DOI:
10.1039/D0RA02807J
(Paper)
RSC Adv., 2020,
10, 25662-25668
Significant effects of two pesticides on the bacteriostatic activity and antioxidant ability of green tea polyphenols†
Received
27th March 2020
, Accepted 22nd June 2020
First published on 7th July 2020
Abstract
Green tea polyphenols (GTPs) are widely used in food preservation because of their strong bacteriostatic activity and antioxidant ability, and whether pesticides as common pollutants in food will affect the function of GTPs is worthy of attention. Therefore, GTPs and two pesticides, namely, acetamiprid (ACE) and diquat dibromide (DIQ) commonly used on food crops were selected as research objects and Vibrio qinghaiensis sp.-Q67 (Q67) as the test organism to explore the effects of pesticide pollutants on the bacteriostatic activity of GTPs by the time-dependent microplate toxicity analysis method (t-MTA). The binary mixture systems of GTPs and two pesticides were designed by the direct equipartition ray design method (EquRay). The effect residual ratio (ERR) method was used to quantify the toxicity interactions of binary mixture systems. Besides, the effects of these two pesticides on the antioxidant capacity of GTPs were investigated. The results indicated that the bacteriostatic activity of GTPs upon interaction with the two pesticides shows certain time characteristics. These two pesticides can affect the bacteriostatic activity of GTPs, which is enhanced or weakened with prolonged duration, i.e. time-dependent synergism or antagonism. The bacteriostatic mechanism of the three substances and their mixtures is produced by affecting the cell morphology or destroying the cell structure, and the long-term antagonism of the three substances may be due to the competition for the active site. The two pesticides can greatly reduce the antioxidant capacity of GTPs. ACE reduces the free radical scavenging ability of GTPs by 14–24% and DIQ by 39–63% in the experimental concentration ratios. In addition, the free radical scavenging ability of GTPs decreases with the increase in the concentration ratio of the two pesticides in the mixture systems.
Introduction
As we all know, green tea polyphenols (GTPs) accounting for 20–35% of the dry weight of tea are the general name of polyphenols with catechin as the main component in tea.1 In recent years, GTPs have been widely produced and used in food preservation2,3 industry because of their good bacteriostatic activity4,5 and antioxidant ability.6,7 According to relevant statistics, total annual production of GTPs can reach 5731 tons by 2020 in China.8 The bacteriostatic ability of GTPs can effectively inhibit the spoilage of food, and its antioxidant ability can maintain the good color of fruits and vegetables. Zhu et al.9 found that when the concentration of tea polyphenol was 250 mg kg−1, it had a good fresh-keeping effect on tomatoes, and the tomatoes still had a good sensory quality after 15 days. More importantly, the appropriate dose of GTPs is harmless to the human body and has a health effect for humans.10,11 Therefore, GTPs as food preservatives have their unique advantages.
However, many pesticides are used on food crops to promote production. Thus, pesticides have become common residual pollutants in food, especially in fruits and vegetables. Chen et al.12 carried out pesticide residue examination on fruits and vegetables in Xiamen market, and found that the highest detected cypermethrin level was 13.92 mg kg−1 in a sample of pakchoi cabbage. Hjorth et al.13 found that 80.4% of samples contained pesticide residues in a total of 724 samples of 46 different fruits and vegetables from eight South American countries. Swarnam and Velmurugan14 found that pesticide residues in some fruits exceeded 2 mg kg−1 in Indian market. Therefore, GTPs may coexist with pesticides in food, especially in fruits and vegetables, and the effects of pesticide pollutants on the bacteriostatic and antioxidant capacity of GTPs should be considered in order to ensure the preservation function of GTPs.
This study aims to explore the effects of pesticide pollutants on the bacteriostatic and antioxidant capacity of GTPs. To do so, two commonly used pesticides, namely, acetamiprid (ACE) and diquat dibromide (DIQ) in tea were selected as research objects. A freshwater luminous bacterium Vibrio qinghaiensis sp.-Q67 (Q67) which is very sensitive to toxic substances was selected as the test organism. The bacteriostatic ability data of GTPs, two pesticides and their binary mixtures to Q67 were determined by the time-dependent microplate toxicity analysis method (t-MTA).15–19 The binary mixture system of GTPs and two pesticides was constructed by the direct equipartition ray design (EquRay) method.20 In addition, the effect residual ratio (ERR)19 method based on the concentration addition model (CA)21–26 was used to evaluate the interaction between pesticides and GTPs. The bacteriostatic mechanism of the three substances and their mixtures to Q67 was preliminarily determined by observing the cellular morphology.5,27 Besides, the free radical scavenging ability of binary mixture rays was determined by the method of salicylic acid.28
Materials and methods
Chemicals
GTPs (green tea polyphenols) were purchased from Shanghai Yuanye Biotechnology Co., Ltd (Shanghai, China). Two pesticides, namely, acetamiprid (ACE) and diquat dibromide (DIQ) were purchased from the National Pesticide Quality Supervision and Testing centre of Shenyang Chemical Research Institute (Shenyang, China). All the reagents were of analytical grade and used as received without further purification. The physical and chemical properties of the three reagents, the concentration of the stock solution, and the dilution factor (f) are listed in Table 1. The storage solution was prepared with Milli-Q water and stored at 4 °C.
Table 1 Some physiochemical properties, stocks, dilution factors (f), the fitted results by Weibull function and some statistics of GTPs and two pesticides
Name |
Abbr. |
Molecular weight |
CAS-RN |
Stocks (mol L−1) |
f |
Time (h) |
αa |
βa |
RMSE |
R |
pEC50 |
α and β refer to the position and shape parameters in the Weibull function, respectively. pEC50 refers to the negative logarithm of the median effective concentrations. |
Green tea polyphenols |
GTPs |
281.36 |
84650-60-2 |
0.005 |
0.6 |
0.25 |
8.53 |
2.81 |
0.022 |
0.9979 |
3.17 |
2 |
7.82 |
2.51 |
0.048 |
0.9908 |
3.26 |
4 |
7.87 |
2.28 |
0.058 |
0.9896 |
3.61 |
8 |
6.90 |
1.87 |
0.056 |
0.9874 |
3.89 |
12 |
7.21 |
1.83 |
0.056 |
0.9868 |
4.14 |
Acetamiprid |
ACE |
222.67 |
135410-20-7 |
0.006 |
0.6 |
0.25 |
4.76 |
1.49 |
0.037 |
0.9930 |
3.44 |
22 |
4.38 |
1.37 |
0.031 |
0.9944 |
3.47 |
4 |
4.78 |
1.43 |
0.027 |
0.9963 |
3.60 |
8 |
4.56 |
1.30 |
0.026 |
0.9961 |
3.46 |
12 |
4.67 |
1.42 |
0.021 |
0.9975 |
3.55 |
Diquat dibromide |
DIQ |
344.05 |
85-00-7 |
0.0002 |
0.4 |
0.25 |
7.33 |
2.00 |
0.020 |
0.9884 |
0 |
2 |
15.00 |
4.03 |
0.028 |
0.9710 |
0 |
4 |
3.15 |
0.68 |
0.092 |
0.9426 |
5.17 |
8 |
4.99 |
0.88 |
0.100 |
0.9598 |
6.09 |
12 |
9.68 |
1.48 |
0.071 |
0.9863 |
6.79 |
Bacterial culture
The freeze-dried luminescent bacterium Vibrio qinghaiensis sp.-Q67 (Q67) was purchased from Beijing Hamamatsu Corp., Ltd. (Beijing, China). The preparation of the culture medium and culture process of Q67 are detailed in the literature.16
Determination of bacteriostatic activity
The bacteriostatic data of GTPs, two pesticides and their binary mixtures to Q67 were obtained by t-MTA. Using a 96 microporous plate as an experimental carrier, 200 μL purified water was added to the surrounding 36 holes to prevent the edge effect. In the 2nd, 6th, 7th and 11th rows of 24 holes, 100 μL purified water was added as the blank control, and the drug was diluted to 12 different concentration gradients into the remaining 36 wells by a pre-determined dilution factor. The relative luminous unit (RLU) of each hole was measured at 0.25 h, 2 h, 4 h, 8 h and 12 h, respectively, and the luminous inhibition toxicities of single components and its mixtures to Q67 were calculated using eqn (1) to reflect the bacteriostatic activity of the pollutants to Q67 at each time point. The design of the microplate is detailed in the literature.15,16 |
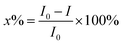 | (1) |
where I0 is the average RLU of the blank control group and I the average RLU at each concentration gradient.
Experimental design of mixtures
There are numerous concentration ratios and concentrations of binary mixtures. The direct equipartition ray design (EquRay) method can well select the representative mixtures to reflect the interaction characteristics in the whole concentration range.20 Therefore, binary mixture systems of GTPs and two pesticides were constructed by the EquRay method. Each mixture system contains five rays with different concentration ratios (pis), and each ray was diluted to 12 different concentration gradients according to the dilution factor obtained by the pre-experiment. The pis of each component of binary mixtures is shown in Table 2.
Table 2 Components and their concentration ratios in binary mixture rays
Ray |
PGTPs |
PACE |
Ray |
PGTPs |
PDIQ |
R1 |
5.605 × 10−1 |
4.395 × 10−1 |
R1 |
9.996 × 10−1 |
4.496 × 10−4 |
R2 |
3.378 × 10−1 |
6.622 × 10−1 |
R2 |
9.989 × 10−1 |
1.123 × 10−3 |
R3 |
2.032 × 10−1 |
7.968 × 10−1 |
R3 |
9.978 × 10−1 |
2.244 × 10−3 |
R4 |
1.131 × 10−1 |
8.869 × 10−1 |
R4 |
9.955 × 10−1 |
4.478 × 10−3 |
R5 |
4.854 × 10−2 |
9.515 × 10−1 |
R5 |
9.889 × 10−1 |
1.112 × 10−2 |
Concentration-effect curve fitting
The bacteriostatic data obtained by t-MTA were fitted by logit (eqn (2)) or the Weibull (eqn (3)) functions. The calculation of effective concentrations and 95% observation-based confidence intervals (OCIs) was completed using the APTox software.26 The two function formulas are as follows: |
E = 1/(1 + exp(−α − β × log10(c)))
| (2) |
|
E = 1 − exp(−exp(α + β × log10(c)))
| (3) |
where E represents the effect (0 ≤ E ≤ 1), c the concentration of a single compound or mixture, and α and β the model parameters.
Effects of the two pesticides on the bacteriostatic activity of GTPs
The interaction between GTPs and the two pesticides was evaluated by the ERR method19 based on the CA model. The calculation of ERR was completed using the APTox software.26 Mathematically, the ERR model can be formulated as: |
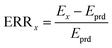 | (4) |
where Ex is the fitting effect or the effect corresponding to the upper limit or lower limit of the OCIs and Eprd the effect value predicted by CA at the same concentration. The ERR with confidence intervals is noted as ERRimp. When the ERRimp is significantly larger/less than 0, say the interaction is synergism/antagonism. When 0 is located between the confidence intervals, it is additive action or has no interaction.
Bacteriostatic mechanism to Q67
In order to investigate the bacteriostatic mechanism of GTPs, two pesticides and their binary mixtures on Q67, the cell morphology of Q67 exposed to GTPs, two pesticides and their binary mixtures at a concentration of EC80 for 12 h was measured. First, the Q67 bacterial suspension of logarithmic growth period was placed in a conical flask. Then, GTPs, two pesticides and their binary mixtures were added to the conical flask, respectively, so that the concentration of drugs in the suspension was equal to the EC80 of GTPs, two pesticides and their binary mixtures at 12 h. Lastly, the conical flask was cultured in a constant temperature incubator at 22 ± 1 °C for 12 h. After 12 h, the cell morphology of Q67 was observed using a scanning electron microscope. The specific steps for the preparation of electron microscope samples can be found in the relevant literature.5,27
The effects of two pesticides on the antioxidant ability of GTPs
The free radical scavenging ability of GTPs and two pesticide binary mixtures under different pis (experimental group) were determined by the method of salicylic acid.28 Then, replace the pesticides in binary mixtures with an equal volume of Milli-Q water as control (blank group) to determine the free radical scavenging ability of GTPs. The greater the difference between the free radical scavenging ability of the experimental group and the control group, the greater the effects of pesticides on the antioxidant capacity of GTPs.
Results and discussion
Bacteriostatic activity of individual components to Q67
The nonlinear least square method was used to fit the concentration–response data of GTPs and two pesticides to Q67 at 0.25 h, 2 h, 4 h, 8 h and 12 h. The results indicated that the Weibull function can effectively characterize the concentration–response relationship of GTPs, ACE and DIQ (R > 0.9, RMSE < 0.1). The specific fitting results and relevant statistic parameters are shown in Table 1. The time–concentration–response curves (t-CRCs) of single components are shown in Fig. 1.
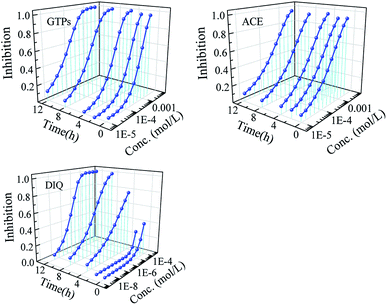 |
| Fig. 1 t-CRCs of GTPs and two pesticides. | |
From Fig. 1, it was found that the time characteristics of the bacteriostatic activity of GTPs and two pesticides to Q67 are different. The bacteriostatic activity of GTPs increases slowly with the prolongation of exposure time within 0.25–12 h, while the bacteriostatic activity of DIQ decreases within 0.25–2 h, but increases within 2–12 h, and the bacteriostatic activity of ACE to Q67 is not affected by exposure time. Combined with Table 1, taking the negative logarithm of EC50 (pEC50) as the bacteriostatic activity index, the bacteriostatic activity order at 0.25 h and 2 h is as follows: ACE (pEC50 = 3.44–3.47) > GTPs (pEC50 = 3.17–3.26) > DIQ (pEC50 = 0). The bacteriostatic activity order at 4 h, 8 h and 12 h is as follows: DIQ (pEC50 = 3.81–6.79) > GTPs (pEC50 = 3.61–4.14) > ACE (pEC50 = 3.55–3.60).
In conclusion, the antibacterial effect of GTPs and two pesticides on Q67 varies with the exposure time.29,30 In addition, it was noted that besides the good bacteriostatic activity of GTPs, the two pesticides, as toxic substances, have inhibitory toxicity to bacteria as they are pesticides with high toxicity to nontarget organisms.31,32
Effects of the two pesticides on the bacteriostatic activity of GTPs
The Weibull function can also effectively characterize the concentration–response relationship of binary mixture rays of GTPs and two pesticides (R > 0.9, RMSE < 0.1). The fitting results and relevant statistic parameters are shown in Tables S1 and S2.† The observed concentration-effect data with 95% OCIs fitted CRCs and the predicted curves by CA of representative rays are shown in Fig. S1 and S2.†
The ERR method based on the CA model can respond to the relative deviation of predicted and observed results and has the ability to quantitatively evaluate the toxicity interactions.19 Therefore, in this study, the ERR method was used to quantify the toxicity interactions between the components. The ERRimp of each ray is shown in Fig. 2 and 3.
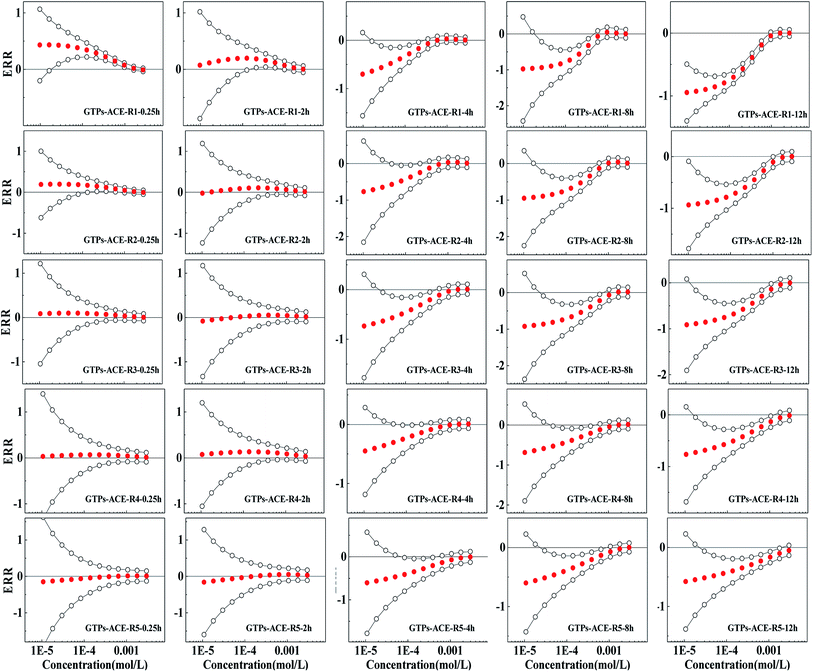 |
| Fig. 2 ERR ( ) with confidence intervals ( ) of five rays for GTPs-ACE mixture systems. | |
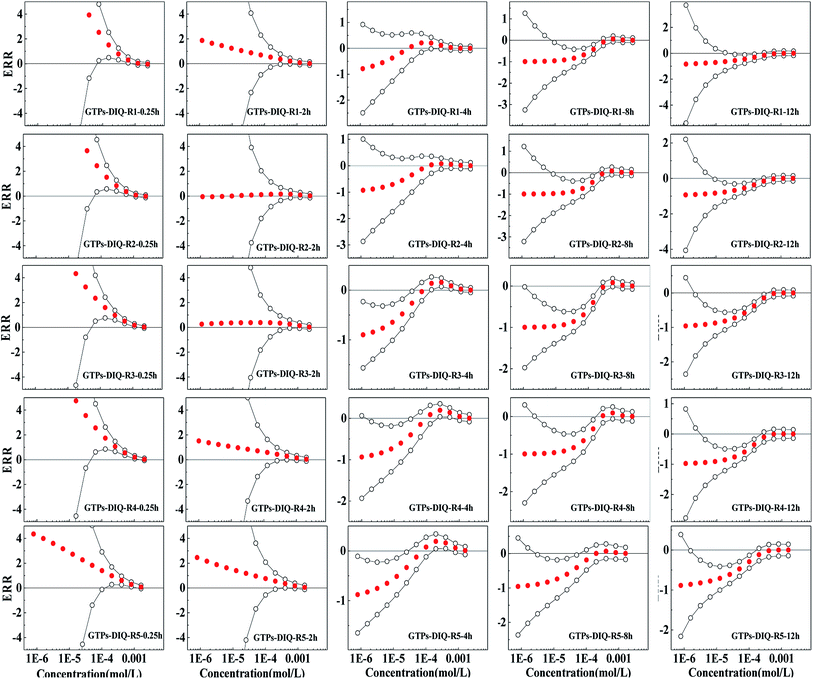 |
| Fig. 3 ERR ( ) with confidence intervals ( ) of five rays for GTPs-DIQ mixture systems. | |
It can be observed from Fig. 2 that the five rays of the GTPs-ACE binary mixture systems show additive action or synergism in exposure times of 0.25 h and 2 h, i.e. 0 is located between the confidence intervals or ERRimp is larger than 0. However, with the prolongation of exposure time, the five mixture rays all show antagonism, and ERRimp is significantly less than 0 after 4 h. The antagonism of five rays is mainly concentrated in the middle- and low-concentration region, and the concentration region of antagonism expands with the prolonged exposure time. From Fig. 3, it can be observed that the five mixture rays of GTPs-DIQ binary mixture systems show additive action or synergism in exposure times of 0.25 h and 2 h, but with the prolongation of exposure time, the toxicity interaction changes from additive action and synergism to antagonism, which is similar to GTPs-ACE binary mixture systems. However, the antagonism of five rays of GTPs-DIQ binary mixture systems gradually shifts from the low-concentration region to the middle-concentration region with time lengthening.
Therefore, from the results of toxicity interaction analysis, it can be observed that ACE and DIQ can affect the bacteriostatic activity of GTPs, and the influence mode differs in different exposure times. The short-term synergism of the mixture system will strengthen the bacteriostatic ability of GTPs, while the long-term antagonism of the mixture system will lead to a decrease in the bacteriostatic ability of GTPs.
The bacteriostatic mechanism to Q67
The cell morphology of Q67 exposed to EC80 of GTPs, two pesticides and their representative rays for 12 h is shown in Fig. 4.
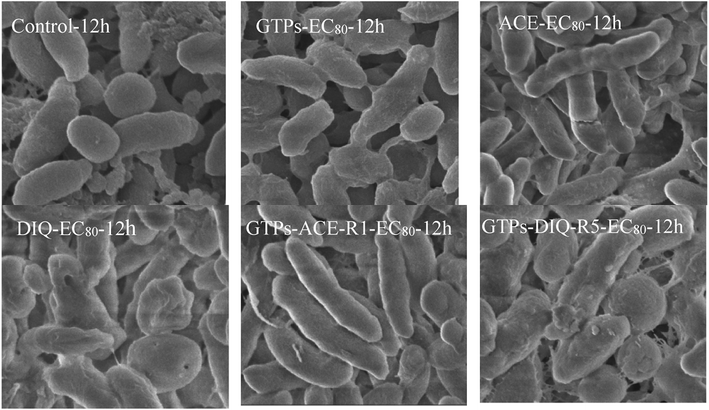 |
| Fig. 4 Cell morphology of Q67 exposed to EC80 of GTPs, two pesticides and their representative rays for 12 h. | |
From Fig. 4, the morphology of Q67 cells is changed compared with the control group. GTPs and DIQ make Q67 cells bond with each other and destroy the membrane structure of bacteria. While ACE prolonged Q67 cells and ruptured Q67 cells. The rays with the greatest long-term bacteriostatic activity in two binary mixture systems were selected to observe their effects on the morphology of Q67 cells. It was found that mixtures also damage the morphology of Q67 cells. Besides, it was found that the amount of bacteria in the experimental group is lower than that in the control group after centrifugation. This suggests that GTPs, two pesticides and their mixtures can inhibit the reproduction of bacteria. Ben Lagha et al.5 also found that tea polyphenols could destroy the cell structure of the bacteria. Therefore, the three substances may have similar antibacterial mechanisms, and the long-term antagonism of the three substances is due to the competition for the active site.33
Effects of the two pesticides on the antioxidant ability of GTPs
In order to characterize the effects of two pesticides on the antioxidant ability of GTPs, the free radical scavenging ability of each binary mixture ray was determined by the salicylic acid method. The results are shown in Fig. 5. As can be observed from Fig. 5, the free radical scavenging ability of each binary mixture ray is lower than that of the control group. ACE reduces the free radical scavenging ability of GTPs by 14–24% and DIQ by 39–63% in the experimental concentration ratios. This indicates that pesticide pollutants can damage the antioxidant capacity of GTPs, and the extent of damage is greater for DIQ than for ACE.
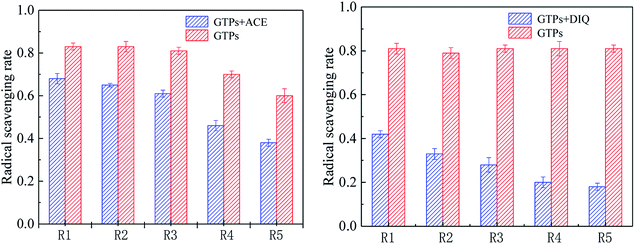 |
| Fig. 5 Effects of ACE and DIQ on GTPs' radical scavenging ability in different concentration ratios. | |
Besides, the reductions in the free radical scavenging ability of GTPs were linearly fitted with PACE and PDIQ. The results show that there is a good linear relationship between the reductions in the free radical scavenging ability of GTPs and the concentration ratios of ACE and DIQ (Fig. 6). In addition, the free radical scavenging ability of GTPs decreases with the increase in the concentration ratio of the two pesticides in the mixture systems (R > 0.8, P < 0.05).
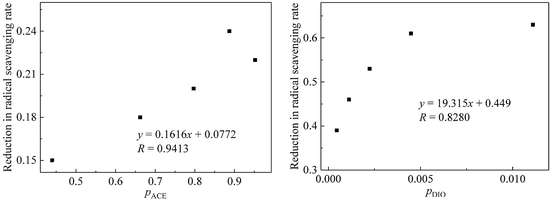 |
| Fig. 6 Relationship between the reductions in the free radical scavenging ability of GTPs and the concentration ratio of two pesticides. | |
The antioxidant capacity of GTPs plays an important role in their preservation effect.34 The loss of antioxidant capacity of GTPs means that it can no longer protect food from oxidative damage. Therefore, pesticide residues in food should be avoided as far as possible in order to ensure the preservation function of GTPs, and the influence of pesticide residues in the formulation of GTP usage should also be considered.
Conclusion
GTPs and the two pesticides used all have antibacterial activity, and their bacteriostatic activity shows certain time characteristics. Both ACE and DIQ can affect the bacteriostatic activity of GTPs. The bacteriostatic activity of GTPs will be enhanced when the two pesticides and GTPs coexist for a short time. However, the bacteriostatic activity of GTPs will be weakened when the two pesticides and GTPs coexist for a longer time. That is to say, the mixtures of the two pesticides and GTPs exhibit time-dependent synergism or antagonism. The bacteriostatic mechanism of GTPs, two pesticides and their mixtures on Q67 involves affecting the cell morphology or destroying the cell structure. Therefore, GTPs and two pesticides may have similar antibacterial mechanisms, and the long-term antagonism of the three substances is due to the competition for the active site. The two pesticides can greatly reduce the antioxidant capacity of GTPs, and the extent of damage is greater for DIQ than for ACE. In addition, the free radical scavenging ability of GTPs decreases with the increase in the concentration ratio of the two pesticides in the mixture systems.
Conflicts of interest
There are no conflicts to declare.
Acknowledgements
The authors are grateful to the National Natural Science Foundation of China (No. 21677001), Natural Science Foundation of Anhui Province, China (No. 1708085MB50), the Science and Technology Major Projects of Anhui Province (18030801106), and the Key Research and Development Plan of Anhui Province (201904a07020070) for their financial support.
References
- L. Zhang, C. T. Ho, J. Zhou, J. S. Santos, L. Armstrong and D. Granato, Compr. Rev. Food Sci. Food Saf., 2019, 18, 1474–1495 CrossRef CAS.
- W. D. Wang and Y. E. Sun, Cell. Mol. Biol., 2016, 62, 44 CrossRef PubMed.
- I. Gutiérrez-del-Río, J. Fernández and F. Lombó, Int. J. Antimicrob. Agents, 2018, 52, 309–315 CrossRef PubMed.
- S. Bansal, N. Syan, P. Mathur and S. Choudhary, Med. Chem. Res., 2012, 21, 3347–3360 CrossRef CAS.
- A. Ben Lagha, B. Haas and D. Grenier, Sci. Rep., 2017, 7, 44815 CrossRef CAS PubMed.
- B. Frei and J. V. Higdon, J. Nutr., 2003, 133, 3275S–3284S CrossRef CAS PubMed.
- P. A. Coentráo, V. L. Teixeira and A. D. Netto, Nat. Prod. Commun., 2011, 6, 651–656 Search PubMed.
- X. F. Zuo, J. H. Kong, X. F. Yang, Y. Gao, J. Y. Zhou, X. Q. Su, R. Tan and C. H. Diao, China Tea Processing, 2019, 4, 14–20 Search PubMed.
- J. L. Zhu, J. Feng, L. Y. Niu and Z. Lu., Food Ind., 2020, 41, 164–169 Search PubMed.
- Y. Miyata, Y. Shida, T. Hakariya and H. Sakai, Molecules, 2019, 24, 193 CrossRef PubMed.
- M. Lodovici, C. Casalini, C. D. Filippo, E. Copeland and P. Dolara, Food Chem. Toxicol., 2000, 38, 1085–1088 CrossRef CAS PubMed.
- C. Chen, Y. Qian, Q. Chen, C. Tao, C. Li and Y. Li, Food Control, 2011, 22, 1114–1120 CrossRef CAS.
- K. Hjorth, K. Johansen, B. Holen, A. Andersson, H. B. Christensen, K. Siivinen and M. Toome, Food Control, 2011, 22, 1701–1706 CrossRef CAS.
- T. P. Swarnam and A. Velmurugan, Environ. Monit. Assess., 2013, 185, 6119–6127 CrossRef CAS PubMed.
- J. Zhang, T. T. Ding, X. Q. Dong and Z. Q. Bian, RSC Adv., 2018, 8, 26089 RSC.
- J. Zhang, S. S. Liu, X. Q. Dong and M. Chen, RSC Adv., 2015, 5, 107076–107082 RSC.
- L. Liu, S. S. Liu, M. Yu, J. Zhang and F. Chen, Anal. Methods, 2015, 7, 9912–9917 RSC.
- L. Feng, S. S. Liu, K. Li, H. X. Tang and H. L. Liu, J. Hazard. Mater., 2017, 327, 11–17 CrossRef CAS PubMed.
- R. Qu, S. S. Liu, F. Chen and K. Li, RSC Adv., 2016, 6, 21012–21018 RSC.
- R. N. Dou, S. S. Liu, L. Y. Mo, H. L. Liu and F. C. Deng, Environ. Sci. Pollut. Res., 2010, 18, 734–742 CrossRef PubMed.
- G. J. Howard and T. F. Webster, J. Theor. Biol., 2009, 259, 469–477 CrossRef CAS PubMed.
- T. Backhaus, M. Faust, M. Scholze, P. Gramatica, M. Vighi and L. H. Grimme, Environ. Toxicol. Chem., 2004, 23, 258–264 CrossRef CAS PubMed.
- Y. Iwasaki and P. Gauthier, Environ. Toxicol. Chem., 2016, 35, 526–527 CrossRef CAS PubMed.
- N. Cedergreen, H. Sorensen and C. Svendsen, Sci. Total Environ., 2012, 427, 229–237 CrossRef PubMed.
- H. L. Ge, M. Zhou, D. Z. Lv, M. Y. Wang, C. Z. Dong, Y. Wan, Z. S. Zhang and S. R. Wang, Int. J. Mol. Sci., 2019, 20, 6163 CrossRef PubMed.
- S. S. Liu, J. Zhang, Y. H. Zhang and L. T. Tan, Acta Chim. Sin., 2012, 70, 1511–1517 CrossRef CAS.
- L. K. Ng, R. Sherburne, D. E. Taylor and M. E. Stiles, J. Bacteriol., 1985, 164, 338–343 CrossRef CAS PubMed.
- N. W. Li, C. H. Liu and K. Huang, Food Sci., 2011, 32, 58–60 CAS.
- J. Baas, T. Jager and B. Kooijman, Sci. Total Environ., 2010, 408, 3735–3739 CrossRef CAS PubMed.
- Y. Fan, S. S. Liu, R. Qu, K. Li and H. L. Liu, RSC Adv., 2017, 7, 6080–6088 RSC.
- R. C. Ray, Environ. Pollut., 1983, 32, 147–155 CrossRef CAS.
- L. G. Bermudez-Humaran and P. Langellam, Curr. Inorg. Chem., 2012, 8, 248–253 CAS.
- J. P. Groten, V. J. Feron and S. Jürgen, Trends Pharmacol. Sci., 2001, 22, 316–322 CrossRef CAS PubMed.
- S. M. Ojagh, M. A. Sahari, M. Rezaei and S. V. Hosseini, Int. J. Agric. Biol., 2011, 1, 174–181 Search PubMed.
Footnote |
† Electronic supplementary information (ESI) available. See DOI: 10.1039/d0ra02807j |
|
This journal is © The Royal Society of Chemistry 2020 |