DOI:
10.1039/D0RA04568C
(Paper)
RSC Adv., 2020,
10, 25669-25678
Magnetic phase transition from paramagnetic in Nb2AlC-MAX to superconductivity-like diamagnetic in Nb2C-MXene: an experimental and computational analysis
Received
23rd May 2020
, Accepted 25th June 2020
First published on 7th July 2020
Abstract
Transition metal carbides (TMCs) have recently emerged as competent members among the family of two-dimensional (2D) materials, owing to their promising applications. There are many promising applications of MXenes; however, their magnetic properties lack a wide margin, both experimentally as well as theoretically, which needs to be investigated for potential use in spintronics. In this study, we carried out a comprehensive etching process via selective extraction of Al layers from Nb2AlC-MAX using a wet electrochemical route under well-optimized conditions to obtain fine 2D-Nb2C MXene sheets. Structural analysis using X-ray diffraction (XRD) confirms the effective removal of Al followed by confirmation of a 2D layered structure from morphological analysis using scanning electron microscopy (SEM). Zero-field-cooled (ZFC) and field-cooled (FC) measurements of MAX and MXene at different field strengths were performed using a superconducting quantum interference device (SQUID). Magnetic measurements reveal the paramagnetic nature of Nb2AlC-MAX measured under 5 mT; however, this changes to a clear superconductor-like diamagnetic behavior with a shift of the magnetization from positive to negative values at low temperatures when measured under 5 mT and 10 mT for Nb2C MXene. The diamagnetism, however, is changed to paramagnetism at 100 mT, which shows the existence of critical fields known typically for a type-II superconductor. To gain an insight into this unusual behavior in MXene, density functional theory (DFT) first-principles calculation was also performed in Wein2K software using spin-polarized generalized gradient approximation (sp-GGA). The magnetic moment of the compound is calculated to be negative, which corresponds well with the experimental finding and suggests that the negative magnetic moment originated from the d-orbital of Nb2C. The present report provides a pathway to deeply understanding the existence of superconductivity-like diamagnetic behavior in Nb2C MXene, which is useful for future magnetic applications.
In two-dimensional (2D) materials, magnetism at the nanoscale is at the forefront of many cutting-edge technological applications, such as spintronic devices. Naguib et al. have synthesized a new class of two-dimensional materials, known as MXenes (MnXn+1), discovered in 2011, giving a possibility of magnetism in such 2D materials and their promising uses in spintronic devices.1 These 2D layer structured early transition metal carbides and/or nitrides are known as MXenes, so named to indicate their structural similarities with graphene.2 MXenes are derived from 3D MAX phases (space group P63/mmc) in which “M” is an early transition metal, e.g., Ti, Ta, V, etc.; “A” is mainly a group 13 or group 14 element (group III-A or IV-A), e.g., Si, Al; “X” is a carbide, nitride or can be both; and n = 1, 2, 3 represents the number of layers, forming 211, 312 and 413 phases.3–5 Over the past decade, this new material has gained enormous attention, thus developing an entirely new research field to disclose the properties of the 2D state of this material. The materials in the 2D regime own a cluster of astonishing physical properties as compared to the 3D nature, but intrinsic two-dimensional magnetism has proved to be challenging. As 2D semiconductors have revamped the field of electronics, similarly, magnetism in 2D materials could remodel spintronic devices that can employ a spin degree of freedom.6,7
Nb2C MXene was first synthesized by Naguib et al., but they just reported its electrochemical activity as a promising electrode material.4 Further work has been reported in Nb2C with various biomedical applications, energy storage, supercapacitors, and nanoelectronics.9–19 As far as the magnetism in such 2D MXenes is concerned, it remains less investigated, and this research void needs to be filled. Recently, Babar et al. reported the observation of superconductivity in as-prepared powdered Nb2C for the first time, with the highest onset transition temperature Tc,onset = 12.5 K among the MXene family. However, the authors did not discuss the magnetic nature of the parent Nb2AlC MAX itself and did not reason for the presence of unusual magnetic effects in MXene.8 MXenes are favorable members of 2D magnetism, and different magnetic natures are computationally predicted in various carbide and nitride MXenes.7,20 The existence of novel room-temperature ferromagnetic order in doped MXene and the coexistence of different magnetic phases in MXene, along with experimental evidence, indicate its potential of hosting diverse magnetic natures.21,22 Considerable research has been focused on these 2D structures due to their importance and favorable applications, such as spintronics. MXene could provide a vast platform for exploring the magnetic properties and is one of the best candidates that can host superconductivity as compared to other members of the 2D family. Experimental studies are generally dependent upon numerous variables, thus affecting the research pace. However, density functional theory-based first principles calculation and theoretical simulations are a successful way to examine and foresee the properties of low-dimensional materials. This provoked us to theoretically explore superconductivity in Nb2C and their validation through superconductivity measurements of experimentally synthesized Nb2C MXene. In this work, we report a systematic etching mechanism of Nb2C MXene to obtain fine 2D sheets via a chemical etching route using hydrofluoric (HF) acid. Structural and morphological studies using the X-ray diffraction technique (XRD), scanning electron microscopy (SEM) and elemental analysis by energy-dispersive X-ray spectroscopy (EDX) show the effective removal of Al from the parent 3D-Nb2AlC MAX, thus revealing an accordion-like sheet structure. Optical analysis indicates a significant reduction in bandgap after chemical etching. Magnetic properties were carried out to observe the signatures of superconductivity (a perfect diamagnetic state, negative magnetic moment) and its magnetic nature at room temperature. To study the magnetic nature of as-prepared powder-form Nb2C, density functional theory (DFT) first principles calculation was carried out through Wein2K using spin-polarized generalized gradient approximation (sp-GGA). The magnetic moment of the compound is calculated to be −0.00485, which although but small is important, as the value is negative, which is an indication of the presence of diamagnetism in Nb2C. Here, the detailed chemical etching process, magnetic properties of Nb2AlC MAX and its effect on magnetic phase of Nb2C MXene, and the density functional theory calculation are reported, which were not discussed by Babar et al. in ref. 8.
Results and discussion
The structural and phase purity of resultant MXene powder was characterized by X-ray diffractometer (XRD, Bruker, D8 Advance, Germany) using Cu-Kα radiation. X-ray diffraction (XRD) patterns of Nb2AlC MAX and 2D layered MXene are shown (see Fig. 2a and b). There are two main peaks in the XRD pattern of MAX, the first peak at θ = 12.9° and the second peak at θ = 38.8°. After chemical treatment, the total disappearance of the second peak indicates the removal of Al from the parent compound.
The broadening of the peak at θ = 12.9° and its downshifting indicates that MAX is converted into MXene. Middle Al layers can be selectively removed due to the weaker nature of Nb–Al bonds than the Nb–C bond in the Nb2AlC MAX phase after high-temperature treatment with hydrofluoric acid.4,23 Weaker hydrogen bonding in M–Al layers comes in place of stronger metallic bonds that result in facile separation of sheets upon HF treatment and subsequent intercalation of water molecules during the washing process. These different relative bond strengths allow the selective removal of Al without affecting the M–C layer,8,23,24 resulting in hexagonally stacked 2D sheets of Nb2C MXene. The elemental composition, obtained from energy-dispersive X-ray spectrum (EDX), is shown in the table provided in Fig. 2. The EDX elemental mapping shows a significant reduction of Al content after HF treatment, showing the successful etching process. Both O and F are also present in MXene as functional groups, which are unavoidable during the synthesis process. It is worth noting that aluminum in Nb2AlC is very active and could easily adsorb oxygen. After the etching, Al is dissolved, and oxygen contents associated with Al are therefore decreased. The high content of O in MAX phases may be attributed to several reasons. Oxygen contaminations in primary precursors (i.e., metal powders) emanate oxygen impurity phases such as alumina.25 Experimental studies on similar MAX phases reveal that oxygen could possibly substitute carbon without affecting the MAX structure.26 J. Rosen et al. have reported oxygen as a potential element in comparison to nitrides or carbides that could dictate the carbide/nitride lattice sites.27 T. Liao et al. have also indicated that the treatment of MAX at high-temperature conditions could trigger oxygen to reside at c-sites.28 They reported 50% of carbon being replaced by oxygen content. Moreover, due to the high affinity of aluminum towards oxygen, weighing the initial precursors in an open environment or MAX precursors may lead to the formation of Al2O3 even if the synthesis is carried out in vacuum.28,29 The results also suggest that the formation of such impurities unintentionally incorporate significant oxygen content. Elevated temperature treatment of MAX phase with strong HF etchant leads to the removal of Al and its associated content, resulting in a more ordered structure.30
To observe the morphology of the sheets, scanning electron microscope (SEM) images at different resolutions were recorded using a field emission electron microscope (FESEM, VEGA3-TSCAN) operated at 20 kV. Structural morphological images, determined by scanning electron microscope (SEM) of MAX and MXene, are shown in Fig. 2c and d, respectively, showing a layered structure of the resultant MXene compound having a typical MXene morphology.31–35 Transmission electron microscopy (TEM) images of Nb2C-MXene are shown in Fig. 2e, clearly revealing the typical MXene layered structure. The exfoliated 2D-Nb2C MXene sheets are transparent and quite thin, as observed by TEM imaging. The variation of c-LP of MAX to MXene is presented in Fig. 1b as c-LP was increased to 22.6 Å from 13.83 Å (MAX phase), which satisfies the facile separation of the sheets after HF treatment.4,36,37
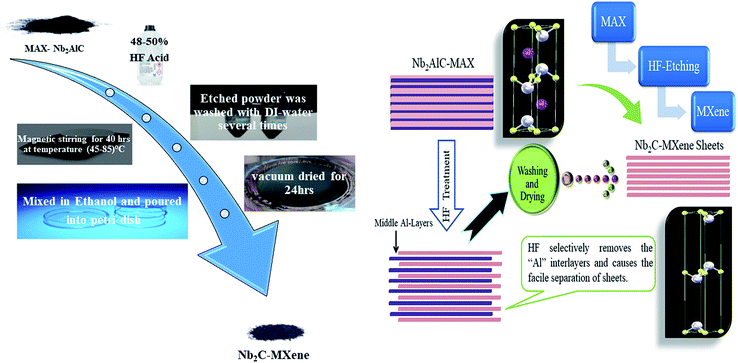 |
| Fig. 1 Detailed synthesis schematics and etching mechanism to obtain 2D Nb2C-MXene sheets. | |
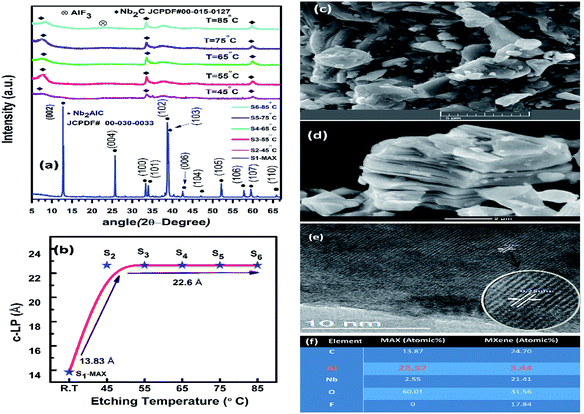 |
| Fig. 2 (a) XRD pattern of S1 (pure MAX), S2 (MXene etched at 45 °C), S3 (55 °C), S4 (65 °C), S5 (75 °C), S6 (85 °C). (b) Variation of c-LP of MAX and MXene for different sample series. (c and d) SEM images of MAX and MXene. (e) TEM image of Nb2C MXene flakes showing typical layered structure. (f) Table showing the atomic percentage of elemental composition of MAX and MXene obtained from EDX. | |
Raman spectra were measured with Horiba Scientific, Xplora Raman analyzer with a laser wavelength of 532 nm in the region of 200–2500 cm−1. Fig. 3a and b shows the Raman of Nb2AlC before and after HF treatment done at various temperatures. The maximum number of peaks is downshifted, broadened, and shifted to higher wavenumber after terminating with F and OH groups, which indicates a strengthening of the bond between atoms.38–41 Peaks I and II in MAX are suppressed after HF treatment, indicating the removal of Al atoms or exchange of Al atoms by lighter atoms.42 Peak III was broadened and downshifted, which is related to C atoms.43 Peaks IV and V are representative of D and G bands of carbon species. The D band characterizes carbon disordered structure to sp3 hybrid carbon, and G is the graphitic band or sp2 carbon.44 The ratio of intensity of ID to IG tells us about the crystallinity defects, showing more ordered MXene phase than the MAX phase.45,46 The ID/IG ratio of intensities in Fig. 3c tells us about the crystalline defects, which shows more ordered MXene phases than the MAX itself. This ratio is 1.6 for MAX, and it varies between 0.89 to 1.33 for MXene at different temperatures.45 This shows that the sample obtained and carried forward at 55 °C is more ordered, with less defect density as compared to the other samples synthesized at different temperatures.
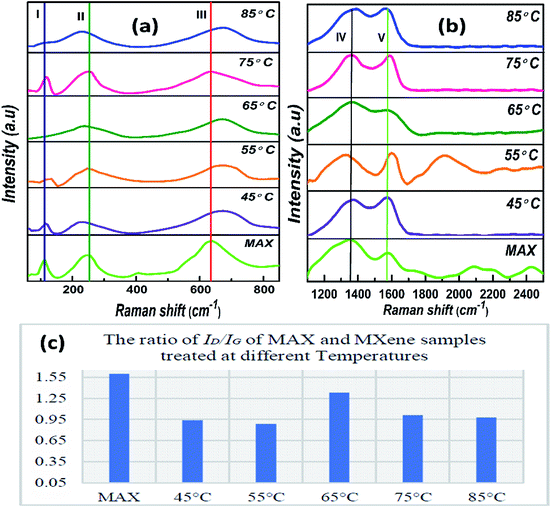 |
| Fig. 3 (a) Raman spectra of (S1) MAX and (S2) MXene at 45 °C, (S3) 55 °C, (S4) 65 °C, (S5) 75 °C, and (S6) 85 °C, (b) D and G-bands, (c) ID/IG ratio of MAX and MXene at different temperatures. | |
The Fourier transform infrared (FTIR) spectra of Nb2C etched at different temperatures, i.e., 55 °C, 65 °C, 75 °C and 85 °C, and are compared to the FTIR spectrum of pure MAX (Fig. 4a), indicating three absorption bands at 2357 cm−1, 2295 cm−1, and 483 cm−1, respectively, which are common and appear in all samples. These bonds can be attributed to the triply bonded carbons, Nb
C
Nb, and Nb–C, respectively.47 It is to be noted that the FTIR spectra of Nb2C is irrelevant to the etched temperature and shows a similar trend. A bond at 1643 cm−1 can be attributed to unsaturation mainly due to the C
C bond in the MXene structure. The presence of C–H bonds can also be indicated by the bands at 2947 cm−1 and 1363 cm−1. Based on the spectra and presence of different functional groups, surface functionalization can be observed in our MXene sheets due to the presence of a distinct C
O band at 1734 cm−1 and C–F band at 1211 cm−1.48,49 The absence of these bonds in the spectrum of the MAX phase shows that the etched MXene may have C
O, C–F, and O–H surface terminations.50
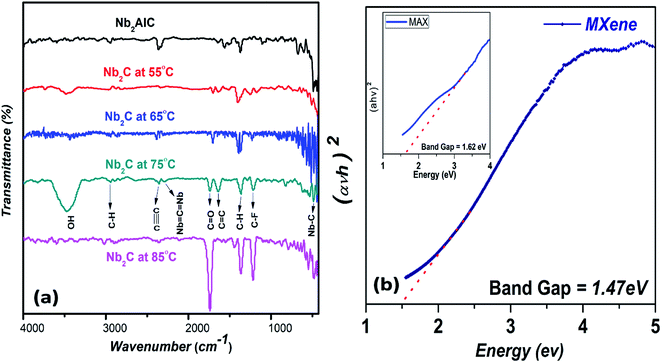 |
| Fig. 4 (a) Fourier transform infrared (FTIR) spectra of MAX and Nb2C-MXene synthesized at different conditions. (b) Band gap analysis of MAX and MXene. | |
Tauc's plot was used to calculate the band gap of MAX and MXene using following equation:
where
h = Planck's constant,
A = constant,
ν = the frequency of irradiated light,
Eg = the bandgap energy, and
α = the absorbance of light.
51 The bandgaps of MAX and MXene were obtained by plotting (
αhν)
2 against bandgap energy
Eg.
52–56 The bandgaps of MAX and MXene came out to be 1.62 eV and 1.47 eV, respectively, as shown in
Fig. 4b. The reduction in the bandgap from MAX to MXene can be attributed to the presence of different surface terminations (with O, OH, and F), with bandgaps ranging from 0.45 eV to 1.8 eV.
4,57–60
The magnetic nature of MXene has remained a less-explored research field, with only a few reports available on its magnetic nature. Their magnetic nature is predicted based on the magnetic transition metal elements (e.g., Cr, Mn, V, Fe, and Ni) or in the form of any doped configurations. There exists a large gap between experimental studies and their theoretical verification, or vice versa. Due to limitations in synthesis techniques, there were no experimental reports on the magnetic properties of as-prepared MXene. Until recently, Babar et al. have synthesized Nb2C MXene and among the MXene family. In this report, the magnetic nature of Nb2AlC-MAX and Nb2C MXene is shown. Fig. 5a and b show the zero-field-cooled (ZFC) and field-cooled (FC) magnetization curves measured for Nb2AlC MAX and Nb2C MXene, respectively, under an applied magnetic field of 5 mT using a SQUID magnetometer (Quantum Design, MPMS). From these curves, one can see that the magnetic behaviors of MAX and MXene are different.
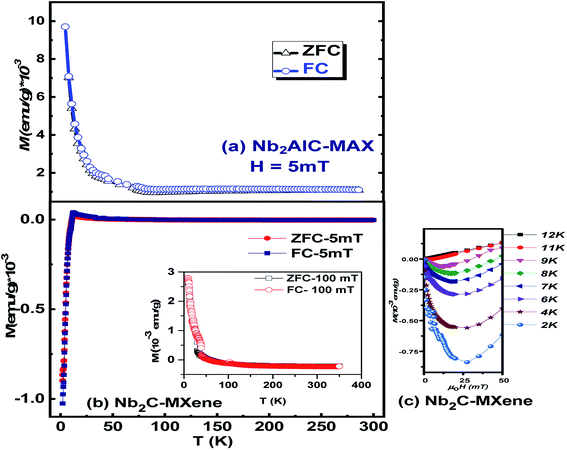 |
| Fig. 5 (a) ZFC–FC measurements of MAX at a field of 5 mT. (b) ZFC–FC measurements of MXene at 5 mT and 10 mT with inset of ZFC–FC curves at a field strength of 100 mT. (c) Variation of magnetic behavior with increase in temperature. | |
The FC–ZFC curves of MAX correspond to a paramagnetic material in which the magnetization increases positively with the decrease in temperature, whereas the FC–ZFC curves of MXene are opposite; the magnetization reverses its direction at a critical temperature and becomes negative at low temperatures. This trend of MXene fits well for a typical superconductivity-like diamagnetic response of a material. Recently, Babar et al. reported the Meissner effect in Nb2C MXene, which showed typical type-II superconductivity in the material with an onset transition temperature of 12.5 K. However, the authors did not discuss the origin of this behavior, as it might be inherent to the Nb2AlC MAX itself, and the contribution of the spin of the constituent elements needed to be discussed. Here, the magnetization vs. temperature (M–T) trend of MAX does not show any diamagnetic transition at any temperature, but a paramagnetic behavior.61–63 Hence, the MAX structure itself is not responsible for the previously observed superconductivity-like diamagnetism in MXene.
Contrary to the MAX phase, the FC–ZFC curves shown in Fig. 5b clearly indicate a magnetic phase transition showing a clear diamagnetic behavior that gives traces of the superconductivity effect in MXene. The inset of Fig. 5b indicates M–T curves of Nb2C MXene at a much higher applied field of 100 mT, at which the magnetization becomes positive at low temperature and is no longer a diamagnetic material. The sharp upward trend in magnetization, as well as the splitting between ZFC–FC curves at low temperature, indicates a significant paramagnetic contribution arising from the Nb ions.64
This also gives an indication of the existence of a threshold field that supports the presence of superconductivity in Nb2C MXene, as the threshold field corresponds to a type-II superconductor.8 Fig. 5c shows the quadrant of magnetization vs. magnetic field (M–H) curves measured at 2 K, 4 K, 6 K, 7 K, 8 K, 9 K, 11 K and 12 K. The curves clearly show a transition of magnetization from low-negative to high-negative, and then to low-negative values, representing the presence of Meissner effect at these temperatures as well as the presence of the respective threshold fields. At 12 K, the M–H response is linear to the field and refers purely to a paramagnetic curve, indicating the transition temperature.
Several theoretical studies have been carried out to explore the magnetic properties of different MXenes. The Cr-based carbide and nitride MXenes are predicted to be magnetic according to theoretical studies carried out by M. Khazei et al., and their electronic properties were reported to be altered upon adding the surface terminations.65 Different functionalized MXenes are predicted to be semiconductors with the bandgap range of 0.25–2 eV. Bare MXenes (MXenes without surface terminations) are supposed to have metallic nature, but the OH, O or F terminated MXenes show semiconductive nature, with bandgaps ranging from 0.05–1.8 eV.66,67 The asymmetric functionalized MXene, such as the Janus Mn2N, is a ferromagnet, while Mn2C is reported to be anti-ferromagnetic.68 Zhang et al. have reported the paramagnetic nature of as-prepared Ti3C2 and its magnetic variation on different synthesis conditions.69 Akgenc et al. theoretically pointed out the ferromagnetic half-metal and antiferromagnetic semiconductor nature of Ti2C MXene.70 Since the magnetic behavior of 2D nanostructures could be intrinsic or defect-induced, the wet chemical etching route could produce 2D sheets with intrinsic magnetic properties with high-yield and low-cost mass production.71 Considering the above findings, the magnetic behavior of our sample is found to be independent of the surface functionalization, providing the clue towards the intrinsic behavior of our Nb2C MXene. Its superconductivity-like behavior (the Meissner effect and the perfect diamagnetism at low temperature, negative magnetic moment) is calculated by density functional theory calculations.
Our group has recently reported a detailed experimental and theoretical analysis on stable ferromagnetism in Ti3C2 MXene. This report carries out experimental magnetic analysis with theoretical validations of doped and undoped Ti3C2 MXene.72 To further explore the reason for the existence of the observed superconducting-diamagnetism in Nb2C MXene (niobium carbide MXene), the structure was simulated in WIEN2k package, which employs the full-potential linear augmented plane wave method.73,74 The structure was optimized for the c-lattice parameter obtained from XRD analysis, where the space group is P63/mmc and the positions for niobium (Nb) and carbon (C) are (1/3, 2/3, u) and (0, 0, 0), respectively. For the minimization of the structures, 500 k-points were used in the irreducible Brillouin zone, with a k-mesh of 14 × 14 × 2, while the self-consistent field was also performed at the same number of k-points. The function that is used for solving Kohn–Sham equations is spin-polarized Perdew–Burke–Ernzerhof generalized gradient approximation (PBE-GGA).75
The crystal structure of Nb2C is shown in Fig. 6a. The magnetic moment of the compound is calculated to be −0.00485 μB, which although small is yet important, as the value is negative, which is an indication of the presence of diamagnetism in Nb2C. Moreover, the magnetic moment of Nb and C, as well as the interstitial position, are −0.00046 μB, −0.00017 μB, and −0.00485 μB, respectively. From the density of states (DOS) calculations, DOS versus energy graph is obtained, where Fig. 6b–d shows the total DOS for the compound, total DOS for Nb and partial DOS (PDOS) for the d-orbital of Nb, and total DOS of C with PDOS of the p-orbital of C. In Fig. 6b, at Fermi energy, there is a very small difference between the DOS of spin up and spin down.
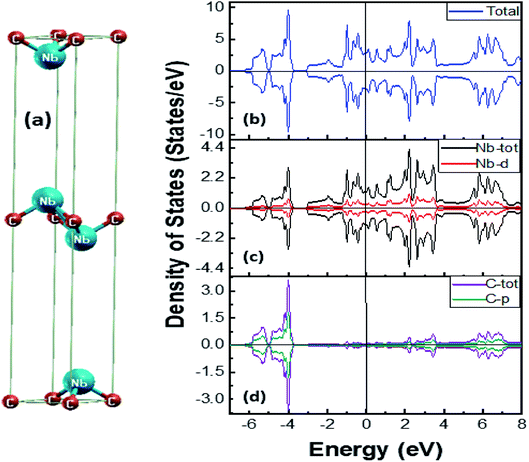 |
| Fig. 6 (a) Crystal structure of Nb2C in unit cell with space group P63/mmc. (b) Total density of states for Nb2C, (c) total DOS of Nb with partial DOS of Nb-3d shell, and (d) total DOS of C and partial DOS of C-2p shell. | |
Fig. 6c and d clarifies the reason for the negative moment, which is the d-orbital of Nb2C, whereas for C, the majority of states present is the valence band, and for Nb, the DOS is spread through the energy range, i.e., valence band and conduction band. Lei et al. have studied the different phases of Mo2C with functional groups and suggested superconducting behavior. The critical temperature varied according to the functional groups and was found to be in the range of 0 K to 13 K.76 Baber et al. showed that pristine MXene Nb2C exhibits type-II superconductor-like behavior. Magnetization versus applied field curves showed that the behavior is diamagnetic below 12.5 K.8 The negative total magnetic moment, i.e., −0.00485 μB in the present calculation, is an indication towards that diamagnetic behavior of Nb2C. The abovementioned computational studies verify the experimental superconductor-like diamagnetic nature of Nb2C MXene.
Experimental section
The materials used for the etching process include hydrofluoric acid (50 wt% in H2O, ≥99.99%), niobium aluminum carbide (Nb2AlC) powder (95% pure), de-ionized water, and absolute ethanol.
Synthesis of Nb2C MXene
To obtain Nb2C MXene, the wet chemical etching route was followed. The Nb2AlC MAX powder (200 mesh size) was immersed in 48–50% concentrated HF (1 g
:
10 ml, Sigma Aldrich) at room temperature. Etching at continuous magnetic stirring for 90 hours4 was not successful. We started etching at an optimized time of 40 hours while varying the temperature from 45 °C to 85 °C in equal intervals of 10 °C. After the etching process was completed, centrifugation was done, and each sample was washed repeatedly with deionized water. After removing the supernatant, the resulting powder was collected using ethanol and was left at room temperature to dry. The synthesis schematics and detailed etching mechanism are shown in Fig. 1. The rudimental yield comes out to be approximately 100% (yield here is defined as the ratio of the mass obtained for MXene after etching with the mass of MAX taken at the start). The best sample was the one etched at 55 °C, for an optimized etching time of 40 hours. This etching scheme came out to be effective to obtain the required accordion-like layered MXene structure.
HF handling protocol
It is always risky to handle hydrofluoric acid owing to its harmful and noxious attributes irrespective of its concentration. HF effectively penetrates into the skin, causing damage to deep layer tissues and bones without any visible effects within 1–24 hours.77 This leads to the death of tissues and limb loss. So, it is directed to use safety goggles, acid-resistant apron, face-shield and polyvinyl chloride (PVC) or polythene gloves to avoid any direct contact of HF with your skin. Since the inhalation of HF can cause swelling of the respiratory tract, in order to avoid it, the reactions should be carried out in a protective fume hood along with the usage of masks at all times.78,79
Conclusion
In conclusion, we have successfully synthesized 2D-Nb2C MXene sheets via wet-chemical etching using hydrofluoric (HF) acid. Structural analysis indicated the effective removal of the Al layer, and SEM images show the formation of the resultant layered structure. FTIR spectra indicate the presence of surface terminations that are unavoidable during the etching process. The optical analysis shows a reduction in bandgap that was characterized due to the presence of surface functionalization. Raman spectra show that the MXene is more ordered than the MAX itself. The magnetic analysis shows the variation of the magnetic behavior of MXene upon application of different field strengths as well as entirely different behaviors of MAX and MXene. Our theoretical analysis validates the diamagnetic nature of MXene. Although the value of the negative magnetic moment is small, it very importantly signifies the experimental diamagnetic nature of MXene, giving clear signatures of the possible existence of superconductivity in Nb2C MXene, which can be verified further by transport measurement to make it a 100% certainty in the future. It is notable that to our knowledge, this is the first study on Nb2C-MXene that conducts experimental studies in combination with the theoretical validation of experimental findings. For our MXene, these physical properties are significant, which could make it a leading member of the 2D MXene family in terms of its novel magnetic nature for possible application in two-dimensional spintronics.
Conflicts of interest
The authors declare no competing financial interest.
Acknowledgements
The authors are thankful to the Higher Education Commission (HEC) of Pakistan for providing research funding under the Project No 6040/Federal/NRPU/R&D/HEC/2016 and HEC/USAID for financial support under the Project No HEC/R&D/PAKUS/2017/783. The author also thanks the School of Natural Sciences (SNS) at the National University of Sciences and Technology (NUST), Islamabad, Pakistan, for partial financial support.
References
- M. Naguib, et al., Two-dimensional nanocrystals produced by exfoliation of Ti3AlC2, Adv. Mater., 2011, 23(37), 4248–4253 CrossRef CAS.
- M. Naguib, O. Mashtalir, J. Carle, V. Presser, J. Lu, L. Hultman, Y. Gogotsi and M. W. Barsoum, ACS Nano, 2012, 6, 1322 CrossRef CAS PubMed.
- M. Naguib, et al., 25th anniversary article: MXenes: a new family of two-dimensional materials, Adv. Mater., 2014, 26(7), 992–1005 CrossRef CAS.
- M. Naguib, et al., New two-dimensional niobium and vanadium carbides as promising materials for Li-ion batteries, J. Am. Chem. Soc., 2013, 135(43), 15966–15969 CrossRef CAS PubMed.
- B. Scheibe, et al., Study on the magnetic properties of differently functionalized multilayered Ti3C2Tx MXenes and Ti-Al-C carbides, Appl. Surf. Sci., 2019, 479, 216–224 CrossRef CAS.
- S. Z. Butler, et al., Progress, challenges, and opportunities in two-dimensional materials beyond graphene, ACS Nano, 2013, 7(4), 2898–2926 CrossRef CAS PubMed.
- J. Pan, S. Lany and Y. Qi, Computationally driven two-dimensional materials design: what is next?, ACS Nano, 2017, 11(8), 7560–7564 CrossRef CAS PubMed.
- Z. U. D. Babar, et al., Peculiar Magnetic Behavior and Meissner Effect in Two-dimensional Layered Nb2C MXene, 2D Materials, 2020, 7, 035012 CrossRef.
- O. Mashtalir, et al., Amine-assisted delamination of Nb2C MXene for Li-ion energy storage devices, Adv. Mater., 2015, 27(23), 3501–3506 CrossRef CAS.
- A. Byeon, et al., Lithium-ion capacitors with 2D Nb2CTx (MXene)–carbon nanotube electrodes, J. Power Sources, 2016, 326, 686–694 CrossRef CAS.
- C. Zhang, et al., Synthesis and charge storage properties of hierarchical niobium pentoxide/carbon/niobium carbide (MXene) hybrid materials, Chem. Mater., 2016, 28(11), 3937–3943 CrossRef CAS.
- H. Lin, et al., A two-dimensional biodegradable niobium carbide (MXene) for photothermal tumor eradication in NIR-I and NIR-II biowindows, J. Am. Chem. Soc., 2017, 139(45), 16235–16247 CrossRef CAS PubMed.
- J. Halim, et al., Synthesis of two-dimensional Nb1.33C (MXene) with randomly distributed vacancies by etching of the quaternary solid solution (Nb2/3Sc1/3)2AlC MAX phase, ACS Appl. Nano Mater., 2018, 1(6), 2455–2460 CrossRef CAS.
- X. Han, et al., Therapeutic mesopores construction on 2D Nb2C MXenes for targeted and enhanced chemo-photothermal cancer therapy in NIR-II biowindow, Theranostics, 2018, 8(16), 4491 CrossRef CAS PubMed.
- C. Peng, et al., A hydrothermal etching route to synthesis of 2D MXene (Ti3C2, Nb2C): Enhanced exfoliation and improved adsorption performance, Ceram. Int., 2018, 44(15), 18886–18893 CrossRef CAS.
- S. Tu, et al., MXene-Derived Ferroelectric Crystals, Adv. Mater., 2019, 31(14), 1806860 CrossRef PubMed.
- J. Halim, et al., Electronic and optical characterization of 2D Ti2C and Nb2C (MXene) thin films, J. Phys.: Condens. Matter, 2019, 31(16), 165301 CrossRef CAS.
- M. Fatima, et al., Nb-Doped MXene With Enhanced Energy Storage Capacity and Stability, Front. Chem., 2020, 8, 168 CrossRef PubMed.
- M. A. Iqbal, et al., Ti3C2-MXene/Bismuth Ferrite Nanohybrids for Efficient Degradation of Organic Dyes and Colorless Pollutants, ACS Omega, 2019, 4(24), 20530–20539 CrossRef CAS PubMed.
- J. He, P. Lyu and P. Nachtigall, New two-dimensional Mn-based MXenes with room-temperature ferromagnetism and half-metallicity, J. Mater. Chem. CJ. Mater. Chem. C, 2016, 4(47), 11143–11149 RSC.
- M. Iqbal, et al., Co-existence of Novel Ferromagnetic/Anti-ferromagnetic Phases in Two-dimensional Ti3C2 MXene, 2019, arXiv preprint arXiv:1907.12588 Search PubMed.
- S. Rafiq, et al., Novel room-temperature ferromagnetism in Gd-doped 2-dimensional Ti3C2Tx MXene semiconductor for spintronics, J. Magn. Magn. Mater., 2020, 497, 165954 CrossRef.
- M. Naguib and Y. Gogotsi, Synthesis of two-dimensional materials by selective extraction, Acc. Chem. Res., 2015, 48(1), 128–135 CrossRef CAS PubMed.
- J. Halim, Synthesis and Characterization of 2D Nanocrystals and Thin Films of Transition Metal Carbides (MXenes), Linköping University Electronic Press, Diss, 2014 Search PubMed.
- G. W. Bentzel, N. J. Lane, S. C. Vogel, K. An, M. W. Barsoum and E. A. N. Caspi, A High-Temperature Neutron Diffraction Study of Nb2AlC and TiNbAlC, J. Am. Ceram. Soc., 2015, 98(3), 940–947 CrossRef CAS.
- T. Prikhna, O. Ostash, V. Sverdun, M. Karpets, T. Zimych, A. Ivasyshin, T. Cabioc’h, et al., Presence of Oxygen in Ti-Al-C MAX Phases-Based Materials and their Stability in Oxidizing Environment at Elevated Temperatures, in Proceedings of the International Conference on Oxide Materials for Electronic Engineering, 2017, vol. 59 Search PubMed.
- J. Rosen, P. Å. Persson, M. Ionescu, A. Kondyurin, D. R. McKenzie and M. M. M. Bilek, Oxygen incorporation in Ti2Al C thin films, Appl. Phys. Lett., 2008, 92(6), 064102 CrossRef.
- T. Liao, J. Wang, M. Li and Y. Zhou, First-principles study of oxygen incorporation and migration mechanisms in Ti2AlC, J. Mater. Res., 2009, 24(10), 3190–3196 CrossRef CAS.
- M. Dahlqvist and J. Rosén, Oxygen incorporation and defect formation in Ti2AlC, V2AlC and Cr2AlC from first-principles calculations, 2014 Search PubMed.
- B. Scheibe, V. Kupka, B. Peplińska, M. Jarek and K. Tadyszak, The influence of oxygen concentration during max phases (Ti3AlC2) preparation on the α-Al2O3 microparticles content and specific surface area of multilayered mxenes (Ti3C2Tx), Materials, 2019, 12(3), 353 CrossRef CAS PubMed.
- M. Naguib, et al., Two-dimensional nanocrystals produced by exfoliation of Ti3AlC2, Adv. Mater., 2011, 23(37), 4248–4253 CrossRef CAS PubMed.
- Yi Tang, et al., Synthesis of nitrogen-doped two-dimensional Ti3C2 with enhanced electrochemical performance, J. Electrochem. Soc., 2017, 164(4), A923–A929 CrossRef CAS.
- C. Yang, et al., Improved capacitance of nitrogen-doped delaminated two-dimensional titanium carbide by urea-assisted synthesis, Electrochim. Acta, 2017, 225, 416–424 CrossRef CAS.
- Y. Wen, et al., Nitrogen-doped Ti3C2Tx MXene electrodes for high-performance supercapacitors, Nano Energy, 2017, 38, 368–376 CrossRef CAS.
- L. M. Viculis, et al., Intercalation and exfoliation routes to graphite nanoplatelets, J. Mater. Chem., 2005, 15(9), 974–978 RSC.
- C. Peng, P. Wei, X. Chen, Y. Zhang, F. Zhu, Y. Cao, H. Wang, H. Yu and F. Peng, A hydrothermal etching route to synthesis of 2D MXene (Ti3C2, Nb2C): enhanced exfoliation and improved adsorption performance, Ceram. Int., 2018, 44(15), 18886–18893 CrossRef CAS.
- G. Zhao, H. Lv, Y. Zhou, X. Zheng, C. Wu and C. Xu, Self-assembled sandwich-like MXene-derived nanocomposites for enhanced electromagnetic wave absorption, ACS Appl. Mater. Interfaces, 2018, 10(49), 42925–42932 CrossRef CAS PubMed.
- A. Champagne, L. Shi, T. Ouisse, B. Hackens and J. Charlier, Electronic and vibrational properties of V2C-based MXenes: From experiments to first-principles modeling, Phys. Rev. B, 2018, 97, 115439 CrossRef CAS.
- T. Hu, J. Wang, H. Zhang, Z. Li, M. Hu and X. Wang, Vibrational properties of Ti3C2 and Ti3C2T2 (T = O, F, OH) monosheets by first-principles calculations: a comparative study, Phys. Chem. Chem. Phys., 2015, 17, 9997–10003 RSC.
- R. B. Rakhi, B. Ahmed, M. N. Hedhili, D. H. Anjum, and H. N. Alshareef, Effect of post-etch annealing gas composition on the structural and electrochemical properties of Ti2CTx MXene electrodes for supercapacitor applications, 2015 Search PubMed.
- Y. Yoon, T. A. Le, A. P. Tiwari, I. Kim, M. W. Barsoum, and H. Lee, Low temperature solution synthesis of reduced, 2018, pp. 22429–22438 Search PubMed.
- P. Tumor, H. Lin, S. Gao, C. Dai, Y. Chen, J. Shi, H. Lin, S. Gao, C. Dai, Y. Chen, and J. Shi, Two-Dimensional Biodegradable Niobium Carbide (MXene) for Two-Dimensional Biodegradable Niobium Carbide (MXene) for Photothermal Tumor Eradication in NIR-I and NIR-II Bio-Windows, 2017 Search PubMed.
- V. Presser, M. Naguib, L. Chaput, A. Togo, G. Hug and M. W. Barsoum, First-order Raman scattering of the MAX phases: Ti2AlN, Ti2AlC0.5N0.5, Ti2AlC, (Ti0.5V0.5)2AlC, V2AlC, Ti3AlC2, and Ti3GeC2, J. Raman Spectrosc., 2012, 43(1), 168–172 CrossRef CAS.
- T. Su, R. Peng, Z. D. Hood, M. Naguib, I. N. Ivanov and J. Kahk, et al., One-step Synthesis of Nb2O5/C/Nb2C (MXene) Composites and Their Use as Photocatalysts for Hydrogen Evolution, ChemSusChem, 2011, 11, 688 CrossRef PubMed.
- K. Wang, Laser Based Fabrication of Graphene, 2013 Search PubMed.
- J. Yang, X. Zhao, X. Shan, H. Fan, L. Yang, Y. Zhang and X. Li, Blue-shift of UV emission in ZnO/graphene composites, J. Alloys Compd., 2013, 556, 1–5 CrossRef CAS.
- W. Acchar and d. S. José Roberto Bezerra, Surface characterization of alumina reinforced with niobium carbide obtained by polymer precursor, Mater. Res., 2006, 9(3), 271–274 CrossRef CAS.
- G. Socrates, Infrared and Raman characteristic group frequencies: tables and charts, John Wiley & Sons, 2004 Search PubMed.
- Y. Li, et al., Facile preparation of in situ coated Ti3C2Tx/Ni0.5Zn0.5Fe2O4 composites and their electromagnetic performance, RSC Adv., 2017, 7(40), 24698–24708 RSC.
- J. Zhu, C. Alexander and U. Schwingenschlögl, Nb-based MXenes for Li-ion battery applications, Phys. Status Solidi RRL, 2015, 9(12), 726–729 CrossRef CAS.
- Z. Ma, et al., Tunable band structures of heterostructured bilayers with transition-metal dichalcogenide and MXene monolayer, J. Phys. Chem. C, 2014, 118(10), 5593–5599 CrossRef CAS.
- G. Zou, et al., Synthesis of MXene/Ag composites for extraordinary long cycle lifetime lithium storage at high rates, ACS Appl. Mater. Interfaces, 2016, 8(34), 22280–22286 CrossRef CAS PubMed.
- Z. Zhang, et al., Self-reduction synthesis of new MXene/Ag composites with unexpected electrocatalytic activity, ACS Sustainable Chem. Eng., 2016, 4(12), 6763–6771 CrossRef CAS.
- S. Myhra, J. A. A. Crossley and M. W. Barsoum, Crystal-chemistry of the Ti3AlC2 and Ti4AlN3 layered carbide/nitride phases—characterization by XPS, J. Phys. Chem. Solids, 2001, 62(4), 811–817 CrossRef CAS.
- P. Kubelka, Ein Beitrag zur Optik der Farbanstriche (Contribution to the optic of paint), Zeitschrift für technische Physik, 1931, 12, 593–601 Search PubMed.
- X. Xu, et al., Synthesis and photocatalytic behaviors of high surface area BiFeO3 thin films, J. Am. Ceram. Soc., 2011, 94(8), 2296–2299 CrossRef CAS.
- M. Naguib, O. Mashtalir, J. Carle, V. Presser, J. Lu, L. Hultman, Y. Gogotsi and M. W. Barsoum, ACS Nano, 2012, 6, 1322 CrossRef CAS PubMed.
- I. R. Shein and A. L. Ivanovskii, Comput. Mater. Sci., 2012, 65, 104 CrossRef CAS.
- M. Khazaei, M. Arai, T. Sasaki, C.-Y. Chung, N. S. Venkataramanan, M. Estili, Y. Sakka and Y. Kawazoe, Adv. Funct. Mater., 2012, 23, 2185 CrossRef.
- S. Wang, Yu-L. Du and W.-He Liao, Tunable band gap and optical properties of surface-functionalized Sc2C monolayer, Chin. Phys. B, 2017, 26(1), 017806 CrossRef.
- P. Balan Aravind, et al., Magnetic properties and photocatalytic applications of 2D sheets of nonlayered manganese telluride by liquid exfoliation, ACS Appl. Nano Mater., 2018, 1(11), 6427–6434 CrossRef.
- H. T. Masood, et al., Low temperature ferromagnetic properties of CdS and CdTe thin films, Chin. Phys. B, 2017, 26(6), 067503 CrossRef.
- A. T. Raghavender, et al., Nano-ilmenite FeTiO3: Synthesis and characterization, J. Magn. Magn. Mater, 2013, 331, 129–132 CrossRef CAS.
- Patel and S. K. Singh, Single-crystalline Gd-doped BiFeO3 nanowires: R3c-to-Pn21a phase transition and enhancement in high-coercivity ferromagnetism, J. Mater. Chem. C, 2018, 6(3), 526–534 RSC.
- M. Khazaei, M. Arai, T. Sasaki, C. Y. Chung, N. S. Venkataramanan, M. Estili, Y. Sakka and Y. Kawazoe, Novel electronic and magnetic properties of two-dimensional transition metal carbides and nitrides, Adv. Funct. Mater., 2013, 23(17), 2185–2192 CrossRef CAS.
- M. Naguib, M. Kurtoglu, V. Presser, J. Lu, J. Niu, M. Heon, L. Hultman, Y. Gogotsi and M. W. Barsoum, Two-imensional nanocrystals produced by exfoliation of Ti3AlC2, Adv. Mater., 2011, 23(37), 4248–4253 CrossRef CAS PubMed.
- I. R. Shein and A. L. Ivanovskii, Graphene-like titanium carbides and nitrides Tin+ 1Cn, Tin+1Nn (n= 1, 2, and 3) from de-intercalated MAX phases: First-principles probing of their structural, electronic properties and relative stability, Comput. Mater. Sci., 2012, 65, 104–114 CrossRef CAS.
- N. C. Frey, A. Bandyopadhyay, H. Kumar, B. Anasori, Y. Gogotsi and V. B. Shenoy, Surface-engineered MXenes: electric field control of magnetism and enhanced magnetic anisotropy, ACS Nano, 2019, 13(3), 2831–2839 CrossRef CAS PubMed.
- K. Zhang, M. Di, L. Fu, Y. Deng, Y. Du and N. Tang, Enhancing the magnetism of 2D carbide MXene Ti3C2Tx by H2 annealing, Carbon, 2020, 157, 90–96 CrossRef CAS.
- B. Akgenc, A. Mogulkoc and E. Durgun, Phase-dependent electronic and magnetic properties of Ti2C monolayers, J. Appl. Phys., 2020, 127(8), 084302 CrossRef.
- J. Xu, W. Li and Y. Hou, Two-dimensional magnetic nanostructures, Trends Chem., 2020, 2(2), 163–173 CrossRef.
- J. Fatheema, M. Fatima, N. B. Monir, A. S. Khan and S. Rizwan, A comprehensive computational and experimental analysis of stable ferromagnetism in layered 2D Nb-doped Ti3C2 MXene, Physica E Low Dimens. Syst. Nanostruct., 2020, 114253 CrossRef CAS.
- K. Schwarz, P. Blaha and G. K. H. Madsen, Electronic structure calculations of solids using the WIEN2k package for material sciences, Comput. Phys. Commun., 2002, 147, 71–76 CrossRef.
- P. Blaha, K. Schwarz, G. K. H. Madsen, D. Kvasnicka and J. Luitz, Computer code WIEN2K, Vienna University of Technology, 2001 Search PubMed.
- J. P. Perdew, K. Burke and M. Ernzerhof, Generalized gradient approximation made simple, Phys. Rev. Lett., 1996, 77, 3865 CrossRef CAS PubMed.
- J. Lei, A. Kutana and B. I. Yakobson, Predicting stable phase monolayer Mo2C (MXene), a superconductor with chemically-tunable critical temperature, J. Mater. Chem. C, 2017, 5, 3438–3444 RSC.
- M. Alhabeb, K. Maleski, B. Anasori, P. Lelyukh, L. Clark, S. Sin and Y. Gogotsi, Guidelines for synthesis and processing of two-dimensional titanium carbide (Ti3C2T x MXene), Chem. Mater., 2017, 29(18), 7633–7644 CrossRef CAS.
- T. Liu, Hydrofluoric Acid, Skin, 2015, 62 Search PubMed , http://li.chemistry.ucsc.edu/wp-content/uploads/2015/12/Hydrofluoric-Acid-UCSC-Li-201512-Updated.pdf..
- G. Lunn and G. Lawler, Safe use of hazardous chemicals, Curr. Protoc. Microbiol., 2006,(1), 1A-3 Search PubMed.
|
This journal is © The Royal Society of Chemistry 2020 |
Click here to see how this site uses Cookies. View our privacy policy here.