DOI:
10.1039/D0RA07698H
(Paper)
RSC Adv., 2020,
10, 38478-38483
Highly enantioselective copper-catalyzed propargylic amination to access N-tethered 1,6-enynes†
Received
8th September 2020
, Accepted 12th October 2020
First published on 20th October 2020
Abstract
A highly enantioselective copper-catalyzed propargylic amination starting from benzylic allylic amines has been developed with a new chiral N,N,P ligand. A series of N-tethered 1,6-enynes were synthesized in good to excellent yields with excellent enantioselectivities. Utilization of transition metal-catalyzed cycloisomerization of 1,6-enynes provides several enantioselectively enriched chiral five-membered N-heterocycles efficiently.
The N-tethered 1,6-enyne skeleton is a highly versatile motif which plays a key role in organic synthesis.1 In particular, N-tethered 1,6-enynes are key synthetic precursors of transition metal catalyzed cycloisomerizations, providing diversity of nitrogen-containing heterocycles (N-heterocycles) efficiently.2 In the last decades, lots of effort has been dedicated to developing enantioselective methods to synthesize N-tethered 1,6-enynes.3,4 Among those methods, through C–N bond formation of allylic amines and alkynes was regarded as a promising approach. For examples, addition of alkynylides to N-allyliminium intermediates generated in situ could yield N-tethered 1,6-enynes.3b,c Using this strategy, Knochel and coworkers disclosed a copper-catalyzed three component reaction for the preparation of N-tethered 1,6-enynes with moderate ees (Scheme 1a).3b However, this reaction is limited to the synthesis of internal alkynes. In 2010, the Nishibayashi group reported a high enantioselective copper-catalyzed asymmetric propargylic amination, giving the desired product in 87% ee, but only one example was studied (Scheme 1b).5 Thus, a general and practical method to synthesize N-tethered 1,6-enynes in high enantioselectivities is highly desirable.
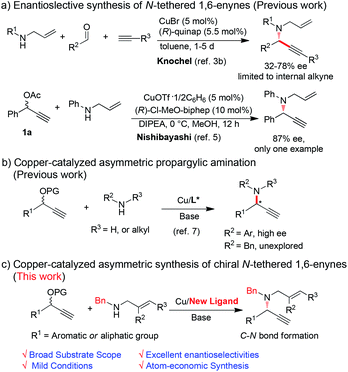 |
| Scheme 1 Enantioselective synthesis of N-tethered 1,6-enynes. | |
Copper-catalyzed asymmetric propargylic amination of propargylic esters and amines is a powerful method to construct C–N bond for the preparation of propargylic amines.6 Nishibayashi, van Maarseveen and Hu et al. achieved several pioneering works by their asymmetric catalytic systems.7 However, those systems are still suffering from low efficiency and limited substrates scope. For instance, both primary and second amines bearing aryl substituted groups were suitable substrates for obtaining excellent ees. However, aryl groups are difficult to remove, thus obstacling its application in organic synthesis. Copper-catalyzed asymmetric propargylic amination of propargylic esters with benzylic amines has not been investigated, probably attributing to their stronger basicity and flexible configuration. It has been well known that benzylic amines not only play important roles in organic synthesis but also are core structures of many pharmaceuticals and bioactive compounds.8 Therefore, devising for the asymmetric propargylic amination from benzylic amines are very important, and remains a challenging task.
Considering the important role of N-tethered 1,6-enynes in organic synthesis and our continuing effort in propargylic substitutions,9 we developed a new catalyst system to realize copper-catalyzed asymmetric propargylic aminations efficiently and mildly. Diverse N-tethered 1,6-enynes could be obtained in excellent enantioselectivities. Furthermore, several N-heterocycles could be synthesized by the transition metal promoted cycloisomerization of thus obtained 1,6-enynes.
We began our investigation by using the phenyl-2-propynyl acetate 1a in combination with N-allylbenzylamine 2a as model substrates (Table 1). Examination of the influence of chiral ligands showed that Ph-PyBOX (L1) could catalyze the reaction smoothly at room temperature, giving the target product 3a in 81% yield with 50% ee (entry 1). To improve the enantioselectivity of this transformation, we checked different analogs of the PyBOX, but all the ligands gave poor results (entries 2–4). When using chiral diphosphine ligands such as Cl–MeO-BIHPEP (L5), no product was obtained (entry 5). To our delight, improved ee of 82% was obtained by using tridentate ligand L6 developed by Hu et al. (entry 6).10 We then prepared a novel ligand L7 bearing two pyridyl group. This ligand gave an even higher ee (86%) (entry 7). When using Cu(OAc)2·H2O as the catalyst instead of CuI, we obtained the product in 87% yield with 93% ee (entry 8). Optimized conditions were finally established by lowering the temperature to −20 °C, the reaction was completed in three hours even with 5 mol% of catalyst loading (entry 9).
Table 1 Optimization of the reaction conditiona
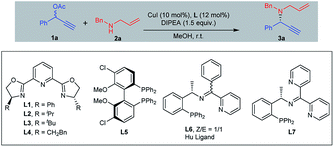
|
Entry |
L |
t (h) |
Yieldb (%) |
eec (%) |
Reaction conditions: 1a (0.2 mmol), 2a (0.3 mmol), MeOH (0.1 M), DIPEA (1.5 equiv.), CuI (10 mol%), L (12 mol%). Isolated yield after flash chromatography. The ee value was determined by HPLC analysis on a chiral stationary phase. Cu(OAc)2·H2O was used instead of CuI. Cu(OAc)2·H2O (5 mol%), L7 (6 mol%), −20 °C. DIPEA = diisopropylethylamine. |
1 |
L1 |
2 |
81 |
50 |
2 |
L2 |
2 |
82 |
25 |
3 |
L3 |
2 |
15 |
19 |
4 |
L4 |
2 |
82 |
21 |
5 |
L5 |
2 |
<5 |
— |
6 |
L6 |
2 |
78 |
82 |
7 |
L7 |
2 |
83 |
86 |
8d |
L7 |
2 |
87 |
93 |
9e |
L7 |
3 |
90 |
97 |
The scope of the reaction with respect to the propargylic esters was then investigated under the optimal conditions. By introducing electron-withdrawing or -donating groups at the para-position of the phenyl group, the reaction delivered the products 3b–3g with 90–99% ee values. Different substitutions on the meta- and ortho-position were also proved compatible for this reaction, again, the corresponding products 3h–3l were obtained with 95–99% ee. Notably, hetero-aromatic esters served as suitable substrates (3m–3n). To our delight, aliphatic-substituted propargylic substrates reacted smoothly with allylic amine 2a by using perfluorobenzoyl instead of acetate as the protecting group of propargylic alcohols.11 Several secondary propargylic esters worked well, providing the desired products 3o–3s in excellent ees. The chain length (from one to three) did not have much influence on the enantioselectivities. This work is different from Zhang's work reported recently, which at least two carbons aliphatic chain is necessary to obtain high enantioselectivities.11b Pleasingly, the reaction exhibited high functional-group tolerance. The propargylic esters bearing alkene, ether and thioether moieties underwent the reaction smoothly (3t–3w). Furthermore, the reaction also worked well with steric hindrance propargylic esters, giving the products 3x and 3y in good yield with 92–97% ee (Scheme 2).
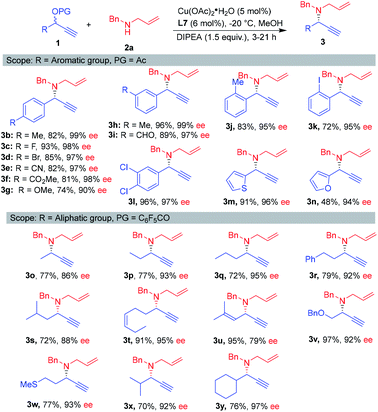 |
| Scheme 2 Scope of propargylic esters. aReaction conditions: 1 (0.2 mmol), 2a (0.3 mmol), MeOH (0.1 M), DIPEA (1.5 equiv.), Cu(OAc)2·H2O (5 mol%), L7 (6 mol%), −20 °C. | |
The scope of benzylic allylic amines was examined next (Scheme 3). Diversity of functional groups on the allylic amines such as methyl, iodine, hydroxyl, phenyl and ester groups were well tolerated, delivering the corresponding products 4a–4f in 49–97% yield with 91–99% ee. Heterocycle substituents such as 2-furyl and 2-thienyl have no significant effect on the reaction course, and the amination products 4g–4h were obtained in good yields and excellent enantioselectivities. Aromatic secondary amine was compatible for the reaction, giving propargylic amination product 4i in 82% yield with 94% ee.12 It seemed that the size of the substitutions on allylic amines did not affect the efficiency of this reaction. Different size groups such as methyl, allyl and tert-butyl were compatible for the reaction, providing the desired products 4j–4l in 60–74% yield with 95–98% ee. Interestingly, 3-pyrrodine also proved as a suitable substrate and 1,6-enyne 4m was obtained in 71% yield with 84% ee.
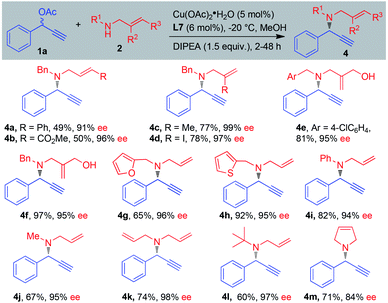 |
| Scheme 3 Scope of benzylic allylic amines. aReaction conditions: 1a (0.2 mmol), 2 (0.3 mmol), MeOH (0.1 M), DIPEA (1.5 equiv.), Cu(OAc)2·H2O (5 mol%), L7 (6 mol%), −20 °C. | |
The chiral N,N,P ligand L7 could be prepared by condensation of commercial available chiral amine 5 and di-2-pyridyl ketone 6 in 66% yield in one step.10 The tridentate coordination mode of L7 with Cu(I) was unambiguously confirmed by X-ray analysis of CuCl/L7 complexes (Scheme 4).13
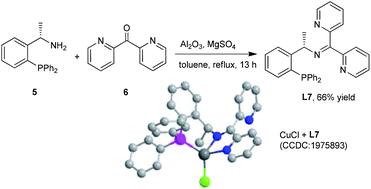 |
| Scheme 4 Synthesis of N,N,P ligand and the complexes of CuCl and L7. | |
Based on the previous literatures,5,7c,i we proposed the possible mechanism of the reaction (Scheme 5). In the first step, the copper complex forms π complex A with substrate 1a. Deprotonation with DIPEA gives the copper acetylide B. This intermediate loses the acetate group forms Cu–allenylidene complex C, where the intermediate D bearing a cationic γ-carbon exists as a resonance structure of C. Subsequently, the amine attacks the copper–allenylidene complex C, followed by a hydrogen atom shift, gives a Cu–π-alkyne complex E. After the ligand exchange, the product is released, completing the catalytic cycle.
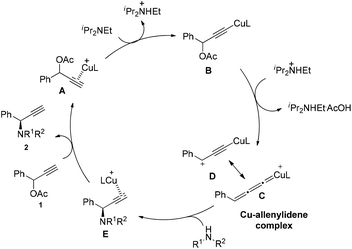 |
| Scheme 5 Proposed reaction mechanism. | |
The significant interest in chiral 1,6-enynes is based on their ability to be readily converted into enantiomerically enriched cyclic compounds. To illustrate the utility of our products, we prepared four different highly substituted scaffolds (Scheme 6). Importantly, bicyclic and polycyclic products were obtained efficiently. By means of enyne metathesis of 3a by Grubbs 1st generation catalyst afforded 2,5-dihydro-1H-pyrrole 7 in 71% yield.14 A novel polycyclic pyrrole 8 was synthesized by Ir-catalyzed cycloisomerization/Diels–Alder reaction/dehydrogenative aromatization of the 1,6-enyne 3a.3a,15 To the best of our knowledge, it is the best result among the literature for synthesizing this scaffold. Moreover, bicyclohexadiene 9 was synthesized by the intermolecular Ru-catalyzed [2 + 2 + 2] carbocyclization reaction with more than 20
:
1 dr.16 Additionally, Sonogashira coupling proceeded smoothly to afford 10 in 85% yield.17 Terminal deuteration of alkyne was achieved in very mild conditions, providing the deuterated alkyne 11 in 65% yield.18 Of particular importance, Rh-catalyzed intramolecular cyclization of enyne 4f afforded functionalized cyclic compound 12 with a chiral quaternary carbon center and a ketone moiety in 60% yield.2a
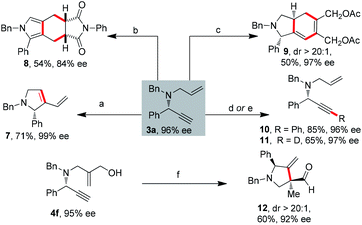 |
| Scheme 6 Derivatization of the enantiomerically enriched 1,6-enynes. aGrubbs catalyst 1st generation (10 mol%), 40 °C, CH2Cl2; b[Ir(cod)Cl]2 (10 mol%), AcOH (6 equiv.), N-phenylmaleimide (1.5 equiv.), toluene, reflux; c[RuCl(cod)(Cp*)] (10 mol%), but-2-yne-1,4-diyl diacetate (3 equiv.), THF, 60 °C; dPd(PPh3)2Cl2 (5 mol%), CuI (7.5 mol%), PhI (1.1 equiv.), 50 °C; eK2CO3 (3 equiv.), MeCN, D2O; fRh(cod)2Cl (10 mol%), rac-BINAP, 40 °C. | |
Remarkably, this reaction can be further applied to the formal total synthesis of (+)-conessine, which was isolated from the bark of Holarrhena antidysenterica and had been used in the treatment of dysentery.19 As shown in Scheme 7, carbaldehyde 14 was easily available from inexpensive 6-methoxy-1-tetralone 13 by NaBH4 reduction, elimination-vinylogous Vilsmeier reaction in multigram quantities with excellent overall yield (82%).19e Subsequently the reductive amination of 14 provided allylic amine 15 in 96% yield. The asymmetric propargylic… amination of 15 with aliphatic propargylic ester 1m gave the corresponding 1,6-enyne 16 in 95% yield with 84% ee, which is the key synthetic intermediate to the target natural product (+)-conessine.19a,20 It is worth noting that purification by column chromatography was required only in the last step among the four-step synthetic route.
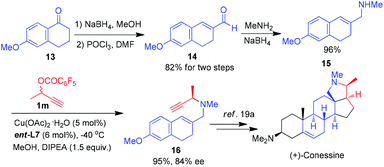 |
| Scheme 7 Asymmetric formal total synthesis of (+)-conessine. | |
Conclusions
In summary, we have developed a highly enantioselective propargylic amination of propargylic esters with benzylic allylic amines, which is a practical and general method for the synthesis of chiral N-tethered 1,6-enynes. The reaction shows a very broad substrate scope regarding the propargylic esters and allylic amines. Subsequently, transition metal-catalyzed cycloisomerization reaction affords the functionalized cyclic and polycyclic pyrolines derivatives, which could not be easily synthesized by traditional methods. Furthermore, the formal total synthesis of (+)-conessine is achieved.
Conflicts of interest
The authors declare no competing financial interest.
Acknowledgements
Generous financial support from the National Natural Science Foundation of China (21801087) and Fundamental Research Funds for the Central Universities CCNU (CCNU19QN064) is gratefully acknowledged. We thank Prof. Houhua Li (Perking University) for helpful discussion.
Notes and references
-
(a) J. Xuan and A. Studer, Radical Cascade Cyclization of 1,n-Enynes and Diynes for the Synthesis of Carbocycles and Heterocycles, Chem. Soc. Rev., 2017, 46, 4329–4346 CAS;
(b) J. D. Ricker and L. M. Geary, Recent Advances in the Pauson–Khand Reaction, Top. Catal., 2017, 60, 609–619 CrossRef CAS;
(c) Y. Hu, M. Bai, Y. Yang and Q. Zhou, Metal-Catalyzed Enyne Cycloisomerization in Natural Product Total Synthesis, Org. Chem. Front., 2017, 4, 2256–2275 RSC;
(d) V. Michelet, P. Y. Toullec and J.-P. Genêt, Cycloisomerization of 1,n-Enynes: Challenging Metal-Catalyzed Rearrangements and Mechanistic Insights, Angew. Chem., Int. Ed., 2008, 47, 4268–4315 CrossRef CAS;
(e) L. Zhang, J. Sun and S. A. Kozmin, Gold and Platinum Catalysis of Enyne Cycloisomerization, Adv. Synth. Catal., 2006, 348, 2271–2296 CrossRef CAS.
-
(a) Y. Oonishi, S. Masusaki, S. Sakamoto and Y. Sato, Rhodium(I)-Catalyzed Enantioselective Cyclization of Enynes by Intramolecular Cleavage of the Rh-C Bond by a Tethered Hydroxy Group, Angew. Chem., Int. Ed., 2019, 58, 8736–8739 CrossRef CAS;
(b) H. Zheng, Y. Wang, C. Xu, Q. Xiong, L. Lin and X. Feng, Diversified Cycloisomerization/Diels–Alder Reactions of 1,6-Enynes through Bimetallic Relay Asymmetric Catalysis, Angew. Chem., Int. Ed., 2019, 58, 5327–5331 CrossRef CAS;
(c) S. Yoshizaki, Y. Nakamura, K. Masutomi, T. Yoshida, K. Noguchi, Y. Shibata and K. Tanaka, Rhodium-Catalyzed Asymmetric [2 + 2 + 2] Cycloaddition of 1,6-Enynes with Cyclopropylideneacetamides, Org. Lett., 2016, 18, 388–391 CrossRef CAS;
(d) T. Shibata, Y. Tahara, K. Tamura and K. Endo, Enantioselective Syntheses of Various Chiral Multicyclic Compounds with Quaternary Carbon Stereocenters by Catalytic Intramolecular Cycloaddition, J. Am. Chem. Soc., 2008, 130, 3451–3457 CrossRef CAS;
(e) T. Shibata and Y. Tahara, Enantioselective Intramolecular [2 + 2 + 2] Cycloaddition of 1,4-Diene-ynes: A New Approach to the Construction of Quaternary Carbon Stereocenters, J. Am. Chem. Soc., 2006, 128, 11766–11767 CrossRef CAS;
(f) P. A. Evans, K. W. Lai and J. R. Sawyer, Regio- and Enantioselective Intermolecular Rhodium-Catalyzed [2 + 2 + 2] Carbocyclization Reactions of 1,6-Enynes with Methyl Arylpropiolates, J. Am. Chem. Soc., 2005, 127, 12466–12467 CrossRef CAS;
(g) T. Shibata, Y. Arai and Y. Tahara, Enantioselective Construction of Quaternary Carbon Centers by Catalytic [2 + 2 + 2] Cycloaddition of 1,6-Enynes and Alkynes, Org. Lett., 2005, 7, 4955–4957 CrossRef CAS.
-
(a) Y. Yamamoto and H. Hayashi, Multi-component Coupling Synthesis of Polycyclic Pyrrole Derivatives via Ir- and Rh-Catalyzed Cycloisomerizations with Microwave Heating, Tetrahedron, 2007, 63, 10149–10160 CrossRef CAS;
(b) N. Gommermann, C. Koradin, K. Polborn and P. Knochel, Enantioselective Copper(I)-Catalyzed Three-Component Reaction for the Preparation of Propargylamines, Angew. Chem., Int. Ed., 2003, 42, 5763–5766 CrossRef CAS;
(c) C. Koradin, K. Polborn and P. Knochel, Enantioselective Synthesis of Propargylamines by Copper-Catalyzed Addition of Alkynes to Enamines, Angew. Chem., Int. Ed., 2002, 41, 2535–2538 CrossRef CAS;
(d) B. Jiang and M. Xu, Catalytic Diastereoselective Pauson−Khand Reaction: An Efficient Route to Enantiopure Cyclopenta[c]proline Derivatives, Org. Lett., 2002, 4, 4077–4080 CrossRef CAS.
- T. Sugiishi and H. Nakamura, Zinc(II)-Catalyzed Redox Cross-Dehydrogenative Coupling of Propargylic Amines and Terminal Alkynes for Synthesis of N-Tethered 1,6-Enynes, J. Am. Chem. Soc., 2012, 134, 2504–2507 CrossRef CAS.
- G. Hattori, K. Sakata, H. Matsuzawa, Y. Tanabe, Y. Miyake and Y. Nishibayashi, Copper-Catalyzed Enantioselective Propargylic Amination of Propargylic Esters with Amines: Copper-Allenylidene Complexes as Key Intermediates, J. Am. Chem. Soc., 2010, 132, 10592–10608 CrossRef CAS.
- For selected reviews on the Cu-catalyzed asymmetric propargylic substitution:
(a) X.-H. Hu, Z.-T. Liu, L. Shao and X.-P. Hu, Recent Advances in Catalytic Stereocontrolled Cycloaddition with Terminal Propargylic Compounds, Synthesis, 2015, 47, 913–923 CrossRef CAS;
(b) D.-Y. Zhang and X.-P. Hu, Recent Advances in Copper-Catalyzed Propargylic Substitution, Tetrahedron Lett., 2015, 56, 283–295 CrossRef CAS;
(c) E. B. Bauer, Transition-Metal-Catalyzed Functionalization of Propargylic Alcohols and Their Derivatives, Synthesis, 2012, 44, 1131–1151 CrossRef CAS;
(d) Y. Nishibayashi, Transition-Metal-Catalyzed Enantioselective Propargylic Substitution Reactions of Propargylic Alcohol Derivatives with Nucleophiles, Synthesis, 2012, 44, 489–503 CrossRef CAS;
(e) C.-H. Ding and X.-L. Hou, Catalytic Asymmetric Propargylation, Chem. Rev., 2011, 111, 1914–1937 CrossRef CAS;
(f) Y. Miyake, S. Uemura and Y. Nishibayashi, Catalytic Propargylic Substitution Reactions, ChemCatChem, 2009, 1, 342–356 CrossRef CAS;
(g) R. J. Detz, H. Hiemstra and J. H. van Maarseveen, Catalyzed Propargylic Substitution, Eur. J. Org. Chem., 2009, 6263–6276 CrossRef CAS;
(h) N. Ljungdahl and N. Kann, Transition-Metal-Catalyzed Propargylic Substitution, Angew. Chem., Int. Ed., 2009, 48, 642–644 CrossRef CAS.
-
(a) Z.-T. Liu, Y.-H. Wang, F.-L. Zhu and X.-P. Hu, Enantioselective Copper-Catalyzed Formal [4 + 2] Cycloaddition of o-Aminophenol Derivatives with Propargylic Esters for Synthesis of Optically Active 3,4-Dihydro-2H-1,4-benzoxazines, Org. Lett., 2016, 18, 1190–1193 CrossRef CAS;
(b) D.-Y. Zhang, L. Shao, J. Xu and X.-P. Hu, Copper-Catalyzed Asymmetric Formal [3 + 2] Cycloaddition of Propargylic Acetates with Hydrazines: Enantioselective Synthesis of Optically Active 2-Pyrazolines, ACS Catal., 2015, 5, 5026–5030 CrossRef CAS;
(c) F.-Z. Han, F.-L. Zhu, Y.-H. Wang, Y. Zou, X.-H. Hu, S. Chen and X.-P. Hu, Highly Enantioselective Copper-Catalyzed Propargylic Substitution of Propargylic Acetates with 1,3-Dicarbonyl Compounds, Org. Lett., 2014, 16, 588–591 CrossRef CAS;
(d) C. Zhang, Y.-H. Wang, X.-H. Hu, Z. Zheng, J. Xu and X.-P. Hu, Chiral Tridentate P,N,N Ligands for Highly Enantioselective Copper-Catalyzed Propargylic Amination with both Primary and Secondary Amines as Nucleophiles, Adv. Synth. Catal., 2012, 354, 2854–2858 CrossRef CAS;
(e) A. Yoshida, G. Hattori, Y. Miyake and Y. Nishibayashi, Copper-Catalyzed Enantioselective Propargylic Amination of Nonaromatic Propargylic Esters with Amines, Org. Lett., 2011, 13, 2460–2463 CrossRef CAS;
(f) R. J. Detz, Z. Abiri, R. le Griel, H. Hiemstra and J. H. van Maarseveen, Enantioselective Copper-Catalysed Propargylic Substitution: Synthetic Scope Study and Application in Formal Total Syntheses of (+)-Anisomycin and (-)-Cytoxazone, Chem.–Eur. J., 2011, 17, 5921–5930 CrossRef CAS;
(g) G. Hattori, Y. Miyake and Y. Nishibayashi, Copper-Catalyzed Diastereo- and Enantioselective Sequential Reactions of Propargylic Acetates with (E)-2,4-Pentadienylamine, ChemCatChem, 2010, 2, 155–158 CrossRef CAS;
(h) G. Hattori, H. Matsuzawa, Y. Miyake and Y. Nishibayashi, Copper-Catalyzed Asymmetric Propargylic Substitution Reactions of Propargylic Acetates with Amines, Angew. Chem., Int. Ed., 2008, 47, 3781–3783 CrossRef CAS;
(i) R. J. Detz, M. M. E. Delville, H. Hiemstra and J. H. van Maarseveen, Enantioselective Copper-Catalyzed Propargylic Amination, Angew. Chem., Int. Ed., 2008, 47, 3777–3780 CrossRef CAS.
-
(a) Y. Liu, A. Afanasenko, S. Elangovan, Z. Sun and K. Barta, Primary Benzylamines by Efficient N-Alkylation of Benzyl Alcohols Using Commercial Ni Catalysts and Easy-to-Handle Ammonia Sources, ACS Sustainable Chem. Eng., 2019, 7, 11267–11274 CrossRef CAS;
(b) J.-B. Peng, F.-P. Wu, C. Xu, X. Qi, J. Ying and X.-F. Wu, Direct Synthesis of Benzylic Amines by Palladium-Catalyzed Carbonylative Aminohomologation of Aryl Halides, Commun. Chem., 2018, 1, 29 CrossRef;
(c) T. Ide, J. P. Barham, M. Fujita, Y. Kawato, H. Egami and Y. Hamashima, Regio- and Chemoselective Csp3-H Arylation of Benzylamines by Single Electron Transfer/Hydrogen Atom Transfer Synergistic Catalysis, Chem. Sci., 2018, 9, 8453–8460 RSC.
- H. Xu, L. Laraia, L. Schneider, K. Louven, C. Strohmann, A. P. Antonchick and H. Waldmann, Highly Enantioselective Catalytic Vinylogous Propargylation of Coumarins Yields a Class of Autophagy Inhibitors, Angew. Chem., Int. Ed., 2017, 56, 11232–11236 CrossRef CAS.
-
(a) F.-L. Zhu, Y. Zou, D.-Y. Zhang, Y.-H. Wang, X.-H. Hu, S. Chen, J. Xu and X.-P. Hu, Enantioselective Copper-Catalyzed Decarboxylative Propargylic Alkylation of Propargyl β-Ketoesters with a Chiral Ketimine P,N,N-Ligand, Angew. Chem., Int. Ed., 2014, 53, 1410–1414 CrossRef CAS;
(b) C. Zhang, X.-H. Hu, Y.-H. Wang, Z. Zheng, J. Xu and X.-P. Hu, Highly Diastereo- and Enantioselective Cu-Catalyzed [3 + 3] Cycloaddition of Propargyl Esters with Cyclic Enamines toward Chiral Bicyclo[n.3.1] Frameworks, J. Am. Chem. Soc., 2012, 134, 9585–9588 CrossRef CAS.
- Aliphatic propargylic esters were less investigated in asymmetric propargylic substitution
(a) Q. Zhu, B. Meng, C. Gu, Y. Xu, J. Chen, C. Lei and X. Wu, Diastereo- and Enantioselective Synthesis of Quaternary α-Amino Acid Precursors by Copper-Catalyzed Propargylation, Org. Lett., 2019, 21, 9985–9989 CrossRef CAS;
(b) X. Gao, R. Cheng, Y.-L. Xiao, X.-L. Wan and X. Zhang, Copper-Catalyzed Highly Enantioselective Difluoroalkylation of Secondary Propargyl Sulfonates with Difluoroenoxysilanes, Chem, 2019, 5, 2987–2999 CrossRef CAS;
(c) K. Nakajima, M. Shibata and Y. Nishibayashi, Copper-Catalyzed Enantioselective Propargylic Etherification of Propargylic Esters with Alcohols, J. Am. Chem. Soc., 2015, 137, 2472–2475 CrossRef CAS . Also see ref. 7d and e.
- The absolute configuration of 4a was assigned by comparison with the literature (see the ESI†). All other compounds was assigned by analogy.
- CCDC 1975893 (CuCl + L7) contain the supplementary crystallographic data for this paper.
- Q. Yang, H. Alper and W.-J. Xiao, Efficient Method for the Synthesis of Chiral Pyrrolidine Derivatives via Ring-Closing Enyne Metathesis Reaction, Org. Lett., 2007, 9, 769–771 CrossRef CAS.
- Y. Yamamoto, H. Hayashi, T. Saigoku and H. Nishiyama, Domino Coupling Relay Approach to Polycyclic Pyrrole-2-carboxylates, J. Am. Chem. Soc., 2005, 127, 10804–10805 CrossRef CAS . The absolute configuration of 8 was not assigned in this paper.
- R. Liu, L. Giordano and A. Tenaglia, Ruthenium-Catalyzed [2 + 2 + 2] Cycloaddition of 1,6-Enynes and Unactivated Alkynes: Acess to Ring-Fused Cyclohexadienes, Chem.–Asian J., 2017, 12, 2245–2257 CrossRef CAS.
- K. Sonogashira, Development of Pd–Cu Catalyzed Cross-coupling of Terminal Acetylenes with sp2-Carbon Halides, J. Organomet. Chem., 2002, 653, 46–49 CrossRef CAS.
- S. P. Bew, G. D. Hiatt-Gipson, J. A. Lovell and C. Poullain, Mild Reaction Conditions for the Terminal Deuteration of Alkynes, Org. Lett., 2012, 14, 456–459 CrossRef CAS.
-
(a) B. Jiang and M. Xu, Highly Enantioselective Construction of Fused Pyrrolidine Systems that Contain a Quaternary Stereocenter: Concise Formal Synthesis of (+)-Conessine, Angew. Chem., Int. Ed., 2004, 43, 2543–2546 CrossRef CAS;
(b) A. M. Lannang, S. Anjum, J. G. Tangmouo, K. Krohn and M. I. Choudhary, Conessine Isolated from Holarrhena Floribunda, Acta Crystallogr., Sect. E: Struct. Rep. Online, 2007, 63, 4398–4399 CrossRef;
(c) M. E. Kopach, A. H. Fray and A. I. Meyers, An Asymmetric Route to the Conanine BCDE Ring System. A Formal Total Synthesis of (+)-Conessine, J. Am. Chem. Soc., 1996, 118, 9876–9883 CrossRef CAS;
(d) K. K. Bhutani, M. Ali, S. R. Sharma, R. M. Vaid and D. K. Gupta, Three New Steroidal Alkaloids from the Bark of Holarrhena antidysenterica, Phytochemistry, 1988, 27, 925–928 CrossRef CAS;
(e) K. Tiefenbacher, L. Tröndlin, J. Mulzer and A. Pfaltz, An Expeditious Asymmetric Formal Synthesis of the Antibiotic Platensimycin, Tetrahedron, 2010, 66, 6508–6513 CrossRef CAS.
- The R-configuration of Ligand (ent-L7) was used. The data of compound 15 consistent with data reported in the literature. (see the ESI†).
Footnotes |
† Electronic supplementary information (ESI) available: General information, experimental procedures, NMR spectra, and HPLC spectra (PDF) crystallographic structure of complexes of CuCl and L7 (CIF). CCDC 1975893. For ESI and crystallographic data in CIF or other electronic format see DOI: 10.1039/d0ra07698h |
‡ S. L. and J. H. contributed equally. |
|
This journal is © The Royal Society of Chemistry 2020 |
Click here to see how this site uses Cookies. View our privacy policy here.