. Muriana, R. T. Peterson, S. V. Cruchten and L. I. Zon, Chem. Res. Toxicol., 2020, 33(1), 95–118 Search PubMed
. - OECD, Test No. 236: Fish Embryo Acute Toxicity (FET) Test OECD Guidelines for the Testing of Chemicals, Section 2, OECD Publishing, Paris, France, 2013 Search PubMed
. - International Council for Harmonisation of Technical Requirements for Pharmaceuticals for Human Use Detection of Toxicity to Reproduction for Human Pharmaceuticals S5(R3), https://www.fda.gov/media/108894/download, July 31, 2019.
- M. N. Calienni, C. F. Temprana, M. J. Prieto, D. Paolino, M. Fresta, A. B. Tekinay, V. Alonso and J. Montanari, Drug Delivery Transl. Res., 2017, 8(3), 496–514 Search PubMed
. - D. Raldua and B. Pina, Expert Opin. Drug Metab. Toxicol., 2014, 10, 685–697 Search PubMed
. - L. Y. Rizzo, S. K. Golombek, M. E. Mertens, Y. Pan, D. Laaf, J. Broda and T. Lammers, J. Mater. Chem. B, 2013, 1, 3918–3925 RSC
. - A. S. N. A. Syed, S. E. Ashari and N. Salim, Int. J. Nanomed., 2018, 13, 6456–6479 Search PubMed
. - E. H. Ukomadu, M. Sacha, A. Richter and K. Hussein, Biopharm. Drug Dispos., 2019, 40, 217–224 CrossRef
. - S. H. Hung, Y. H. Lin and G. B. Lee, J. Micromech. Microeng., 2010, 20, 045026 CrossRef
. - S. F. Ng, J. Rouse, D. Sanderson and G. Eccleston, Pharmaceutics, 2010, 2, 209–223 CrossRef CAS
. - J. Twist and J. Zatz, J. Pharm. Sci., 1988, 77, 538–540 CrossRef
. - J. Twist and J. Zatz, J. Soc. Cosmet. Chem., 1986, 37, 429–444 CAS
. - M. Corbo, T. W. Schultz, G. K. Wong and G. A. van Buskirk, Pharm Tech, 1993, 9, 112–128 Search PubMed
. - E. S. Mahdi, A. M. Noor, M. H. Sakeena, G. Z. Abdullah, M. F. Abdulkarim and M. A. Sattar, Int. J. Nanomed., 2011, 6, 2499 CrossRef CAS
. - V. P. Shah, J. S. Elkins, S. Y. Lam and J. P. Skelly, Int. J. Pharm., 1989, 53, 53–59 CrossRef CAS
. - M. Tsai, Y. Fu, Y. Lin, Y. Huang and P. Wu, PLoS One, 2014, 9(7), 1–7 CrossRef CAS
. - H. Asgeirsson, A. Thalme and O. Weiland, Infect. Dis., 2018, 3, 175–192 CrossRef
. - B. Shi, D. Y. Leung, P. A. Taylor and H. Li, J. Invest. Dermatol., 2018, 138, 1668–1671 CrossRef CAS
. - J. Kim, B. E. Kim and D. Y. Leung, Allergy Asthma Proc., 2019, 40, 84–92 CrossRef CAS
. - A. F. Cardona and S. E. Wilson, Clin. Infect. Dis., 2015, 61, S69–S78 CrossRef CAS
. - S. Esposito, M. Bassetti, E. Concia, G. De Simone, F. G. De Rosa, P. Grossi, A. Novelli, F. Menichetti, N. Petrosillo and M. Tinelli, J. Chemother., 2017, 29, 197–214 CrossRef CAS
. - G. E. Stein and E. M. Wells, Curr. Med. Res. Opin., 2010, 26, 571–588 CrossRef CAS
. - N. Dasguptaa, S. Ranjanab, S. Mundraa, C. Ramalingama and A. Kumarc, Int. J. Food Prop., 2015, 1–21 Search PubMed
. - A. F. Lajis, M. Basri, R. Mohamad, M. Hamid, S. E. Ashari, N. Ishak, A. Zookiflie and A. B. Arif, Chem. Pap., 2013, 67(6), 573–585 CAS
. - R. Mohamad, M. S. Mohamed, N. Suhaili, M. M. Salleh and A. Ariff, Biotechnol. Mol. Biol. Rev., 2010, 5(2), 24–37 CAS
. - S. Burt, Int. J. Food Microbiol., 2004, 94(3), 223–253 CrossRef CAS
. - J. Pannu, A. McCarthy, A. Martin, T. Hamouda, S. Ciotti, L. Ma, J. Sutcliffe and J. R. Baker Jr, Antimicrob. Agents Chemother., 2011, 55, 4211–4217 CrossRef CAS
. - T. Hamouda, A. Myc, B. Donovan, A. Y. Shih, J. D. Reuter and J. R. Baker Jr, Microbiol. Res., 2001, 156, 1–7 CrossRef CAS
. - T. Braunbeck, M. Bottcher, H. Hollert, T. Kosmehl, E. Lammer, E. Leist, M. Rudolf and N. Seitz, ALTEX, 2005, 22, 87–102 Search PubMed
. - T. Braunbeck and E. Lammer, Draft detailed review paper on fish embryo toxicityassays, UBA Contract Number 203 85 422, Umweltbundesamt-German Federal Environment Agency, Germany, 2006, p. 136 Search PubMed
. - E. Lammer, G. J. Carr, K. Wendler, J. M. Rawlings, S. E. Belanger and T. Braunbeck, Comp. Biochem. Physiol., Part C: Toxicol. Pharmacol., 2009, 149, 196–209 CAS
. - A. Hill, O. G. Nacoulma and T. R. Guiguemde, J. Ethnopharmacol., 2006, 103(2), 236–240 CrossRef
. - W. X. Wang, Aquat. Toxicol., 2011, 105(3), 9–15 CrossRef CAS
. - A. Sharan, H. Zhu, H. Xie, H. Li, J. Tang, W. Tang and Y. Xia, Sci. Rep., 2015, 5, 9302 CrossRef
. - S. Rajabi, A. Ramazani, M. Hamidi and T. Naji, J. Pharm. Sci., 2015, 23(1), 1 Search PubMed
.
|
This journal is © The Royal Society of Chemistry 2020 |