DOI:
10.1039/D0RA05211F
(Paper)
RSC Adv., 2020,
10, 29835-29842
Microwave dielectric properties of low-temperature-fired MgNb2O6 ceramics for LTCC applications
Received
13th June 2020
, Accepted 6th August 2020
First published on 12th August 2020
Abstract
MgNb2O6 ceramics doped with (Li2O–MgO–ZnO–B2O3–SiO2) glass were synthesized by the traditional solid phase reaction route. The effects of LMZBS addition on microwave dielectric properties, grain growth, phase composition and morphology of MgNb2O6 ceramics were studied. The SEM results show dense and homogeneous microstructure with grain size of 1.72 μm. Raman spectra and XRD patterns indicate the pure phase MgNb2O6 ceramic. The experimental results show that LMZBS glass can markedly decrease the sintering temperature from 1300 °C to 925 °C. Higher density and lower porosity make ceramics have better dielectric properties. The MgNb2O6 ceramic doped with 1 wt% LMZBS glass sintered at 925 °C for 5 h, possessed excellent dielectric properties: εr = 19.7, Q·f = 67
839 GHz, τf = −41.01 ppm °C−1. Moreover, the favorable chemical compatibility of the MgNb2O6 ceramic with silver electrodes makes it as promising material for low temperature co-fired ceramic (LTCC) applications.
1. Introduction
The forward evolution of electronics, power systems, satellite broadcasting and wireless communication,1 especially the rapid development of 5G communication, have posed great demands for high integration and miniaturization.2,3 And considering their excellent microwave dielectric properties, microwave dielectric materials have attracted more attention in passive electronic components, such as dielectric resonators, filters and other devices.4,5 Additionally, LTCC technology is favored by advanced products because of their high demands for miniaturization and hybrid integration for electronic systems. For instance, Guo et al.44–46 successfully lowered the sintering temperature of ceramics by ions substitution and addition of low melting point oxide. In order to co-fire with silver electrode, ceramics must be synthesized at low temperature, less than 960 °C.6,7 Hence, the aim of the present work is to decrease the sintering temperature of MgNb2O6 ceramics and then achieve excellent chemical compatibility with silver for the first time to satisfy the demands for LTCC applications. Generally, the sintering temperature of ceramic materials can be effectively reduced by the following methods: (1) use the raw materials with lower melting temperature, such as Bi2Mo2O9 ceramics.8 (2) Use ultrafine powders as raw materials to decrease the densification temperature.9 (3) Adopt proper synthesis process, such as co-precipitation, sol–gel and hydrothermal methods.10,11 (4) Use reasonable ion substitution.12,13 (5) Select appropriate low-melting glass or oxide to reduce the sintering temperature. The commonly used low-melting glasses are PbO–Bi2O3–B2O3–ZnO–TiO2, B2O3–CuO, Li2O–B2O3–SiO2–CaO–Al2O3.14,15
Binary magnesium niobate ceramics with rhombic columnar structure are widely investigated because of their excellent microwave dielectric properties.16,17 However, the pure phase MgNb2O6 ceramic is not easy to obtain, as reported by Liou et al.18 Pullar et al.19 reported that MgNb2O6 ceramic sintered at 1300 °C for 2 h exhibited remarkable microwave properties: εr = 19.9, Q·f = 79
600 GHz, τf = −64.9 ppm °C−1. Tian et al.20 reported that MgNb2O6 ceramic were not only successfully sintered at 1050 °C, but also achieved remarkable microwave properties: εr = 21.5, Q·f = 108
000 GHz, τf = −44 ppm °C−1 by adding CuO–B2O3 as sintering aid. However, the sintering temperature is still too high.
As known, the synthesis of pure-phase MgNb2O6 ceramics is not easy.23 In this work, pure-phase MgNb2O6 ceramic was synthesized successfully by optimizing process parameters. Such as, the slightly excessive MgO, reasonable extension of the milling time and increase in the pre-sintering time. George, Sumesh, et al.44 reported that the addition of LMZBS glass decreased the sintering temperature of Li2MgSiO4 ceramics from 1250 to 875 °C. Besides, the LMZBS glass melts to form a liquid phase and wet the grains of MgNb2O6, thereby promoting the densification of MgNb2O6.44 In addition, magnesium oxide in LMZBS glass can compensate for the volatilization of MgO in MgNb2O6, so LMZBS glass plays a great role in low-temperature sintering. In the present work, we have chosen LMZBS glasses as the firing aid to reduce the sintering temperature of MgNb2O6 ceramics. To our best knowledge, there's been no relevant report about the co-fired experiment of MgNb2O6 ceramics with silver electrodes. In this work, the chemical compatibility of MgNb2O6 ceramics with Ag electrodes have been studied for the first time. Meanwhile, the effects of LMZBS glass addition on the microstructure, density and dielectric properties of MgNb2O6 ceramics were also studied.
2. Experimental procedures
2.1. Materials preparation
MgNb2O6 ceramics were synthesized by solid-state process using high-purity MgO and Nb2O5 (all purity > 99.9%) as original materials were. The stoichiometric ratio of MgO and Nb2O5 was 1.01
:
1. (The slightly excessive MgO is beneficial to inhibit the production of the secondary phases Mg4Nb9O2 and Nb2O5
24,25). The powders were milled for 24 h, dried, and finally calcined at 1000 °C for 8 h. (An appropriate increase in the pre-sintering time is conducive to the formation of single-phase MgNb2O6 ceramic, and a reasonable extension of the milling time will increase the uniformity of particles and decrease particle size23,26). Li2O–MgO–ZnO–B2O3–SiO2 (LMZBS) glass was synthesized by quenching method according to the mole percentage of: Li2CO3
:
MgO
:
ZnO
:
B2O3
:
SiO2 = 20
:
20
:
20
:
20
:
20 (all purity > 99%). The LMZBS glass21,22 was melted and quenched at 900 °C. Then different weight percentages of LMZBS glasses (x = 0.1 wt%, 0.5 wt%, 1 wt%, 1.5 wt%, 2 wt%) were added to the calcined MgNb2O6 powders. After the second ball-milling for 12 h, the mixtures were dried, mixed with 10% PVB and then pressed into small cylinders 12 mm in diameter and 6 mm in height. Ultimately, the pressed cylinders pellets were sintered at 850–950 °C for 5 h.
2.2. Characterization analysis
The bulk density of all samples was measured by Archimedes method. The phase composition and crystal structure were analyzed by X-ray diffractometer (Philips X'Pert Pro MPD, The Netherlands). The scanning angle was ranged from 20° to 70°. The operating voltage and current of Cu Kα radiation were 40 kV and 30 mA, respectively. Raman spectroscopy was used to analyze (InVia, Renishaw, UK) the crystal structure of MgNb2O6 ceramic. The microstructure was studied detailed by scanning electron microscope (SEM, JSM-6490LV; JEOL, Japan).27 Moreover, the distribution of elements was collected by X-ray spectrometer (EDX, Genesis; EDAX, USA). The dielectric constant (εr) and quality factor (Q·f)28 were calculated by a network analyzer (N5230A, USA) combining the resonant cavity method and the Hakki–Coleman method in TE011 mode. The resonant frequency temperature coefficient (τf) was calculated as follows:29 |
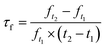 | (1) |
where t1 = 25 °C and t2 = 85 °C, corresponding resonance frequencies are ft1 and ft2, respectively.
3. Results and discussion
3.1. Crystal structure and phase composition
Fig. 1 indicates the XRD patterns of the MgNb2O6 ceramics with different LMZBS additions sintered at 925 °C for 5 h. All the ceramics samples show an orthorhombic columbite structure MgNb2O6 phase (PDF file no. 88-0708). By comparing the diffraction peaks with the standard PDF card, no second phase was found within the measurement error range of the instrument, indicating that pure phase MgNb2O6 ceramics were formed through slightly excessive MgO in the synthesis process and LMZBS glass had no significant effect on phase composition for MgNb2O6 ceramics.
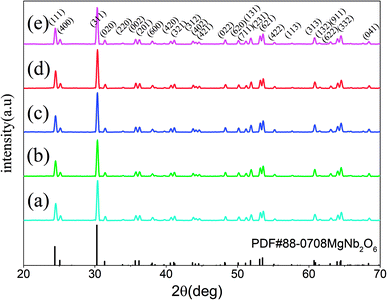 |
| Fig. 1 XRD patterns of MgNb2O6–x wt% LMZBS ceramics sintered at 925 °C for 5 h: (a) x = 0.1, (b) x = 0.5, (c) x = 1.0, (d) x = 1.5, and (e) x = 2.0. | |
Fig. 2 shows the Raman spectra of MgNb2O6 ceramics at 925 °C. Raman spectral is often used to study the crystal structure of materials. According to the symmetry of crystal, the Raman models are provided by the Bilbao Crystallographic Server for analysis:30
|
ΓRaman = 13Ag + 13Au + 14B1g + 14B1u + 13B2g + 13B2u + 14B3g + 14B3u
| (2) |
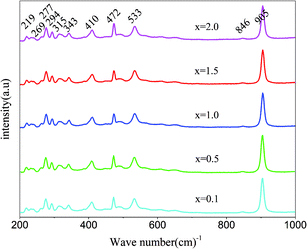 |
| Fig. 2 Raman spectra of the MgNb2O6–x wt% LMZBS (x = 0.1–2.0) ceramics sintered at 925 °C for 5 h. | |
Theoretically, MgNb2O6 ceramic have 108 vibration modes. However, only several Raman modes could be observed due to the interaction or overlapping. The bands in wavelength from 200 to 250 cm−1 were assigned to the twisting vibration in and between chains; the bands in wavelength from 250 to 400 cm−1 were attributed to the twisting vibration of octahedron; the bands in wavelength from 400 to 1000 cm−1 were related to the stretching vibration of Nb–O bonds. Weak peak 846 cm−1 corresponds to the antisymmetric stretching vibration mode of Nb–Ot of NbO6 octahedron in double chain plane (among them, Ot represents an oxygen atom linked to a Nb atom and two Mg atoms), this is consistent with the Raman spectrum reported by Y. C. You et al.31 It can be observed from Fig. 2 that no new Raman peak appears. By comparing the position of Raman peak, it is found that the additions of LMZBS have no influence on Raman spectrum.
3.2. Microstructure analysis
Fig. 3 and 4 show the SEM diagram and distribution of grain sizes of MgNb2O6 ceramics sintered at 925 °C. LMZBS glass has a remarkable influence on the average grain size and density of MgNb2O6 ceramics. In Fig. 3a, it can be intuitively seen that the grains are loose and irregular, and the average grain size was 0.68 μm, which is related to low amount of LMZBS glass. With the amount of LMZBS glass increasing, the grains started to grow, as shown in Fig. 3b. When the doping amount reaches 1 wt%, uniform and dense microstructure with clear grain boundaries could be observed. The average grain size reached to 1.72 μm and there was barely any hole. Because an appropriate amount of LMZBS glass will melt to form a liquid phase during sintering, which will make the grains of ceramic moist and thus promote the densification of ceramics. Nevertheless, with the further increase of doping amount, the grain grew abnormally and the average size of the grain is 1.48 μm. From Fig. 3e, abnormal grains growth can be obviously observed. The average grain size increased to 2.28 μm, and the grain boundary was indistinct, which may deteriorate the microwave dielectric characteristics. These results show that the proper amount of LMZBS glass can increase relative density and decrease porosity.
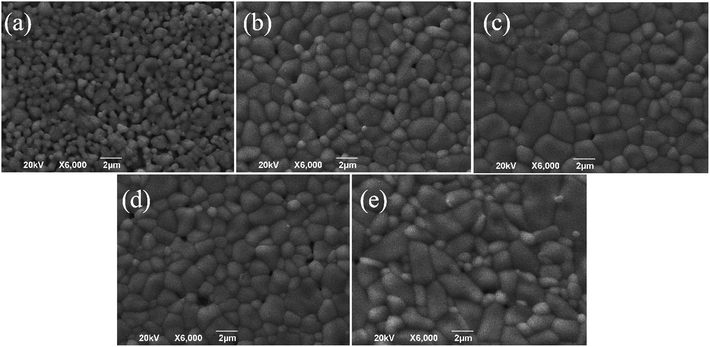 |
| Fig. 3 Surface SEM micrographs of MgNb2O6–x wt% LMZBS ceramics sintered at 925 °C (a) x = 0.1, (b) x = 0.5, (c) x = 1.0, (d) x = 1.5, (e) x = 2.0. | |
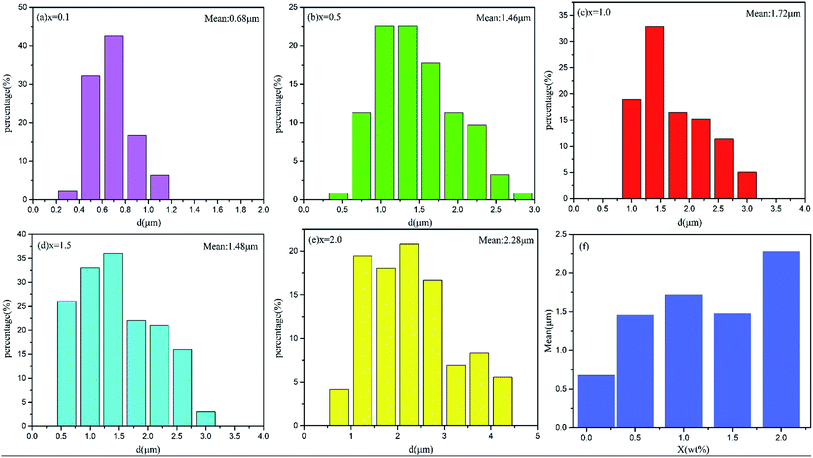 |
| Fig. 4 The grain sizes distribution of MgNb2O6–x wt% LMZBS (x = 0.1, 0.5, 1.0, 1.5 and 2.0) ceramics sintered at 925 °C. (a–e) Grain sizes distribution, (f) mean grain size. | |
3.3. Dielectric characteristics analysis
Fig. 5 shows the apparent density of MgNb2O6 ceramic with different doping amounts at different sintering temperatures. When the doping amount ranged from 0.1 wt% to 1.0 wt%, the density increased under same sintering temperature.32 Finally, MgNb2O6 ceramic with 1.0 wt% LMZBS glass at 925 °C had the highest apparent density. This is attributed to the increase in liquid phase. However, with the further increase of the doping amount, the apparent density decreased slightly. This was because the excessive LMZBS addition leaded to excessive liquid phase, resulting in abnormal grain growth and porosity, which eventually leaded to the decrease in the density of MgNb2O6 ceramic.
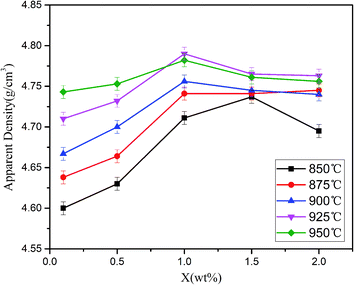 |
| Fig. 5 Apparent densities of MgNb2O6–x wt% LMZBS (0.1 ≤ x ≤ 2.0) ceramics sintered at different temperatures. | |
According to Fig. 6, it can be observed that when the doping amount is in the range of 0.1 wt% to 1.0 wt%, the dielectric constant is related to the doping proportion exhibiting a similar tendency as the apparent density. As known, second phase, molecular volume, structural characteristics, density and ionic polarizability affect the dielectric constant.33,34 As shown in Fig. 1, there is no impurity in the ceramic system, so the influence of the second phase on the dielectric constant can be excluded. In this paper, apparent density is the primary factor impacting the dielectric constant. High density means low porosity and large dielectric constant. Therefore, dielectric constant is related to the density.
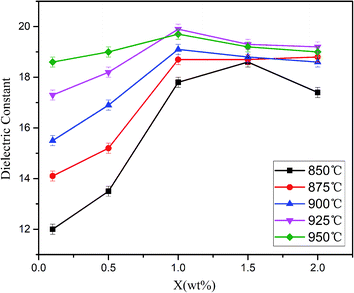 |
| Fig. 6 The dielectric constant of MgNb2O6–x wt% LMZBS (0.1 ≤ x ≤ 2.0) ceramics sintered at various temperatures for 5 h. | |
Fig. 7 demonstrates the Q·f value of MgNb2O6 ceramic under different sintering temperatures and different LMZBS amounts. The Q·f value increased firstly until reaching the peak and decreased with the amount of LMZBS glass gradually increasing. Samples with same LMZBS amount got different Q·f at different temperature and we can see that ceramic got the highest Q·f at 925 °C. Besides, for the samples sintered at 925 °C with 1.0 wt% LMZBS glass, the optimal Q·f value was 67
839 GHz. The results demonstrate that 925 °C is the optimization sintering temperature for MgNb2O6 ceramic in terms of densification and dielectric performance. In general, the dielectric loss is mainly composed of two modules: internal loss and external loss. Among them, the intrinsic loss is difficult to avoid, because it is the inherent nature of the material. However, external losses are affected by various factors, including second phase, porosity, grain boundaries, average grain size, and microstructure defects, etc.35,36 XRD patterns show that there is no second phase, so the influence of secondary phase on Q·f can be excluded. In this work, microwave dielectric loss is mainly affected by apparent density. With the increase of doping amount, the variation in Q·f value are roughly similar to the change of apparent density. This is because the Q·f value is impacted by the density.37,38 Therefore, the proper amount of LMZBS glass can effectively improve the Q·f value of MgNb2O6 ceramic, which can make the dielectric ceramics have higher relative density and lower porosity.
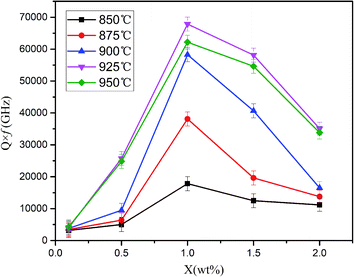 |
| Fig. 7 The Q·f values of MgNb2O6–x wt% LMZBS (0.1 ≤ x ≤ 2.0) ceramics sintered at various temperatures. | |
Fig. 8 exhibits the τf of MgNb2O6–x wt% LMZBS (x = 0.1, 0.5, 1.0, 1.5 and 2.0) ceramics at optimal temperatures. It can be found that with the increase of glass addition, τf value ranged from −50.7 ppm °C−1 to −41.01 ppm °C−1. In general, the smaller the |τf|, the better the thermal stability of dielectric ceramics.39 In this work, the phase composition and crystal structure had no significant effect on τf value. Therefore, the change of τf value can be attributed to famous Lichtenecker empirical logarithmic rule:40
where
V1,
V2 and
τf1,
τf2 are the volume fractions and
τf values of pure MgNb
2O
6 and LMZBS, respectively (
V1 +
V2 = 1). On the one hand,
τf value of pure MgNb
2O
6 ceramic is −64.9 ppm °C
−1.
19 On the other hand, low-melting glass LMZBS have negative
τf value,
41 which may explain the reason why the
τf value changed slightly. Adding
τf compensation materials have proved to be an effective method to adjust the
τf to near zero. Therefore, in the next step, we may choose the CaTiO
3 (+800 ppm °C
−1) and SrTiO
3 (+1600 ppm °C
−1) to adjust
τf for the MgNb
2O
6 ceramic.
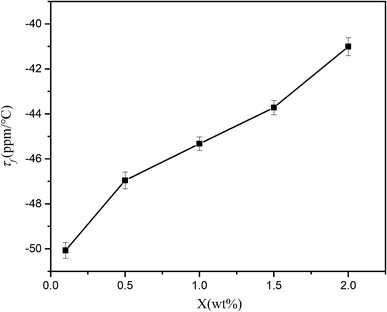 |
| Fig. 8 τf values for the MgNb2O6–x wt% LMZBS ceramics sintered at 925 °C. | |
It is imperative to research the chemical compatibility of the MgNb2O6 ceramics with silver for practical LTCC applications. Fig. 9a exhibits the XRD pattern of the 1.0 wt% LMZBS-doped MgNb2O6 ceramics co-fired with 20 wt% Ag powders sintered at 925 °C. No chemical reaction occurred between MgNb2O6 ceramics and Ag. Fig. 9b–d show the back scattered electron images and elements distribution of the fracture surface. The EDX analysis proves that there is no reaction between ceramic and silver. The whole experimental results confirm that the 1.0 wt% LMZBS-doped MgNb2O6 ceramic can be compatible with Ag. Besides, the comparison between this work and previous literature is listed in Table 1. It can be observed that the MgNb2O6 ceramics doped with LMZBS exhibited chemical compatibility with silver electrodes without deteriorating microwave dielectric properties, which makes MgNb2O6 ceramics as promising material for LTCC applications.
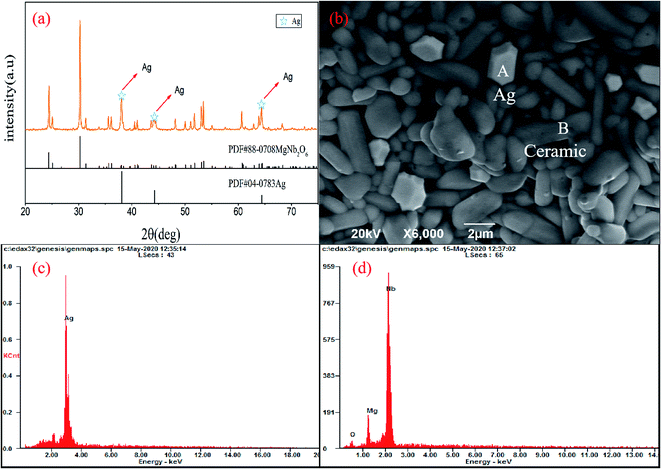 |
| Fig. 9 XRD, SEM and EDX analysis of MgNb2O6 ceramics doped with 1.0 wt% LMZBS and co-fired with 20 wt% Ag at 925 °C for 5 h. (a) XRD pattern, (b) back scattered electron images, (c) EDX analysis on A spot, and (d) EDX analysis on B spot. | |
Table 1 Comparison of microwave dielectric properties among MgNb2O6 ceramics
Material |
Dopant |
Temperature (°C) |
εr |
Q·f (GHz) |
τf (ppm) |
Co-fired with Ag |
References |
MgNb2O6 |
— |
1300 |
19.9 |
79 600 |
−64.9 |
No |
Pullar et al.19 |
MgNb2O6 |
B2O3 |
1260 |
21.5 |
115 800 |
−48 |
No |
Huang et al.42 |
MgNb2O6 |
CuO–B2O3 |
1050 |
21.5 |
108 000 |
−44 |
No |
Tian Z. Q. et al.20 |
MgNb2O6 |
Fe2O3 |
1140 |
20.5 |
70 000 |
−49 |
No |
Hsu et al.43 |
MgNb2O6 |
LMZBS |
925 |
19.7 |
67 839 |
−41.01 |
Yes |
This work |
4. Conclusions
MgNb2O6 ceramics doped with LMZBS glass were prepared by solid phase reaction process. The effects of LMZBS amount on the phase composition, microstructure, grain growth, apparent density, chemical compatibility with Ag and microwave properties of ceramics were studied. Raman spectra and XRD demonstrate single columbite MgNb2O6 phase. The scanning electron microscope (SEM) results indicated that the homogeneous and dense microstructure appeared at 925 °C. The relative density and εr have similar variation tendency. With the increase of LMZBS amount, τf value shifted towards positive direction. Particularly, the MgNb2O6–1.0 wt% LMZBS ceramic obtained at 925 °C possessed optimum microwave dielectric characteristics: εr = 19.7, Q·f = 67
839 GHz, τf = −41.01 ppm °C−1. Meanwhile, XRD pattern and EDX elements analysis proved that the MgNb2O6 ceramic showed excellent chemical compatibility with Ag, which confirmed the applicability of MgNb2O6 ceramic in LTCC.
Conflicts of interest
There are no conflicts to declare.
References
- Q. Liao, L. Li, X. Ren, X. Yu, D. Guo and M. Wang, A low sintering temperature low loss microwave dielectric material ZnZrNb2O8, J. Am. Ceram. Soc., 2012, 95(11), 3363–3365 CrossRef CAS.
- M. T. Sebastian and H. Jantunen, High Temperature Cofired Ceramic (HTCC), Low Temperature Cofired Ceramic (LTCC), and Ultralow Temperature Cofired Ceramic (ULTCC) Materials, Microwave Materials and Applications 2V Set, 2017, pp. 355–425 Search PubMed.
- W. Wang, L. Li, S. Xiu, B. Shen and J. Zhai, Microwave dielectric properties of (Mg0.4Zn0.6)2SiO4–CaTiO3 ceramics sintered with Li2CO3–H3BO3 for LTCC technology, J. Alloys Compd., 2015, 639, 359–364 CrossRef CAS.
- G. Wang, H. Zhang, C. Liu, H. Su, L. Jia, J. Li and G. Gan, Low-Temperature Sintering Li3Mg1.8Ca0.2NbO6 Microwave Dielectric Ceramics with LMZBS Glass, J. Electron. Mater., 2018, 47(8), 4672–4677 CrossRef CAS.
- D. Zhou, L. X. Pang, D. W. Wang, C. Li, B. B. Jin and I. M. Reaney, High permittivity and low loss microwave dielectrics suitable for 5G resonators and low temperature co-fired ceramic architecture, J. Mater. Chem. C, 2017, 5(38), 10094–10098 RSC.
- H. Ren, M. Dang, H. Wang, T. Xie, S. Jiang, H. Lin and L. Luo, Sintering behavior and microwave dielectric properties of B2O3-La2O3-MgO-TiO2 based glass-ceramic for LTCC applications, Mater. Lett., 2018, 210, 113–116 CrossRef CAS.
- G. G. Yao, P. Liu and H. W. Zhang, Novel Series of Low-Firing Microwave Dielectric Ceramics: Ca5A4(VO4)6(A2+ = Mg, Zn), J. Am. Ceram. Soc., 2013, 96(6), 1691–1693 CrossRef CAS.
- H. Zhou, H. Wang, Y. Chen, K. Li and X. Yao, Low temperature sintering and microwave dielectric properties of Ba3Ti5Nb6O28 ceramics with BaCu(B2O5) additions, Mater. Chem. Phys., 2009, 113(1), 1–5 CrossRef CAS.
- J. Xiang, Z. Xie, Y. Huang and H. Xiao, Synthesis of Ti(C, N) ultrafine powders by carbothermal reduction of TiO2 derived from sol–gel process, J. Eur. Ceram. Soc., 2000, 20(7), 933–938 CrossRef CAS.
- Y. S. Hong, H. B. Park and S. J. Kim, Preparation of Pb(Mg13Nb23)O3 powder using a citrate-gel derived columbite MgNb2O6 precursor and its dielectric properties, J. Eur. Ceram. Soc., 1998, 18(6), 613–619 CrossRef CAS.
- S. Ananta, Phase and morphology evolution of magnesium niobate powders synthesized by solid-state reaction, Mater. Lett., 2004, 58(22–23), 2781–2786 CrossRef CAS.
- G. Wang, H. Zhang, X. Huang, F. Xu, G. Gan, Y. Yang and L. Jin, Correlations between the structural characteristics and enhanced microwave dielectric properties of V–modified Li3Mg2NbO6 ceramics, Ceram. Int., 2018, 44(16), 19295–19300 CrossRef CAS.
- G. Wang, H. Zhang, C. Liu, H. Su, J. Li, X. Huang and F. Xu, Low temperature sintering and microwave dielectric properties of novel temperature stable Li3Mg2NbO6-0.1TiO2 ceramics, Mater. Lett., 2018, 217, 48–51 CrossRef CAS.
- Y. Liao, F. Xu, D. Zhang, T. Zhou, Q. Wang, X. Wang and H. Zhang, Low temperature firing of Li0.43Zn0.27Ti0.13Fe2.17O4 ferrites with enhanced magnetic properties, J. Am. Ceram. Soc., 2015, 98(8), 2556–2560 CrossRef CAS.
- E. A. Nenasheva and N. F. Kartenko, Low-sintering ceramic materials based on Bi2O3–ZnO–Nb2O5 compounds, J. Eur. Ceram. Soc., 2006, 26(10–11), 1929–1932 CrossRef CAS.
- G. Wang, D. Zhang, Y. Lai, X. Huang, Y. Yang, G. Gan and L. Jin, Ultralow loss and temperature stability of Li3Mg2NbO6-xLiF ceramics with low sintering temperature, J. Alloys Compd., 2019, 782, 370–374 CrossRef CAS.
- R. C. Pullar, The synthesis, properties, and applications of columbite niobates (M2+ Nb2O6): a critical review, J. Am. Ceram. Soc., 2009, 92(3), 563–577 CrossRef CAS.
- Y. C. Liou, M. H. Weng and C. Y. Shiue, CaNb2O6 ceramics prepared by a reaction-sintering process, Mater. Sci. Eng., B, 2006, 133(1–3), 14–19 CrossRef CAS.
- R. C. Pullar, J. D. Breeze and N. M. Alford, Characterization and microwave dielectric properties of M2+ Nb2O6 ceramics, J. Am. Ceram. Soc., 2005, 88(9), 2466–2471 CrossRef CAS.
- Z. Q. Tian and L. Lin, Low temperature sintering and microwave dielectric properties of MgNb2O6 ceramics, J. Mater. Sci.: Mater. Electron., 2009, 20(9), 867–871 CrossRef CAS.
- G. Wang, H. Zhang, C. Liu, H. Su, L. Jia, J. Li and G. Gan, Low-Temperature Sintering Li3Mg1.8Ca0.2NbO6 Microwave Dielectric Ceramics with LMZBS Glass, J. Electron. Mater., 2018, 47(8), 4672–4677 CrossRef CAS.
- K. Manu and M. T. Sebastian, Tape casting of low permittivity Wesselsite–Glass composite for LTCC based microwave applications, Ceram. Int., 2016, 42(1), 1210–1216 CrossRef CAS.
- K. N. Singh and P. K. Bajpai, Synthesis, characterization and dielectric relaxation of phase pure columbite MgNb2O6: optimization of calcination and sintering, Phys. B, 2010, 405(1), 303–312 CrossRef CAS.
- K. Sreedhar and A. Mitra, Formation of lead magnesium niobate perovskite from MgNb2O6 and Pb3Nb2O8 precursors, Mater. Res. Bull., 1997, 32(12), 1643–1649 CrossRef CAS.
- A. Belous, O. Ovchar, B. Jancar and J. Bezjak, The effect of non-stoichiometry on the microstructure and microwave dielectric properties of the columbites A2+Nb2O6, J. Eur. Ceram. Soc., 2007, 27(8–9), 2933–2936 CrossRef CAS.
- S. Ananta, R. Brydson and N. W. Thomas, Synthesis, formation and characterisation of MgNb2O6 powder in a columbite-like phase, J. Eur. Ceram. Soc., 1999, 19(3), 355–362 CrossRef CAS.
- G. Wang, D. Zhang, X. Huang, Y. Rao, Y. Yang, G. Gan and C. Liu, Crystal structure and enhanced microwave dielectric properties of Ta5+ substituted Li3Mg2NbO6 ceramics, J. Am. Ceram. Soc., 2020, 103(1), 214–223 CrossRef CAS.
- G. Wang, D. Zhang, F. Xu, X. Huang, Y. Yang, G. Gan and L. Jin, Correlation between crystal structure and modified microwave dielectric characteristics of Cu2+ substituted Li3Mg2NbO6 ceramics, Ceram. Int., 2019, 45(8), 10170–10175 CrossRef CAS.
- X. Shi, H. Zhang, D. Zhang, F. Xu, Y. Zheng, G. Wang and L. Jia, Correlation between structure characteristics and dielectric properties of Li2Mg3-xCuxTiO6 ceramics based on complex chemical bond theory, Ceram. Int., 2019, 45(17), 23509–23514 CrossRef CAS.
- E. Kroumova, M. I. Aroyo, J. M. Perez-Mato, A. Kirov, C. Capillas, S. Ivantchev and H. Wondratschek, Bilbao crystallographic server: useful databases and tools for phase-transition studies, Phase Transitions A Multinatl. J., 2003, 76(1–2), 155–170 CrossRef CAS.
- Y. C. You, H. L. Park, Y. G. Song, H. S. Moon and G. C. Kim, Stable phases in the MgO-Nb2O5 system at 1250°C, J. Mater. Sci. Lett., 1994, 13(20), 1487–1489 CrossRef CAS.
- G. Wang, D. Zhang, G. Gan, Y. Yang, Y. Rao, F. Xu and L. Jin, Synthesis, crystal structure and low loss of Li3Mg2NbO6 ceramics by reaction sintering process, Ceram. Int., 2019, 45(16), 19766–19770 CrossRef CAS.
- Q. Liao, L. Li, X. Ren, X. Yu, Q. Meng and W. Xia, A new microwave dielectric material Ni0.5Ti0.5NbO4, Mater. Lett., 2012, 89, 351–353 CrossRef CAS.
- E. S. Kim, D. H. Kang, J. M. Yang, H. S. Shin, N. I. Zahari and H. Ohsato, Crystal structure and dielectric properties of Ca0.85Nd0.1TiO3-LnAIO3 ceramics, IEEE Trans. Ultrason. Ferroelectrics Freq. Contr., 2008, 55(5), 1075–1080 Search PubMed.
- M. Guo, S. Gong, G. Dou and D. Zhou, A new temperature stable microwave dielectric ceramics: ZnTiNb2O8 sintered at low temperatures, J. Alloys Compd., 2011, 509(20), 5988–5995 CrossRef CAS.
- S. J. Penn, N. M. Alford, A. Templeton, X. Wang, M. Xu, M. Reece and K. Schrapel, Effect of porosity and grain size on the microwave dielectric properties of sintered alumina, J. Am. Ceram. Soc., 1997, 80(7), 1885–1888 CrossRef CAS.
- S. H. Yoon, G. K. Choi, D. W. Kim, S. Y. Cho and K. S. Hong, Mixture behavior and microwave dielectric properties of (1−x) CaWO4–xTiO2, J. Eur. Ceram. Soc., 2007, 27(8–9), 3087–3091 CrossRef CAS.
- Z. Fang, B. Tang, F. Si, E. Li, H. Yang and S. Zhang, Phase evolution, structure and microwave dielectric properties of Li2+xMg3SnO6 (x= 0.00–0.12) ceramics, Ceram. Int., 2017, 43(16), 13645–13652 CrossRef CAS.
- P. Zhang, M. Yang and M. Xiao, Sintering behavior, crystalline structure and microwave dielectric properties of Li2(Ni1-xMgx)3TiO6 (0≤x≤1) ceramics, Ceram. Int., 2018, 44(17), 21607–21612 CrossRef CAS.
- L. X. Pang, H. Wang, D. Zhou and X. Yao, A new temperature stable microwave dielectric with low-firing temperature in Bi2MoO6–TiO2 system, J. Alloys Compd., 2010, 493(1–2), 626–629 CrossRef CAS.
- T. Joseph, M. T. Sebastian, H. Sreemoolanadhan and V. K. Sree Nageswari, Effect of glass addition on the microwave dielectric properties of CaMgSi2O6 ceramics, Int. J. Appl. Ceram. Technol., 2010, 7, E98–E106 CrossRef.
- C. L. Huang and K. H. Chiang, Improved high-Q microwave dielectric material using B2O3-doped MgNb2O6 ceramics, Mater. Sci. Eng., A, 2008, 474(1–2), 243–246 CrossRef.
- C. H. Hsu, C. F. Tseng and C. L. Huang, Microwave dielectric properties of MgNb2O6 ceramics with Fe2O3 additives, Jpn. J. Appl. Phys., 2005, 44(11R), 8043 CrossRef CAS.
- S. George, P. S. Anjana, V. N. Deepu, P. Mohanan and M. T. Sebastian, Low-temperature sintering and microwave dielectric properties of Li2MgSiO4 ceramics, J. Am. Ceram. Soc., 2009, 92(6), 1244–1249 CrossRef CAS.
- H. H. Guo, D. Zhou, C. Du, P. J. Wang, W. F. Liu, L. X. Pang and S. Trukhanov, Temperature stable Li2Ti0.75 (Mg1/3Nb2/3)0.25O3-based microwave dielectric ceramics with low sintering temperature and ultra-low dielectric loss for dielectric resonator antenna applications, J. Mater. Chem. C, 2020, 8(14), 4690–4700 RSC.
- H. H. Guo, D. Zhou, L. X. Pang and Z. M. Qi, Microwave dielectric properties of low firing temperature stable scheelite structured (Ca, Bi)(Mo, V) O4 solid solution ceramics for LTCC applications, J. Eur. Ceram. Soc., 2019, 39(7), 2365–2373 CrossRef CAS.
|
This journal is © The Royal Society of Chemistry 2020 |