DOI:
10.1039/D0RA07157A
(Paper)
RSC Adv., 2020,
10, 35381-35388
A non-metal route to realize the bio-based polyester of poly(hexylene succinate): preparation conditions, side-reactions and mechanism in sulfonic acid-functionalized Brønsted acidic ionic liquids†
Received
20th August 2020
, Accepted 16th September 2020
First published on 24th September 2020
Abstract
A biodegradable linear bio-based polyester of poly(hexylene succinate) was effectively prepared in non-metal sulfonic acid-functionalized Brønsted acidic ionic liquids (SFBAILs) as both the catalyst and the polymerization medium, and the processes of polycondensation and post-polycondensation in SFBAILs were also investigated. In addition, the side reactions which were detrimental to the growth of Mw of poly(hexylene succinate) were evaluated and the synthesis mechanism of poly(hexylene succinate) catalyzed by SFBAILs was discussed with the help of DFT calculations. The result shows that both the imidazole ring and the sulfonic group on cations of SFBAILs play an important role in the catalytic process.
Introduction
Biomass is one of the largest quantities of natural resources in the world, and every year 104.9 petagrams of carbon biomass is produced.1 Biomass derived from agricultural residues and forest by-products has both easy processability and relatively low cost.2 Thus, biomass can be called a “green resource”, and fits well with the concept “sustainable development”, i.e., “sustainable development is a social development which fulfils the needs of present generations without endangering the possibilities of fulfilment of the needs of future generations”.3 However, this material is not fully utilized and a large quantity of biomass is simply burned and causes pollution every year.
To solve this problem, a new material, biomass polyesters, has been formed. Polyesters formed by biomass are among the most promising polymers. Polyesters are usually biodegradable and biocompatible, and the monomers from biomass are easily accessed. Moreover, different monomers with different structures and properties makes polyesters have a wide range of applications.4 Due to their multiple properties, polyesters formed by biomass become a research focus of green chemistry. The synthesis of polyesters is usually realized by polycondensation.
However, polycondensation generally requires harsh conditions. A high temperature (160–300 °C) and vacuum are applied at polycondensation to remove small molecules from the polymerization systems and obtain a relatively high molecular weight. This traditional method is usually limited by low heat transfer efficiency caused by viscous reaction systems and side reactions due to the high temperature. The catalyst is also a considerable aspect of the polycondensation due to the long reaction time (more than several hours). However, most recent studies focused on metal catalysts, such as metal salts, metal oxides or metal alkoxides, in particular, catalysts based on Sn or Zn and metals inevitably remain in the products, limiting the use of products.5 In Yoon's work, high molecular weight poly(L-lactic acid) was obtained by Ti(OBu)4. Although 130 kDa of molecular weight could be achieved, the use of metal catalysis is a necessary.6 The method of solution reaction is also mentioned in Fradet's work as a resolution for low molecular weight caused by traditional methods,7 but the expensive or toxic starting monomers, activating agents and solvents limit the method. In addition, with the higher standard of environmentally friendliness, using fewer organic solvents or recyclable solvents is necessary due to a large amount of waste during the process of producing polymers.8
Considering these problems, there has recently been growing interest in ionic liquids, which were applied to synthesize polymers such as polyimide, polyamide,9 polysulfone,10 and polyester,11 or copolymers such as polyesteramide.12 Brönsted acidic ionic liquids (BAILs) are ones with task-specific functionality and well known in polymers synthesis for its remarkable ability of catalysis.13 A simple route can be used to separate BAILs from the products. Moreover, BAILs have the properties of conventional ionic liquids such as non-volatility, non-flammability, a wide liquidus range and high conductivity. With so many advantages, they are being used in many fields.14
In Fradet's works,15 some types of polyesters (mainly linear polyesters of 12-hydroxydodecanoic acid hyperbranched polyester of 2,2-bis(hydroxymethyl)propanoic acid) were produced in two types of Brönsted acidic ionic liquids, that is, HSO4− anionic and Tf2N− anionic BAILs, in a relatively mild reaction condition. In our recent work,16 the high molecular weight copolymer of L-lactic acid and ε-caprolactone were achieved in SFBAILs in a mild condition. Thus, Brönsted acidic ionic liquids are potentially selected for polycondensation.
Poly(hexylene succinate) is a type of novel and promising polyester plasticizer for the modification of poly(vinyl chloride) (PVC) and can be employed as an alternative of the traditional dioctyl phthalate (DOP).17 However, few investigations concentrated on this polyester synthesized in BAILs. And not only that, the side reactions which usually happen in the post-polycondensation process limit the growth of Mw of this product and restrict the further practical applications. Therefore, it is necessary to study the side reactions in this process.
In this report, we investigated the polycondensation and post-polycondensation process of linear monomers of succinic acid and 1,6-hexanediol in sulfonic acid-functionalized Brönsted acidic ionic liquids (SFBAILs), which belong to a Brönsted acidic ionic liquids and are known for their catalytic capability. And the most suitable reaction condition was explored. Moreover, the side reactions during the post-polycondensation in SFBAILs were discussed, and an interesting paradox between our observations and the theory proposed by Fradet et al. was witnessed. To explain this phenomenon, density functional theory (DFT) calculations were firstly carried out here and an explanation for this paradox was worked out, which refined this already existed theory. Moreover, with the help of DFT method, a simple but reasonable model was established and the catalytic mechanism of SFBAILs was revealed.
Results and discussion
In this work, six sulfonic acid-functionalized Brönsted acidic ionic liquids (SFBAILs) with different cations and anions were synthesized and were used as catalysts and media. The polycondensation and post-polycondensation processes of oligo(hexylene succinate) were investigated in these SFBAILs (Table 1).
Table 1 H0 values of SFBAILs determined by UV-vis spectroscopy at 50 °C
SFBAILs |
Cations |
Anions |
H0a |
The Hammett acidities of SFBAILs were determined using the Hammett method with UV-vis spectroscopy at 50 °C, and 25 mg L−1 of 2,4-dichloro-6-nitroaniline were used as the indicator. From ref. 18. |
[BSMIM][HSO4] |
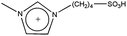 |
HSO4− |
−3.34 |
[BSMIM][TFS] |
CF3SO3− |
−4.06 |
[BSMIM][TS] |
p-CH3(C6H4)SO3− |
−3.50 |
[BSMIM][MS] |
CH3SO3− |
−3.34 |
[BSMIM][BF4] |
BF4− |
−3.68 |
[BSPy][HSO4] |
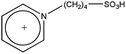 |
HSO4− |
−3.30b |
Acidity of SFBAILs
Herein, the Hammett acidity functions (H0) were used to evaluate the acidity of SFBAILs by UV-vis spectroscopy. The results are listed in ESI (Fig. S1, S2 and Table S1).†
Hammett acidity function is defined as19
Here, p
K(I)
aq is the p
Ka value of the aqueous solution of the indicator, and the p
Ka value of the indicator 2,4-dichloro-6-nitroaniline here is −3.31. [IH
+] is the molar concentration of indicator protonated by SFBAILs and [I] is the molar concentration of the unprotonated indicator.
As shown in Table 1, the acidities of SFBAILs determined by the Hammett method here range from −3.34 to −4.06, which has no difference from that of pyridinium-type sulfonic acidic ionic liquids according to the literature.
In our previous work, the acidities of SFBAILs were compared with the acidities of corresponding conventional organic sulfonic ionic liquids. We found that the order of SFBAILs acidity was related to the acidity of proton acid corresponding to the anions of SFBAILs, which may be due to the combination of concentrated anions of SFBAILs with protons. The stronger cohesiveness of anions and protons corresponds to the lower concentration of free hydrogen ions, and lower acidity (Scheme 1). However, anions hardly affect on the acidity of SFBAILs, because all of them have identical sulfonic acid group and acidity.
 |
| Scheme 1 Dissociation equilibrium of sulfonic acid-functionalized Brönsted acidic ionic liquids. | |
Thermal stability of SFBAILs
The thermal stability of SFBAILs is very important because the post-polycondensation usually requires a relatively high polymerization temperature. Most of the SFBAILs have excellent thermostability, and their decomposition temperatures are higher than 250 °C. However, [BSMIM][BF4] shows a different decomposition process. There are two decomposition stages at approximately 110 °C and 310 °C. The weight loss between two stages is approximately 20%, which can be attribute to the weight loss of BF3 due to the conversion of [BSMIM][BF4] to [BSMIM][F] (Fig. 1).
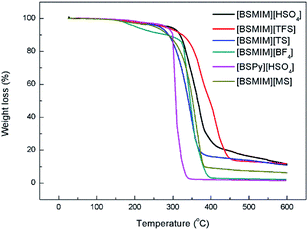 |
| Fig. 1 Thermogravimetric curves of different sulfonic acid-functionalized Bronsted acidic ionic liquids. | |
Post-polycondensation in SFBAILs
Miscellaneous post-polycondensation conditions in SFBAILs were investigated in this work. Table 2 shows that the type of anions and cations has no effect on the molecular weight of polymers in this system. The molecular weight of polymer in [BSMIM][HSO4] can reach 22 kDa, which is the highest. The molecular weight of polymer in [BSMIM][BF4] is minimum, which can be explained by the decomposition of [BSMIM][BF4] determined by the analysis of thermal stability.
Table 2 Post-polycondensation of oligo(hexylene succinate) in sulfonic acid-functionalized Brönsted acidic ionic liquidsa
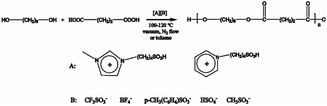
|
Run |
SFBAILs |
Temp. (°C) |
Water removalb |
Mw (kDa) |
PDI |
Yield (%) |
Post-polycondensation: post-polycondensation at the given temperature for 60 min. In the nitrogen flow systems, flow rate of 500 mL min−1 applied over the reaction. |
1 |
[BSMIM][HSO4] |
110 |
N2-flow |
22.0 |
1.78 |
80.1 |
2 |
[BSMIM][TFS] |
110 |
N2-flow |
22.0 |
1.79 |
76.5 |
3 |
[BSMIM][TS] |
110 |
N2-flow |
15.0 |
1.56 |
81.2 |
4 |
[BSMIM][MS] |
110 |
N2-flow |
15.1 |
1.63 |
66.3 |
5 |
[BSMIM][BF4] |
110 |
N2-flow |
9.9 |
1.50 |
52.4 |
6 |
[BSPy][HSO4] |
110 |
N2-flow |
12.7 |
1.60 |
78.7 |
7 |
[BSMIM][HSO4] |
110 |
Toluene |
10.4 |
1.75 |
76.2 |
8 |
[BSMIM][HSO4] |
110 |
2000 Pa |
7.3 |
1.57 |
77.3 |
9 |
[BSMIM][HSO4] |
110 |
1000 Pa |
12.8 |
1.64 |
79.2 |
10 |
[BSMIM][HSO4] |
100 |
N2-flow |
14.3 |
1.59 |
69.8 |
11 |
[BSMIM][HSO4] |
120 |
N2-flow |
13.9 |
1.62 |
65.1 |
Furthermore, the different polymerization temperature was studied. The most suitable temperature is 110 °C, followed by 100 °C, and 120 °C is the least. This result is attributed to the fact that a lower temperature can contribute to a lower reaction rate while a higher temperature can generate more side reactions.
Moreover, experiments in different methods of water removal were conducted to evaluate the most effective method to remove water. Clearly, the effective method is blowing nitrogen. The vacuum degree in the vacuum system is an important aspect of affecting the molecular weight of polymer. When the system reaches 2000 Pa, the water in this system can hardly be moved. However, when the system reaches 1000 Pa, the water can be relatively effectively removed. In our previous work,20 toluene was used to eliminate water since the formation of azeotrope between water and toluene can help water remove in reaction system. However toluene to remove water does not appear effective due to the low temperature that cannot help the azeotrope of water and toluene to effectively evaporate.
Dynamics of the polycondensation and post-polycondensation in SFBAILs
The polycondensation process. The polycondensation process was monitored by the SEC method and the molecular weights of oligomers with different reaction times were measured. The results are shown in Fig. 2. The results indicate that the molecular weights of oligomers increase with the reaction time in 9 h. However, the growth rate of oligomer's molecular weight decreases. For further research on this process, 1H NMR was used to evaluate the kinetics. The oligomers were dissolved in CDCl3. Fig. 3 shows the 1H NMR spectra of the oligomer and polymer of succinic acid and 1,6-hexanediol at different times.
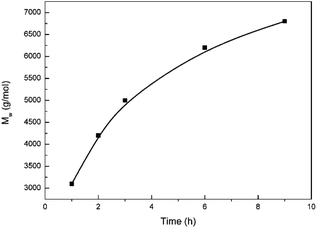 |
| Fig. 2 Mw of oligo(hexylene succinate) with reaction time. | |
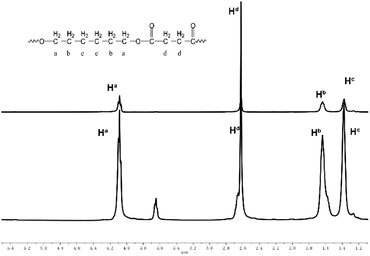 |
| Fig. 3 1H NMR spectra (CDCl3, 400 MHz) of oligo(hexylene succinate) at the 2nd hour (below) and poly(hexylene succinate) at the 30th minute (above). | |
Herein, P is used and defined as the conversion ratio, and P can be calculated by the concentration of the reactive functional group –OH. Thus, P can be defined as
P = ([OH]0 − [OH]t)/[OH]0 |
The concentration of the reactive functional group –OH can be found from 1H NMR spectra according to different chemical shifts between the main chains and the end groups.
In addition, the self-catalytic esterification usually applies to the third-order reaction model. The third-order rate equation can be defined as
The results were shown in Fig. 4, and it has a good linear fitting, which implies that the third-order reaction model can be used to describe the polycondensation process. The main reason for the decrease in the molecular weight growth rate is the reaction consumption of acid instead of the system viscosity at 160 °C. In fact, at this temperature, the reaction system can still be easily magnetically stirred, which proves a relatively low viscosity during this process.
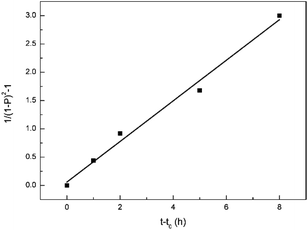 |
| Fig. 4 Variation of (1/(1 − p)2 − 1) versus reaction time (t–t0) of copolymerization of succinic acid and 1,6-hexamethylene glycol. | |
The study of post-polycondensation process. The post-polycondensation process in [BSMIM][HSO4] and [BSMIM][TFS] were examined and the results were shown in Fig. 5. The results indicate that at the beginning of the reaction, the molecular weight of polymer quickly increases in both SFBAILs. However, a decrease in molecular weight can be observed after 30 min in [BSMIM][TFS]. At the beginning of the reaction, the growth rate of Mw fits well with linear since acid-catalyzed polyesterifications are second-order reactions,21 However, after 30 min, the polymerization medium viscosity increases, and water in this system cannot be easily moved, which, limits the molecular weight growth of polymers in SFBAILs. In fact, at 110 °C, the reaction medium was more viscous than that at 160 °C, which can explain this limitation. And a decrease was observed in [BSMIM][TFS].
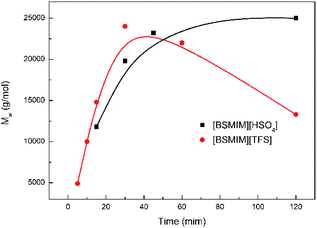 |
| Fig. 5 Mw of poly(hexylene succinate) in [BSMIM][TFS] and [BSMIM][HSO4] at different times. | |
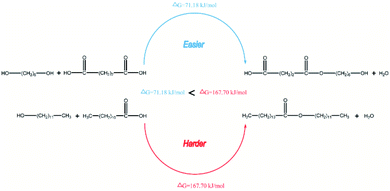 |
| Fig. 6 The DFT calculations of free energies of two esterification processes. | |
For more information about this polymerization in SFBAILs, the decrease in molecular weight of polymer in SFBAILs was studied. Fig. 7 shows the 1H NMR of polyesters catalyzed by [BSMIM][HSO4] and [BSMIM][TFS], and Table 3 listed all the impurities. The resonance at 3.40 ppm, which proved another structure formed, is etherification, a well-known reaction happening between two OH-groups in the acidic environmental (Scheme 2). In fact, etherification can occur when aliphatic hydroxyl groups appear in SFBAILs.22 Etherification can consume –OH end-groups and limit the polyesterification process, so the molecular weight decreases. However, this side reaction appears not to occur in [BSMIM][HSO4]. In fact, in Fradet's case,23 SFBAIL 3-butyl-1-(butyl-4′-sulfonic acid) imidazolium hydrogen sulfate ([BBSMIM][HSO4]) can react with hydroxyl group of 12-hydroxydodecanoic acid (12-HDA) and sulfonic ester was formed (Scheme 3), then transesterification occurred between sulfonic ester and carboxylic acid. The concentration of hydroxyl group decreased, which limited the etherification process. If this theory is right, sulfonic esters can be formed between the hydroxyl groups and [BSMIIM][HSO4], demonstrating that the type of anions in SFBAILs are very essential in the behaviors of SFBAILs when they are used as the catalyst and the medium for polycondensation.
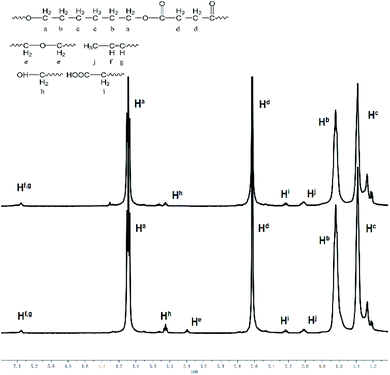 |
| Fig. 7 1H NMR spectra (CDCl3, 400 MHz) of poly(hexylene succinate) catalysed by [BSMIM][TFS] (below) and by [BSMIM][HSO4] (above). | |
Table 3 The impurities examined by the 1H NMR spectra of polyesters in [BSMIM][TFS] and [BSMIM][HSO4]
Entry |
Structure |
Resonance |
1 |
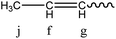 |
Hf,g (5.3–5.4 ppm) and Hj (1.8–2.2 ppm) |
2 |
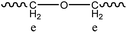 |
He (3.2–3.4 ppm) |
 |
| Scheme 2 Side reaction of etherification in [BSMIM][TFS] during the post-polycondensation process. | |
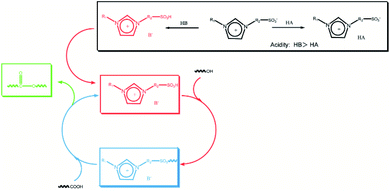 |
| Scheme 3 Formation of sulfonic esters in SFBAILs. | |
However, this theory seems inapplicable because ethers were found in polymer in [BSMIM][TFS] since CF3SO3H is a relatively strong acid, [BSMIM][TFS] is formed by sulfonic acid and CF3SO3−, sulfonic esters can be easily formed and no ethers should be observed. Therefore, further discussion is required. To obtain more information about the reaction, simple DFT calculations were conducted here at the method of B3LYP/def2svp in Gaussian 16 software to evaluate the difference of kinetics of these two reactions and the results were listed in Fig. 8, and all energies given here were the sum of electronic and thermal free energies at 110 °C. Grimme's empirical dispersion-correction and solvation effects (using the PCM model with dichloroethane as the solvent24) were introduced to all calculations, optimization and frequency analysis. As indicated before, the routes proposed by Fradet's work went through two competitive experiments: one is the formation of sulfonate esters and the other is the main reaction, and more sulfonate esters means less side reactions. When compared the free energy of corresponding reactions in [BSMIM][TFS] and [BBSIm][HSO4] at 373.15 K, as shown in Fig. 6, the free energy of esterification in our case is 71.18 kJ mol−1 and 167.70 kJ mol−1 in Fradet's case. The difference of ΔG between two esterification reactions implies that the main reaction between 1,6-hexanediols and succinic acid is easier to happen than dodecan-1-ol and dodecanoic acid. Hence, since the main reaction route in our case is easier to happen, and less sulfonate esters will be formed, and ethers formation in [BSMIM][TFS] and [BSMIM][HSO4] is more possible to happen. However, this seems not explain why ethers found only in [BSMIM][TFS], and maybe this cause by the stronger acidity and more sulfonic acid in [BSMIM][TFS], which promotes the etherification process.
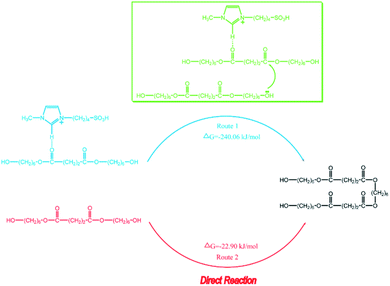 |
| Fig. 8 The DFT calculations of free energies of esterification process with or without cation of SFBAILs catalysis (model based on Tang's work). | |
In addition, the resonance at 5.35 ppm reflects the formation of double bonds (Schemes 4 and 5). In this case, the double bonds may be caused by the dehydration of the –OH end-group catalyzed by SFBAILs or the scission of ester bonds and ether bonds, which is similar to other polyesters in SFBAILs.25
 |
| Scheme 4 Formation of double bonds through esters or ethers. | |
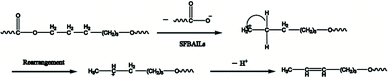 |
| Scheme 5 The possible mechanism of the formation of double bonds through esters or ethers. | |
Possible mechanism of post-polycondensation in SFBAILs. The mechanism of post-polycondensation in SFBAILs was investigated with the DFT method. In Tang's recent work,26 the polycondensation of poly(ethylene 2,5-furandicarboxylate) in [C2MIM]BF4 was found to go through the formation of hydrogen bond between ionic liquids and carbonyl oxygen, which helped the –OH group to attack carbonyl oxygen. Under such a premise, the model of SFBAILs and reactants was established and the result was listed in Fig. 8. For post-polycondensation of poly(hexylene succinate) in SFBAILs, DFT calculations show that when the reaction went through the formation of hydrogen bond process (route 1), the free energy is about 217 kJ mol−1 less than the free energy of the reaction without bond formation at 110 °C (route 2). This result shows that the cations of SFBAILs catalyzed the post-polycondensation stage by the hydrogen bond between carbonyl oxygen and the hydrogen on imidazole ring, moreover, the optimization result shows that the sulfonic acid group on cations of SFBAILs can play as a proton donor and –OH group is the acceptor, and this can also promote the esterification process (Fig. 9).
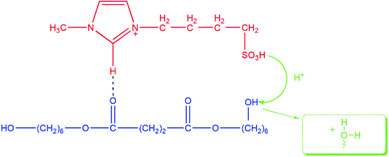 |
| Fig. 9 The optimization model of hydrogen bonds between intermediate and cation of SFBAILs catalysis. | |
Recycle of SFBAILs
As “green” solvents, SFBAILs have good recyclability. SFBAILs can be easily recycled by using deionized water or isopropanol. The results of post-polycondensation in [BSMIM][HSO4] after being recycled thrice are showed in Fig. 10. There is no big difference in molecular weights, showing that [BSMIM][HSO4] has good recyclability in this reaction system.
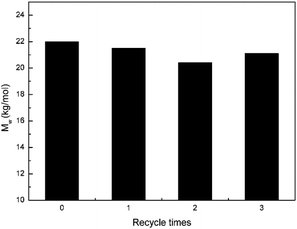 |
| Fig. 10 Post-polycondensation of 1,6-hexanediol and succinic acid after 3 recycled times. | |
Conclusions
In conclusion, the polycondensation process and post-polycondensation process of the biomass material of poly(hexylene succinate) in SFBAILs have been investigated. The polycondensation process in 9 h fits well with the third-order reaction model and there is no obvious viscosity limitation. The post-polycondensation process in SFBAILs was influenced by the temperature, the acidity and thermostability of SFBAILs, and the water removal method. In this system, the type of cations or anions of SFBAILs has no evident effect on the molecular weight. The kinetic study indicates that the post-polycondensation process is a SFBAILs acid-catalyzed polymerization, and can be evaluated by the second-order reaction model. After 30 min of polymerization, the system becomes inevitably viscous and limits the growth of molecular weight of poly(hexylene succinate). In addition, the formation of ethers was found in poly(hexylene succinate) in [BSMIM][TFS], and double bonds was found in both SFBAILs. The anions of SFBAILs may play an important role in the formation of ethers during the post-polycondensation process. As DFT results show, during the post-polycondensation stage, both the imidazole ring and the sulfonic group on cations of SFBAILs participate in the catalytic process. Finally, the recycled SFBAILs keep their excellent performance after 3 recycles, and this is a completely green polymerization reaction.
Experimental
Materials
N-Methylimidazole (99%), pyridine (99.8%), 1,4-butyl sultone (99%), tetrafluoroboric acid (50% aqueous solution), methanesulfonic acid (99.5%), trifluoromethanesulfonic acid (98%), and 2,4-dichloro-6-nitroaniline (98%) were purchased from Aladdin (Shanghai, China). Sulfuric acid (98%), and p-toluene sulfonic acid monohydrate (98%) were purchased from Beijing Chemical Works (Beijing, China). All solvents were purchased from Beijing Tong Guang Fine Chemicals Company. All chemicals were used as received.
Characterization
NMR spectra were recorded on a Bruker Avance 400 MHz spectrometer at room temperature and the CDCl3 solution containing 0.03% of tetramethylsilane (TMS) was used as an internal standard. Thermogravimetric analysis (TGA) was performed with a METTLER STRAE SW 9.30 thermogravimetric analyser using nitrogen flow as protective gas, and the heating rate is 10 K min−1. The UV spectra were recorded on a PGeneral TU-1901 UV-vis spectrophotometer at 50 °C, and 2,4-dichloro-6-nitroaniline was used as an indicator at the concentration of 25 mg L−1 in SFBAILs. The calibration curve was made by using the unprotonated indicator which was dissolved in chloroform at concentrations of 5–25 mg L−1, and the correlation coefficient is 0.9954.
Synthesis of SFBAILs
All SFBAILs were synthesized as previously described.27 The mole ratio of N-methylimidazole or pyridine with sulfuric acid (98%) is 1
:
1 to obtain the zwitterions at 80 °C in toluene. Zwitterions were washed by ethyl acetate and dried under vacuum at 60 °C overnight. Then zwitterions were mixed with equimolar protonic acids to obtain the corresponding SFBAILs. SFBAILs were washed with ethyl acetate 5 times and dried in vacuum at 80 °C for 48 h.
Synthesis of oligo(hexylene succinate)
The general procedure is as follows28: 29.523 g (0.25 mol) of succinic acid was mixed with slightly excessive 1,6-hexanediol of 30.488 g (0.258 mol) in a glass tube equipped with a nitrogen inlet, a nitrogen outlet and a magnetic stirrer. The mixture was heated at 160 °C for 1–12 h. After cooling to room temperature, white and crisp solids were obtained. The product was confirmed by 1H NMR.
Post-polycondensation of oligo(hexylene succinate) in SFBAILs
A typical method as follows: the post-polycondensation procedure is as follows: 0.4484 g oligo(hexylene succinate) and 0.3164 g (1 mmol) [BSMIM][HSO4] were introduced in a glass tube with a nitrogen inlet, a nitrogen outlet and a mechanical stirrer. Then, the glass tube was placed in an oil bath at 110 °C under nitrogen flow (500 mL min−1). At the end of the reaction, 10 mL of isopropanol was added and the mixture was heated under reflux for 10 min to eliminate the remaining ionic liquid. After cooling, the precipitate was filtrated and washed with 10 mL of isopropanol and 5 mL of diethyl ether. Then the product was dried in vacuum at 45 °C overnight. The final product was examined by 1H NMR spectra and no impurity were detected except the double bonds and ethers caused by the side reactions.
Conflicts of interest
There are no conflicts to declare.
Notes and references
- C. B. Field, M. J. Behrenfeld, J. T. Randerson and P. Falkowski, Science, 1998, 281, 237–240 CrossRef CAS.
- S. Lems, H. J. van der Kooi and J. de Swaan Arons, Green Chem., 2002, 4, 308–313 RSC.
- J. Deng, M. Li and Y. Wang, Green Chem., 2016, 18, 4824–4854 RSC.
- C. Vilela, A. F. Sousa, A. C. Fonseca, A. C. Serra, J. F. J. Coelho, C. S. R. Freire and A. J. D. Silvestre, Polym. Chem., 2014, 5, 3119–3141 RSC.
- A. Kaoukabi, F. Guillen, H. Qayouh, A. Bouyahya, S. Balieu, L. Belachemi, G. Gouhier and M. Lahcini, Ind. Crops Prod., 2015, 72, 16–23 CrossRef CAS.
- G.-X. Chen, H.-S. Kim, E.-S. Kim and J.-S. Yoon, Eur. Polym. J., 2006, 42, 468–472 CrossRef CAS.
- A. Fradet and M. Tessier, in Synthetic methods in step-growth polymers, ed. M. E. Rogers and T. E. Long, J. Wiley & Sons, NewYork, 2003, ch. 2, pp. 17–132 Search PubMed.
- T. Erdmenger, C. Guerrero-Sanchez, J. Vitz, R. Hoogenboom and U. S. Schubert, Chem. Soc. Rev., 2010, 39, 3317–3333 RSC.
- S. Dewilde, W. Dehaen and K. Binnemans, Green Chem., 2016, 18, 1639–1652 RSC.
- J. Wang and Z. Liu, Green Chem., 2012, 14, 3204–3210 RSC.
- J. Dou and Z. Liu, Green Chem., 2012, 14, 2305–2313 RSC.
-
(a) A. Basterretxea, E. Gabirondo, A. Sanchez-Sanchez, A. Etxeberria, O. Coulembier, D. Mecerreyes and H. Sardon, Eur. Polym. J., 2017, 95, 650–659 CrossRef CAS;
(b) A. Sanchez-Sanchez, A. Basterretxea, D. Mantione, A. Etxeberria, C. Elizetxea, A. de la Calle, S. García-Arrieta, H. Sardon and D. Mecerreyes, J. Polym. Sci., Part A: Polym. Chem., 2016, 54, 2394–2402 CrossRef CAS.
- A. S. Amarasekara and N. C. Okorie, Catal. Commun., 2018, 108, 108–112 CrossRef CAS.
- Z. Xue, L. Qin, J. Jiang, T. Mu and G. Gao, Phys. Chem. Chem. Phys., 2018, 20, 8382–8402 RSC.
-
(a) E.-M. Dukuzeyezu, H. Lefebvre, M. Tessier and A. Fradet, Polymer, 2010, 51, 1218–1221 CrossRef CAS;
(b) S. Zhang, V. Lemaire, A. Féret, H. Lefebvre, M. Tessier and A. Fradet, Polym. Chem., 2013, 4, 1538–1545 RSC.
- Q. Peng, K. Mahmood, Y. Wu, L. Wang, Y. Liang, J. Shen and Z. Liu, Green Chem., 2014, 16, 2234–2241 RSC.
- C. Gao, X. Zhang, J. Sun, Z. Yuan, S. Han, Y. Liu and S. Ji, J. Appl. Polym. Sci., 2018, 135, 46388 CrossRef.
- H. Xing, T. Wang, Z. Zhou and Y. Dai, J. Mol. Catal. A: Chem., 2007, 264, 53–59 CrossRef CAS.
-
(a) L. P. Hammett and A. J. Deyrup, J. Am. Chem. Soc., 1932, 54, 2721–2739 CrossRef CAS;
(b) M. J. Jorgenson and D. R. Hartter, J. Am. Chem. Soc., 1963, 85, 878–883 CrossRef CAS.
- D. He, Y. Wu, Z. Liu and T. Zhao, RSC Adv., 2017, 7, 39604–39610 RSC.
- G. Odian, Principles of polymerization, John Wiley & Sons, Inc., Hoboken, New Jersey, 2004 Search PubMed.
- S. Zhang, A. Feret, H. Lefebvre, M. Tessier and A. Fradet, Chem. Commun., 2011, 47, 11092–11094 RSC.
- S. Zhang, H. Lefebvre, M. Tessier and A. Fradet, Green Chem., 2011, 13, 2786–2793 RSC.
- Y. Jing, R. Zhu, C. Liu and D. Zhang, J. Org. Chem., 2017, 82, 12267–12275 CrossRef CAS.
- T. J. Schildhauer, I. Hoek, F. Kapteijn and J. A. Moulijn, Appl. Catal., A, 2009, 358, 141–145 CrossRef CAS.
- X. L. Qu, M. Jiang, B. Wang, J. Deng, R. Wang, Q. Zhang, G. Y. Zhou and J. Tang, ChemSusChem, 2019, 12, 4927–4935 CrossRef CAS.
-
(a) A. C. Cole, J. L. Jensen, I. Ntai, K. L. T. Tran, K. J. Weaver, D. C. Forbes and J. H. Davis, J. Am. Chem. Soc., 2002, 124, 5962–5963 CrossRef CAS;
(b) M. Yoshizawa, M. Hirao, K. Ito-Akita and H. Ohno, J. Mater. Chem., 2001, 11, 1057–1062 RSC.
- C. Fu and Z. Liu, Polymer, 2008, 49, 461–466 CrossRef CAS.
Footnote |
† Electronic supplementary information (ESI) available: UV-vis spectra and DFT calculations results. See DOI: 10.1039/d0ra07157a |
|
This journal is © The Royal Society of Chemistry 2020 |
Click here to see how this site uses Cookies. View our privacy policy here.