DOI:
10.1039/D0RA04167J
(Paper)
RSC Adv., 2020,
10, 23250-23253
A new oxygen-rich energetic salt dihydrazine tetranitroethide: a promising explosive alternative with high density and good performance†
Received
27th March 2020
, Accepted 4th June 2020
First published on 17th June 2020
Abstract
A novel high-energy salt with good oxygen balance, dihydrazine tetranitroethide (5), has been synthesized and characterized by FT-IR spectroscopy, NMR spectroscopy, elemental analysis, and X-ray single crystal diffraction. Compound 5 exhibits high crystal density (1.81 g cm−3) and impressive detonation velocity (9508 m s−1) and detonation pressure (37.9 GPa), showing potential applications as a high performance explosive and a promising additive of propellants.
For applications in propellants, explosives and pyrotechnics, new energetic materials are required with high energy, insensitivity, high thermal stability and environment-friendly decomposition gases.1 To achieve these goals, chemists have adopted a variety of strategies, such as construction of nitrogen-rich compounds,2 energetic salts,3 metal organic framework (MOFs)4 and cage structure molecules.5 Oxygen balance (OB) is an important parameter to weigh the detonation performance of the nitrogen-rich compounds. Generally explosives may exhibit good performance when the OB close to zero.6 One conventional method of increasing the OB of energy materials is to introduce nitro groups, a typical high-energy and oxygen-rich substituent.7 Recently, gem-nitro and poly-nitro fragments, such as dinitromethyl,8 trinitroethyl9 and trinitromethyl,10 have been of interest to researchers. The compounds consisting of poly-nitro groups exhibit good OB, which largely improves their detonation velocities and pressures.11 In our recent study,12 we considered using tetranitroethylene instead of nitro as a pre-packaged module, linked to insensitive backbones, just like building blocks. The tetranitroethylene fragment has a high nitrogen content, a positive OB, and high energy within the molecule.13 The molecules consisting of the tetranitroethylene fragment are expected to be excellent energetic materials with superior properties.12 But tetranitroethylene is an unstable intermediate, which is difficult to isolate.14 Hexnitroethane,15 a stable tetranitroethylene derivative, can be effectively used to synthetize poly-nitro bridged compound (Scheme 1). Previously, we discussed the possibility of constructing poly-nitro bridged-ring compounds with nitrogen-containing heterocycles and tetranitroethylene by using the Diels–Alder reaction.12,16
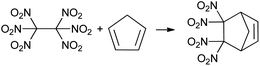 |
| Scheme 1 Synthetic pathway for poly-nitro bridged compound.14 | |
Dipotassium tetranitroethide,17 another tetranitroethylene derivative, lead another thought to synthesize high energy salts with the tetranitroethane anion. The salt-based energetic materials often exhibit lower vapour pressure and higher densities than the non-ionic energetic materials.3c In addition, the energetic salt can improve the properties by selecting different constituent ions.18 Based on the advantages of high-energy salt, we chose tetranitroethane anion and hydrazine cation to construct energetic salt by considering both energy and sensitivity. The synthetic route can be seen in Scheme 2. The good OB and large amounts of hydrogen-bonds prompt the target compound (dihydrazine tetranitroethide, 5) with suitable sensitivity and good performance. 5 possesses a crystal density of 1.81 g cm−3, detonation velocity of 9508 m s−1 and detonation pressure of 37.9 GPa, which are higher than those of RDX. In addition, the salt-formation improves the carcinogenic and high toxic properties of hydrazine.
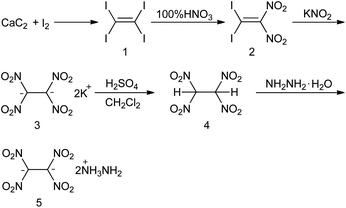 |
| Scheme 2 The route to synthesis of 5. | |
The intermediate dipotassium tetranitroethide (3) was prepared from tetraiodoethylene (1) after two nitrification reactions, according to literature.19 The suspension of 3 in dichloromethane dissolves in concentrated sulfuric acid to give the solution of tetranitroethane (4) in dichloromethane. Treatment of the solution of 4 with hydrazine hydrate resulted in dihydrazine tetranitroethide (5), a yellow solid precipitated, that was confirmed by single crystal X-ray diffraction. Single crystal 5 is crystallized by using the method of the evaporation of water.
Tetranitroethane 4 is also an unstable compound that is soluble in dichloromethane and difficult to isolate. It decomposed and released a brown gas as the solvent removed. Thus, we analysed the UV spectrum of the solution of 4, instead of the pure compound 4. Compared with the experimental and calculated UV spectrum of solution 4 (Fig. 1), we find that the maximum absorption wavelengths of the two curves match well, 240 nm (tested) and 239.5 nm (calculated). It can be inferred that compound 4 would be tetranitroethane.
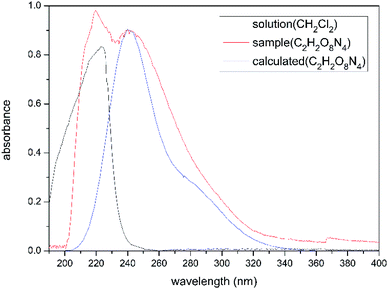 |
| Fig. 1 The UV spectrum curve of tetranitroethane 4. | |
Dihydrazine tetranitroethide 5 in the monoclinic space group C2/c with a good density of 1.81 g cm−3 (298 K). The gem-dinitro group is nearly planar, with the torsion angle of O5–N3–C2–N4, 179.52(17)°. However, the torsion angle of N3–C2–C2′–N4′ and N4–C2–C2′–N3′ are 77.57 (255)° and 77.57 (255)°, respectively, which shows the twist of adjacent dinitromethyl groups is caused by the steric effect. The strong hydrogen-bond interactions are presented between ammonium and nitro groups (Fig. 2b). The details of donor–acceptor distance are given in the ESI.† Many studies have shown that the hydrogen-bonds enhance the stability of energetic molecules. At the molecular level, intermolecular hydrogen bonds between hydrazine cation and nitro groups play an important role in stabilizing energetic compounds. This kind of hydrogen-bonding interaction and cation–anion contact in the energetic salt is suggested as part of the explanation for closer packing, which causes the good density. As seen in Fig. 2c, the tetranitroethide anions are found in cross-stacking arrangements and layer by layer. While the hydrazine cation in the crystal, as the adhesive between the bricks, help to create a better stacking arrangement.
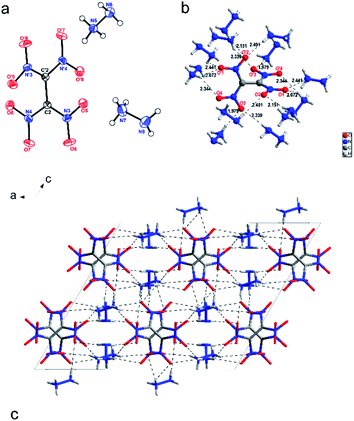 |
| Fig. 2 (a) Molecular structure of 5; (b) hydrogen-bonding interactions of 5 between hydrazine cation and tetranitroethide anion; (c) packing diagram of 5 (unit cell viewed along the b axis). | |
The physical properties and calculated detonation performances are summarized in Table 1. 5 exhibits moderate thermal stability with a decomposition temperature of 105 °C. The OB of 5 (5.8%) is significantly enhanced by four nitro groups. OB is calculated based on the formation of carbon monoxide during the combustion process. The oxygen content of 5 is 46.7% which exceeds that of hexogen (RDX, 43.2%). The density of 5 is 1.81 g cm−3, which is a high grade in organic energetic salt. The heat of combustion (ΔcH) is measured by oxygen bomb calorimetry; the ΔcH of compound 5 is 7797 J g−1. The heat of formation (ΔfH), −17.4 kJ mol−1, is obtained from the heat of combustion by Hess's law. Using the density and the calculated ΔfH, the detonation performance including detonation velocity (VD) and detonation pressure (P) were evaluated using EXPLO5 program package.20 Compound 5 has a detonation velocity of 9508 m s−1 and a detonation pressure of P: 37.9 GPa, which is better than RDX (8748 m s−1; 34.9 GPa) and similar to HMX (9320 m s−1; 39.5 GPa). The sensitivities to impact and friction are 1.25 J and 34 N, respectively.
Table 1 Physical properties of dihydrazine tetranitroethide 5 and comparison with ADN, RDX and HMX
Comp. |
5 |
ADNi |
RDXj |
HMXj |
Oxygen balance (based on CO) for CaHbOcNd, 1600 (c − a − b/2)/Mw, Mw = molecular weight. Density calculated by crystal information. Heat of formation. Decomposition temperature (onset) under nitrogen gas (DSC, 5 °C min−1). Detonation velocity (calculated with Explo5 v6.01). Detonation pressure (calculated with Explo5 v6.01). Impact sensitivity. Friction sensitivity. Ref. 6a. Ref. 3c. |
OBa [%] |
5.8 |
25.8 |
0 |
0 |
db [g cm−3] |
1.81 |
1.81 |
1.82 |
1.91 |
ΔfHc [kJ mol−1] |
−17.4 |
−149.8 |
93 |
105 |
Tdd [°C] |
105 |
159 |
230 |
287 |
VDe [m s−1] |
9508 |
7860 |
8977 |
9320 |
Pf [GPa] |
37.9 |
23.6 |
35.2 |
39.6 |
ISg [J] |
1.25 |
3–5 |
7.4 |
7.4 |
FSh [N] |
34 |
64–72 |
120 |
120 |
In summary, the energetic salt 5 was synthesized and fully characterized by FT-IR, 1H NMR, 13C NMR, DSC and elemental analysis. The structure of 5 was confirmed by single-crystal X-ray diffraction analysis. The results show that 5 has high crystal density of 1.81 g cm−3, 298 K, positive oxygen balance (5.8%), good sensitivities (IS = 1.25 J, FS = 34 N), and good detonation properties (VD = 9508 m s−1, P = 37.9 GPa). Its promising energetic properties highlight the application prospects as a high rocket propellant constituent.
Experimental section
Synthesis of dihydrazine teranitroethide (5)
Synthesis of dipotassium tetranitroethide (3) was based on a literature procedure.19 Concentrated sulfuric acid was added to a suspension of 3 (4 mmol, 1.1 g) dichloromethane (30 mL) at −40 °C and stirred for 1 h. Then the mixture was treated by a quick separation using a separating funnel, which gave the solution of tetranitroethylene in dichloromethane 4 (3 mmol, quantitative analysis using ultraviolet spectrum). Hydrazine hydrate (6 mmol) was added dropwise to the tetranitroethylene solution at −40 °C, collected the yellow precipitate by suction filtration, yield 5 (0.65 g). Yellow solid, 80% yield. Td (onset): 105 °C. 1H NMR (400 MHz, deuterium oxide): δ 8.26 (s, 1H) ppm. 13C NMR (101 MHz, deuterium oxide): δ 166.61 ppm. IR (KBr pellet): v 3436, 3325, 3137, 1611, 1540, 1462, 1308 cm−1. C2H10N8O8 (274.15): calcd C, 8.76; H, 3.68; N, 40.87%. Found: C, 8.10; H, 3.71; N, 41.23%.
Theoretical section
The low-lying singlet electronic transition was explored using the time-dependent DFT (TD-DFT) method at the CAM-B3LYP/6-31G* level of theory to simulate the UV spectra of compound 4.21 The IEFPCM continuum solvent model was adopted to simulate the dichloromethane surrounding. All calculations were performed with the Gaussian 09 suite of programs.22
Conflicts of interest
There are no conflicts to declare.
Acknowledgements
We are thankful to the projects of NSAF (U1830134), NSFC (21905023 and 21911530096), China Postdoctoral Science Foundation funded project (2018M640080), State Key Laboratory of Explosion Science and Technology (ZDKT18-02) for their generous financial support.
Notes and references
- M. A. Petrie, J. A. Sheehy, J. A. Boatz, G. Rasul, G. K. S. Prakash, G. A. Olah and K. O. Christe, J. Am. Chem. Soc., 1997, 119, 8802–8808 CrossRef CAS.
-
(a) D. Fischer, J. L. Gottfried, T. M. Klapoetke, K. Karaghiosoff, J. Stierstorfer and T. G. Witkowski, Angew. Chem., Int. Ed., 2016, 55, 16132–16135 CrossRef CAS PubMed;
(b) M. H. V. Huynh, M. A. Hiskey, E. L. Hartline, D. P. Montoya and R. Gilardi, Angew. Chem., Int. Ed., 2004, 43, 4924–4928 CrossRef CAS PubMed;
(c) N. Fischer, D. Izsak, T. M. Klapoetke, S. Rappenglueck and J. Stierstorfer, Chem.–Eur. J., 2012, 18, 4051–4062 CrossRef CAS PubMed;
(d) W. Q. Zhang, J. H. Zhang, M. C. Deng, X. J. Qi, F. D. Nie and Q. H. Zhang, Nat. Commun., 2017, 8, 1–7 CrossRef PubMed.
-
(a) J. Zhang, Q. Zhang, T. T. Vo, D. A. Parrish and J. M. Shreeve, J. Am. Chem. Soc., 2015, 137, 1697–1704 CrossRef CAS PubMed;
(b) L. Hu, P. Yin, G. Zhao, C. L. He, G. H. Imler, D. A. Parrish, H. X. Gao and J. M. Shreeve, J. Am. Chem. Soc., 2018, 140, 15001–15007 CrossRef CAS PubMed;
(c) H. Gao and J. M. Shreeve, Chem. Rev., 2011, 111, 7377–7436 CrossRef CAS PubMed;
(d) J. Zhang, L. A. Mitchell, D. A. Parrish and J. M. Shreeve, J. Am. Chem. Soc., 2015, 137, 10532–10535 CrossRef CAS PubMed.
-
(a) S. Zhang, Q. Yang, X. Liu, X. Qu, Q. Wei, G. Xie, S. Chen and S. Gao, Coord. Chem. Rev., 2016, 307, 292–312 CrossRef CAS;
(b) J. Zhang and J. N. M. Shreeve, Dalton Trans., 2016, 45, 2363–2368 RSC;
(c) Y. Tang, C. He, L. A. Mitchell, D. A. Parrish and J. n. M. Shreeve, Angew. Chem., Int. Ed., 2016, 55, 5565–5567 CrossRef CAS PubMed.
-
(a) R. L. Simpson, P. A. Urtiew, D. L. Ornellas, G. L. Moody, K. F. J. Scribner and D. M. Hoffman, Propellants, Explos., Pyrotech., 1997, 22, 249–255 CrossRef CAS;
(b) X. Song, J. Li, H. Hou and B. Wang, J. Comput. Chem., 2009, 30, 1816–1820 CrossRef CAS PubMed;
(c) P. E. Eaton, M. X. Zhang, R. Gilardi, N. Gelber, S. Iyer and R. Surapaneni, Propellants, Explos., Pyrotech., 2002, 27, 1–6 CrossRef CAS;
(d) T. Hou, H. Ruan, G. Wang and J. Luo, Eur. J. Org. Chem., 2017, 6957–6960, DOI:10.1002/ejoc.201701403;
(e) T. J. Hou, J. Zhang, C. J. Wang and J. Luo, Org. Chem. Front., 2017, 4, 1819–1823 RSC.
-
(a) X. X. Zhao, S. H. Li, Y. Wang, Y. C. Li, F. Q. Zhao and S. P. Pang, J. Mater. Chem. A, 2016, 4, 5495–5504 RSC;
(b) P. He, J.-G. Zhang, K. Wang, X. Yin and T.-L. Zhang, J. Org. Chem., 2015, 80, 5643–5651 CrossRef CAS PubMed.
- Q. Yu, P. Yin, J. H. Zhang, C. L. He, G. H. Imler, D. A. Parrish and J. M. Shreeve, J. Am. Chem. Soc., 2017, 139, 8816–8819 CrossRef CAS PubMed.
-
(a) Y. X. Tang, C. L. He, G. H. Imler, D. A. Parrish and J. M. Shreeve, Dalton Trans., 2019, 48, 7677–7684 RSC;
(b) J. C. Zhang, J. H. Zhang, D. A. Parrish and J. M. Shreeve, J. Mater. Chem. A, 2018, 6, 22705–22712 RSC;
(c) Y. Tang, C. He, G. H. Imler, D. A. Parrish and J. N. M. Shreeve, Chem.–Eur. J., 2017, 23, 16401–16407 CrossRef CAS PubMed;
(d) J. Zhang, S. Dharavath, L. A. Mitchell, D. A. Parrish and J. N. M. Shreeve, J. Am. Chem. Soc., 2016, 138, 7500–7503 CrossRef CAS PubMed;
(e) C. L. He and J. M. Shreeve, Angew. Chem., Int. Ed., 2016, 55, 772–775 CrossRef CAS PubMed.
- P. Yin, Q. Zhang, J. Zhang, D. A. Parrish and J. N. M. Shreeve, J. Mater. Chem. A, 2013, 1, 7500–7510 RSC.
-
(a) T. M. Klapotke, B. Krumm, T. Reith and C. C. Unger, J. Org. Chem., 2018, 83, 10505–10509 CrossRef PubMed;
(b) S. Dharavath, J. Zhang, G. H. Imler, D. A. Parrish and J. N. M. Shreeve, J. Mater. Chem. A, 2017, 5, 4785–4790 RSC.
- D. E. Chavez, D. A. Parrish and L. Mitchell, Angew. Chem., Int. Ed., 2016, 55, 8666–8669 CrossRef CAS PubMed.
- P. He, H. Z. Mei, L. Wu, J. Q. Yang, J. G. Zhang, A. Cohen and M. Gozin, J. Phys. Chem. A, 2018, 122, 3320–3327 CrossRef CAS PubMed.
- P. Yin, Q. Yu, S. Dharavath and J. N. M. Shreeve, J. Mater. Chem. A, 2018, 6, 15815–15820 RSC.
- D. T. Kurt Baum, J. Org. Chem., 1985, 50, 4 CrossRef.
- T. S. Griffin and K. Baum, J. Org. Chem., 1980, 45, 2880–2883 CrossRef CAS.
- J. Q. Yang, X. D. Gong, H. Z. Mei, T. Li, J. G. Zhang and M. Gozin, J. Org. Chem., 2018, 83, 14698–14702 CrossRef CAS PubMed.
- M. Dyke and R. L. Sass, J. Phys. Chem., 1968, 72, 266–268 CrossRef CAS.
- P. K. Swain, H. Singh and S. P. Tewari, J. Mol. Liq., 2010, 151, 87–96 CrossRef CAS.
- K. Baum, S. S. Bigelow, N. V. Nguyen, T. G. Archibald, R. Gilardi, J. L. Flippenanderson and C. George, J. Org. Chem., 1992, 57, 235–241 CrossRef CAS.
- M. Sućeska, EXPLO5 V6.01 Program, Brodarski Institute, Zagreb, Croatia, 2012 Search PubMed.
- R. Improta and V. Barone, J. Am. Chem. Soc., 2004, 126, 14320–14321 CrossRef CAS PubMed.
- M. J. Frisch, G. W. Trucks, H. B. Schlegel, G. E. Suzerain, M. A. Robb, J. J. R. Cheeseman, J. A. Montgomery, T. Vreven, K. N. Kudin, J. C. Burant, J. M. Millam, S. S. Iyengar, J. Tomasi, V. Barone, B. Mennucci, M. Cossi, G. Scalmani, N. Rega, G. Petersson, H. Nakatsuji, M. Hada, M. Ehara, K. Toyota, R. Fukuda, J. Hasegawa, M. Ishida, T. Nakajima, Y. Honda, O. Kitao, H. Nakai, M. Klene, X. Li, J. E. Knox, H. P. Hratchian, J. B. Cross, V. Bakken, C. Adamo, J. Jaramillo, R. Gomperts, R. E. Stratmann, O. Yazyev, A. J. Austin, R. Cammi, C. Pomelli, J. W. Ochterski, P. Y. Ayala, K. Morokuma, G. A. Voth, P. Salvador, J. J. Dannenberg, V. G. Zakrzewski, S. Dapprich, A. D. Daniels, M. C. Strain, O. Farkas, D. K. Malick, A. D. Rabuck, K. Raghavachari, J. B. Foresman, J. V. Ortiz, Q. Cui, A. G. Baboul, S. Clifford, J. Cioslowski, B. Stefanov, G. Liu, A. Liashenko, P. Piskorz, I. Komaromi, R. L. Martin, D. J. Fox, T. Keith, M. A. Al-Laham, C. Y. Peng, A. Nanayakkara, M. Challacombe, P. M. W. Gill, B. Johnson, W. Chen, M. W. Wong, C. Gonzalez and J. A. Pople, Gaussian 09, Revision A.02, Wallingford, 2009 Search PubMed.
Footnote |
† Electronic supplementary information (ESI) available: Includes experimental procedure, DSC plot, NMR spectra, and crystallographic data. CCDC 1946324. For ESI and crystallographic data in CIF or other electronic format see DOI: 10.1039/d0ra04167j |
|
This journal is © The Royal Society of Chemistry 2020 |