DOI:
10.1039/D0RA04461J
(Paper)
RSC Adv., 2020,
10, 25828-25835
A convenient method for synthesis of terpyridines via a cooperative vinylogous anomeric based oxidation†
Received
19th May 2020
, Accepted 29th June 2020
First published on 8th July 2020
Abstract
The presented study is the first report of the synthesis of terpyridines in the presence of a nanomagnetic catalyst instead of harmful reagents. Herein, Fe3O4@O2PO2(CH2)2NH3+CF3CO2− as a retrievable nanocatalyst with magnetic properties was applied for the multi-component reaction between acetylpyridine derivatives (2 or 3 or 4-isomer), aryl aldehydes and ammonium acetate under conventional heating conditions in the absence of any solvent. The derived terpyridines were obtained with acceptable yields and brief reaction times via a cooperative vinylogous anomeric based oxidation route. Fe3O4@O2PO2(CH2)2NH3+CF3CO2− showed a high capability for recovery and reuse in the mentioned reaction.
Introduction
Nowadays, immobilizing catalytically homogeneous species onto the surface of core–shell materials with magnetic cores for the preparation of nanomagnetic heterogeneous catalysts has attracted enormous interest. The insoluble and paramagnetic natures of these kinds of catalysts,1–6 provide efficient and simple isolation of them from the reaction mixture with an externally applied magnetic field. Because of their retrievability, high surface area, biocompatibility and possibility of modification of the surface of the magnetic nanoparticles with both organic and inorganic compounds, these catalysts have found comprehensive and significant uses among academic and industrial scientists.7–11 They are robust catalysts which have been applied in various kinds of organic synthesis.12–23
In conformity with the principles of green chemistry, such as increase the efficiency of chemical reactions, the atomic economy, reduce derivatives, decrease the utilization of organic solvents and waste production, simplify processes and design for energy efficiency, multi-component reactions (MCRs) have been presented and extended as a powerful method for the preparation of structurally diverse drug-like compounds (expansion of large chemical libraries of drug-like compounds).24–32
Anomeric effect is an important stereoelectronic phenomenon which had been described as the axial superiority for an electronegative substituent (acceptor group) positioned adjacent to a donor group (lone pairs) rather than equatorial position.33–39 In the other hand, this concept is a kind of negative hyperconjugation can lead sharing of the electron density from lone-pair orbital to a vacant anti-bonding sigma orbital (n → σ*) (Scheme 1).40,41
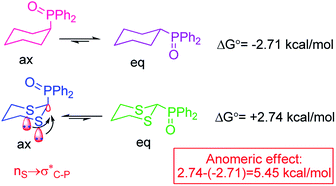 |
| Scheme 1 Anomeric effect leads to more stability axial conformer 2-diphenylphosphinoyl 1,3-dithiane.42,43 | |
Anomeric effect has a potent role to explain some eccentric phenomena in the chemistry knowledge. For instance, anomeric effect justifies why bis-peroxides A and B can be more thermodynamically stable than mono-peroxides, and can even melt without decomposition at 131 and 142 °C, respectively. Anomalous stability of these compounds is associated with strong anomeric
interactions in two peroxide groups which separated by a one-atom bridge (Scheme 2).44
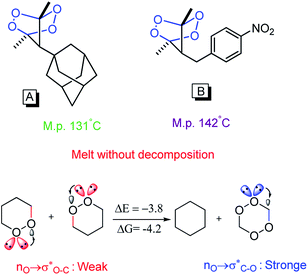 |
| Scheme 2 Stability of bis-peroxides due to the anomeric effect. | |
The anomeric effect can also be extended through double bonds in what has been named the vinylogous anomeric effect (VAE). The VAE had been applied for the stereoelectronic supported interactions of unshared electron pairs to vacant anti-bonding sigma orbitals (n → σ*) over four bonds (Scheme 3).45–53
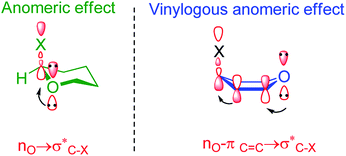 |
| Scheme 3 Anomeic effect in tetrahydropyrane versus a vinylogous anomeric effect in pyran.54 | |
Katritzky et al. by low temperature X-ray crystallography have been shown that facile C–N bond dissociation of the benzotriazole residue in compound C, have been related to a vinylogous anomeric effect (VAE) (Scheme 4).54
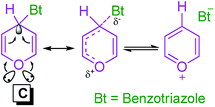 |
| Scheme 4 VAE causes facile C–N bond dissociation of the benzotriazole residue. | |
Based on above mentioned facts, “cooperative anomeric and/or vinylogous anomeric based oxidation” terms represent a new mechanistic vision and major driving force for the oxidative aromatization of some susceptible heterocyclic molecules. Recently, these concepts have been reviewed.55,56
Pyridines are a privileged category of N-heteroaromatics found in numerous natural and synthetic compounds with a wide range of biological, pharmaceutical, agrochemical and physiological applications such as anti-ulcer,57 antineoplastic,58 antimicrobial,59–62 antiviral,63 antitubercular,64 antitumor,65–69 and cardiotonic70 properties. Among these compounds, terpyridines (tpys) by reason of their π-stacking ability, directional H-bonding and coordination properties are outstanding building blocks in supramolecular chemistry.71 In addition, terpyridines (tpys) have been applied broadly in various fields such as molecular biology,72,73 antitumor chemo-therapeutics,74 photosensitization,75 and asymmetric catalysis.76 Also, they are able to coordinate to a variety of transition-metal ions as ligands and form the corresponding complexes.77–79 A class of these ligands is becoming popular as a building block for the assembly of coordination polymers and networks.80–95 Therefore, the construction of novel terpyridines and/or methodologies has attracted many attentions. So far many protocols were reported for the synthesis of these valuable compounds.96–104 Traditional protocols for synthesis of tpys have many drawbacks such as multi-step procedure, the use of microwave irradiation, a long reaction time, low yield, utilization of organic solvents like glycol and harmful reagents for environment such as sodium or potassium hydroxide and acetic acid. Therefore, the presentation of more efficient and greener methods for the synthesis of tpys is highly desirable.
For overcome to these demerits, for the first time we applied a recoverable nanocatalyst with magnetic properties instead of harmful reagent for one-pot synthesis of tpys under conventional heating conditions in the absence of any solvent. Also, we generalize the presented protocol by applying various isomer of acetylpyridine (2 or 3 or 4-isomer) and introduce a new mechanistic route (CVABO) for the synthesis of tpys. In this paper, to develop the concept of “cooperative vinylogous anomeric based oxidation (CVABO)”105–110 and in continuation of our efforts to develop new protocols for the synthesis of pyridine derivatives,106–110 we investigated the catalytic performance of Fe3O4@O2PO2(CH2)2NH3+CF3CO2− in construction of terpyridins (Scheme 5).
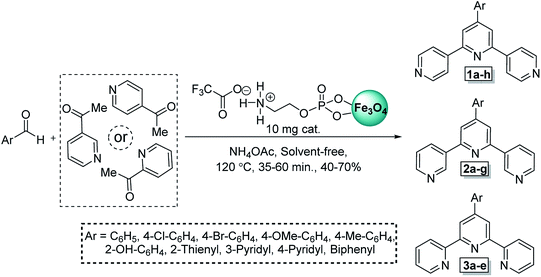 |
| Scheme 5 Construction of terpyridines in the presence a catalytic amount of Fe3O4@O2PO2(CH2)2NH3+CF3CO2−. | |
Results and discussion
The reusable nano magnetic catalyst with ionic liquid tags namely Fe3O4@O2PO2(CH2)2NH3+CF3CO2− was synthesized according to our recently reported procedure.106
In first step, for determine the optimal reaction conditions, we chose condensation between of benzaldehyde, 4-acetylpyridine and ammonium acetate as a model reaction. For obtaining the appropriate conditions, effective parameters including various solvents, temperature and amount of catalyst were exactly probed. Based on the obtained data as summarized in Table 1, the highest experimental yield and lowest reaction time was achieved in the presence of 10 mg catalyst at 120 °C in the absence of any solvent (Table 1, entry 3). In addition to, for demonstration of the last step of the proposed mechanism, the optimized reaction conditions were performed under nitrogen and argon atmospheres. The results under air, nitrogen and argon atmospheres are same (Table 1, entry 3).
Table 1 Influence of the different parameters on construction of terpyridine 1aa
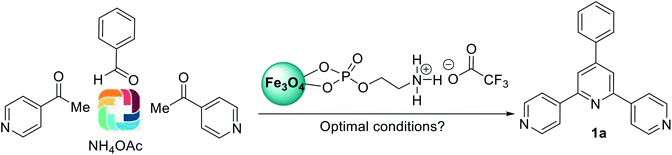
|
Entry |
Solvent |
Temperature (°C) |
Load of catalyst (mg) |
Time (min) |
Yieldb (%) |
Reaction conditions: benzaldehyde (1.0 mmol, 0.106 g), 4-acetyl-pyridine (2.0 mmol, 0.242 g) and ammonium acetate (2.0 mmol, 0.154 g). Reported yields are referred to isolated yields. The achieved data for testing the model reaction under air, nitrogen and argon atmospheres are similar. |
1 |
— |
80 |
10 |
90 |
60 |
2 |
— |
100 |
10 |
60 |
75 |
3c |
— |
120 |
10 |
50 |
80 |
4 |
— |
140 |
10 |
50 |
78 |
5 |
— |
120 |
15 |
50 |
80 |
6 |
— |
120 |
5 |
60 |
65 |
7 |
— |
120 |
— |
90 |
30 |
8 |
H2O |
Reflux |
10 |
90 |
40 |
9 |
EtOH |
Reflux |
10 |
90 |
40 |
10 |
CH3CN |
Reflux |
10 |
90 |
43 |
11 |
EtOAc |
Reflux |
10 |
90 |
35 |
12 |
CH2Cl2 |
Reflux |
10 |
90 |
Trace |
13 |
n-Hexane |
Reflux |
10 |
90 |
Trace |
Afterwards, the scope, limitations and generality of the presented protocol developed by applying different aryl aldehydes, acetylpyridine (2, 3 and 4-isomers) and ammonium acetate using of Fe3O4@O2PO2(CH2)2NH3+CF3CO2− as a recoverable catalyst under the optimal qualifications. As disclose in Table 2, all desired molecules were synthesized in brief times with acceptable yields.
Table 2 Fe3O4@O2PO2(CH2)2NH3+CF3CO2− catalyzed synthesis of terpyridinesa
Reaction condition: aryl aldehyde (1.0 mmol), acetylpyridine derivatives (2.0 mmol, 0.242 g) and ammonium acetate (2.0 mmol, 0.154 g), solvent free, 120 °C, catalyst = 10 mg. Reported yields are referred to isolated yields. |
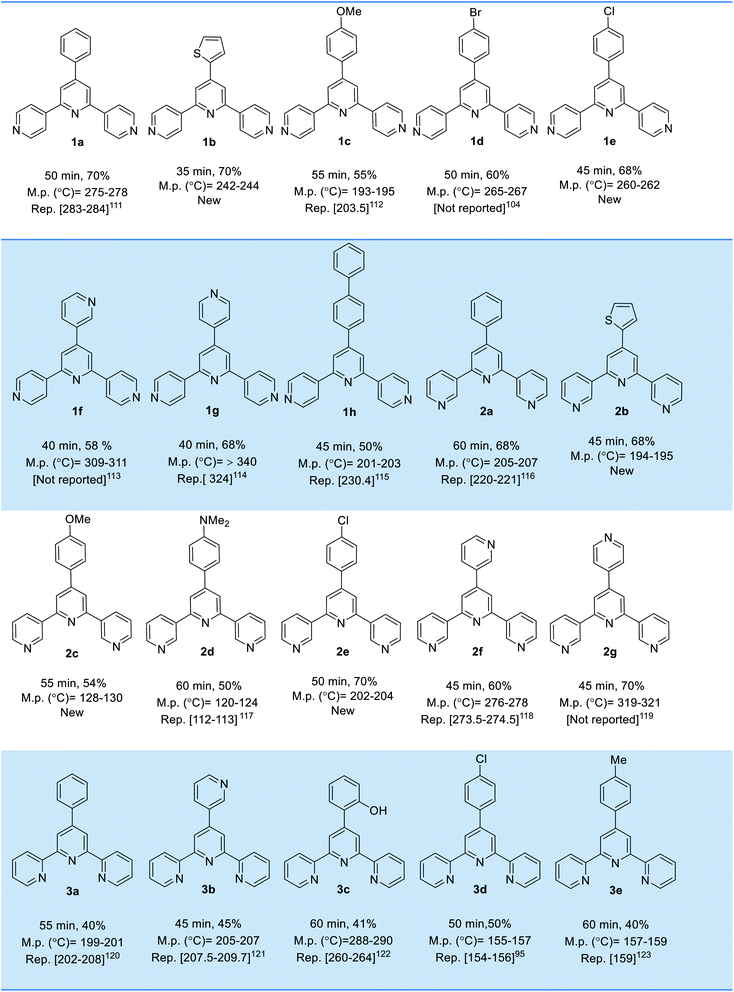 |
In a separate study, the possibility of recycling Fe3O4@O2PO2(CH2)2NH3+CF3CO2− was probed by the model reaction between benzaldehyde, 4-acetylpyridine and ammonium acetate in 50 minute. After each individual run, hot ethanol was added to the reaction mixture to dissolve unreacted starting materials and target product. Thus the insoluble nanocatalyst conveniently isolated from the reaction mixture utilizing a magnet bar. Then, the reusable catalyst was utilizable after washing with ethanol and drying for subsequent run. As depicted in Fig. 1, it can be inferred that the catalyst has good recovery capability for reusing test.
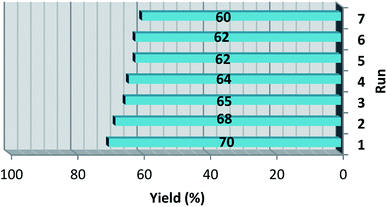 |
| Fig. 1 The reusing test of Fe3O4@O2PO2(CH2)2NH3+CF3CO2− | |
A suggested plausible reaction mechanism for the preparation of desirable molecule 2a via a cooperative vinylogous anomeric based oxidation mechanism is portrayed in Scheme 6. Initially, in the presence of Fe3O4@O2PO2(CH2)2NH3+CF3CO2−, enolic form of 3-acetylpyridine attacked to activated benzaldehyde to produce intermediate A. In continuation, chalcone intermediate A is attacked by second molecule of 3-acetylpyridine in enolic form to afford intermediate B. Afterwards, the enamine intermediate C is produced from the reaction of ammonium acetate and intermediate B. In the next step, through intramolecular nucleophilic attack, intermediate C converted to intermediate D. In the last step, lone pair electrons of N atom through C–C double bonds interact with a vacant anti-bonding orbital of C–H bond
and weaken it which favored hydride transfer and H2 releasing from intermediate D to generate the aromatized molecule 2a. The achieved data from optimization of reaction under argon and nitrogen atmospheres verified our suggestion for oxidation and aromatization of intermediate D.
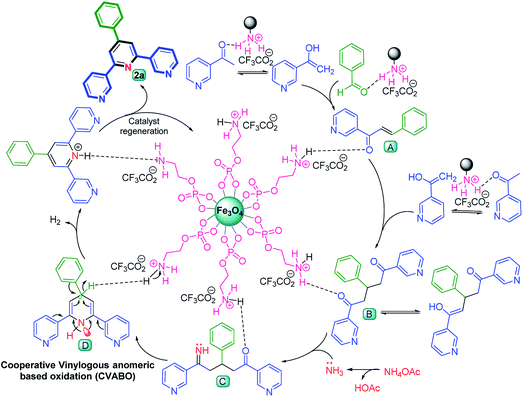 |
| Scheme 6 A proposed reaction mechanism for preparation of target molecule 2a via a cooperative vinylogous anomeric based oxidation mechanism. | |
Experimental
General
The commercially available chemicals were obtained from Fluka and Merck chemical companies and used as received without further purification. The reaction progress and purity of the prepared structures were monitored by TLC performed with silica gel SIL G/UV 254 plates. FT-IR spectra were recorded on a PerkinElmer Spectrum Version 10.02.00 using KBr pellets. The 1H NMR (301 MHz) and 13C NMR (76 MHz) spectra were recorded on a Bruker spectrometer (δ in ppm) using DMSO-d6 as solvent with chemical shifts measured relative to Si(CH3)4 as internal standard. Melting points were determined with a Buchi B-545 melting point apparatus in open capillary tubes.
General procedure for synthesis of Fe3O4@O2PO2(CH2)2NH3+CF3CO2−
The reusable nano magnetic catalyst with ionic liquid tags namely Fe3O4@O2PO2(CH2)2NH3+CF3CO2− was synthesized according to our recently reported protocol.106
General procedure for the synthesis of terpyridines
To a mixture of aromatic aldehydes (1.0 mmol), acetylpyridine derivatives (2.0 mmol, 0.242 g) and ammonium acetate (2.0 mmol, 0.154 g), 10 mg of Fe3O4@O2PO2(CH2)2NH3+CF3CO2− was added. The result mixture was stirred in the absence of any solvent at 120 °C for suitable times (Table 2). The progress of reaction was checked by TLC (n-hexane/ethylacetate as eluent). After completions of reactions, for separation of the catalyst, hot ethanol was added to the reaction mixtures. Then, insoluble nanocatalyst conveniently isolated from the reaction mixture utilizing a magnet bar. It was washed with ethanol and reused for subsequent reaction. Finally, the solvent was removed and the crude solids obtained were purified using ethanol. The desired products were obtained in acceptable isolated yields as presented in Table 2.
Selected spectral data
4′-(2-Thiophenyl)-4,2′:6′,4′′-terpyridine (1b). A light green solid, mp = 242–244 °C. FT-IR (KBr, ν, cm−1): 3035, 1592, 1557, 1538, 1403, 828. 1H NMR (301 MHz, DMSO) δppm: 8.80 (d, 4H, J = 6 Hz, pyridine), 8.40 (s, 2H, pyridine), 8.32 (d, 4H, J = 6 Hz, pyridine), 8.20 (d, 1H, J = 3 Hz, thiophene), 7.88 (d, 1H, J = 3 Hz, thiophene), 7.34 (t, 1H, J = 3 Hz, thiophene). 13C NMR (76 MHz, DMSO) δppm: 155.1, 150.9, 145.5, 144.3, 140.4, 129.7, 129.4, 128.4, 121.6, 117.5.
4′-(4-Chlorophenyl)-4,2′:6′,4′′-terpyridine (1e). A white solid, mp = 260–262 °C. FT-IR (KBr, ν, cm−1): 3035, 1592, 1559, 1493, 1389, 1092, 812. 1H NMR (301 MHz, DMSO) δppm: 8.80 (d, 4H, J = 6 Hz, pyridine), 8.53 (s, 2H, pyridine), 8.36 (d, 4H, J = 6 Hz, pyridine), 8.20 (d, 2H, J = 6 Hz, aromatic), 7.69 (d, 2H, J = 6 Hz, aromatic). 13C NMR (76 MHz, DMSO) δppm: 155.0, 150.9, 149.4, 145.7, 136.1, 135.2, 129.9, 129.6, 121.7, 119.4.
4′-Phenyl-3,2′:6′,3′′-terpyridine (2a). A white solid, mp = 205–207 °C. FT-IR (KBr, ν, cm−1): 3034, 1595, 1558, 1483, 1390, 803. 1H NMR (301 MHz, DMSO) δppm: 9.55–9.54 (m, 2H, pyridine), 8.75–8.71 (m, 4H, pyridine), 8.40 (s, 2H, pyridine), 8.15–8.12 (m, 2H, aromatic), 7.64–7.56 (m, 5H, aromatic). 13C NMR (76 MHz, DMSO) δppm: 155.2, 150.6, 150.4, 148.8, 137.6, 135.0, 134.5, 130.1, 129.6, 128.0, 124.3, 118.0.
4′-(2-Thiophenyl)-3,2′:6′,3′′-terpyridine (2b). A white solid, mp = 194–195 °C. FT-IR (KBr, ν, cm−1): 3055, 1603, 1547, 1428, 1024, 805, 695. 1H NMR (301 MHz, DMSO) δppm: 9.50 (s, 2H, pyridine), 8.73–8.67 (m, 4H, pyridine), 8.30 (s, 2H, pyridine), 8.17 (dd, 1H, J1 = 3.6 Hz, J2 = 0.9 Hz, thiophene), 7.85 (dd, 1H, J1 = 4.8 Hz, J2 = 0.6 Hz, thiophene), 7.60 (dd, 2H, J1 = 7.8 Hz, J2 = 4.8 Hz, pyridine), 7.33 (dd, 1H, J1 = 5.1 Hz, J2 = 3.9 Hz, thiophene). 13C NMR (76 MHz, DMSO) δppm: 155.3, 150.7, 148.7, 143.9, 140.7, 134.9, 134.19, 129.4, 129.3, 128.2, 124.3, 116.1.
4′-(4-Methoxyphenyl)-3,2′:6′,3′′-terpyridine (2c). A white solid, mp = 128–130 °C. FT-IR (KBr, ν, cm−1): 3031, 1597, 1541, 1489, 812. 1H NMR (301 MHz, DMSO) δppm: 9.53 (s, 2H, pyridine), 8.71 (s, 4H, pyridine), 8.35 (s, 2H, pyridine), 8.11 (d, 2H, J = 6 Hz, aromatic), 7.59 (s, 2H, aromatic), 7.15 (d, 2H, J = 3 Hz, aromatic), 3.88 (s, 3H, OMe). 13C NMR (76 MHz, DMSO) δppm: 161.1, 155.0, 150.5, 149.9, 148.7, 134.9, 134.6, 129.7, 129.3, 124.2, 117.3, 115.0, 55.9.
4′-(4-Chlorophenyl)-3,2′:6′,3′′-terpyridine (2e). A white solid, mp = 202–204 °C. FT-IR (KBr, ν, cm−1): 3044, 1609, 1577, 1545, 1497, 1420, 1024, 803, 701. 1H NMR (301 MHz, DMSO) δppm: 9.53 (s, 2H, pyridine), 8.73–8.70 (m, 4H, pyridine), 8.40 (s, 2H, pyridine), 8.17 (d, 2H, J = 9 Hz, aromatic), 7.65 (d, 2H, J = 9 Hz, aromatic), 7.60 (dd, 2H, J1 = 9 Hz, J2 = 6 Hz, aromatic). 13C NMR (76 MHz, DMSO) δppm: 155.2, 150.7, 149.0, 148.8, 136.4, 135.0, 134.94, 134.4, 129.8, 129.5, 124.3, 117.9.
4′-(4-Methylphenyl)-2,2′:6′,2′′-terpyridine (3e). A white solid, mp = 157–159 °C. FT-IR (KBr, ν, cm−1): 3050, 1602, 1584, 1551, 1467, 1386, 780. 1H NMR (301 MHz, DMSO) δppm: 8.85–8.83 (m, 2H, pyridine), 8.79 (s, 2H, pyridine), 8.76–8.74 (m, 2H, pyridine), 8.12 (td, 2H, J1 = 6 Hz, J2 = 1.2 Hz), 8.01–7.99 (m, 2H, aromatic), 7.70–7.66 (m, 2H, aromatic), 7.64–7.59 (m, 2H, aromatic), 1.68 (s, 3H, Me). 13C NMR (76 MHz, DMSO) δppm: 155.7, 154.9, 149.5, 149.3, 137.5, 137.5, 129.49, 129.4, 126.9, 124.5, 120.9, 118.0, 25.5.
Conclusions
In conclusions, for the first time a nanomagnetic catalyst was applied for one-pot preparation of terpyridines. In this study, the catalytic performance of Fe3O4@O2PO2(CH2)2NH3+CF3CO2− as a retrievable nanocatalyst with magnetic properties was investigated for the synthesis of terpyridines through a multi-component reaction between various isomer of acetylpyridine, aryl aldehyds and ammonium acetate under conventional heating and solvent-free reaction conditions with acceptable yields and brief times. For final step of the mechanistic route for the synthesis of target molecules we suggested a cooperative vinylogous anomeric based oxidation mechanism as a new mechanistic route. Also, Fe3O4@O2PO2(CH2)2NH3+CF3CO2− showed excellent reusability in the investigated multi-component reactions. Other advantage of presented protocol are overcoming to drawbacks of traditional procedures such as multi-step procedure, use of microwave irradiation, long reaction time, low yield, utilization of organic solvents like glycol and harmful reagents for environment such as sodium or potassium hydroxide and acetic acid.
Conflicts of interest
There are no conflicts to declare.
Acknowledgements
We thank the Bu-Ali Sina University and Iran National Science Foundation (INSF) (Grant Number: 98001912) for financial support.
Notes and references
- A. H. Lu, E. e. L. Salabas and F. Schüth, Angew. Chem., Int. Ed., 2007, 46, 1222 CrossRef CAS PubMed.
- R. N. Grass, E. K. Athanassiou and W. J. Stark, Angew. Chem., Int. Ed., 2007, 46, 4909 CrossRef CAS PubMed.
- G. M. Whitesides, C. L. Hill and J.-C. Brunie, Ind. Eng. Chem. Process Des. Dev., 1976, 15, 226 CrossRef CAS.
- P. D. Stevens, G. Li, J. Fan, M. Yen and Y. Gao, Chem. Commun., 2005, 4435 RSC.
- H. Lu, W. Schmidt, N. Matoussevitch, H. Bönnemann, B. Spliethoff, B. Tesche, E. Bill, W. Kiefer and F. Schüth, Angew. Chem., Int. Ed., 2004, 116, 4403 CrossRef.
- S. C. Tsang, V. Caps, I. Paraskevas, D. Chadwick and D. Thompsett, Angew. Chem., Int. Ed., 2004, 116, 5763 CrossRef.
- M. Sheykhan, L. Mamani, A. Ebrahimi and A. Heydari, J. Mol. Catal. A: Chem., 2011, 335, 253 CrossRef CAS.
- A. R. Kiasat and S. Nazari, J. Mol. Catal. A: Chem., 2012, 365, 80 CrossRef CAS.
- A. R. Kiasat and S. Nazari, J. Incl. Phenom. Macrocycl. Chem., 2013, 76, 363 CrossRef CAS.
- A. Pfeifer, K. Zimmermann and C. Plank, Pharm. Res., 2012, 29, 1161 CrossRef CAS PubMed.
- M. Z. Kassaee, H. Masrouri and F. Movahedi, Monatsh. Chem., 2010, 141, 317 CrossRef CAS.
- P. Li, L. Wang, L. Zhang and G. W. Wang, Adv. Synth. Catal., 2012, 354, 1307 CrossRef CAS.
- S. Wittmann, A. Schätz, R. N. Grass, W. J. Stark and O. Reiser, Angew. Chem., Int. Ed., 2010, 49, 1867 CrossRef CAS PubMed.
- B. Kaboudin, R. Mostafalu and T. Yokomatsu, Green Chem., 2013, 15, 2266 RSC.
- Y. Wang and J.-K. Lee, J. Mol. Catal. A: Chem., 2007, 263, 163 CrossRef CAS.
- L. M. Rossi, F. P. Silva, L. L. Vono, P. K. Kiyohara, E. L. Duarte, R. Itri, R. Landers and G. Machado, Green Chem., 2007, 9, 379 RSC.
- V. Polshettiwar and R. S. Varma, Org. Biomol. Chem., 2009, 7, 37 RSC.
- L. Aschwanden, B. Panella, P. Rossbach, B. Keller and A. Baiker, ChemCatChem, 2009, 1, 111 CrossRef CAS.
- B. Karimi and E. Farhangi, Chem.–Eur. J., 2011, 17, 6056 CrossRef CAS PubMed.
- T. Zeng, L. Yang, R. Hudson, G. Song, A. R. Moores and C.-J. Li, Org. Lett., 2010, 13, 442 CrossRef PubMed.
- B. Karimi and E. Farhangi, Adv. Synth. Catal., 2013, 355, 508 CAS.
- S. Rostamnia, K. Lamei, M. Mohammadquli, M. Sheykhan and A. Heydari, Tetrahedron Lett., 2012, 53, 5257 CrossRef CAS.
- M. Sheykhan, H. Mohammadnejad, J. Akbari and A. Heydari, Tetrahedron Lett., 2012, 53, 2959 CrossRef CAS.
- L. Reguera and D. G. Rivera, Chem. Rev., 2019, 119, 9836 CrossRef CAS PubMed.
- S. Zhi, X. Ma and W. Zhang, Org. Biomol. Chem., 2019, 17, 7632 RSC.
- E. M. de Marigorta, J. M. de L. Santos, A. M. O. de Retana and J. F. Palacios, Beilstein J. Org. Chem., 2019, 15, 1065 CrossRef CAS PubMed.
- S. Sadjadi, M. M. Heravi and N. Nazari, RSC Adv., 2016, 6, 53203 RSC.
- B. H. Rotstein, S. Zaretsky, V. Rai and A. K. Yudin, Chem. Rev., 2014, 114, 8323 CrossRef CAS PubMed.
- Q. Wang, D.-X. Wang, M. –X. Wang and J. Zhu, Acc. Chem. Res., 2018, 51, 1290 CrossRef CAS PubMed.
- D. Zhang and W. Hu, Chem. Rec., 2017, 17, 1 CrossRef PubMed.
- L. Levi and T. J. J. Muller, Chem. Soc. Rev., 2016, 45, 2825 RSC.
- M. Haji, Beilstein J. Org. Chem., 2016, 12, 1269 CrossRef CAS PubMed.
- C. Romers, C. Altona, H. R. Buys and E. Havinga, Top. Stereochem., 1969, 4, 39 CAS.
- E. Juaristi and G. Cuevas, Tetrahedron, 1992, 48, 5019 CrossRef CAS.
- P. P. Graczyk and M. Mikolajczyk, Top. Stereochem., 1994, 21, 159 CAS.
- N. S. Zefirov and N. M. Shekhman, Russ. Chem. Rev., 1971, 40, 315 CrossRef.
- E. Juaristi, Conformational Behavior of Six- Membered Rings, VCH Publishers, New York 1995 Search PubMed.
- E. Juaristi and G. Cuevas, The Anomeric Effect. CRC Press: Boca Raton, FL, 1994 Search PubMed.
- I. Tvaroska and J. P. Carver, J. Phys. Chem., 1996, 100, 11305 CrossRef CAS.
- J. F. King, R. Rathore, Z. Guo, M. Li and N. C. Payne, J. Am. Chem. Soc., 2000, 122, 10308 CrossRef CAS.
- J. F. King, M. Li, A. Z. Cheng and V. Dave, Heteroatom Chem, 2002, 13, 397 CrossRef CAS.
- E. Juaristi, L. Valle, C. Mora- Uzeta, B. A. Valenzuela, P. Joseph-Nathan and M. F. Fredrich, J. Org. Chem., 1982, 47, 5038 CrossRef CAS.
- E. Juaristi, L. Valle, B. A. Valenzuela and M. A. Aguilar, J. Am. Chem. Soc., 1986, 108, 2000 CrossRef CAS.
- G. P. Gomes, V. Vil, A. Terent’ev and I. V. Alabugin, Chem. Sci., 2015, 6, 6783 RSC.
- A. R. Katritzky, P. J. Steel and S. N. Denisenko, Tetrahedron, 2001, 57, 3309 CrossRef CAS.
- D. P. Curran and Y. G. Suh, Carbohydr. Res., 1987, 171, 161 CrossRef CAS PubMed.
- S. E. Denmark, M. S. Dappen, N. L. Sear and R. T. Jacobs, J. Am. Chem. Soc., 1990, 112, 3466 CrossRef CAS.
- M. D. Drew, M. C. Wall and J. T. Kim, Tetrahedron Lett., 2012, 53, 2833 CrossRef CAS.
- C. Jakel and K. H. Dotz, J. Organomet. Chem., 2001, 624, 172 CrossRef CAS.
- A. Nowacki, D. Walczak and B. Liberek, Carbohydr. Res., 2012, 352, 177 CrossRef CAS PubMed.
- A. Nowacki and B. Liberek, Carbohydr. Res., 2013, 371, 1 CrossRef CAS PubMed.
- M. Asgari and D. Nori-Shargh, Struct. Chem., 2017, 28, 1803 CrossRef CAS.
- A. Nowacki and B. Liberek, Carbohydr. Res., 2018, 462, 13 CrossRef CAS PubMed.
- R. Katritzky, P. J. Steel and S. N. Denisenko, Tetrahedron, 2001, 57, 3309 CrossRef.
- M. Yarie, Iran. J. Catal., 2017, 7, 85 CAS.
- M. Yarie, Iran. J. Catal., 2020, 10, 79 Search PubMed.
- S. Y. Cho, S. K. Kang, S. S. Kim, H. G. Cheon, J. K. Choi and E. K. Yum, Bull. Korean Chem. Soc., 2001, 22, 1217 CAS.
- L. Mao-Chin, L. Tai-Shun, C. G. Joseph, C. H. Ann and S. C. Alan, J. Med. Chem., 1996, 39, 2586 CrossRef PubMed.
- W. J. Yeong, B. I. Weon, K. R. Jae, M. J. Shim, B. K. Won and C. C. Eung, Bioorg. Med. Chem., 2004, 12, 5909 CrossRef PubMed.
- F. E. Goda, A. A.-M. Abdel-Aziz and O. A. Attef, Bioorg. Med. Chem., 2004, 12, 1845 CrossRef CAS PubMed.
- M. Satoshi, H. Takayuki and K. Mitsuo, J. Agric. Food Chem., 1997, 45, 2345 CrossRef.
- J. I. Sebat, A. J. Paszczynski, M. S. Cortese and R. I. Crawford, Appl. Environ. Microbiol., 2001, 67, 3924 CrossRef PubMed.
- M. Sylvie, R. Jean-Louis, G. Christophe, A. Hassan, S. Robert, A. Graciella, D. C. Erik, B. Jan and G. Alain, Bioorg. Med. Chem., 2002, 10, 941 CrossRef.
- G. H. Mikail, M. R. Arnold and G. F. Scott, J. Med. Chem., 2001, 44, 1560 CrossRef PubMed.
- A. Tiwari, W. R. Waud and R. F. Struck, Bioorg. Med. Chem., 2002, 10, 3593 CrossRef CAS PubMed.
- K. C. Nicolaou, R. Scarpelli, B. Bollbuck, B. Werschkun, M. Pereira, M. Wartmann, K. Altmann, D. Zaharevitz, R. Gussio and P. Giannakakou, Chem. Biol., 2000, 7, 593 CrossRef CAS PubMed.
- D. Kovala-Demertzi, A. Boccarelli, M. A. Demertzis and M. Coluccia, Chemotherapy, 2007, 53, 148 CrossRef CAS PubMed.
- L. Mao-Chin, L. Tai-Shun and S. C. Alan, J. Med. Chem., 1992, 35, 3667 CrossRef PubMed.
- W. Yuqiang, L. Mao-Chin, L. Tai-Shun and S. C. Alan, J. Med. Chem., 1992, 35, 3672 CrossRef PubMed.
- C. Altomare, S. Cellamare, L. Summo, P. Fossa, L. Mosti and A. Carotti, Bioorg. Med. Chem., 2000, 8, 909 CrossRef CAS PubMed.
- J.-M. Lehn, Supramolecular Chemistry, Concepts and Perspectives, VCH: Weinheim, 1995 Search PubMed.
- B. N. Trawick, A. T. Daniher and J. K. Bashkin, Chem. Rev., 1998, 98, 939 CrossRef CAS PubMed.
- H. C. Latham, P. R. Cook, A. Rodger and G. Lowe, FEBS Lett., 1996, 380, 73 CrossRef.
- M. J. Clarke, Coord. Chem. Rev., 2003, 236, 209 CrossRef CAS.
- A. Islam, H. Sugihara and H. J. Arakawa, Photochem. Photobiol. A, 2003, 158, 131 CrossRef CAS.
- G. Chelucci and R. P. Thummel, Chem. Rev., 2002, 102, 3129 CrossRef CAS PubMed.
- C. Li, W. Fan and D. A. Straus, J. Am. Chem. Soc., 2004, 126, 7750 CrossRef CAS PubMed.
- K. C. Jantunen and B. L. Scott, J. Am. Chem. Soc., 2006, 128, 6322 CrossRef CAS PubMed.
- L. Raehm and C. Hamann, Org. Lett., 2000, 2, 1991 CrossRef CAS PubMed.
- M. Barquın, J. Cancela, M. J. Gonzalez Garmendia, J. Quintanilla and U. Amador, Polyhedron, 1998, 17, 2373 CrossRef.
- G. W. V. Cave and C. L. Raston, J. Supramol. Chem., 2002, 2, 317 CrossRef CAS.
- L. Hou and D. Li, Inorg. Chem. Commun., 2005, 8, 190 CrossRef CAS.
- X.-Z. Li, M. Li, Z. Li, J.-Z. Hou, X.-C. Huang and D. Li, Angew. Chem., Int. Ed., 2008, 47, 6371 CrossRef CAS PubMed.
- E. C. Constable, G. Zhang, C. E. Housecroft, M. Neuburger and J. A. Zampese, CrystEngComm, 2009, 11, 2279 RSC.
- E. C. Constable, G. Zhang, E. Coronado, C. E. Housecroft and M. Neuburger, CrystEngComm, 2010, 12, 2139 RSC.
- E. C. Constable, G. Zhang, C. E. Housecroft, M. Neuburger and J. A. Zampese, CrystEngComm, 2010, 12, 2146 RSC.
- E. C. Constable, G. Zhang, C. E. Housecroft, M. Neuburger and J. A. Zampese, CrystEngComm, 2010, 12, 3733 RSC.
- B.-C. Wang, Q.-R. Wu, H.-M. Hu, X.-L. Chen, Z.-H. Yang, Y.-Q. Shangguan, M.-L. Yang and G.-L. Xue, CrystEngComm, 2010, 12, 485 RSC.
- J. Song, B.-C. Wang, H.-M. Hu, L. Gou, Q.-R. Wu, X.-L. Yang, Y.-Q. Shangguan, F.-X. Dong and G.-L. Xue, Inorg. Chim. Acta, 2011, 366, 134 CrossRef CAS.
- J. Heine, J. Schmedt auf der Günne and S. Dehnen, J. Am. Chem. Soc., 2011, 133, 10018 CrossRef CAS PubMed.
- X.-Z. Li, X.-P. Zhou, D. Li and Y. –G. Yin, CrystEngComm, 2011, 13, 6759 RSC.
- K.-R. Ma, F. Ma, Y.-L. Zhu, L.-J. Yu, X.-M. Zhao, Y. Yang and W.-H. Duan, Dalton Trans., 2011, 40, 9774 RSC.
- J. Song, B.-C. Wang, H.-M. Hu, L. Gou, Q.-R. Wu, X.-L. Yang, Y.-Q. Shangguan, F.-X. Dong and G.-L. Xue, Inorg. Chim. Acta, 2011, 366, 134 CrossRef CAS.
- E. C. Constable, G. Zhang, C. E. Housecroft and J. A. Zampese, CrystEngComm, 2011, 13, 6864 RSC.
- E. C. Constable, C. E. Housecroft, P. Kopecky, M. Neuburger, J. A. Zampese and G. Zhang, CrystEngComm, 2012, 14, 446 RSC.
-
(a) S. Tu, T. Li, F. Shi, F. Fang, S. Zhu, X. Wei and Z. Zong, Chem. Lett., 2005, 34, 732 CrossRef CAS;
(b) J. Dehaudt, J. Husson and L. Guyard, Green Chem., 2011, 13, 3337 RSC.
- S. Tu, T. Li, F. Shi, Q. Wang, J. Zhang, J. Xu, X. Zhu, X. Zhang, S. Zhu and D. Shi, Synthesis, 2005, 3045 CrossRef CAS.
- Y. Ma, S. Zhang, H. Wei, Y. Dong, L. Shen, S. Liu, Q. Zhao, L. Liu and W.-Y. Wong, Dalton Trans., 2018, 47, 5582 RSC.
- T. A. Matias, A. P. Mangoni, S. H. Toma, F. N. Rein, R. C. Rocha, H. E. Toma and K. Araki, Eur. J. Inorg. Chem., 2016, 2016, 5547 CrossRef CAS.
- A. Hussain, S. Gadadhar, T. K. Goswami, A. A. Karande and A. R. Chakravarty, Eur. J. Med. Chem., 2012, 50, 319 CrossRef CAS PubMed.
- R. J. Sullivan, J. Kim, C. Hoyt, L. A. Silks and M. Schlaf, Polyhedron, 2016, 108, 104 CrossRef CAS.
- P. C. Mondal and A. K. Manna, New J. Chem., 2016, 40, 5775 RSC.
- G. Wang, X. Fu, L. He, Q. Miao and G. Peng, Chem. Phys. Lett., 2014, 614, 243 CrossRef CAS.
- C.-L. Liu, C.-J. Zheng, X.-K. Liu, Z. Chen, J.-P. Yang, F. Li, X.-M. Ou and X.-H. Zhang, J. Maert. Chem. C, 2015, 3, 1068 RSC.
- S. Noura, M. Ghorbani, M. A. Zolfigol, M. Narimani, M. Yarie and M. Oftadeh, J. Mol. Liq., 2018, 271, 778 CrossRef CAS.
- F. Karimi, M. A. Zolfigol and M. Yarie, Mol. Catal., 2019, 463, 20 CrossRef CAS.
- J. Afsar, M. A. Zolfigol, A. Khazaei, M. Zarei, Y. Gu, D. A. Alonso and A. Khoshnood, Mol. Catal., 2020, 482, 110666 CrossRef CAS.
- S. Kalhor, M. Yarie, M. Rezaeivala and M. A. Zolfigol, Res. Chem. Intermediat., 2019, 45, 3453 CrossRef CAS.
-
(a) P. Ghasemi, M. Yarie, M. A. Zolfigol, A. A. Taherpour and M. Torabi, ACS Omega, 2020, 5, 3207 CrossRef CAS PubMed;
(b) S. Babaee, M. Zarei, H. Sepehrmansourie, M. A. Zolfigol and S. Rostamnia, ACS Omega, 2020, 5, 6240 CrossRef CAS PubMed.
- M. Torabi, M. Yarie and M. A. Zolfigol, Appl. Organometal. Chem., 2012, 33, 4933 CrossRef.
- H. L. Anderson, S. Anderson and J. K. M. Sanders, J. Chem. Soc. Perkin Trans. I, 1995, 2231 RSC.
- Y. M. Klein, E. C. Constable, C. E. Housecroft, J. A. Zampese and A. Crochet, CrystEngComm, 2014, 16, 9915 RSC.
- J. Yoshida, S.-I. Nishikiori and H. Yuge, J. Coord. Chem., 2013, 66, 4344 CrossRef CAS.
- F. Kroehnke, Synthesis, 1976, 1 CrossRef CAS.
- E. C. Constable, C. E. Housecroft, M. Neuburger, J. Schönle, S. Vujovic and J. A. Zampese, Polyhedron, 2013, 60, 120 CrossRef CAS.
- K. W. Merz and R. Barchet, Arch. Pharm. Ber. Dtsch. Pharm. Ges., 1964, 297, 423 CrossRef CAS PubMed.
- E. C. Constable, C. E. Housecroft, M. Neuburger, S. Vujovic, J. A. Zampese and G. Zhang, CrystEngComm, 2012, 14, 3554 RSC.
- R. L. Frank and R. W. Meikle, J. Am. Chem. Soc., 1950, 72, 4184 CrossRef CAS.
- S. A. Sotnik, R. A. Polunin, M. A. Kiskin, A. M. Kirillov, V. N. Dorofeeva, K. S. Gavrilenko, I. L. Eremenko, V. M. Novotortsev and S. V. Kolotilov, Inorg. Chem., 2015, 54, 5169 CrossRef CAS PubMed.
- M. N. Patel, D. S. Gandhi and P. A. Parmar, Spectrochim. Acta, Part A, 2011, 84, 243 CrossRef CAS PubMed.
- B.-S. Jeong, H. Choi, Y.-S. Kwak and E.-S. Lee, Bull. Korean Chem. Soc., 2011, 32, 3566 CrossRef CAS.
- X.-J. Long, J.-W. Dai and J.-Z. Wu, J. Coord. Chem., 2012, 65, 316 CrossRef CAS.
- T. Ezhilarasu, A. Sathiyaseelan, P. T. Kalaichelvan and S. Balasubramanian, J. Mol. Struct., 2017, 1134, 265 CrossRef CAS.
Footnote |
† Electronic supplementary information (ESI) available. See DOI: 10.1039/d0ra04461j |
|
This journal is © The Royal Society of Chemistry 2020 |