DOI:
10.1039/D0RA03825C
(Paper)
RSC Adv., 2020,
10, 25418-25425
Co-delivery of chlorantraniliprole and avermectin with a polylactide microcapsule formulation
Received
28th April 2020
, Accepted 29th June 2020
First published on 3rd July 2020
Abstract
Improving drug utilization of water-based pesticide formulations is facile and feasible to efficiently avoid serious pesticide residues and reduce the resulting environmental pollution. A co-delivery system of two- or multi-pesticides within one formulation could enhance drug efficiency and decrease usage amounts of pesticides due to the synergistic effect of the loaded multiple pesticides. Herein, we reported a porous polylactide (PLA) microcapsule formulation for co-delivery of avermectin (Av) and chlorantraniliprole (CAP). A double emulsion method combined with premix membrane emulsion (PME) was adopted to produce the Av/CAP-loaded porous microcapsules (Av/CAP P-MCs) with prolonged drug release, high loading content and entrapment efficiency, as well as good light and thermal stability. Compared with single Av- or CAP-loaded microcapsule formulations, the Av/CAP P-MCs exhibited higher biotoxicity against Plutella xylostella. These results reveal that the Av/CAP co-delivery system may be a promising candidate to be further explored as a facile, effective and environmentally-friendly pesticide formulation.
Introduction
Pesticides have widely been used to improve productivity and quality of food in agriculture.1 Traditional commercial pesticide formulations mainly include suspension concentrates, emulsifiable concentrates and wettable powders.2–6 Among them, emulsifiable concentrates contain considerable organic solvents. It is well documented that these traditional formulations lose more than 80% of applied pesticides in the spraying process because of biodegradation, chemical degradation, photolysis and evaporation. To replace this loss and keep efficient concentration of pesticides, repeated applications of traditional formulations result in excessive pesticide and organic solvent residues, which is seriously harmful to both the environment and humans.7–9 To reduce pesticide residues and environmental pollution as far as possibly, pesticide encapsulation with controlled drug release behaviors to improve pesticide utilization have received an increasing interest.10–16
Chlorantraniliprole (CAP) and avermectin (AV) are two popular broad-spectrum pesticides with low toxicity and no cross-resistance to existing pesticides.17–19 CAP is a selective insecticide belonging to the family of antranilic diamides. CAP can permeate into the plant through the leaf surface and also be absorbed by root, so it has long persistence and good stability.20 By exciting the insect ryanodine receptors in muscle and nervous tissue, CAP can trigger uncontrolled release of calcium from intracellular stores, causing cessation, lethargy, muscle paralysis and finally death of target organisms.21–23 Av, an extensively used insecticide, acaricide and nematicide, is activate stomach toxicity that has an effect on keeping the γ-aminobutyric acid-gated chloride channels, glutamate-gated chloride channel, and other chlorine channels open in insect muscle membranes, resulting in interdicting the synaptic transmission from internuncial neurons to motor nerve cells and the synaptosome peripheral nerve conduction between the neuromuscular system.24 Av can disturb the nerve of pest, but its residual activity is not ideal.25
“Synergy” can definite as two or more chemicals providing a larger effect than predicted implies which we can estimate joint effects of chemicals under inevitable presumption. Cedergreen et al. defined about synergy mechanism on pesticide toxicity that it occurs from chemical interaction.26 Interactions between chemicals can mainly affect six processes that are significant to the resultant toxicity of a chemical towards an organism: bioavailability, uptake, internal transportation, metabolization, binding at the target site and excretion. Currently, there only have a few reports to confirm that mixture of Av and CAP in various concentrations has a synergistic effect and higher efficiency compared to Av or CAP solely.27,28 In previous reports, we adopted double-emulsion method with premix membrane emulsion (PME) to develop PLA and starch uniform microcapsule formulations for controlled release of CAP and Av, and their control efficacies against Plutella xylostella were similar to the commercial formulations.29,30
Multidrug or drug/gene co-delivery systems, allowing the consecutive release of two or more drugs and genes, have extensively developed for cancer therapy since simultaneous delivery of multidrug can improve therapeutic outcomes, synergistic effects, and targeting moiety.31–37 Various carriers including liposomes, micelles, inorganic nanoparticles, and nanovesicles have been reported for constructing high-efficiency and safe co-delivery systems.38–43 For this tactics, co-delivery of two or more pesticides within one carrier will allow the administration of efficient drug concentration at lower doses and then help to greatly reduce pesticide residues and environmental pollution. Although co-delivery systems for pesticides are seldom reported, an efficient and safe co-delivery carrier is necessary for developing novel environment-friendly pesticide formations.
Our previous works have fabricated several microcapsule formations for controlled release of Lambda-Cyhalothrin (LC), Av or CAP.29,30,44 A W/O/W double emulsion method combined with premix membrane emulsion (PME) was adopted to produce uniform starch and polylactide (PLA) microcapsules with high loading contents. Wherein, LC and Av were loaded in microcapsule shells, but CAP was inside cavity of microcapsules due to lower solubility of CAP in organic solvent in comparison to LC and Av. The bioassay studies have proved that control efficacies of these microcapsule formulations against P. xylostella were compared even superior to the corresponding commercial formulations of LC, Av and CAP.
In this study, we utilized PME combined with S/O/W double emulsion method to further prepare novel AV/CAP co-delivery microcapsule formulations by simultaneously encapsulating soluble Av into PLA shells and excessively insoluble CAP inside the microcapsules. Adjusting osmotic agents and process parameters of PME, we produced various porous microcapsules with controlled surface morphology and size. The Av and CAP release behaviors could be precisely tuned by regulating the size and surface morphology. The Av/CAP-loaded microcapsule formulation possessed good thermal and light stability, showing superior control efficiency on P. xylostella to the commercial Av and CAP formulations.
Materials and methods
Chemicals
Polylactide (PLA) was kindly provided by Dongguan Zhuyou Plastic Co., Ltd (Dongguan, China). Bovine serum albumin (BSA) was obtained from Beijing Biodee Biotechnology Co., Ltd (Beijing, China). Commercial avermectin (Av) (18 g L−1) was purchased from Shandong Zouping Pesticide Co., Ltd. Commercial chlorantraniliprole (CAP) (200 mg mL−1) was purchased from Shanghai Shengnong Pesticide Co., Ltd. Technical Av (94.3%) and CAP (95%) were purchased from Qilu Pharmaceutical (Inner Mongolia) Co., Ltd (Huhhot, China) and DuPont Co., Ltd, respectively. Poly(vinyl alcohol) (PVA) with a Mw of 30
000–70
000 and a hydrolysis of 87–89% was purchased from Aldrich. The specification of the dialysis membrane purchased from Beijing Tianan Technology Co., Ltd, (Beijing, China). The fast membrane emulsification equipment (FMEM-500M) and Shirasu Porous Glass (SPG) membrane were purchased from National Engineering Research Center for Biotechnology. The SPG membranes were annulus cylinders with pore sizes of 7.0 μm. Other chemical reagents were of analytical grade and purchased from Beijing Chemical Works (Beijing, China).
Preparation of the Av/CAP PLA microcapsules (Av/CAP MCs)
The pesticide concentration was the ratio of pesticide to PLA. The Av/CAP MCs were prepared with a S/O/W double-emulsion method combined with PME. Firstly, 0.4 g of PLA was dissolved in 8.7 g of methylene chloride with various amounts of Av (weight ratios of Av/PLA: 0%, 30%, 40%, and 50% w/w). CAP (weight ratios of CAP/PLA: 0%, 2.5%, 5%, and 7.5% w/w) was dispersed in 2 g of deionized water by sonication to produce CAP suspensions. Mixture of Av solution and CAP suspension was sonicated to form the S/O primary emulsion. Then, PVA was dissolved in water and used as external water phase (W). The primary emulsion was immediately poured into external water phase under mechanical stirring to prepare the coarse S/O/W double emulsion and passed through SPG membrane under a certain nitrogen pressure for several times to obtain uniform double emulsion. The droplets were solidified under magnetic stirring overnight (6 hours). The microcapsules were collected via centrifugation. The obtained microcapsules were freeze-dried using a lyophilizer and the dried powder was stored at 4 °C prior to use.
Porous microcapsules were also constructed with a similar process method by additionally using BSA as an osmotic agent (0.4 g of BSA in internal water phase).
Statistical analysis of the data was analyzed by using Excel (Microsoft Inc.) and SPSS statistical analysis software (Version 21, IBM Corp.) for one-way analysis of variance (ANOVA) with Duncan's multiple-range test (p < 0.05). Experimental results were reported in the term of mean.
Characterization of the microcapsules
The morphology of the microcapsules was observed via scanning electron microscopy (SEM; JSM-6700F, JEOL, Japan) with 5 kV accelerating voltage. The instances were adhered to metal stubs using double-sided tape and vacuum-coated with a thin layer of platinum using a sputter coater (EM SCD 500, Leica, GER). The sizes of microcapsules were measured by laser scatter using a zetasizer (Zetasizer Nano ZS90, Malvern, UK). Fourier transform infrared spectra were recorded using a KBr method with a spectrometer (Tensor 27, Bruker, GER).
Drug loading content and entrapment efficiency
The drug loading was the actual contents of Av and CAP in the microcapsules. The entrapment rate was the ratio of actual-to-theoretical contents of the specific drug. The microcapsules were fully dissolved in methylene chloride to release all the loaded drugs, and then the solution was dried via reduced-pressure distillation to obtain dry precipitation. The Av and CAP were extracted using methanol from the precipitates. The methanol solution was further filtered to form a clear solution for UV-Vis spectrophotometer analysis at wavelengths of 245 and 260 nm to measure Av and CAP contents.
Bioassay studies
A preliminary bioassay of the Av/CAP microcapsules formulations against P. xylostella were evaluated using the Av microcapsules and CAP microcapsules as controls. The commercial formulation was diluted to 100, 20, 5, 1, 0.5, or 0.1 mg L−1 with 0.05% Triton. A series of aqueous microcapsule suspensions with the same pesticide concentration were prepared. After thoroughly washed and dried, the cabbage leaves were immersed in the different concentration solutions for 10 s and placed into culture dishes after drying in room temperature. The 3rd instars of P. xylostella were chosen as samples and took them into cabbage leaves gently by brushes. Each culture dishes involved ten P. xylostella and the treatment was carried for three replications. In the control treatments, the leaves were only immersed in 0.05% triton. All of the P. xylostella were reared in a climatic chamber to investigate the mortalities after 48 h at 25 °C. Using the death estimation standard represented death samples. Assessments were made on a dead/alive basis, and the SPSS software was used to calculate toxicity regression equations, LC50 and 95% confidence limits. The toxicity regression equation was given as below:
where Y is the mortality rate of treated group. E is the logarithm of pesticide dosage. Then the LC50 was derived from the best-fit line obtained. LC50 means the dosage when the mortality rate of the treated group was 50%.
Stability studies
According to the FAO/WHO Manuals, the microcapsules were stored at 55 °C for 14 days to accelerate thermal degradation, and at 4 °C as the default test conditions.45 The microcapsules were kept in glass tubes and stored at 4 °C and 55 °C for 14 days, and the variation of AV and CAP contents was constantly tested with predetermine time intervals. Light stability under UV irradiation of the microcapsules was tested as follows. A certain amount of microcapsules were dispersed with a methanol/water mixture (70
:
30, v/v) and divided equally into some culture dishes. In the center of the reactor, a 500 W (Emax = 365 nm) UV lamp was applied to the sample at a constant temperature of 25 °C during the experiments. The culture dish was removed from the reactor at 0, 12, 24, 36, 48, 60, 72 h and the Av and CAP contents in the culture dish were determined by the method described above.
Results and discussion
Preparation of the Av/CAP PLA microcapsules (Av/CAP MCs)
Uniform microcapsules could improve adhesion and permeability of the pesticide on target crops and to achieve effective utilization and high bioavailability of the pesticide. Similar to our previous report, we constructed the Av/CAP MCs by combining the double emulsion method (S/O/W) with PME and the porous holes were formed by the osmosis induction method as shown in Fig. 1.
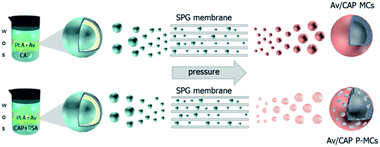 |
| Fig. 1 Schematic description for producing the Av/CAP MCs and Av/CAP P-MCs. | |
We directly dispersed CAP in the inner aqueous phase (S) and dissolved Av and PLA in the oil phase (O). So, CAP and Av were encapsulated inside the microcapsules and into the PLA shells via S/O/W method, of which innermost phase (S) was water suspension of solid CAP, and oil phase (O) was organic solution of Av and PLA. The osmotic agent, BSA, was added in the inner water phase to induce different osmotic pressure between internal and external water. The distinct osmotic pressure could press water molecules in the external phase into the inner water phase through the oil phase, resulting in formation of some water zones among the oil phase. After evaporation of methylene chloride and removal of water by freeze-drying, the pores in the shells were created to yield the porous microcapsules, Av/CAP P-MCs.
The SEM images in Fig. 2a showed that the prepared Av/CAP microcapsules with spherical shapes and smooth surface were uniform. The results indicated that uniform microcapsules could be successfully produced by PME combined with double-emulsion. The encapsulation of Av and CAP in the microcapsules were verified using FTIR measurement. Fig. 2b showed that the microcapsules had strong characteristic peaks of PLA (2968, 1758 and 1091 cm−1), CAP (3259, 1639, 1533, 761 and 632 cm−1) and Av (2968 and 989 cm−1). The results confirmed that both Av and CAP were loaded into the PLA microcapsule. Compared to commercial CAP, the peak of CAP wasn't obvious due to small amount CAP inside the CAP/Av MCs. As a result, we successfully produced uniform microcapsule carriers with co-loaded Av and CAP based on PME combined with double emulsion method.
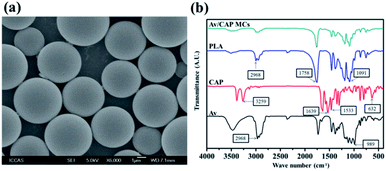 |
| Fig. 2 (a) SEM image of the Av/CAP MCs and (b) IR spectra of the Av, CAP, PLA and Av/CAP MCs. | |
Preparation of the porous Av/CAP PLA microcapsules (Av/CAP P-MCs)
The porous surfaces of the microcapsules would have a great effect on the release behavior of loaded Av and CAP pesticides. Pores on the microcapsule shells could enhance surface area and create special penetration channels, accelerating release of loaded Av and CAP. It is also noted that the dispersed CAP was loaded with large size inside the microcapsules. The CAP was very difficult to be released through the solid surface of the normal microcapsules. So, the porous structure is indispensable for development of the efficient microcapsule co-delivery system. Here, we adopted osmosis induction method to prepare the porous microcapsules.
The surface morphology of microcapsules had an obvious distinction with and without adding osmosis agent. Fig. 3a showed that the surface of Av/CAP MCs was smooth. As a comparison, the surface of the microcapsules possessed many micro-pores after adding osmosis agent (Fig. 3b). The results indicated that adding osmosis agent (BSA) in the internal water phases could induce to form pores on the surface of microcapsules. To study the inner structures of the microcapsules, SEM was performed to observe hollow chamber of the microcapsules cut by super thin razor blade. Fig. 3c and d showed that the cut microcapsules with/without porous structures both were bowl-like hemispheres, proving the resulted Av/CAP MCs and Av/CAP P-MCs had hollow core/shell structures. The DLS results of Fig. 3e and f indicated that the particle sizes of the Av/CAP MCs and Av/CAP P-MCs were ca. 3.5 and 3.4 μm with a narrow polydispersity of 0.04, which well agreed with the SEM results in Fig. 3c and d.
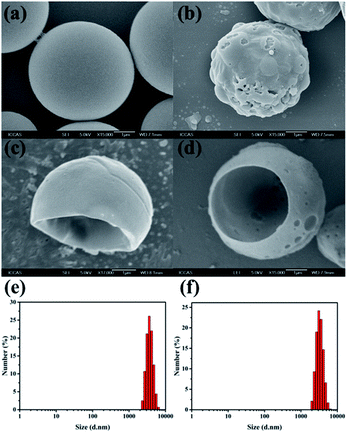 |
| Fig. 3 SEM images of the (a and c) Av/CAP MCs and (b and d) Av/CAP P-MCs before (a and b) and after (c and d) cut with a super thin razor blade. Size distribution of the (e) Av/CAP MCs and (f) Av/CAP P-MCs. | |
The loading content and entrapment efficiency
High-efficient pesticide formulations usually require high loading content and entrapment efficiency of pesticides in carriers for saving time, manpower and resources during the preparation process as well as avoiding extensive use in the spraying process. Here, to optimize Av/CAP microcapsule formulation, we tried various feed weight ratios of Av (30–50%) and CAP (2.5–7.5%) to PLA by fixing the PLA concentration in the oil phase to investigate the loading content and entrapment efficiency of Av and CAP.
The concentration of Av and CAP had an effect on the ability to trap Av and CAP within the PLA shell and inside the microcapsules. As shown in Table 1, the loading content and entrapment efficiency of Av and CAP both increased when higher feeding ratios of Av and CAP (increasing Av at constant CAP and increasing CAP at constant Av) were applied. For Av, 50 wt% Av of PLA had a higher loading content and entrapment efficiency. For CAP, the loading content only reach 0.52% even adopting a saturated solution of CAP as oil phase. In comparison, Table 1 indicated the CAP loading content could achieve 4.80% with a high entrapment efficiency to be ∼88%, demonstrating that encapsulation of CAP in the inner core of the microcapsule could greatly improve the CAP loading content. Considering the feed ratio at 50 wt% Av and 7.5 wt% CAP to PLA could yield the higher loading content with 87–88% entrapment efficiency, we selected this feed ratio to produce the microcapsules with or without porous structures for further study.
Table 1 Drug loading contents (LC) and entrapment efficiencies (EE) of various Av/CAP MCs with different feed ratios of Av and CAP to PLAa
Av of PLA (%) |
CAP of PLA (%) |
LC of Av (%) |
EE of Av (%) |
LC of CAP (%) |
EE of CAP (%) |
Values with the same subscript letter within each column are not significant different (p > 0.05). |
30 |
2.5 |
18.58a |
82.04b |
1.48c |
78.41a |
5 |
18.55a |
83.50a |
3.20f |
86.28b |
7.5 |
18.77a |
86.03c |
4.80i |
87.87bc |
40 |
2.5 |
24.06c |
85.73f |
1.36b |
77.51a |
5 |
24.05c |
87.19d |
3.06e |
88.60c |
7.5 |
23.93b |
88.25g |
4.45h |
87.57bc |
50 |
2.5 |
28.08d |
85.64d |
1.28a |
77.75a |
5 |
28.06d |
86.97e |
2.81d |
86.96bc |
7.5 |
28.07d |
88.41h |
4.17g |
87.49bc |
Comparison of the pesticide loading contents
In the preparation process, the small molecular Av dissolved in the oil phase and the solid CAP dispersed in the inner water phase were adopted to prepare the Av/CAP co-delivery system. The way to co-load Av and CAP into the same microcapsule may have a mutual effect on their loading content and entrapment efficiency. To evaluate their mutual effect, we further studied the loading content and entrapment efficiency of the Av/CAP microcapsules as well as those of the Av and CAP microcapsules (single pesticide-delivery system).
Fig. 4 showed that the loading contents of Av and CAP were not obviously different for the co-delivery microcapsules (Av/CAP MCs and Av/CAP P-MCs) and their corresponding single delivery microcapsules (Av MCs or CAP MCs). In detail, the Av contents were ca. 28.0%, 28.1%, and 28.1% for the Av MCs, Av/CAP MCs and Av/CAP P-MCs, and the CAP contents were ca. 4.1%, 4.2%, and 4.2% for the CAP MCs, Av/CAP MCs and Av/CAP P-MCs, respectively. Further, the entrapment efficiencies of Av and CAP were almost the same (87–88%) for single Av- or CAP-delivery system and Av/CAP co-delivery systems. The results powerfully proved that co-load of Av and CAP had a neglectable effect on their individual load content and entrapment efficiency. This no distinct should ascribe to independent load of Av and CAP into the shell and cavity of microcapsules, resulted from utilization of Av in the oil phase and CAP in the inner water phase. Fig. 4 further indicated that the Av/CAP MCs and Av/CAP P-MCs had similar loading contents of Av and CAP at their same feeding ratios, demonstrating the porous structure had no influence on the loading content of pesticides. As a result, the technique could successfully and efficiently construct the porous microcapsule formulations for co-delivery of Av and CAP as same as the single Av or CAP microcapsule formulations.
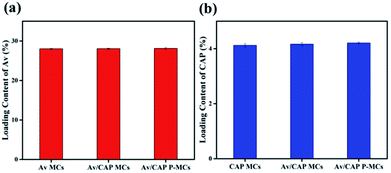 |
| Fig. 4 Drug loading contents (a) of Av in the Av MCs, Av/CAP MCs and Av/CAP P-MCs, and (b) of CAP in the CAP MCs, Av/CAP MCs and Av/CAP P-MCs. | |
Controlled released of Av and CAP
Instead of qualitative release behaviors for the traditional pesticide formulations, novel pesticide formulations with accurate and release capability were desired for customized practical applications. Tuning porosity of the microcapsules is a feasible way to precisely control release of loaded pesticide. Fig. 5 showed that the commercial Av and CAP released 91.2% and 91.4% within 12 h, which is much faster than the Av/CAP MCs and Av/CAP P-MCs. The result suggested the prepared microcapsules presented relatively slow release and maintained the sustained release for a longer period compared to the commercial Av and CAP. Furthermore, the Av/CAP P-MCs possessed a faster release rate of Av and CAP than the Av/CAP MCs. For example, the cumulative release of Av for the Av/CAP P-MCs reached 97.5% after 120 h, compared to 92.1% after 120 h and 98.4% at 168 h for the Av/CAP MCs (Fig. 5a). The similar result for CAP release was also observed in Fig. 5b. The cumulative release of CAP for the Av/CAP P-MCs after 54 h reached 98.7%, compared to 85.1% and 98.8% for the Av/CAP MCs after 54 and 84 h. The result illustrated that introduction of pores could increase diffusion and release of pesticides from the microcapsules.
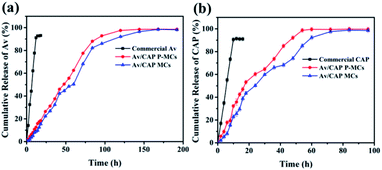 |
| Fig. 5 Release behaviours of (a) Av and (b) CAP of the commercial Av or CAP, Av/CAP MCs and Av/CAP P-MCs. | |
Bioassay studies
To evaluate feasibility of co-delivery system formulation using the porous microcapsule suspension as a novel pesticide formulation, bioactivities of various microcapsule suspensions against P. xylostella were studied using bioassay. Fig. 6 indicated that the Av/CAP P-MCs had the lowest LC50 value of 18.1 μg mL−1 compared to 46.3, 32.4, 28.5, 24.6 and 21.9 μg mL−1 for the CAP, Av, Av/CAP MCs, commercial CAP and Av. The result displayed the Av/CAP co-delivery system was more efficient than single delivery system because of synergistic effect of Av and CAP, which would improve drug efficiency and decrease drug dosage.
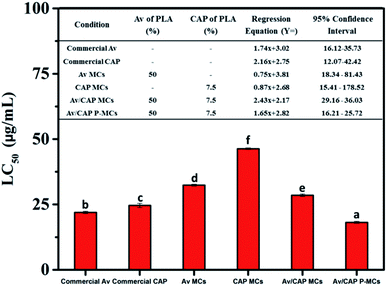 |
| Fig. 6 The LC50 values of the various microcapsules in bioassay study. Values with the same subscript letter within each column are not significant different (p > 0.05). | |
UV-shielding properties of the microcapsules
The commercial Av and CAP could be easily degraded under UV irradiation, which reduce pesticide utilization. This technique consolidating Av and CAP into the shell and inner cavity of the microcapsules could efficiently protect Av and CAP from direct exposure to the environment including UV irradiation, which could achieve high light stability. Fig. 7a showed around 32.2% and 65.3% of the commercial Av were degraded after 12 and 24 h, and completely decomposed after 36 h. In comparison, only 21.2% and 23.7% of Av were destroyed for the Av/CAP MCs and Av/CAP P-MCs even after 72 h. Similar result was also observed for CAP. Unlike quick decomposition of CAP under UV irradiation, e.g., 15.2% and 53.0% of the pristine CAP lost after 12 and 72 h, only 8.4% and 9.5% of CAP were degraded for the Av/CAP MCs and Av/CAP P-MCs after 72 h (Fig. 7b). Fig. 7 also indicated that Av and CAP in the Av/CAP P-MCs were degraded with a slight faster than those in the Av/CAP MCs due to their porous surfaces.
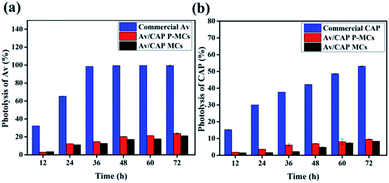 |
| Fig. 7 Photolysis percentage of (a) Av in the commercial Av, Av/CAP MCs and Av/CAP P-MCs formulations, and (b) CAP in the commercial CAP, Av/CAP MCs and Av/CAP P-MCs formulations under UV irradiation. | |
Thermal-stability of the microcapsules
The long-term storage stability is very important for pesticide formulations for practical usage in agriculture since delamination, caking and degradation of the active pesticide may occur during storage. To study storage stability, the various microcapsule samples were kept under 4 °C and 55 °C for 14 days to determine their thermal stability.
Fig. 8a and b showed that the microcapsules could keep their original shapes at 55 °C for 14 days. Fig. 8c–f further indicated that a negligible and small loss of Av and CAP was observed for the Av/CAP MCs and Av/CAP P-MCs after storage at and 4 °C and 55 °C for 14 days, demonstrating the solid microcapsules had good storage stability.
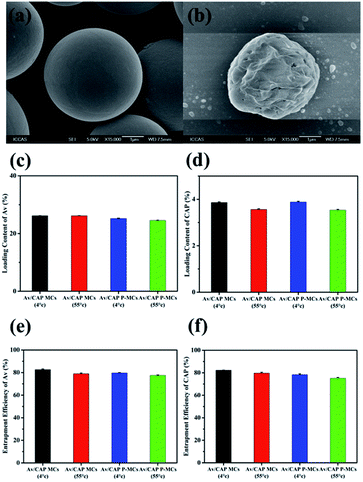 |
| Fig. 8 SEM images of the (a) Av/CAP MCs and (b) Av/CAP P-MCs at 55 °C after 14 days. (c) Av and (d) CAP contents of Av/CAP MCs and Av/CAP P-MCs after kept under 4 °C and 55 °C for 14 days. Entrapment efficiency of (e) Av and (f) CAP in the Av/CAP MCs and Av/CAP P-MCs after kept under 4 °C and 55 °C for 14 days. | |
Conclusions
In summary, we developed a water-based PLA microcapsule formulation for controlled co-delivery of Av and CAP via PME combined with a double-emulsion method. The microcapsule formulation presented good UV and thermal stability, and had a high drug loading content and entrapment efficiency, with an optimal feed ratio to be 50 wt% Av of PLA and 7.5 wt% CAP of PLA. Compared with the commercial Av and CAP, Av microcapsules and CAP microcapsules, the Av/CAP co-delivery system showed a superior bioactivity against pest. Such a strategy for co-encapsulation of two pesticides should be a greatly potential for development of environmental-friendly pesticide formations with improved pesticide utilization in agricultural field.
Conflicts of interest
There are no conflicts to declare.
Acknowledgements
We greatly thank funding support from Thai Government Scholarship and the Major National Scientific Research Program of China (No. 2014CB932200).
Notes and references
- M. W. Aktar, D. Sengupta and A. Chowdhury, Impact of Pesticides Use in Agriculture: Their Benefits and Hazards, Interdiscip. Toxicol., 2009, 2, 1–12 Search PubMed.
- C. J. Preftakes, J. J. Schleier 3rd, G. R. Kruger, D. K. Weaver and R. K. D. Peterson, Effect of Insecticide Formulation and Adjuvant Combination on Agricultural Spray Drift, PeerJ, 2019, 7, e7136 CrossRef PubMed.
- M. Nuruzzaman, Y. Liu, M. M. Rahman, R. Dharmarajan, L. Duan, A. F. M. J. Uddin and R. Naidu, Nanoencapsulation, Nano-guard for Pesticides: A New Window for Safe Application, J. Agric. Food Chem., 2016, 64, 1447–1483 CrossRef CAS PubMed.
- J. Feng, Q. Zhang, Q. Liu, Z. Zhu, D. J. McClements and S. M. Jafari, Application of Nanoemulsions in Formulation of Pesticides, in Nanoemulsions, ed. S. M. Jafari and D. J. McClements, Academic Press, 2018, ch. 12, pp. 379–413 Search PubMed.
- A. P. Pratap and D. N. Bhowmick, Pesticides as Microemulsion Formulations, J. Dispersion Sci. Technol., 2008, 29, 1325–1330 CrossRef CAS.
- I. Barber and K. Barrett, The Effect of a Pesticide Suspension Concentrate Formulation on Daphnia Magna Straus Reproduction, Sci. Total Environ., 1993, 134, 853–858 CrossRef.
- U. Bajwa and K. S. Sandhu, Effect of Handling and Processing on Pesticide Residues in Food - A Review, Int. J. Food Sci. Technol., 2014, 51, 201–220 CrossRef CAS PubMed.
- M. F. A. Jallow, D. G. Awadh, M. S. Albaho, V. Y. Devi and N. Ahmad, Monitoring of Pesticide Residues in Commonly Used Fruits and Vegetables in Kuwait, Int. J. Environ. Res. Public Health, 2017, 833, 1–12 Search PubMed.
- M. Hvězdová, P. Kosubová, M. Košíková, K. E. Scherr, Z. Šimek, L. Brodský, M. Šudoma, L. Škulcová, M. Sáňka, M. Svobodová, L. Krkošková, J. Vašíčková, N. Neuwirthová, L. Bielská and J. Hofman, Currently and Recently Used Pesticides in Central European Arable Soils, Sci. Total Environ., 2018, 613–614, 361–370 CrossRef PubMed.
- B. Huang, F. Chen, Y. Shen, K. Qian, Y. Wang, C. Sun, X. Zhao, B. Cui, F. Gao, Z. Zeng and H. Cui, Advances in Targeted Pesticides with Environmentally Responsive Controlled Release by Nanotechnology, Nanomaterials, 2018, 8, 102 CrossRef PubMed.
- M. Kah and T. Hofmann, Nanopesticide Research: Current Trends and Future Priorities, Environ. Int., 2014, 63, 224–235 CrossRef CAS.
- W. Guan, L. Tang, Y. Wang and H. Cui, Fabrication of an Effective Avermectin Nanoemulsion Using a Cleavable Succinic Ester Emulsifier, J. Agric. Food Chem., 2018, 66, 7568–7576 CrossRef CAS PubMed.
- M. Yu, Y. Junwei, J. Liang, Z. Zeng, B. Cui, X. Zhao, C. Sun, Y. Wang, G. Liu and H. Cui, Development of Functionalized Abamectin Poly(lactic acid) Nanoparticles with Regulatable Adhesion to Enhance Foliar Retention, RSC Adv., 2017, 7, 11271–11280 RSC.
- L. Cao, Y. Liu, C. Xu, Z. Zhou, P. Zhao, S. Niu and Q. Huang, Biodegradable Poly(3-hydroxybutyrate-co-4-hydroxybutyrate) Microcapsules for Controlled Release of Trifluralin with Improved Photostability and Herbicidal Activity, Mater. Sci. Eng., C, 2019, 102, 134–141 CrossRef CAS PubMed.
- D. Yang, B. Cui, C. Wang, X. Zhao, Z. Zeng, Y. Wang, C. Sun, G. Liu and H. Cui, Preparation and Characterization of Emamectin Benzoate Solid Nanodispersion, J. Nanomater., 2017, 2, 1–9 Search PubMed.
- Y. Wang, A. Wang, C. Wang, B. Cui, C. Sun, X. Zhao, Z. Zeng, Y. Shen, F. Gao, G. Liu and H. Cui, Synthesis and Characterization of Emamectin-benzoate Slow-release Microspheres with Different Surfactants, Sci. Rep., 2017, 7, 12761 CrossRef PubMed.
- K. S. Bentley, J. L. Fletcher and M. D. Woodward, Chlorantraniliprole: An Insecticide of the Anthranilic Diamide Class, in Hayes' Handbook of Pesticide Toxicology, ed. R. Krieger, Academic Press, New York, 3rd edn, 2010, ch. 102, pp. 2231–2242 Search PubMed.
- W. C. Campbell, Ivermectin and Abamectin, Springer, New York, 1989 Search PubMed.
- J. E. Casida and K. A. Durkin, Pesticide Chemical Research in Toxicology: Lessons from Nature, Chem. Res. Toxicol., 2017, 30, 94–104 Search PubMed.
- A. Adams, J. Gore, A. Catchot, F. Musser, D. Cook, N. Krishnan and T. Irby, Residual and Systemic Efficacy of Chlorantraniliprole and Flubendiamide Against Corn Earworm (Lepidoptera: Noctuidae) in Soybean, J. Econ. Entomol., 2016, 109, 2411–2417 CrossRef CAS PubMed.
- D. B. Sattelle, D. Cordova and T. R. Cheek, Insect Ryanodine Receptors: Molecular Targets for Novel Pest Control Chemicals, Invertebr. Neurosci., 2008, 8, 107 CrossRef CAS PubMed.
- A. K. Sharma, W. T. Zimmerman, S. K. Singles, K. Malekani, S. Swain, D. Ryan, G. McQuorcodale and L. Wardrope, Photolysis of Chlorantraniliprole and Cyantraniliprole in Water and Soil: Verification of Degradation Pathways via Kinetics Modeling, J. Agric. Food Chem., 2014, 62, 6577–6584 CrossRef CAS PubMed.
- D. Cordova, E. A. Benner, M. D. Sacher, J. J. Rauh, J. S. Sopa, G. P. Lahm, T. P. Selby, T. M. Stevenson, L. Flexner, S. Gutteridge, D. F. Rhoades, L. Wu, R. M. Smith and Y. Tao, Anthranilic diamides: A New Class of Insecticides with a Novel Mode of Action, Ryanodine Receptor Activation, Pestic. Biochem. Physiol., 2006, 84, 196–214 CrossRef CAS.
- S. W. Ludmerer, V. A. Warren, B. S. Williams, Y. Zheng, D. C. Hunt, M. B. Ayer, M. A. Wallace, A. G. Chaudhary, M. A. Egan, P. T. Meinke, D. C. Dean, M. L. Garcia, D. F. Cully and M. M. Smith, Ivermectin and Nodulisporic Acid Receptors in Drosophila melanogaster Contain Both γ-Aminobutyric Acid-Gated Rdl and Glutamate-Gated GluClα Chloride Channel Subunits, Biochemistry, 2002, 41, 6548–6560 CrossRef CAS PubMed.
- L. Strong and T. A. Brown, Avermectins in Insect Control and Biology: A Review, Bull. Entomol. Res., 2009, 77, 357–389 CrossRef.
- N. Cedergreen, Quantifying Synergy: A Systematic Review of Mixture Toxicity Studies within Environmental Toxicology, PLoS One, 2014, 9, e96580 CrossRef PubMed.
- L. Fengxiang, H. Guowen, F. Qiang, H. Yang, L. Ju, inventor and China National Rice Research Institute, Compound Pesticide of Chlorantraniliprole and Avermectins, CN Pat., 101755774B, 2013 Search PubMed.
- A. I. Billy, F. J. Lindsey, P. H. Eduardo, L. G. Philip, S. T. Paul, S. T. Martin, inventor and Du Pont, Synergistic Mixtures of Chlorantraniliprole and Abamectin, NZ Pat., 589 725, 2005 Search PubMed.
- D. Li, B. Liu, F. Yang, X. Wang, H. Shen and D. Wu, Preparation of Uniform Starch Microcapsules by Premix Membrane Emulsion for Controlled Release of Avermectin, Carbohydr. Polym., 2016, 136, 341–349 CrossRef CAS PubMed.
- B. Liu, Y. Wang, F. Yang, H. Cui and D. Wu, Development of a Chlorantraniliprole Microcapsule Formulation with a High Loading Content and Controlled-Release Property, J. Agric. Food Chem., 2018, 66, 6561–6568 CrossRef CAS PubMed.
- X. Zhou, L. Xu, J. Xu, J. Wu, T. B. Kirk, D. Ma and W. Xue, Construction of a High-Efficiency Drug and Gene Co-Delivery System for Cancer Therapy from a pH-Sensitive Supramolecular Inclusion between Oligoethylenimine-graft-β-cyclodextrin and Hyperbranched Polyglycerol Derivative, ACS Appl. Mater. Interfaces, 2018, 10, 35812–35829 CrossRef CAS PubMed.
- P. Chu, S. Tsai, H. Ko, C. Wu and Y. Lin, Co-Delivery of Natural Compounds with a Dual-Targeted Nanoparticle Delivery System for Improving Synergistic Therapy in an Orthotopic Tumor Model, ACS Appl. Mater. Interfaces, 2019, 11, 23880–23892 CrossRef CAS PubMed.
- N. Mozafari, F. Farjadian, S. Mohammadi Samani, S. Azadi and A. Azadi, Simvastatin-chitosan-citicoline Conjugates Nanoparticles as the Co-delivery System in Alzheimer Susceptible Patients, Int. J. Biol. Macromol., 2020, 156, 1396–1407 CrossRef PubMed.
- S. Mitragotri, Synergistic Effect of Enhancers for Transdermal Drug Delivery, Pharm. Res., 2000, 17, 1354–1359 CrossRef CAS PubMed.
- J. Pan, K. Rostamizadeh, N. Filipczak and V. P. Torchilin, Polymeric Co-Delivery Systems in Cancer Treatment: An Overview on Component Drugs' Dosage Ratio Effect, Molecules, 2019, 24, 1035 CrossRef CAS PubMed.
- K. Li, W. Zhan, Y. Chen, R. K. Jha and X. Chen, Docetaxel and Doxorubicin Codelivery by Nanocarriers for Synergistic Treatment of Prostate Cancer, Front. Pharmacol., 2019, 10, 1436 CrossRef PubMed.
- K. Shi, B. Xue, Y. Jia, L. Yuan, R. Han, F. Yang, J. Peng and Z. Qian, Sustained Co-delivery of Gemcitabine and Cis-platinum Via Biodegradable Thermo-sensitive Hydrogel for Synergistic Combination Therapy of Pancreatic Cancer, Nano Res., 2019, 12, 1389–1399 CrossRef CAS.
- X. Zhao, H. Cui, Y. Wang, C. Sun, B. Cui and Z. Zeng, Development Strategies and Prospects of Nano-based Smart Pesticide Formulation, J. Agric. Food Chem., 2018, 66, 6504–6512 CrossRef CAS PubMed.
- M. C. Camara, E. V. R. Campos, R. A. Monteiro, A. do Espirito Santo Pereira, P. L. de Freitas Proença and L. F. Fraceto, Development of Stimuli-responsive Nano-based Pesticides: Emerging Opportunities for Agriculture, J. Nanobiotechnol., 2019, 17, 100 CrossRef PubMed.
- E. Worrall, A. Hamid, K. Mody, N. Mitter and H. Pappu, Nanotechnology for Plant Disease Management, Agronomy, 2018, 8, 285 CrossRef CAS.
- X. Guo, Z. Zhao, D. Chen, M. Qiao, F. Wan, D. Cun, Y. Sun and M. Yang, Co-delivery of Resveratrol and Docetaxel Via Polymeric Micelles to Improve the Treatment of Drug-resistant Tumors, Asian J. Pharm. Sci., 2019, 14, 78–85 CrossRef PubMed.
- G. Yu, Q. Ning, Z. Mo and S. Tang, Intelligent Polymeric Micelles for Multidrug Co-delivery and Cancer Therapy, Artif. Cells, Nanomed., Biotechnol., 2019, 47, 1476–1487 CrossRef CAS PubMed.
- I. Khan, G. Joshi, K. T. Nakhate, Ajazuddin, R. Kumar and U. Gupta, Nano-Co-Delivery of Berberine and Anticancer Drug Using PLGA Nanoparticles: Exploration of Better Anticancer Activity and In Vivo Kinetics, Pharm. Res., 2019, 36, 149 CrossRef PubMed.
- B. Liu, Y. Wang, F. Yang, X. Wang, H. Shen, H. Cui and D. Wu, Construction of a Controlled-release Delivery System for Pesticides Using Biodegradable PLA-based Microcapsules, Colloids Surf., B, 2016, 144, 38–45 CrossRef CAS PubMed.
- WHO, Pesticide Specifications, Manual on the development and use of FAO and WHO specifications for Pesticides, second revision of the first edn, World Health Organization and Food and Agriculture Organization of the United Nations, Rome, 2010 Search PubMed.
|
This journal is © The Royal Society of Chemistry 2020 |