DOI:
10.1039/D0RA07739A
(Paper)
RSC Adv., 2020,
10, 38468-38477
Stereoselective synthesis and application of isopulegol-based bi- and trifunctional chiral compounds†
Received
9th September 2020
, Accepted 10th October 2020
First published on 19th October 2020
Abstract
A new family of isopulegol-based bi- and trifunctional chiral ligands was developed from commercially available (−)-isopulegol. Nucleophilic addition of primary amines towards (+)-α-methylene-γ-butyrolactone was accomplished, followed by reduction of the obtained β-aminolactones to provide aminodiols in highly stereoselective reactions. Epoxidation of (−)-isopulegol and subsequent oxirane ring opening with primary amines resulted in N-substituted aminodiols. The regioselective ring closure of these aminodiols with formaldehyde was also investigated. Benzylation of isopulegol furnished O-benzyl-protected isopulegol, which was transformed into aminoalcohols via epoxidation and ring opening of the corresponding epoxides. First benzyl-protected isopulegol was subjected to hydroxylation and epoxidation, then aminolysis of the served oxiranes delivered aminodiols. On the other hand, (−)-isopulegol was oxidised to diol, which was again converted into both dibenzyl- and monobenzyl-protected diol derivatives. The products were transformed into aminoalcohols and aminodiols, respectively, by aminolysis of their epoxides. The ring opening of epoxides, derived from diols with primary amines was also performed producing aminotriols. Dihydroxylation of (−)-isopulegol or derivatives with OsO4/NMO gave isopulegol-based di-, tri- and tetraols. The antimicrobial activity and antioxidant property, measuring DPPH˙ free radical scavenging activity of aminodiol and aminotriol derivatives as well as di-, tri- and tetraols were also explored. In addition, structure–activity relationships were examined from the aspects of substituent effects and stereochemistry on the aminodiol and aminotriol systems.
Introduction
Monoterpenes constitute an interesting group of plant secondary metabolites.1,2 They are readily available, relatively nontoxic and inexpensive constituents. Moreover, monoterpenes possess many important pharmacological activities.3 For example, limonene and perillyl alcohol have chemopreventive activity against cancer,4–6 whereas linalool and eucalyptol exert synergistic antiproliferative and anticholinesterase effects.7,8 In addition, some of these compounds, such as 1,8-cineole, geraniol, linalool,9 thymol10 along with limonene, α-pinene, β-pinene, γ-terpinene and linalyl acetate,8 as well as santolina alcohol, borneol, sabinol, trans-sabinyl acetate and α-thujone,10 have been found to be relatively potent DPPH˙ radical scavengers. This property is directly related to their structures.11 It is worth pointing out that essential oils also display excellent antimicrobial activity.12–14 For instance, linalool and α-terpineol exhibited strong activity against periodontopathic and cariogenic bacteria,15 while citral, linalool and β-pinene had an effect on Saccharomyces cerevisiae.16 Furthermore, linalyl acetate, (+)-menthol and thymol were found to be efficient against Staphylococcus aureus and Escherichia coli,17 while thymol, carvacrol, p-cymene and γ-terpinene showed inhibitory activity towards S. aureus and E. coli.18 Apart from proven properties, many monoterpenes exert antibiotic,19,20 nematicidal,21 anti-inflammatory22,23 and analgesic24 influences. Some monoterpenes are used as important flavour agents in foods, drinks, perfumes, cosmetics and tobacco,25 while others such as 1,8-cineole26 and pinene27 have been considered as important biopesticides. Monoterpenes, therefore, are widely used in medicine, industry and agriculture.28–30
We have planned to combine aminodiol moieties of cardiovascular, cytostatic and antiviral effectiveness with monoterpenic skeletons.31–33 Aristeromycin analogues, for example, are widely used as effective agents against a range of viruses, including the human immunodeficiency, hepatitis B, herpes simplex, varicella-zoster, influenza and hepatitis C viruses.34–36 The Abbott aminodiol, found to be a useful building block for the preparation of potent renin inhibitors Zankiren® and Enalkiren®, was introduced into the therapy of hypertension.37,38 Aminodiols can also exert antidepressive activity. For instance, (S,S)-reboxetine is a selective norepinephrine reuptake inhibitor for the treatment of unipolar depression,39 while others such as (2R,3R,7Z)-2-aminotetradec-7-ene-1,3-diol are potent antimicrobial metabolites.40 Besides their varied, well-known influences, aminodiols may serve as starting materials for the synthesis of biologically active natural compounds, e.g. cytoxazone, a selective modulator of the secretion of TH2 cytokine.41,42 Apart from their biological interest, monoterpene-based aminodiols have been demonstrated to be excellent chiral auxiliaries in a wide range of stereoselective transformations including intramolecular radical cyclisation,43 intramolecular [2 + 2] photocycloaddition44 and Grignard addition.45,46
In order to combine the properties of monoterpenes and aminodiols as well as to develop new, efficient and commercially available chiral ligands, naturally occurring chiral monoterpenes such as (+)- and (−)-α-pinene,47–49 (+)-carene,50,51 (+)-camphor,52,53 (−)-fenchone,54 (−)-menthone,55 (−)-myrtenol,56,57 (+)-neoisopulegol,58,59 (S)-perillyl alcohol,60 (−)-pulegone,61 or (+)-sabinol62 have been widely used as key intermediates for the synthesis of aminodiols.
Monoterpene-based diols also possess marked biological properties, e.g. antiparkinsonian activity63 and skin microcirculatory improvement,64,65 whereas monoterpene-based triols have been utilised as cytotoxic66,67 and anti-inflammatory agents.68
Therefore, our primary objective of the present research was to prepare a new library of isopulegol-based bi-, tri- or even tetrafunctionalised chiral synthons, such as aminodiols and aminotriols as well as di-, tri- and tetraols, starting from commercially available natural (−)-isopulegol and to evaluate the influence of these new isopulegol derivatives on antimicrobial attributes on multiple bacterial and yeast strains and their DPPH˙ free-radical scavenging activity.
Results and discussion
The key intermediate (+)-α-methylene-γ-butyrolactone 4 was prepared from commercially available (−)-isopulegol 1. Acetylation of alcohol 1 to its acetate 2, followed by regioselective oxidation of 2 gave diol 3, which was transformed to lactone 4 by two-step oxidation and ring closure of obtained γ-hydroxy-substituted α,β-unsaturated carboxylic acid applying literature methods (Fig. 1).69–74
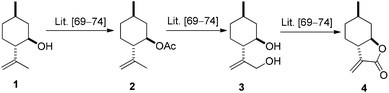 |
| Fig. 1 Synthesis of (−)-isopulegol-based (+)-α-methylene-γ-butyrolactone. | |
Nucleophilic addition of primary amines to α-methylene-γ-butyrolactone 4 has proved to be an efficient method for the preparation of a highly diversified library of β-aminolactones 5–8.58,75 Treatment of β-aminolactones with LiAlH4 resulted in secondary aminodiols 9–12. Debenzylation via hydrogenolysis of aminodiols 9–11 over Pd/C in MeOH gave primary aminodiol 13 in moderate yields. In order to study the regioselectivity of ring closure of the aminodiol function, we attempted to incorporate one of the hydroxy groups of aminodiols into 1,3-oxazinane or 1,3-oxazepinane ring.51,61,76 When aminodiols 9–12 were reacted with HCHO under mild conditions, 1,3-oxazinane were obtained in highly regioselective ring closure. Since either the hydrogenolysis of N-benzyl analogues 9–11 or the formation of the oxazine ring system (14–17) had no effect on the absolute configuration, the relative configuration of the chiral centres of 13–17 is known to be the same as that of 9–12 (Scheme 1).51,76
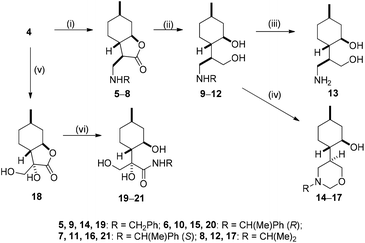 |
| Scheme 1 Synthesis of (−)-isopulegol-based aminodiols. Reaction conditions: (i) RNH2 (1 equiv.), dry EtOH, 25 °C, 20 h, 65–75%; (ii) LiAlH4 (2 equiv.), dry Et2O, 25 °C, 4 h, 50–70%; (iii) 5% Pd/C, H2 (1 atm), MeOH, 25 °C, 24 h, 50–67%; (iv) 35% HCHO, Et2O, 25 °C, 1 h, 64–74%; (v) 2% OsO4/t-BuOH, 50% NMO/H2O, acetone, 25 °C, 24 h, 28%; (vi) RNH2 (4 equiv.), dry THF, 60 °C, 24–72 h, 35–56%. | |
Dihydroxylation of 4 with the OsO4/NMO system furnished 18 in low yield.51,61 The ring opening of α,β-dihydroxylactone 18 was performed by using 4 equivalents of primary amines under reflux conditions in anhydrous THF to form α,β-dihydroxyamides 19–21. It is important to mention that the ring opening of lactones with (R)- and (S)-α-methylbenzylamine required longer reactions than utilizing benzylamine. This is probably due to steric hindrance exerted by the α-methyl group (Scheme 1). Note that the acylation of diols bearing an adjacent amide function forms an important structural moiety with potential biological applications.77 For example, asterobactin and vioprolide A have been identified as a new antibiotic and a new antifungal peptolide, respectively.78,79
The relative configuration of compound 18 was determined by means of NOESY experiments: clear NOE signals were observed between the OH-8 and H-3 as well as OH-9 and H-4 protons (Fig. 2).
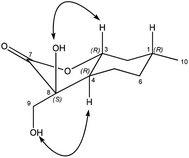 |
| Fig. 2 Determination of the structure of diol 18 by NOESY. | |
Homoallylic epoxidation of (−)-isopulegol 1 with m-CPBA provided a 1
:
1 mixture of epoxides 23a and 23b in good yield.80 The two epoxides were separated by column chromatography to give more polar isomer 23a and less polar isomer 23b. The ring opening of epoxide 23a with different primary amines in the presence of LiClO4 as catalyst delivered aminodiols 24a–27a.81,82 Debenzylation of 24a–26a by hydrogenolysis over Pd/C in MeOH resulted in aminodiol 28a in excellent yields. When aminodiols 24a–27a were treated with HCHO at room temperature, oxazolidines 29a–32a were obtained via highly regioselective ring closures, similar to the regioisomeric 1,3-oxazinane analogues. The other epoxide 23b underwent similar reactions to afford 24b–32b in excellent yields. Dihydroxylation of (−)-isopulegol 1 was performed with OsO4 in the presence of a stoichiometric amount of co-oxidant NMO to afford a diastereoisomeric mixture of 22a and 22b in a ratio of 1
:
1.83 The epimeric mixture was purified by column chromatography followed by recrystallisation to provide 22b in crystalline form and 22a as a colourless oil (Scheme 2).
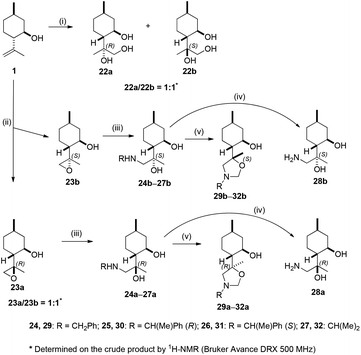 |
| Scheme 2 Synthesis of (−)-isopulegol-based aminodiols. Reaction conditions: (i) 2% OsO4/t-BuOH, 50% NMO/H2O, acetone, 25 °C, 24 h, 33% (22a), 33% (22b); (ii) mCPBA (2 equiv.), Na2HPO4·12H2O (3 equiv.), CH2Cl2, 25 °C, 2 h, 29% (23a), 43% (23b); (iii) RNH2 (2 equiv.), LiClO4 (1 equiv.), MeCN, 70–80 °C, 8 h, 75–95% (23a), 50–90% (23b); (iv) 5% Pd/C, H2 (1 atm), MeOH, 25 °C, 24 h, 87–95% (28a), 85–90% (28b); (v) 35% HCHO, Et2O, 25 °C, 1 h, 89–97% (29a–32a), 85–90% (29b–32b). | |
Gram-scale separation of 23a and 23b turned out to be difficult. In order to enhance the resolution by column chromatography, benzyl-protected isopulegol 33 was prepared.84,85 Epoxidation of 33 with mCPBA furnished a 1
:
1 mixture of epoxides 34a and 34b. After separation by column chromatography, they were subjected to aminolysis with primary amines. Interestingly, epoxide 34b upon aminolysis was transformed preferentially, while 34a did not react. This is probably due to steric hindrance exerted by either the benzyl or the methyl group at the α position in epoxide 34a. Consequently, the mixture of 34a and 34b was used for the ring-opening reaction. The resulting aminoalcohols (35b–38b) could be easily separated from 34a on a gram scale by simple column chromatography with good yields. The synthesis of primary aminodiol 28b was accomplished by hydrogenolysis of 35b–37b over Pd/C in high yields, while debenzylation of 34a provided 23a in a moderate yield of 53% (Scheme 3).
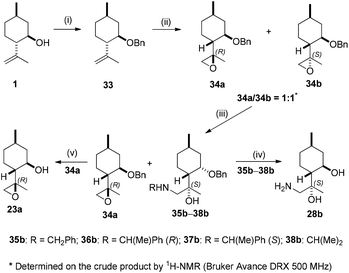 |
| Scheme 3 Synthesis of (−)-isopulegol-based aminodiol derivatives. Reaction conditions: (i) NaH (1.5 equiv.), BnBr (1.5 equiv.), KI (1.5 equiv.), dry THF, 60 °C, 12 h, 70%; (ii) mCPBA (2 equiv.), Na2HPO4·12H2O (3 equiv.), CH2Cl2, 25 °C, 2 h, 43% (34a), 25% (34b); (iii) RNH2 (2 equiv.), LiClO4 (1 equiv.), MeCN, 70–80 °C, 20 h, 31–45%; (iv) 5% Pd/C, H2 (1 atm), MeOH, 25 °C, 24 h, 65–70%; (v) 5% Pd/C, H2 (1 atm), n-hexane : EtOAc = 9 : 1, 25 °C, 24 h, 53%. | |
syn-Selective dihydroxylation of compound 33 with OsO4 in the presence of a stoichiometric amount of co-oxidant NMO produced a 1
:
1.7 epimeric mixture of 39a and 39b in a favourable yield. Our effort to separate the mixture failed. Fortunately, their carbonates, obtained from the diols with triphosgene, could be easily isolated. It is well known that this carbonation reaction maintains the stereochemical configuration of the original diol.86,87 Accordingly, the reactions of 39a and 39b with triphosgene successfully afforded 40a and 40b, respectively. After purification, carbonates 40a and 40b were reduced by LiAlH4 (LAH). The reaction proceeded smoothly giving the corresponding diols 39a and 39b in good yields. It has been reported that reduction with LAH gives the corresponding diol with the same stereochemical configuration of the carbon atoms as of the original moiety.88,89 Debenzylation of 39a and 39b by hydrogenolysis over Pd/C resulted in triols 22a and 22b, respectively, with excellent yields (Scheme 4).
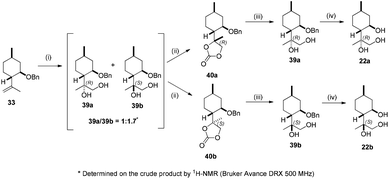 |
| Scheme 4 Synthesis of (−)-isopulegol-based diols. Reaction conditions: (i) 2% OsO4/t-BuOH, 50% NMO/H2O, acetone, 25 °C, 24 h, 88%; (ii) triphosgene (0.5 equiv.), pyridine (4 equiv.), dry CH2Cl2, 25 °C, 2 h, 36% (40a), 36% (40b); (iii) LiAlH4 (2 equiv.), dry Et2O, 0 °C, 4 h, 95% (39a), 56% (39b); (iv) 5% Pd/C, H2 (1 atm), MeOH, 25 °C, 24 h, 95% (39a), 91% (39b). | |
To extend the investigation of the substituent effects in the ring opening of epoxide, 33 was oxidised to 41. The epoxidation of 41 with mCPBA delivered a 4
:
1 mixture of epoxides 42a and 42b. The separation of 42a and 42b was not satisfactory on a gram scale; therefore, the mixture was treated with different primary amines in the presence of LiClO4 resulting in a library of aminodiols. In our delight, aminodiols were well-separated when chiral amines (R)- and (S)-methylbenzylamines were applied, while in the case of benzylamine and isopropylamine, only the major products were isolated. The debenzylation of 43a–45a by hydrogenolysis over Pd/C gave aminodiol 47a with satisfactory yields. Tetraol 49 was prepared by dihydroxylation of 41 with the OsO4/NMO system, followed by hydrogenolysis of 48 over Pd/C (Scheme 5).
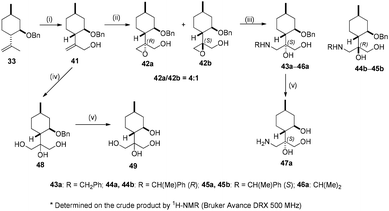 |
| Scheme 5 Synthesis of (−)-isopulegol-based aminotriol derivatives. Reaction conditions: (i) SeO2 (0.24 equiv.), 70% t-BuOOH (4 equiv.), CHCl3, 60 °C, 20 h, then LiAlH4 (3 equiv.), dry Et2O, 0 °C, 6 h, 60%; (ii) mCPBA (2 equiv.), Na2HPO4·12H2O (3 equiv.), CH2Cl2, 25 °C, 2 h, 64% (42a), 15% (42b); (iii) RNH2 (2 equiv.), LiClO4 (1 equiv.), MeCN, 70–80 °C, 6 h, 46–58% (42a), 14% (42b); (iv) NMO/H2O, 2% OsO4/t-BuOH, acetone, 25 °C, 24 h, 60%; (v) 5% Pd/C, H2 (1 atm), MeOH, 25 °C, 24 h, 87–95% (47a), 86% (48). | |
During our attempt to improve the resolution of aminodiols 43b–46b, we realised that O-benzylation of 41 could serve this purpose; however, the synthesis of 50b starting from 41 failed. Fortunately, it was achieved by reacting 3 with benzyl bromide under reflux condition in dry THF. Besides expected product 50b, 50a also formed as a side product. Epoxidation of 50b with mCPBA produced a 1
:
1 mixture of epoxides 51a and 51b. The ring opening of the oxirane mixture was accomplished with different primary amines resulting in a library of aminoalcohols 52a–55a and 52b–55b, respectively. The debenzylation of 52a–54a and 52b–54b by hydrogenolysis over Pd/C gave, respectively, aminotriols 47a and 47b with exceptional yields. Compound 50b was treated with the OsO4/NMO system providing a 3
:
1 mixture of diols 56a and 56b. Removal of the protecting group of 56a gave tetraol 49 with good yield (Scheme 6).
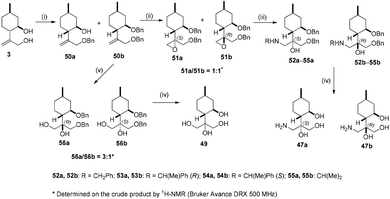 |
| Scheme 6 Synthesis of (−)-isopulegol-based aminotriol derivatives. Reaction conditions: (i) NaH (1.5 equiv.), BnBr (3.0 equiv.), KI (1.5 equiv.), dry THF, 60 °C, 12 h, 40% (50b), 19% (50a); (ii) mCPBA (2 equiv.), Na2HPO4·12H2O (3 equiv.), CH2Cl2, 25 °C, 2 h, 38% (51a), 28% (51b); (iii) RNH2 (2 equiv.), LiClO4 (1 equiv.), MeCN, 70–80 °C, 6 h, 25–40% (51a), 29–42% (51b); (iv) NMO/H2O, 2% OsO4/t-BuOH, acetone, 25 °C, 24 h, 50% (56a), 15% (56b); (v) 5% Pd/C, H2 (1 atm), MeOH, 25 °C, 24 h, 95–98% (47a–b), 83% (56a). | |
The epoxidation of 50a with mCPBA gave a 2
:
1 mixture of epoxides 57a and 57b. The ring opening of this epoxide mixture was carried out with different primary amines to form a library of aminodiols 58a–61a and 58b–61b, respectively. Primary aminotriols 47a and 47b were prepared via the usual way by hydrogenolysis of aminodiols 58a–60a and 58b–60b over Pd/C. Dihydroxylation of 50b with the OsO4/NMO system provided triols 62a and 62b in a 2
:
1 ratio with an excellent yield of 90%. Debenzylation of 62a–b by hydrogenolysis over Pd/C resulted in tetraol 49 with excellent yields (Scheme 7).
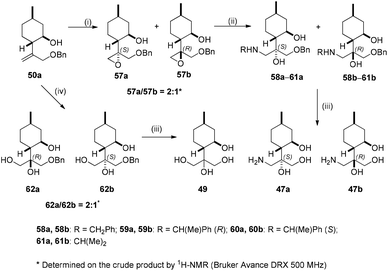 |
| Scheme 7 Synthesis of (−)-isopulegol-based aminotriol derivatives. Reaction conditions: (i) mCPBA (2 equiv.), Na2HPO4·12H2O (3 equiv.), CH2Cl2, 25 °C, 2 h, 38% (57a), 15% (57b); (ii) RNH2 (2 equiv.), LiClO4 (1 equiv.), MeCN, 70–80 °C, 6 h, 39–50% (57a), 16–21% (57b); (iii) 5% Pd/C, H2 (1 atm), MeOH, 25 °C, 24 h, 90–93% (47a–b), 97% (62a), 95% (62b); (iv) NMO/H2O, 2% OsO4/t-BuOH, acetone, 25 °C, 24 h, 59% (62a), 29% (62b). | |
On the other hand, epoxidation of allylic diol 3 with mCPBA was successfully applied to form the mixture of epoxy diols 63a and 63b in a 3.5
:
1 ratio. After separation by chromatography, the oxirane ring of 63a was opened with primary amines and LiClO4 as catalyst to deliver aminotriol library 64a–67a. Primary aminotriol 47a was obtained by debenzylation of the corresponding aminotriols 64a–66a under standard conditions by hydrogenation in the presence of a Pd/C catalyst. Diastereoisomeric aminotriols 65b–66b were prepared by ring opening of 63b with chiral amines (R)- and (S)-methylbenzylamine. The synthesis of tetraol 49 was effectively performed by selective dihydroxylation of 3 with the OsO4/NMO system (Scheme 8).
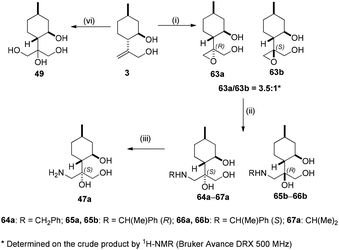 |
| Scheme 8 Synthesis of (−)-isopulegol-based aminotriols. Reaction conditions: (i) mCPBA (2 equiv.), Na2HPO4·12H2O (3 equiv.), CH2Cl2, 25 °C, 2 h, 33% (63a), 7% (63b); (ii) RNH2 (2 equiv.), LiClO4 (1 equiv.), MeCN, 70–80 °C, 6 h, 62–77% (63a), 87–93% (63b); (iii) 5% Pd/C, H2 (1 atm), MeOH, 25 °C, 24 h, 67–75%; (iv) NMO/H2O, 2% OsO4/t-BuOH, acetone, 25 °C, 24 h, 53%. | |
The relative configuration of primary aminotriol 47a was determined through epoxide 63a. To this aim, epoxide 63a was reduced with LiAlH4 (LAH) to the corresponding triol 22a (see configurations in Scheme 9). It has been reported that reduction with LAH gives the corresponding triol with the same stereochemical configuration at the carbon atoms as of the original moiety.88,89 The stereochemical structures of triol 22a is well-known in the literature;83 therefore, the absolute configuration of epoxide 63a could also be determined.
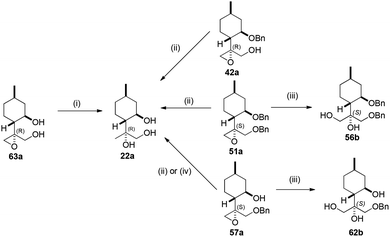 |
| Scheme 9 Determination of the structure of (−)-isopulegol-based aminotriol as well as triol derivatives. Reaction conditions: LiAlH4 (2 equiv.), dry THF, 25 °C, 6 h, 70%; (ii) 5% Pd/C, H2 (1 atm), n-hexane : EtOAc = 9 : 1, 25 °C, 24 h, 90% (42a), 78% (51a), 90% (57a); (iii) 3 M NaOH, DMSO, 25 °C, 2–24 h, 33% (56b), 57% (62b); (iv) LiAlH4 (2 equiv.), dry THF, 25 °C, 6 h then 5% Pd/C, H2 (1 atm), n-hexane : EtOAc = 9 : 1, 25 °C, 24 h, 87%. | |
The absolute configuration of 42a, 51a and 57a was confirmed by debenzylation via hydrogenolysis over Pd/C to provide triol 22a with stereochemical retention. To prove that the stereochemical configuration of the epoxide was maintained during reaction, 57a was reduced with LiAlH4 then debenzylated applying the 5% Pd/C/H2 system to give 22a in good yield. The stereostructure of 56b and 62b were assigned by treatment of 51a and 57a with NaOH taking place with retention of stereochemistry (Scheme 9).90
Since several aminodiols as well as aminotriols exerted antimicrobial activities on various microorganisms,91 antimicrobial activities of the prepared aminodiol and aminotriol analogues were also explored against two yeasts as well as two Gram-positive and two Gram-negative bacteria (Table 1, only the best results are shown).
Table 1 Antimicrobial activity of derivatives expressed in I% values
Anal |
Conc. (μg mL−1) |
Inhibitory effecta (%) ± RSD (%) |
Gram positive |
Gram negative |
Yeast |
B. subtilis |
S. aureus |
E. coli |
P. aeruginosa |
C. albicans |
C. krusei |
Inhibitory effect values less than 20% are considered negligible and not presented numerically. Compounds 1, 3, 9, 13, 14, 18, 19, 22a–b, 24a–b, 28a–b, 30a, 33, 39a–b, 47a–b, 48, 49, 50a, 56b, 62a–b, 64a and 66b were also examined but did not elicit 20% inhibitory effect even at 100 μg mL−1. |
4 |
100 |
31.51 ± 4.58 |
— |
— |
— |
94.30 ± 5.46 |
88.22 ± 5.36 |
10 |
— |
— |
— |
— |
— |
— |
30a |
100 |
46.53 ± 2.55 |
— |
— |
— |
— |
— |
10 |
34.92 ± 6.84 |
— |
— |
— |
— |
— |
30b |
100 |
52.97 ± 8.35 |
— |
— |
23.00 ± 9.26 |
— |
— |
10 |
41.69 ± 10.35 |
— |
— |
— |
— |
— |
35b |
100 |
92.04 ± 1.18 |
— |
— |
— |
25.86 ± 1.43 |
— |
10 |
57.37 ± 6.13 |
— |
— |
— |
— |
— |
41 |
100 |
76.58 ± 11.68 |
— |
— |
— |
23.49 ± 7.28 |
— |
10 |
25.17 ± 6.00 |
— |
— |
— |
— |
— |
43a |
100 |
91.72 ± 3.98 |
— |
— |
30.58 ± 1.51 |
22.64 ± 6.99 |
— |
10 |
— |
— |
— |
— |
— |
— |
45a |
100 |
91.29 ± 1.86 |
— |
— |
23.37 ± 2.81 |
— |
— |
10 |
— |
— |
— |
— |
— |
— |
45b |
100 |
77.98 ± 6.27 |
— |
— |
— |
— |
— |
10 |
1.53 ± 2.93 |
— |
— |
— |
— |
— |
50b |
100 |
76.30 ± 16.90 |
— |
— |
— |
— |
— |
10 |
45.25 ± 11.25 |
— |
— |
— |
— |
— |
52a |
100 |
77.67 ± 3.81 |
73.44 ± 1.78 |
— |
— |
86.64 ± 2.54 |
84.92 ± 4.20 |
10 |
93.88 ± 1.77 |
— |
— |
— |
— |
— |
52b |
100 |
87.23 ± 4.17 |
68.03 ± 4.74 |
— |
— |
81.47 ± 5.04 |
81.00 ± 4.03 |
10 |
94.63 ± 1.01 |
— |
— |
— |
41.25 ± 9.35 |
— |
56a |
100 |
78.20 ± 7.98 |
— |
— |
— |
— |
— |
10 |
— |
— |
— |
— |
— |
— |
58a |
100 |
60.52 ± 2.49 |
— |
— |
26.09 ± 4.61 |
— |
— |
10 |
— |
— |
— |
— |
— |
— |
58b |
100 |
68.93 ± 6.85 |
— |
— |
— |
— |
— |
10 |
34.63 ± 7.99 |
— |
— |
— |
— |
— |
66a |
100 |
31.48 ± 11.69 |
— |
— |
— |
— |
39.76 ± 3.24 |
10 |
— |
— |
— |
— |
— |
— |
Our tests revealed that di-O-benzyl aminotriol derivatives (52a–b) possess potential antimicrobial properties over 80% against both the two Gram-positive and the yeast species. In the case of B. subtilis, these compounds proved to be the most effective agents even at a low concentration of 10 μg mL−1, while other derivatives (45a–b and 58a–b) showed lower activities. Removal of one of the two benzyl protecting groups in aminotriol derivatives (45a–b and 58a–b) led to improved selective inhibition on B. subtilis. The almost complete loss of antibacterial activity resulting from the replacement of all O-benzyloxy groups with hydroxyl group as demonstrated with aminotriol derivatives 66a–b suggests that the benzyl moiety is a key element to have satisfactory antimicrobial activity in the case of aminotriols.
Among aminodiol derivatives, only O-benzyl aminodiol 35b presented activity against B. subtilis, whereas debenzylated derivative 9 had no effect. This result indicates that the O-benzyloxy group attached to the cyclohexyl ring is responsible for activity of the studied antibacterial agents.
The available data demonstrated that the O-benzyloxy group on the cyclohexyl ring (41 and 50b) is much more effective to induce antimicrobial activity than the 1-BnO-propen-2-yl group (50a).
In comparison, α-methylene-γ-butyrolactone 4, the most effective compound against C. albicans and C. krusei, was found to possess highly selective effectiveness on the yeast species.
The synthetic aminodiol and aminotriol derivatives were also evaluated for their antioxidant activity using DPPH assays (Table 2, only the detected activities are shown).
Table 2 Antioxidant effects of active synthetic derivatives expressed in IC50 values
Compound |
DPPH antioxidant activity (μmol mL−1) ± SD |
9 |
8.47 ± 0.56 |
24b |
75.63 ± 0.01 |
28a |
204.77 ± 9.1 |
30a |
72.76 ± 0.03 |
45a |
87.61 ± 0.14 |
58a |
33.74 ± 3.74 |
58b |
56.63 ± 0.01 |
Gallic acid |
0.16 ± 0.01 |
In the DPPH study, aminodiol 9 displayed a potential antioxidant effect, while the aminotriol derivatives (58a–b) had only moderate effects. The results of this survey, namely improvement of antioxidant activity alongside with the replacement of the O-benzyloxy moiety with a hydroxyl group, show that efficiency depends on the hydroxyl function of the cyclohexyl ring more significantly than on the 1-hydroxypropen-2-yl group.
The hydroxyl group of molecules play remarkable role in their antioxidant property.92 Recently, there are two proposed mechanisms by which antioxidants containing hydroxyl group can act protectively. In the first mechanism, the free radical (e.g. DPPH) removes a hydrogen atom from the hydroxyl group that itself becomes a radical, in this way the functional group donates a proton to the free radicals and neutralise it (e.g. DPPH-H). In the second mechanism, called as one-electron transfer, the hydroxyl group can give an electron to the free radical becoming itself a radical cation.93
Although aminotriol 58a was less active than aminodiols, its antioxidant property is still considered to be notable compared with aminotriol 45a. This result again demonstrates that the hydroxyl group on the cyclohexyl ring is necessary for antioxidant property.
Conclusion
A new library of isopulegol-based chiral aminodiols and aminotriols was developed from commercially available (−)-isopulegol. The isopulegol-based chiral di-, tri- and tetraols are promising substrates for the preparation of chiral crown ethers. α,β-Dihydroxyamides, accessed through the ring opening of α,β-dihydroxylactones, are widely applicable in the synthesis of natural products and in saccharide chemistry.
Our result proved again that steric hindrance exerted by both benzyl and methyl groups at the α position in epoxide 34a makes its conformationally constrained structure to restrict the approach of nucleophiles in aminolysis.
O-Benzyl aminotriol and aminodiol derivatives exert markedly selective antibacterial action on B. subtilis, while di-O-benzyl aminotriols have also shown significant effectiveness not only against Gram-positive bacteria strains but also against yeast species. Moreover, our result also indicated the potential antifungal activity of α-methylene-γ-butyrolactones.
In addition, aminodiol and aminotriol derivatives were applied as antioxidant agents in DPPH assay. N-Benzyl aminodiols are still considered to exert notable antioxidant property.
Finally, in vitro studies have clearly shown that the O-benzyl substituent on the cyclohexyl ring in aminodiol and aminotriol derivatives is essential to have antimicrobial effect, whereas the hydroxyl group on this ring is crucial on the antioxidant property. The stereochemistry of the aminotriol and aminotriol derivatives has no influence on either effect.
Conflicts of interest
The authors declare no conflict of interest.
Acknowledgements
We are grateful for financial supports from the EU-funded Hungarian grant GINOP-2.3.2-15-2016-00012, Ministry of Human Capacities, Hungary grant 20391-3/2018/FEKUSTRAT, Hungarian Research Foundation (OTKA No. K 115731), University of Szeged Open Access Fund (grant No. 4969) and Tamás Szilasi for his experimental assistance.
References
- L. M. Ciesla, K. A. Wojtunik-Kulesza, A. Oniszczuk and M. Waksmundzka-Hajnos, Antioxidant synergism and antagonism between selected monoterpenes using the 2,2-diphenyl-1-picrylhydrazyl method: Antioxidant synergism and antagonism between selected monoterpenes, Flavour Fragrance J., 2016, 31, 412–419 CrossRef CAS.
- Y. Wen Xu, Effects of salicylic acid on monoterpene production and antioxidant systems in Houttuynia cordata, Afr. J. Biotechnol., 2012, 11, 1364–1372 Search PubMed.
- F. Bakkali, S. Averbeck, D. Averbeck and M. Idaomar, Biological effects of essential oils – A review, Food Chem. Toxicol., 2008, 46, 446–475 CrossRef CAS.
- N. L. S. Chan, H. Wang, Y. Wang, H. Y. Leung and L. K. Leung, Polycyclic aromatic hydrocarbon-induced CYP1B1 activity is suppressed by perillyl alcohol in MCF-7 cells, Toxicol. Appl. Pharmacol., 2006, 213, 98–104 CrossRef CAS.
- P. L. Crowell, Monoterpenes in breast cancer chemoprevention, Breast Cancer Res. Treat., 1997, 46, 191–197 CrossRef CAS.
- M. N. Gould, Prevention and therapy of mammary cancer by monoterpenes, J. Cell. Biochem., 1995, 59, 139–144 CrossRef.
- B. Rodenak Kladniew, M. Polo, S. Montero Villegas, M. Galle, R. Crespo and M. García de Bravo, Synergistic antiproliferative and anticholesterogenic effects of linalool, 1,8-cineole, and simvastatin on human cell lines, Chem.-Biol. Interact., 2014, 214, 57–68 CrossRef CAS.
- R. Tundis, M. R. Loizzo, M. Bonesi, F. Menichini, V. Mastellone, C. Colica and F. Menichini, Comparative study on the antioxidant capacity and Cholinesterase inhibitory activity of Citrus aurantifolia Swingle, C. aurantium L., and C. bergamia Risso and Poit. Peel Essential Oils, J. Food Sci., 2012, 77, H40–H46 CrossRef CAS.
- G. Ruberto and M. T. Baratta, Antioxidant activity of selected essential oil components in two lipid model systems, Food Chem., 2000, 69, 167–174 CrossRef CAS.
- M. Nikolić, J. Glamočlija, I. C. F. R. Ferreira, R. C. Calhelha, Â. Fernandes, T. Marković, D. Marković, A. Giweli and M. Soković, Chemical composition, antimicrobial, antioxidant and antitumor activity of Thymus serpyllum L., Thymus algeriensis Boiss. and Reut and Thymus vulgaris L. essential oils, Ind. Crops Prod., 2014, 52, 183–190 CrossRef.
- K. A. Wojtunik, L. M. Ciesla and M. Waksmundzka-Hajnos, Model studies on the antioxidant activity of common terpenoid constituents of essential oils by means of the 2,2-diphenyl-1-picrylhydrazyl method, J. Agric. Food Chem., 2014, 62, 9088–9094 CrossRef CAS.
- A. Ahmad, S. van Vuuren and A. Viljoen, Unravelling the complex antimicrobial interactions of essential oils -The case of Thymus vulgaris (Thyme), Molecules, 2014, 19, 2896–2910 CrossRef.
- A. C. R. da Silva, P. M. Lopes, M. M. B. de Azevedo, D. C. M. Costa, C. S. Alviano and D. S. Alviano, Biological activities of α-Pinene and β-Pinene enantiomers, Molecules, 2012, 17, 6305–6316 CrossRef CAS.
- J. Nguefack, O. Tamgue, J. B. L. Dongmo, C. D. Dakole, V. Leth, H. F. Vismer, P. H. Amvam Zollo and A. E. Nkengfack, Synergistic action between fractions of essential oils from Cymbopogon citratus, Ocimum gratissimum and Thymus vulgaris against Penicillium expansum, Food Control, 2012, 23, 377–383 CrossRef CAS.
- S.-N. Park, Y. K. Lim, M. O. Freire, E. Cho, D. Jin and J.-K. Kook, Antimicrobial effect of linalool and α-terpineol against periodontopathic and cariogenic bacteria, Anaerobe, 2012, 18, 369–372 CrossRef CAS.
- N. Belletti, S. S. Kamdem, G. Tabanelli, R. Lanciotti and F. Gardini, Modeling of combined effects of citral, linalool and β-pinene used against Saccharomyces cerevisiae in citrus-based beverages subjected to a mild heat treatment, Int. J. Food Microbiol., 2010, 136, 283–289 CAS.
- D. Trombetta, F. Castelli, M. G. Sarpietro, V. Venuti, M. Cristani, C. Daniele, A. Saija, G. Mazzanti and G. Bisignano, Mechanisms of antibacterial action of three monoterpenes, Antimicrob. Agents Chemother., 2005, 49, 2474–2478 CAS.
- M. Cristani, M. D'Arrigo, G. Mandalari, F. Castelli, M. G. Sarpietro, D. Micieli, V. Venuti, G. Bisignano, A. Saija and D. Trombetta, Interaction of four monoterpenes contained in essential oils with model membranes: implications for their antibacterial activity, J. Agric. Food Chem., 2007, 55, 6300–6308 CrossRef CAS.
- W. T. Langeveld, E. J. A. Veldhuizen and S. A. Burt, Synergy between essential oil components and antibiotics: a review, Crit. Rev. Microbiol., 2014, 40, 76–94 CrossRef CAS.
- V. Pereira, C. Dias, M. C. Vasconcelos, E. Rosa and M. J. Saavedra, Antibacterial activity and synergistic effects between Eucalyptus globulus leaf residues (essential oils and extracts) and antibiotics against several isolates of respiratory tract infections (Pseudomonas aeruginosa), Ind. Crops Prod., 2014, 52, 1–7 CrossRef CAS.
- N. G. Ntalli, F. Ferrari, I. Giannakou and U. Menkissoglu-Spiroudi, Synergistic and antagonistic interactions of terpenes against Meloidogyne incognita and the nematicidal activity of essential oils from seven plants indigenous to Greece, Pest Manag. Sci., 2011, 67, 341–351 CrossRef CAS.
- K. R. Riella, R. R. Marinho, J. S. Santos, R. N. Pereira-Filho, J. C. Cardoso, R. L. C. Albuquerque-Junior and S. M. Thomazzi, Anti-inflammatory and cicatrizing activities of thymol, a monoterpene of the essential oil from Lippia gracilis, in rodents, J. Ethnopharmacol., 2012, 143, 656–663 CrossRef CAS.
- A. Djouahri, B. Saka, L. Boudarene, F. Benseradj, S. Aberrane, S. Aitmoussa, C. Chelghoum, L. Lamari, N. Sabaou and A. Baaliouamer, In vitro synergistic/antagonistic antibacterial and anti-inflammatory effect of various extracts/essential oil from cones of Tetraclinis articulata (Vahl) Masters with antibiotic and anti-inflammatory agents, Ind. Crops Prod., 2014, 56, 60–66 CrossRef CAS.
- A. G. Guimarães, J. S. S. Quintans and L. J. Quintans-Júnior, Monoterpenes with analgesic activity-A systematic review, Phytother. Res., 2013, 27, 1–15 CrossRef.
- A. Aharoni, M. Jongsma and H. Bouwmeester, Volatile science? Metabolic engineering of terpenoids in plants, Trends Plant Sci., 2005, 10, 594–602 CrossRef CAS.
- H. T. Prates, J. P. Santos, J. M. Waquil, J. D. Fabris, A. B. Oliveira and J. E. Foster, Insecticidal activity of monoterpenes against Rhyzopertha dominica (F.) and Tribolium castaneum (Herbst), J. Stored Prod. Res., 1998, 34, 243–249 CrossRef CAS.
- W.-S. Choi, B.-S. Park, Y.-H. Lee, D. Y. Jang, H. Y. Yoon and S.-E. Lee, Fumigant toxicities of essential oils and monoterpenes against Lycoriella mali adults, Crop Prot., 2006, 25, 398–401 CAS.
- B. A. Leita, A. C. Warden, N. Burke, M. S. O'Shea and D. Trimm, Production of p-Cymene and hydrogen from a bio-renewable feedstock–1,8-cineole (Eucalyptus oil), Green Chem., 2010, 12, 70–76 RSC.
- W. Chen and A. M. Viljoen, Geraniol — A review of a commercially important fragrance material, South Afr. J. Bot., 2010, 76, 643–651 CrossRef CAS.
- G. P. P. Kamatou, I. Vermaak, A. M. Viljoen and B. M. Lawrence, Menthol: A simple monoterpene with remarkable biological properties, Phytochemistry, 2013, 96, 15–25 CrossRef CAS.
- A. E. Wróblewski, I. E. Głowacka and D. G. Piotrowska, 1′-Homonucleosides and their structural analogues: A review, Eur. J. Med. Chem., 2016, 118, 121–142 CrossRef.
- J. M. Sadler, S. L. Mosley, K. M. Dorgan, Z. S. Zhou and K. L. Seley-Radtke, Synthetic strategies toward carbocyclic purine–pyrimidine hybrid nucleosides, Bioorg. Med. Chem., 2009, 17, 5520–5525 CrossRef CAS.
- K. A. Jacobson, D. K. Tosh, K. S. Toti and A. Ciancetta, Polypharmacology of conformationally locked methanocarba nucleosides, Drug Discovery Today, 2017, 22, 1782–1791 CrossRef CAS.
- A. C. Allepuz, R. Badorrey, M. D. Díaz-de-Villegas and J. A. Gálvez, Diastereoselective reduction of ketimines derived from (R)-3,4-dihydroxybutan-2-one: an alternative route to key intermediates for the synthesis of anticancer agent ES-285, Tetrahedron: Asymmetry, 2010, 21, 503–506 CrossRef CAS.
- R. K. Mishra, C. M. Coates, K. D. Revell and E. Turos, Synthesis of 2-Oxazolidinones from β-Lactams: stereospecific total synthesis of (−)-Cytoxazone and all of Its stereoisomers, Org. Lett., 2007, 9, 575–578 CrossRef CAS.
- A. Grajewska and M. D. Rozwadowska, Stereoselective synthesis of cytoxazone and its analogues, Tetrahedron: Asymmetry, 2007, 18, 803–813 CrossRef CAS.
- H. Kleinert, S. Rosenberg, W. Baker, H. Stein, V. Klinghofer, J. Barlow, K. Spina, J. Polakowski, P. Kovar and J. Cohen, et al., Discovery of a peptide-based renin inhibitor with oral bioavailability and efficacy, Science, 1992, 257, 1940–1943 CrossRef CAS.
- S. Chandrasekhar, S. Mohapatra and J. S. Yadav, Practical synthesis of Abbott aminodiol: A core unit of the potent renin inhibitor Zankiren, Tetrahedron, 1999, 55, 4763–4768 CrossRef CAS.
- K. Toribatake, S. Miyata, Y. Naganawa and H. Nishiyama, Asymmetric synthesis of optically active 3-amino-1,2-diols from N-acyl-protected allylamines via catalytic diboration with Rh[bis(oxazolinyl)phenyl] catalysts, Tetrahedron, 2015, 71, 3203–3208 CrossRef CAS.
- E. Richelle-Maurer, J.-C. Braekman, M. Kluijver, R. Gomez, G. de Vyver, R. Soest and C. Devijver, Cellular location of (2R,3R,7Z)-2-aminotetradec-7-ene-1, 3-diol, a potent antimicrobial metabolite produced by the Caribbean sponge Haliclona vansoesti, Cell Tissue Res., 2001, 306, 157–165 CrossRef CAS.
- H. Kakeya, M. Morishita, H. Koshino, T. Morita, K. Kobayashi and H. Osada, Cytoxazone: A novel Cytokine modulator containing a 2-Oxazolidinone ring produced by Streptomyces sp., J. Org. Chem., 1999, 64, 1052–1053 CrossRef CAS.
- A. S. Paraskar and A. Sudalai, Enantioselective synthesis of (−)-Cytoxazone and (+)-epi-Cytoxazone, novel cytokine modulators via Sharpless asymmetric epoxidation and l-proline catalyzed Mannich reaction, Tetrahedron, 2006, 62, 5756–5762 CrossRef CAS.
- R. Pedrosa, C. Andrés, J. P. Duque-Soladana and C. D. Rosón, Regio- and stereoselective 6- exo - trig radical cyclisations onto chiral perhydro-1,3-benzoxazines: synthesis of enantiopure 3-alkylpiperidines, Tetrahedron: Asymmetry, 2000, 11, 2809–2821 CrossRef CAS.
- R. Pedrosa, C. Andrés, J. Nieto and S. del Pozo, Synthesis of enantiopure 3-Azabicyclo[3.2.0]heptanes by diastereoselective intramolecular [2+2] photocycloaddition reactions on chiral perhydro-1,3-benzoxazines, J. Org. Chem., 2003, 68, 4923–4931 CrossRef CAS.
- C. Andrés, J. Nieto, R. Pedrosa and N. Villamañán, Synthesis of enantiopure primary amines by stereoselective ring opening of chiral Octahydro-1,3-benzoxazines by Grignard and Organoaluminum reagents, J. Org. Chem., 1996, 61, 4130–4135 CrossRef.
- A. Alberola, C. Andrés and R. Pedrosa, Diastereoselective ring opening of 2-substituted N-Benzyl-4,4, 7α-trimethyl- trans-octahydro-1,3-benzoxazines by Grignard reagents. Highly enantioselective synthesis of primary amines, Synlett, 1990, 1990, 763–765 CrossRef.
- Y.-J. Cherng, J.-M. Fang and T.-J. Lu, Pinane-type tridentate reagents for enantioselective reactions: reduction of ketones and addition of Diethylzinc to aldehydes, J. Org. Chem., 1999, 64, 3207–3212 CrossRef CAS.
- Y.-J. Cherng, J.-M. Fang and T.-J. Lu, A new pinane-type tridentate modifier for asymmetric reduction of ketones with lithium aluminum hydride, Tetrahedron: Asymmetry, 1995, 6, 89–92 CrossRef CAS.
- Z. Szakonyi, A. Hetényi and F. Fülöp, Synthesis of enantiomeric spirooxazolines and spirooxazolidines by the regioselective ring closure of (–)-α-pinene-based aminodiols, Arkivoc, 2007, 2008, 33 Search PubMed.
- Z. Szakonyi, K. Csillag and F. Fülöp, Stereoselective synthesis of carane-based aminodiols as chiral ligands for the catalytic addition of diethylzinc to aldehydes, Tetrahedron: Asymmetry, 2011, 22, 1021–1027 CrossRef CAS.
- Z. Szakonyi, Á. Csőr, A. Csámpai and F. Fülöp, Stereoselective synthesis and modelling-driven optimisation of Carane-based aminodiols and 1,3-Oxazines as catalysts for the enantioselective addition of diethylzinc to benzaldehyde, Chem.–Eur. J., 2016, 22, 7163–7173 CrossRef CAS.
- M. P. Stoyanova, B. L. Shivachev, R. P. Nikolova and V. Dimitrov, Highly efficient synthesis of chiral aminoalcohols and aminodiols with camphane skeleton, Tetrahedron: Asymmetry, 2013, 24, 1426–1434 CrossRef CAS.
- D.-S. Lee, S.-M. Chang, C.-Y. Ho and T.-J. Lu, Enantioselective addition of diethylzinc to aldehydes catalyzed by chiral O, N, O-tridentate phenol ligands derived from camphor, Chirality, 2016, 28, 65–71 CrossRef CAS.
- I. Philipova, V. Dimitrov and S. Simova, Synthesis of new enantiopure aminodiols and their use as ligands for the addition of diethylzinc to benzaldehyde, Tetrahedron: Asymmetry, 1999, 10, 1381–1391 CrossRef CAS.
- S. Panev, A. Linden and V. Dimitrov, Chiral aminoalcohols with a menthane skeleton as catalysts for the enantioselective addition of diethylzinc to benzaldehyde, Tetrahedron: Asymmetry, 2001, 12, 1313–1321 CrossRef CAS.
- K. Csillag, L. Németh, T. A. Martinek, Z. Szakonyi and F. Fülöp, Stereoselective synthesis of pinane-type tridentate aminodiols and their application in the enantioselective addition of diethylzinc to benzaldehyde, Tetrahedron: Asymmetry, 2012, 23, 144–150 CrossRef CAS.
- Y.-J. Cherng, J.-M. Fang and T.-J. Lu, Pinane-type tridentate reagents for enantioselective reactions: reduction of ketones and addition of diethylzinc to aldehydes, J. Org. Chem., 1999, 64, 3207–3212 CrossRef CAS.
- T. Le, P. Bérdi, I. Zupkó, F. Fülöp and Z. Szakonyi, Synthesis and transformation of (-)-Isopulegol-based chiral β-aminolactones and β-aminoamides, Int. J. Mol. Sci., 2018, 19, 3522 CrossRef.
- F. Z. Bamou, T. M. Le, B. Volford, A. Szekeres and Z. Szakonyi, Synthesis and application of 1,2-aminoalcohols with neoisopulegol-based octahydrobenzofuran core, Molecules, 2019, 25, 21 CrossRef.
- R. Outouch, S. Oubaassine, M. Ait Ali, L. El Firdoussi and A. Spannenberg, Crystal structure of (1S ,2R,4 S)-1-[(morpholin-4-yl)methyl]-4-(prop-1-en-2-yl)cyclohexane-1,2-diol, Acta Crystallogr., Sect. E: Crystallogr. Commun., 2015, 71, 79–81 CrossRef CAS.
- T. Gonda, Z. Szakonyi, A. Csámpai, M. Haukka and F. Fülöp, Stereoselective synthesis and application of tridentate aminodiols derived from (+)-pulegone, Tetrahedron: Asymmetry, 2016, 27, 480–486 CrossRef CAS.
- Y. Tashenov, M. Daniels, K. Robeyns, L. Van Meervelt, W. Dehaen, Y. Suleimen and Z. Szakonyi, Stereoselective syntheses and application of chiral bi- and tridentate ligands derived from (+)-Sabinol, Molecules, 2018, 23, 771 CrossRef.
- O. V. Ardashov, A. V. Pavlova, I. V. Il’ina, E. A. Morozova, D. V. Korchagina, E. V. Karpova, K. P. Volcho, T. G. Tolstikova and N. F. Salakhutdinov, Highly Potent Activity of (1R,2R,6S)-3-Methyl-6-(prop-1-en-2-yl)cyclohex-3-ene-1,2-diol in animal models of Parkinson's disease, J. Med. Chem., 2011, 54, 3866–3874 CrossRef CAS.
- D. A. Brown, M. T. Canning, S. L. Nay, A. V. Pena and D. B. Yarosh, Bicyclic monoterpene diols stimulate release of nitric oxide from skin cells, increase microcirculation, and elevate skin temperature, Nitric Oxide, 2006, 15, 70–76 CrossRef CAS.
- D. A. Brown, K. Lesiak, W.-Y. Ren, K. L. Strzelecki and A. A. Khorlin, Bicyclic monoterpene diols induce differentiation of S91 melanoma and PC 12 pheochromocytoma cells by a cyclic Guanosine-monophosphate-dependent pathway, Pigm. Cell Res., 1999, 12, 36–47 CrossRef CAS.
- S. Hammami, A. I. Elshamy, R. E. Mokni, A. Snene, K. Iseki, H. Dhaouadi, Y. Okamoto, M. Suenaga, M. Noji, A. Umeyama and Y. Asakawa, Chemical Constituents of the Aerial Parts of Daucus carota subsp. hispidus growing in Tunisia, Nat. Prod. Commun., 2019, 14, 1934578X1986351 CrossRef.
- P. L. Crowell, Z. Ren, S. Lin, E. Vedejs and M. N. Gould, Structure-activity relationships among monoterpene inhibitors of protein isoprenylation and cell proliferation, Biochem. Pharmacol., 1994, 47, 1405–1415 CrossRef CAS.
- A. E.-H. H. Mohamed, N. S. Mohamed, A. R. Hamed and M.-E. F. Hegazy, Anti-inflammatory activity of highly oxygenated terpenoids from Achillea biebersteinii Afan, Z. Naturforsch. C Biosci., 2016, 71, 429–432 CAS.
- T. J. Brocksom, R. B. dos Santos, N. A. Varanda and U. Brocksom, An efficient synthesis of monoterpene α-methylene-γ-butyrolactones, Synth. Commun., 1988, 18, 1403–1410 CrossRef CAS.
- T. J. Brocksom, J. Tercio and B. Ferreira, A biomimetic synthesis of α-methylene-γ-butyrolactones, Synth. Commun., 1981, 11, 105–119 CrossRef CAS.
- M. Carda and J. A. Marco, Total synthesis of the monoterpenes (−)-mintlactone and (+)-isomintlactone, Tetrahedron, 1992, 48, 9789–9800 CrossRef CAS.
- D. Friedrich and F. Bohlmann, Total synthesis of various elemanolides, Tetrahedron, 1988, 44, 1369–1392 CrossRef CAS.
- M. Schlosser and M. Kotthaus, Isopulegol as a model compound: metalation and substitution of an allylic position in the presence of an unprotected hydroxy function, Eur. J. Org. Chem., 1999, 1999, 459–462 CrossRef.
- S. Serra and C. Fuganti, Enzyme-mediated preparation of enantiomerically pure p-Menthan- 3,9-diols and their use for the synthesis of natural p-menthane lactones and ethers, Helv. Chim. Acta, 2002, 85, 2489–2502 CrossRef CAS.
- N. J. Lawrence, A. T. McGown, J. Nduka, J. A. Hadfield and R. G. Pritchard, Cytotoxic michael-type amine adducts of α-methylene lactones alantolactone and isoalantolactone, Bioorg. Med. Chem. Lett., 2001, 11, 429–431 CrossRef CAS.
- Z. Szakonyi, A. Hetényi and F. Fülöp, Synthesis and application of monoterpene-based chiral aminodiols, Tetrahedron, 2008, 64, 1034–1039 CrossRef CAS.
- D. Wu, J. Li and W. Wang, Selective formation of monoacylated diols through a mild Passerini reaction: selective formation of monoacylated diols through a mild Passerini reaction, Eur. J. Org. Chem., 2018, 2018, 3022–3030 CrossRef CAS.
- A. Nemoto, Y. Hoshino, K. Yazawa, A. Ando, Y. Mikami, H. Komaki, Y. Tanaka and U. Gräfe, Asterobactin, a new Siderophore group antibiotic from Nocardia asteroides, J. Antibiot., 2002, 55, 593–597 CrossRef CAS.
- D. Schummer, G. Höfle, E. Forche, H. Reichenbach, V. Wray and T. Domke, Antibiotics from Gliding bacteria, LXXVI. Vioprolides: new antifungal and cytotoxic peptolides from Cystobacter violaceus, Liebigs Ann., 2006, 1996, 971–978 CrossRef.
- J. H. Kim, H. J. Lim and S. H. Cheon, A facile synthesis of (6S,1′S)-(+)-hernandulcin and (6S,1′R)-(+)-epihernandulcin, Tetrahedron, 2003, 59, 7501–7507 CrossRef CAS.
- B. P. Shivani and A. K. Chakraborti, Zinc(II) perchlorate hexahydrate catalyzed opening of epoxide ring by amines: applications to synthesis of (RS)/(R)-Propranolols and (RS)/(R)/(S)-Naftopidils, J. Org. Chem., 2007, 72, 3713–3722 CrossRef CAS.
- S. C. Bergmeier, The Synthesis of vicinal amino alcohols, Tetrahedron, 2000, 56, 2561–2576 CrossRef CAS.
- Y. Yuasa and Y. Yuasa, Synthesis and absolute configuration at C(8) of ‘p-Menthane-3,8,9-triol’ derived from (-)-Isopulegol, Helv. Chim. Acta, 2004, 87, 2602–2607 CrossRef CAS.
- B. R. Travis, R. S. Narayan and B. Borhan, Osmium tetroxide-promoted catalytic oxidative cleavage of olefins: An
organometallic ozonolysis, J. Am. Chem. Soc., 2002, 124, 3824–3825 CrossRef CAS.
- G. N. Costa, R. M. B. Carrilho, L. D. Dias, J. C. Viana, G. L. B. Aquino, M. Pineiro and M. M. Pereira, Highly efficient Rh(I)/tris-binaphthyl monophosphite catalysts for hydroformylation of sterically hindered alkyl olefins, J. Mol. Catal. A: Chem., 2016, 416, 73–80 CrossRef CAS.
- I. Paterson, E. A. Anderson, S. M. Dalby, J. H. Lim and P. Maltas, The stereocontrolled total synthesis of spirastrellolide A methyl ester. Fragment coupling studies and completion of the synthesis, Org. Biomol. Chem., 2012, 10, 5873–5886 RSC.
- S. Superchi, M. I. Donnoli, G. Proni, G. P. Spada and C. Rosini, Induction of cholesteric mesophases by simple cyclic derivatives of p,p′-disubstituted 1,2-Diphenylethane-1,2-diols: importance of shape and polarizability effects, J. Org. Chem., 1999, 64, 4762–4767 CrossRef CAS.
- I. S. Marcos, L. Castañeda, P. Basabe, D. Díez and J. G. Urones, Synthesis of sibiricinone A, sibiricinone B and leoheterin, Tetrahedron, 2008, 64, 10860–10866 CrossRef CAS.
- P. A. Wender, N. Buschmann, N. B. Cardin, L. R. Jones, C. Kan, J.-M. Kee, J. A. Kowalski and K. E. Longcore, Gateway synthesis of daphnane congeners and their protein kinase C affinities and cell-growth activities, Nat. Chem., 2011, 3, 615–619 CrossRef CAS.
- K. Chen and P. S. Baran, Total synthesis of eudesmane terpenes by site-selective C–H oxidations, Nature, 2009, 459, 824–828 CrossRef CAS.
- T. M. Le, T. Szilasi, B. Volford, A. Szekeres, F. Fülöp and Z. Szakonyi, Stereoselective synthesis and investigation of Isopulegol-based chiral ligands, Int. J. Mol. Sci., 2019, 20, 4050 CrossRef CAS.
- W. Brand-Williams, M. E. Cuvelier and C. Berset, Use of a free radical method to evaluate antioxidant activity, LWT--Food Sci. Technol., 1995, 28, 25–30 CrossRef CAS.
- E. Bendary, R. R. Francis, H. M. G. Ali, M. I. Sarwat and S. El Hady, Antioxidant and structure–activity relationships (SARs) of some phenolic and anilines compounds, Ann. Agric. Sci., 2013, 58, 173–181 CrossRef.
Footnote |
† Electronic supplementary information (ESI) available. See DOI: 10.1039/d0ra07739a |
|
This journal is © The Royal Society of Chemistry 2020 |
Click here to see how this site uses Cookies. View our privacy policy here.