DOI:
10.1039/D0RA02912B
(Paper)
RSC Adv., 2020,
10, 16942-16948
Dehydration in water: frustrated Lewis pairs directly catalyzed allylization of electron-rich arenes and allyl alcohols†
Received
31st March 2020
, Accepted 23rd April 2020
First published on 30th April 2020
Abstract
A frustrated Lewis pair (FLP)-catalyzed allylation of allyl alcohols with electron-rich arenes has been developed. Interestingly, in this reaction, the electron-rich arenes and allyl alcohols are dehydrated in water. What's more, water was the sole byproduct of the reaction. In this protocol, various allyl alcohols can be converted into allyl cations and attacked by the electron-rich arenes to form aryl cation intermediates. Finally, the aryl cation intermediates are deprotonated to give the 1,3-diarylpropenes. In this protocol, indole allyl alcohols can undergo a bimolecular ring closure reaction, and structurally diverse tetrahydroindolo[3,2-b]carbazoles could be smoothly obtained. The reaction is not sensitive to oxygen and has been performed on a gram-scale.
Introduction
The Tsuji–Trost allylation reaction1 is an important way to construct C–C and C–X bonds. In recent years, quite a few breakthroughs have been made in transition metal catalyzed allylation. Muzart,2 Ma,3 Tunge,4 Bruneau,5 and Zhang6 et al. reviewed the latest developments in transition metal catalyzed allylation. At present, the majority of the existing reports are on the reaction of allyl alcohol derivatives as electrophiles.7 The allylation reaction with allyl alcohols as electrophiles has also been reported.8 It has attracted the attention of chemists for its good atom economy. At present, the directed distal allylation and alkylation of arenes have been developed.9 In addition, selective para-functionalization of arenes is also an important research direction.10 The metal-free catalyzed allylation reaction has attracted much attention due to its environmental friendliness, low toxicity and low catalyst loading. Several metal-free catalysts suitable for allylation reactions have been developed, including calix[n]arene sulfonic acids,11 arylboronic acids,12 water,13 1,3-bis(carboxymethyl)imidazolium,14 graphene oxide CO2H,15 and so on.
In recent years, tris(pentafluorophenyl)borane has received widespread attention as an unconventional, non-toxic, air-stable, water-tolerant, and thermal abiding Lewis acid.16 Piers reported in 1996 that tris(pentafluorophenyl)borane catalyzed hydrosilation of aromatic aldehydes, ketones and esters.17 Oestreich demonstrated the mechanism of Piers and confirmed the stereochemical reversal of silicon using chiral silanes.18 Stephan demonstrated that the new strategy for chemical storage of hydrogen in macroboranes may involve Lewis acid–Lewis base synergistic reactivity. This strategy was used to reduce the imines, nitriles and aziridines.19 Fontaine introduced the concept of a frustrated Lewis pairs into hydrocarbon activation, and investigated the carbon dioxide hydroboration of FLP catalysts.20 Repo conducted related research work on the frustrated Lewis pairs in the intramolecular.21 In addition, the frustrated Lewis pairs are also used to activate small molecules such as CO2,22 NO,23 N2O,24 SO2,25 alkenes,26 and alkynes.27 Although many advances have been made in the catalyzed reaction of frustrated Lewis pairs, there are currently no reports about allylation of electron-rich arenes by using the frustrated Lewis pairs as the catalyst.
At present, there are few reports on the reaction of macroboranes in water (Fig. 1). Tang's group reported α-aryl α-diazoesters O–H bond insertion catalyzed by tris(pentafluorophenyl)borane using water as solvent.28 Our group has reported the coupling reaction of naphthoquinones with indoles in water catalyzed by (C6F5)3B.29 The use of macroboranes for dehydration in water may be in line with the concept of green chemistry. Herein, we strived to expand the application range of the frustrated Lewis pairs. What's more, we reported the allylation of a catalyzed electron-rich arenes with allyl alcohols in water by the frustrated Lewis pairs.
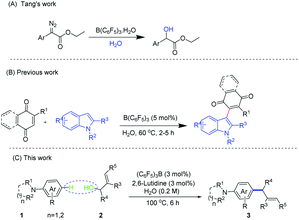 |
| Fig. 1 The reaction of macroboranes in water. | |
Results and discussion
We commenced our investigations by examining the allylation reaction between N,N-dimethylaniline (1a) and 1,3-diphenylallyl alcohol (2a) under various reaction conditions (Table 1). Initially the reaction was carried out under conditions in which (C6F5)3B was used as a catalyst and triphenylphosphine was used as a base. Gratifyingly, a 1,3-diarylpropene product 3a was observed and isolated in 71% yield (entry 1). Tris(1,3,5-trifluorophenyl)borane and tris(2,4,6-trifluorophenyl)borane had a small amount of product formation as a catalyst (entries 2–3). Unfortunately, when the reaction was carried out in the absence of a base, the yield of the reaction was lowered (entries 4 and 8). It was demonstrated that the base could promote the reaction. Gratifyingly, when the reaction was carried out in water, the product could be obtained in a comparable yield to that in acetonitrile (entry 5). We attempted to carry out the reaction without the addition of a catalyst and a base, but the yield of the product was greatly reduced (entry 6). In addition, when the catalyst was omitted, only a small amount of product was formed (entry 7). It was demonstrated that the coordination of the catalyst and the base was more favourable for the occurrence of allylation. Subsequently, we examined the effects of a series of bases on the reaction (entries 9–15). When the reaction was carried out under organic bases, the product was able to be obtained in moderate to excellent yields. But when we used inorganic base, the yield would be greatly reduced. This phenomenon indicated that the inorganic base could not form frustrated Lewis pairs with (C6F5)3B to catalyze the reaction. To our surprise, when the reaction was conducted in 2,6-lutidine as a base, we were pleased to find that the yield of 3a could be dramatically improved to 90% (entry 10). What's more, when the temperature was adjusted to 60 °C, the expected product could not be observed and a lot of the starting materials remained (entry 16). Interestingly, when the temperature was raised to 100 °C, the yield of the product could be increased to 92% (entry 17). Unfortunately, when we performed the reaction using only 2,6-lutidine as a catalyst, we only got trace product (entry 18). In addition, we also tried to decrease the amount of (C6F5)3B and 2,6-lutidine to 1 mol% but the yield was lower (entry 19). At last, the optimal conditions were confirmed: 1a (0.3 mmol), 2a (0.3 mmol), (C6F5)3B (3 mol%), 2,6-lutidine (3 mol%), H2O (1.5 ml), 6 h.
Table 1 Optimization of the reaction conditionsa,b
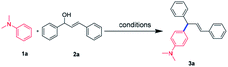
|
Entry |
Catalyst |
Base |
Solvent |
T (°C) |
Yield of 3a (%) |
Reaction conditions: 1a (0.3 mmol), 2a (0.3 mmol), catalyst (3 mol%), base (3 mol%), solvent (1.5 ml), 6 h; yield refers to isolated product. (C6F5)3B (1 mol%) and 2,6-lutidine (1 mol%) was used. |
1 |
(C6F5)3B |
Ph3P |
CH3CN |
80 |
71 |
2 |
(1,3,5-C6H2F3)3B |
Ph3P |
CH3CN |
80 |
Trace |
3 |
(2,4,6-C6H2F3)3B |
Ph3P |
CH3CN |
80 |
Trace |
4 |
(C6F5)3B |
— |
CH3CN |
80 |
58 |
5 |
(C6F5)3B |
Ph3P |
H2O |
80 |
74 |
6 |
— |
— |
H2O |
80 |
3 |
7 |
— |
Ph3P |
H2O |
80 |
5 |
8 |
(C6F5)3B |
— |
H2O |
80 |
59 |
9 |
(C6F5)3B |
Morpholine |
H2O |
80 |
71 |
10 |
(C6F5)3B |
2,6-Lutidine |
H2O |
80 |
90 |
11 |
(C6F5)3B |
DABCO |
H2O |
80 |
88 |
12 |
(C6F5)3B |
Me3P |
H2O |
80 |
78 |
13 |
(C6F5)3B |
P(OPh)3 |
H2O |
80 |
86 |
14 |
(C6F5)3B |
PHPh2 |
H2O |
80 |
86 |
15 |
(C6F5)3B |
Na2CO3 |
H2O |
100 |
38 |
16 |
(C6F5)3B |
2,6-Lutidine |
H2O |
60 |
0 |
17 |
(C6F5)3B |
2,6-Lutidine |
H2O |
100 |
92 |
18 |
— |
2,6-Lutidine |
H2O |
100 |
Trace |
19b |
(C6F5)3B |
2,6-Lutidine |
H2O |
100 |
79 |
With the optimized reaction conditions in hand, we next set out to explore the universality of this method (Scheme 1). Therefore, a series of electron-rich arenes with significant structural diversity, including anilines and phenyl ether, were subjected to this process. When the reaction was carried out with N,N,2-trimethylaniline, we obtained 1,3-diarylpropene in moderate yield (3b). When we carried out the reaction with a meta-substitution or a 2,6-disubstituted N,N-dimethylaniline as a substrate, we were also able to obtain an allylated product, albeit at a low yields (3c–3d). It was possible that the steric hindrance effect of the ortho and meta positions prevented the attack of allyl cations and led to a decrease in yield. Further, this protocol could be suitably applied after the methyl group on N was replaced by n-butyl group, and the allylation product was obtained in a moderate yield (3e). Interestingly, the cyclic aniline compounds were capable of obtaining the allylation products in good to excellent yields (3f–3g). What's more, when a largely hindered benzyl group was attached to the N of the aniline, the product was obtained in excellent yield (3h). The exciting thing was that this protocol showed good compatibility with carbon–carbon double bonds and carbon–carbon triple bonds (3i–3j). Moreover, naphthylamine compound could also be used in this process and gave allylation product in good yield (3k). Furthermore, the 1,2,3-trimethoxybenzene was used, and the 1,3-diarylpropene product could also be formed, albeit at a low yield (3l, 33%). It might be due to the common steric hindrance effect of ortho and meta positions, which greatly reduced the yield. This is consistent with the previous results (3c–3d).
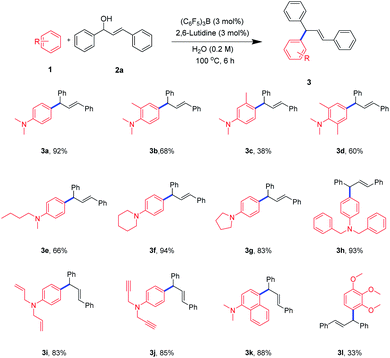 |
| Scheme 1 Substrate scope of electron-rich arenesa,b. aReaction conditions: 1 (0.3 mmol), 2a (0.3 mmol), (C6F5)3B (3 mol%), 2,6-lutidine (3 mol%), H2O (0.2 M), 100 °C, 6 h. bIsolated yields. | |
We next explored the scope of allyl alcohols (Scheme 2). Firstly, we focused our attention on the changes in the allyl alcohol A ring substituent. Unfortunately, the allyl alcohol double bond was shifted and we got a mixture of double bond migrations. TLC showed as only one point that cannot be separated. The ratio of the double bond migration product to non-migrated product was about 1
:
1 by NMR. The possible reason was that the aromatic rings at the ends of allyl cations had almost the same ability to stabilize the positive charge. When the para position of the phenyl group was bonded to an electron-donating substituted group, the product was able to be obtained in excellent yield (3m). In addition, the para position of the phenyl group was attached to an electron withdrawing group such as fluorine, bromine or cyano, and we could also obtain the products in moderate to good yields (3n–3p). In particular, the cyano group of the strong electron withdrawing group caused the uneven distribution of the allyl cation charge, resulting in a product ratio of 5
:
2. In addition, the meta-substituted benzene ring could also give the products in a moderate yields (3q–3r). Excitingly, this reaction also showed good compatibility with heterocycles (3s–3t). When the ring A was the naphthalene, we could also carry out the reaction in good yield (3u). When the reaction was carried out with (2E,4E)-1,5-diphenylpenta-2,4-dien-1-ol, the product was able to be obtained in good yield (3v). Next, we would explore the effect of substituent on the B ring on the reaction. When the B ring was attached to an electron donating substituent, we were able to carry out the reaction in moderate to good yields (3w–3x). In addition, when the B ring was connected to the electron-withdrawing group, the product was also able to be obtained product in moderate yield (3y). Unfortunately, alkyl allyl alcohol and polysubstituted allyl alcohol did not perform the reaction smoothly in water, but the product could be obtained in acetonitrile in moderate yields (3z–3aa).
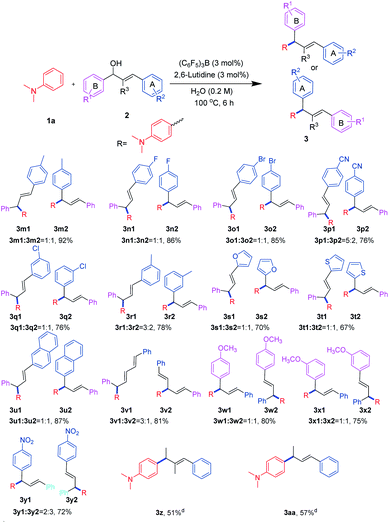 |
| Scheme 2 Substrate scope of allyl alcoholsa,b,c,d. aReaction conditions: 1 (0.3 mmol), 2a (0.3 mmol), (C6F5)3B (3 mol%), 2,6-lutidine (3 mol%), H2O (0.2 M), 100 °C, 6 h. bIsolated yields. cMixture ratio determined by NMR. dAcetonitrile as solvent (seal). | |
The 3 position of indoles is electron-rich, and allylization of indoles have been reported more.30 Herein, we focused our attention to the scope of indoles (Scheme 3). Gratifyingly, various indoles were well applicable in this protocol and were regioselectively allylated to the 3 position of the indoles. In addition, the single-crystal structure of 3af, 3al further confirmed the allylation reaction.31 As an example, when N1 position of indoles was not attached to a substituent or attached to an electron-donating substituted group such as methyl, allyl, we could proceed smoothly in this process to deliver corresponding allylated products in good to excellent yields (3ab–3ad). When N1 position of indoles was substituted by electron-withdrawing groups such as acetyl and pyrimidine we were also able to obtain the products although the yields were slightly reduced (3ae–3af). It was possible that the electron-withdrawing group lowered the density of the electrons at the 3-position electrons, thereby reducing the attack activity of the allyl cations. Interestingly, when the C2 position of the indoles was substituted by a methyl group or a phenyl group, we could also obtain the products in a good to excellent yields, demonstrating that the steric hindrance at the C2 position had little effect on the reaction (3ag–3ah). In addition, when the C5- or C6-position of the indoles were separately substituted for the substituent, we could obtain the 3-allylated indoles at a good yields (3ai–3aj). Excitingly, when the C5 and C6 positions were substituted, we were able to obtain allylated indole in a superior yield (3ak).
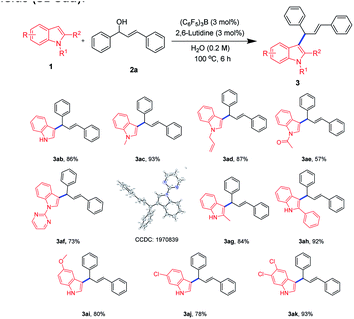 |
| Scheme 3 Substrate scope of indolesa,b. aReaction conditions: 1 (0.3 mmol), 2a (0.3 mmol), (C6F5)3B (3 mol%), 2,6-lutidine (3 mol%), H2O (0.2 M), 100 °C, 6 h. bIsolated yields. | |
Surprisingly, (E)-3-(1-methyl-1H-indol-2-yl)-1-phenylprop-2-en-1-ol (2q) and (E)-1-(4-bromophenyl)-3-(1-methyl-1H-indol-2-yl)prop-2-en-1-ol (2r) were capable of bimolecular ring-closure reaction to obtain tetrahydroindolo[3,2-b]carbazoles (3al–3am). In order to reveal the potential application of this protocol, an amplification scale reaction was implemented. A 1.2 g scale reaction was carried out for N,N-dimethylaniline 1a and (E)-3-(1-hydroxy-3-phenylallyl)benzene-1-ylium 2a, and the product 3a was isolated in 89% yield (Scheme 4). The result showed that the product yield was not affected after the reaction was scaled up, further indicating that the reaction has great potential for application.
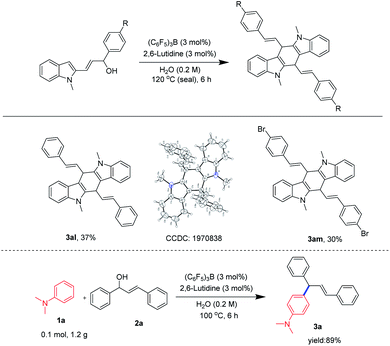 |
| Scheme 4 Bimolecular ring closure and scale-up reaction. | |
Based on others previously reported in literature,19,28,32,33 a plausible mechanism was proposed in Scheme 5. Initially, tris(pentafluorophenyl)borane and 2,6-lutidine formed a frustrated Lewis pairs A. A interacted with allyl alcohol to form [(C6F5)3B(OH)]− and an allyl cation stabilized by base. The electron-rich aromatic ring attacked the allyl cation to form aromatic cation intermediate B. B deprotonated and reacted with [(C6F5)3B(OH)]− to produce water and target products, while the catalyst and base were circulated. In addition, when there was no base, (C6F5)3B could also convert allyl alcohol to allyl cation, thereby completing the reaction process. However, in the absence of a base, the yield of the reaction product was only moderate (Table 1 entries 5 and 8). It might be that the base helped stabilize the allyl cation and improved the yield.
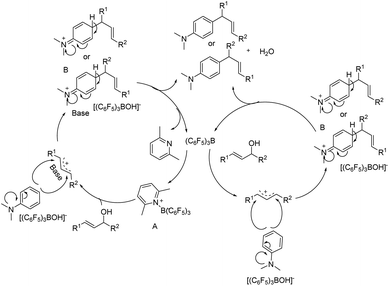 |
| Scheme 5 Proposed mechanism. | |
Conclusions
In summary, a FLP-catalyzed allylization of allyl alcohols with electron-rich arenes in water has been reported for the preparation of 1,3-diarylpropenes. In this protocol, the electron-rich aromatics and allyl alcohols are dehydrated in water. What's more, the reaction has very good atom economy and water is the sole byproduct. This protocol is not only featured with mild conditions and broad scope, but also reveals FLP-catalyzed allylization of electron-rich aromatic rings in water, which would offer a new insight for synthesis of 1,3-diarylpropenes and dihydrocarbazoloindoles.
Conflicts of interest
There are no conflicts to declare.
Acknowledgements
We are grateful for the financial support from CAS “Light of West China” Program, Technological Innovation Program of Chengdu, Sichuan Province, China (No. 2018-YF05-00244-SN) and the Applied Basic Research Programs of Sichuan Province, China (No. 20YYJC0492). We would like to thank Dr Daibing Luo from the Analytical & Testing Center of Sichuan University for X-ray diffraction work and single crystal analysis.
References
-
(a) J. Tsuji, Carbon-Carbon Bond Formation via Palladium Complexes, Acc. Chem. Res., 1969, 2, 144–152 CrossRef CAS
;
(b) B. M. Trost and T. J. Fullerton, New Synthetic Reactions. Allylic Alkylation, J. Am. Chem. Soc., 1973, 95, 292–294 CrossRef CAS
;
(c) B. M. Trost, Asymmetric Transition Metal-Catalyzed Allylic Alkylations, Chem. Rev., 1996, 96, 395–422 CrossRef CAS PubMed
;
(d) B. M. Trost and M. L. Crawley, Asymmetric Transition-Metal-Catalyzed Allylic Alkylations: Applications in Total Synthesis, Chem. Rev., 2003, 103, 2921–2943 CrossRef CAS PubMed
. - J. Muzart, Palladium-catalysed reactions of alcohols. Part B: formation of C-C and C-N bonds from unsaturated alcohols, Tetrahedron, 2005, 61, 4179–4212 CrossRef CAS
. - S.-M. Ma and Z. Lu, Metal-Catalyzed Enantioselective Allylation in Asymmetric Synthesis, Angew. Chem., Int. Ed., 2008, 47, 258–297 CrossRef PubMed
. - J. D. Weaver, A. Recio III, A. J. Grenning and J. A. Tunge, Transition Metal-Catalyzed Decarboxylative Allylation and Benzylation Reactions, Chem. Rev., 2011, 111, 1846–1913 CrossRef CAS PubMed
. - B. Sundararaju, M. Achard and C. Bruneau, Transition metal catalyzed nucleophilic allylic substitution: activation of allylic alcohols via p-allylic species, Chem. Soc. Rev., 2012, 41, 4467–4483 RSC
. - N. A. Butt and W.-B. Zhang, Transition metal-catalyzed allylic substitution reactions with unactivated allylic substrates, Chem. Soc. Rev., 2015, 44, 7929–7967 RSC
. -
(a) M. Choi, J. Park, S. Sharma, H. Jo, S. Han, M. Jeon, N. K. Mishra, S. H. Han, J. S. Lee and I. S. Kim, Trifluoromethylallylation of Heterocyclic C-H Bonds with Allylic Carbonates under Rhodium Catalysis, J. Org. Chem., 2016, 81, 4771–4778 CrossRef CAS PubMed
;
(b) X. Wei, D.-L. Liu, Q.-J. An and W.-B. Zhang, Hydrogen-Bond Directed Regioselective Pd-Catalyzed Asymmetric Allylic Alkylation: The Construction of Chiral α-Amino Acids with Vicinal Tertiary and Quaternary Stereocenters, Org. Lett., 2015, 17, 5768–5771 CrossRef CAS PubMed
;
(c) H. Yorimitsu and K. Oshima, Recent Progress in Asymmetric Allylic Substitutions Catalyzed by Chiral Copper Complexes, Angew. Chem., Int. Ed., 2005, 44, 4435–4439 CrossRef CAS PubMed
;
(d) K. Ohmatsu, M. Ito, T. Kunieda and T. Ooi, Ion-paired chiral ligands for asymmetric palladium catalysis, Nat. Chem., 2012, 4, 473–477 CrossRef CAS PubMed
;
(e) M. Braun and T. Meier, Tsuji-Trost Allylic Alkylation with Ketone Enolates, Angew. Chem., Int. Ed., 2006, 45, 6952–6955 CrossRef CAS PubMed
;
(f) L. Du, P. Cao, J.-W. Xing, Y.-Z. Lou, L.-Y. Jiang, L.-C. Li and J. Liao, Hydrogen-Bond-Promoted Palladium Catalysis: Allylic Alkylation of Indoles with Unsymmetrical 1,3-Disubstituted Allyl Acetates Using Chiral Bis(sulfoxide)Phosphine Ligands, Angew. Chem., Int. Ed., 2013, 52, 4207–4211 CrossRef CAS PubMed
;
(g) A. Misale, S. Niyomchon, M. Luparia and N. Maulide, Asymmetric Palladium-Catalyzed Allylic Alkylation Using Dialkylzinc Reagents: A Remarkable Ligand Effect, Angew. Chem., Int. Ed., 2014, 53, 7068–7073 CrossRef CAS PubMed
;
(h) X.-Y. Jiang, W.-Y. Chen and J.-F. Hartwig, Iridium-Catalyzed Diastereoselective and Enantioselective Allylic Substitutions with Acyclic α-Alkoxy Ketones, Angew. Chem., Int. Ed., 2016, 55, 5819–5823 CrossRef CAS PubMed
;
(i) R. Matsubara and T. F. Jamison, Nickel-Catalyzed Allylic Substitution of Simple Alkenes, J. Am. Chem. Soc., 2010, 132, 6880–6881 CrossRef CAS PubMed
;
(j) K.-Y. Ye, H. He, W.-B. Liu, L.-X. Dai, G. Helmchen and S.-L. You, Iridium-Catalyzed Allylic Vinylation and Asymmetric Allylic Amination Reactions with o-Aminostyrenes, J. Am. Chem. Soc., 2011, 133, 19006–19014 CrossRef CAS PubMed
;
(k) W.-B. Liu, N. Okamoto, E. J. Alexy, A. Y. Hong, K. Tran and B. M. Toltz, Enantioselective γ-Alkylation of α,β-Unsaturated Malonates and Ketoesters by a Sequential Ir-Catalyzed Asymmetric Allylic Alkylation/Cope
Rearrangement, J. Am. Chem. Soc., 2016, 138, 5234–5237 CrossRef CAS PubMed
. -
(a) A. J. Grenning and J. A. Tunge, Deacylative Allylation of Nitroalkanes: Unsymmetric Bisallylation by a Three-Component Coupling, Angew. Chem., Int. Ed., 2011, 50, 1688–1691 CrossRef CAS PubMed
;
(b) Y. Zhang, S.-C. Yin and J.-M. Lu, N-Heterocyclic Carbene-Palladium(II)-1-Methylimidazole Complex Catalyzed Allyl-Aryl Coupling of Allylic Alcohols with Arylboronic Acids in Neat Water, Tetrahedron, 2015, 71, 544–549 CrossRef CAS
;
(c) Y. Gumrukcu, B. D. Bruin and J. N. H. Reek, Hydrogen-Bond-Assisted Activation of Allylic Alcohols for Palladium-Catalyzed Coupling Reactions, ChemSusChem, 2014, 7, 890–896 CrossRef CAS PubMed
;
(d) S. Krautwald, D. Sarlah, M. A. Schafroth and E. M. Carreira, Enantio- and Diastereodivergent Dual Catalysis: α-Allylation of Branched Aldehydes, Science, 2013, 340, 1065–1068 CrossRef CAS PubMed
;
(e) G. S. Kumar and M. Kapur, Ruthenium-Catalyzed, Site-Selective C-H Allylation of Indoles with Allyl Alcohols as Coupling Partners, Org. Lett., 2016, 18, 1112–1115 CrossRef CAS PubMed
;
(f) Y. Bunno, N. Murakami, Y. Suzuki, M. Kanai, T. Yoshino and S. Matsunaga, Cp*Co III-Catalyzed Dehydrative C-H Allylation of 6-Arylpurines and Aromatic Amides Using Allyl Alcohols in Fluorinated Alcohols, Org. Lett., 2016, 18, 2216–2219 CrossRef CAS PubMed
;
(g) K. Miyata, H. Kutsuna, S. Kawakami and M. Kitamura, A Chiral Bidentate sp2-N Ligand, Naph-diPIM: Application to CpRu-Catalyzed Asymmetric Dehydrative C-, N-, and O-Allylation, Angew. Chem., Int. Ed., 2011, 50, 4649–4653 CrossRef CAS PubMed
;
(h) G. Jiang and B. List, Direct Asymmetric α-Allylation of Aldehydes with Simple Allylic Alcohols Enabled by the Concerted Action of Three Different Catalysts, Angew. Chem., Int. Ed., 2011, 50, 9471–9474 CrossRef CAS PubMed
;
(i) X.-H. Huo, G.-Q. Yang, D.-L. Liu, Y.-G. Liu, I. D. Gridnev and W.-B. Zhang, Palladium-Catalyzed Allylic Alkylation of Simple Ketones with Allylic Alcohols and Its Mechanistic Study, Angew. Chem., Int. Ed., 2014, 53, 6776–6780 CrossRef CAS PubMed
;
(j) H. Zhou, L. Zhang, C.-M. Xu and S.-Z. Luo, Chiral Primary Amine/Palladium Dual Catalysis for Asymmetric Allylic Alkylation of β-Ketocarbonyl Compounds with Allylic Alcohols, Angew. Chem., Int. Ed., 2015, 54, 12645–12648 CrossRef CAS PubMed
;
(k) M.-M. Mastandrea, N. Mellonie, P. Giacinto, A. Collado, S. P. Nolan, G. P. Miscione, A. Bottoni and M. Bandini, Gold(I)-Assisted α-Allylation of Enals and Enones with Alcohols, Angew. Chem., Int. Ed., 2015, 54, 14885–14889 CrossRef CAS PubMed
;
(l) Y. Kita, R. D. Kavthe, H. Oda and K. Mashima, Asymmetric Allylic Alkylation of β-Ketoesters with Allylic Alcohols by a Nickel/Diphosphine Catalyst, Angew. Chem., Int. Ed., 2016, 55, 1098–1101 CrossRef CAS PubMed
;
(m) H. Tsukamoto, M. Sato and Y. Kondo, Palladium(0)-catalyzed direct cross-coupling reaction of allyl alcohols with aryl- and vinyl-boronic acids, Chem. Commun., 2004, 1200–1201 RSC
;
(n) H. Tsukamoto, T. Uchiyama, T. Suzuki and Y. Kondo, Palladium(0)-catalyzed direct cross-coupling reaction of allyl alcohols with aryl- and vinyl-boronic acids, Org. Biomol. Chem., 2008, 6, 3005–3013 RSC
;
(o) Y. Gumrukcu, B. D. Bruin and J. N. H. Reek, Dehydrative Cross-Coupling Reactions of Allylic Alcohols with Olefins, Chem.–Eur. J., 2014, 20, 10905–10909 CrossRef CAS PubMed
;
(p) T. Mita, Y. Higuchi and Y. Sato, Highly Regioselective Palladium-Catalyzed Carboxylation of Allylic Alcohols with CO2, Chem.–Eur. J., 2015, 21, 16391–16394 CrossRef CAS PubMed
;
(q) R. Blieck, M. S. Azizi, A. Mifleur, M. Roger, C. Persyn, M. Sauthier and H. Bonin, Nickel-Catalysed Bis-Allylation of Activated Nucleophiles with Allyl Alcohol, Eur. J. Org. Chem., 2016, 1194–1198 CrossRef CAS
;
(r) J. Y. Hamilton, D. Sarlah and E. M. Carreira, Iridium-Catalyzed Enantioselective Allylic Vinylation, J. Am. Chem. Soc., 2013, 135, 994–997 CrossRef CAS PubMed
;
(s) P. Trillo, A. Baeza and C. Njera, Direct Nucleophilic Substitution of Free Allylic Alcohols in Water Catalyzed by FeCl3·6H2O: Which is the Real Catalyst, ChemCatChem, 2013, 5, 1538–1542 CrossRef CAS
. -
(a) T. K. Achar, X.-L. Zhang, R. Mondal, M. S. Shanavas, S. Maiti, S. Maity, N. Pal, R. S. Paton and D. Maiti, Palladium-Catalyzed Directed meta-Selective C-H Allylation of Arenes: Unactivated Internal Olefins as Allyl Surrogates, Angew. Chem., Int. Ed., 2019, 58, 10353–10360 CrossRef CAS PubMed
;
(b) R. Kankanala, J. P. Biswas, S. Jana, T. K. Achar, S. Porey and D. Maiti, Co-ordination assisted distal C-H alkylation of fused heterocycles, Angew. Chem., Int. Ed., 2019, 58, 13808–13812 CrossRef PubMed
;
(c) A. Dey, S. K. Sinha, T. K. Achar and D. Maiti, Accessing Remote meta- and para-C(sp2)-H Bonds with Covalently Attached Directing Groups, Angew. Chem., Int. Ed., 2019, 58, 10820–10843 CrossRef CAS PubMed
;
(d) A. Baccalini, S. Vergura, P. Dolui, S. Maiti, S. Dutta, S. Maity, F. F. Khan, G. K. Lahiri, G. Zanoni and D. Maiti, Cobalt-Catalyzed C(sp2)-H Allylation of Biphenyl Amines with Unbiased Terminal Olefins, Org. Lett., 2019, 21(21), 8842–8846 CrossRef CAS PubMed
. -
(a) A. Maji, A. Dahiya, G. Lu, T. Bhattacharya, M. Brochetta, G. Zanoni, P. Liu and D. Maiti, H-bonded reusable template assisted paraselective ketonisation using soft electrophilic vinyl ethers, Nat. Commun., 2018, 9, 3582–3591 CrossRef PubMed
;
(b) U. Dutta, S. Maiti, S. Pimparkar, S. Maiti, L. R. Gahan, E. H. Krenske, D. W. Lupton and D. Maiti, Rhodium catalyzed template-assisted distal para-C-H olefination, Chem. Sci., 2019, 10, 7426–7432 RSC
;
(c) A. Maji, S. Guin, S. Feng, A. Dahiya, V. K. Singh, P. Liu and D. Maiti, Experimental and Computational Exploration of Para-Selective Silylation Involving H-Bonded Template, Angew. Chem., Int. Ed., 2017, 56, 14903–14907 CrossRef CAS PubMed
;
(d) T. Patra, S. Bag, R. Kancherla, A. Mondal, A. Dey, S. Pimparkar, S. Agasti, A. Modak and D. Maiti, Palladium-Catalyzed Directed para C-H Functionalization of Phenols, Angew. Chem., Int. Ed., 2016, 55, 7751–7755 CrossRef CAS PubMed
;
(e) S. Bag, T. Patra, A. Modak, A. Deb, S. Maity, U. Dutta, A. Dey, R. Kancherla, A. Maji, A. Hazra, M. Bera and D. Maiti, Remote para-C-H Functionalization of Arenes by a D-Shaped Biphenyl Template-Based Assembly, J. Am. Chem. Soc., 2015, 137, 11888–11891 CrossRef CAS PubMed
. - Y.-L. Liu, L. Liu, Y.-L. Wang, Y.-C. Han, D. Wang and Y.-J. Chen, Calix[n]arene sulfonic acids bearing pendant aliphatic chains as recyclable surfactant-type Brønsted acid catalysts for allylic alkylation with allyl alcohols in water, Green Chem., 2008, 10, 635–640 RSC
. -
(a) J. A. McCubbin, H. Hosseini and O. V. Krokhin, Boronic Acid Catalyzed Friedel-Crafts Reactions of Allylic Alcohols with Electron-Rich Arenes and Heteroarenes, J. Org. Chem., 2010, 75, 959–962 CrossRef CAS PubMed
;
(b) C. L. Ricardo, X. Mo, J. A. McCubbin and D. G. Hall, A Surprising Substituent Effect Provides a Superior Boronic Acid Catalyst for Mild and Metal-Free Direct Friedel–Crafts Alkylations and Prenylations of Neutral Arenes, Chem.–Eur. J., 2015, 21, 4218–4223 CrossRef CAS PubMed
. - T. Hirashita, S. Kuwahara, S. Okochi, M. Tsuji and S. Araki, Direct benzylation and allylic alkylation in high-temperature water without added catalysts, Tetrahedron Lett., 2010, 51, 1847–1851 CrossRef CAS
. - M. A. Soriano, L. H. Martínez and I. M. Pastor, 1,3-Bis(carboxymethyl)imidazolium Chloride as a Metal-Free and Recyclable Catalyst for the Synthesis of N-Allylanilines by Allylic Substitution of Alcohols, ACS Sustainable Chem. Eng., 2018, 6, 14063–14070 CrossRef
. - M. Gómez-Martínez, A. Baeza and D. A. Alonso, Pinacol Rearrangement and Direct Nucleophilic Substitution of Allylic Alcohols Promoted by Graphene Oxide and Graphene Oxide CO2H, ChemCatChem, 2017, 9, 1032–1039 CrossRef
. -
(a) N. Gandhamsetty, J. Park, J. Jeong, S.-W. Park, S. Park and S. Chang, Chemoselective Silylative Reduction of Conjugated Nitriles under Metal-Free Catalytic Conditions: β-Silyl Amines and Enamines, Angew. Chem., Int. Ed., 2015, 54, 6832–6836 CrossRef CAS PubMed
;
(b) E. Y.-X. Chen and T. J. Marks, Cocatalysts for Metal-Catalyzed Olefin Polymerization: Activators, Activation Processes, and Structure-Activity Relationships, Chem. Rev., 2000, 100, 1391–1434 CrossRef CAS PubMed
;
(c) K. Ishihara and H. Yamamoto, Arylboron Compounds as Acid Catalysts in Organic Synthetic Transformations, Eur. J. Org. Chem., 1999, 527–538 CrossRef CAS
;
(d) W. E. Piers and T. Chivers, Pentafluorophenylboranes: from obscurity to applications, Chem. Soc. Rev., 1997, 26, 345–354 RSC
;
(e) T. Robert and M. Oestreich, Si-H Bond Activation: Bridging Lewis Acid Catalysis with Brookhart's Iridium(III) Pincer Complex and B(C6F5)3, Angew. Chem., Int. Ed., 2013, 52, 5216–5218 CrossRef
;
(f) W. Meng, X.-Q. Feng and H.-F. Du, Frustrated Lewis Pairs Catalyzed Asymmetric Metal-Free Hydrogenations and Hydrosilylations, Acc. Chem. Res., 2018, 51, 191–201 CrossRef CAS PubMed
;
(g) S.-L. Li, G. Li, W. Meng and H.-F. Du, A Frustrated Lewis Pair Catalyzed Asymmetric Transfer Hydrogenation of Imines Using Ammonia Borane, J. Am. Chem. Soc., 2016, 138, 12956–12962 CrossRef CAS PubMed
. - D. J. Parks and W. E. Piers, Tris(pentafluorophenyl)boron-catalyzed Hydrosilation of Aromatic Aldehydes, Ketones, and Esters, J. Am. Chem. Soc., 1996, 118, 9440–9441 CrossRef CAS
. - S. Rendler and M. Oestreich, Conclusive Evidence for an SN2-Si Mechanism in the B(C6F5)3-catalyzed Hydrosilylation of Carbonyl Compounds: Implications for The Related Hydrogenation, Angew. Chem., Int. Ed., 2008, 47, 5997–6000 CrossRef CAS PubMed
. -
(a) G. C. Welch, R. R. S. Juan, J. D. Masuda and D. W. Stephan, Reversible, Metal-Free Hydrogen Activation, Science, 2006, 314, 1124–1126 CrossRef CAS PubMed
;
(b) P. Spies, G. Erker, G. Kehr, K. Bergander, R. Fröhlich, S. Grimme and D. W. Stephan, Rapid Intramolecular Heterolytic Dihydrogen Activation by A Four-Membered Heterocyclic Phosphane-Borane Adduct, Chem. Commun., 2007, 5072–5074 RSC
;
(c) G. C. Welch and D. W. Stephan, Facile Heterolytic Cleavage of Dihydrogen by Phosphines and Boranes, J. Am. Chem. Soc., 2007, 129, 1880–1881 CrossRef CAS PubMed
;
(d) J. S. J. McCahill, G. C. Welch and D. W. Stephan, Reactivity of ‘Frustrated Lewis Pairs’: Three-Component Reactions of Phosphines, A Borane, and Olefins, Angew. Chem., Int. Ed., 2007, 46, 4968–4971 CrossRef CAS PubMed
;
(e) P. A. Chase, G. C. Welch, T. Jurca and D. W. Stephan, Metal-Free Catalytic Hydrogenation, Angew. Chem., Int. Ed., 2007, 46, 8050–8053 CrossRef CAS PubMed
;
(f) D. W. Stephan and G. Erker, Frustrated Lewis Pair Chemistry: Development and Perspectives, Angew. Chem., Int. Ed., 2015, 54, 6400–6441 CrossRef CAS PubMed
;
(g) D. W. Stephan, Frustrated Lewis Pairs, J. Am. Chem. Soc., 2015, 137, 10018–10032 CrossRef CAS PubMed
;
(h) D. W. Stephan, Frustrated Lewis Pairs: from Concept to Catalysis, Acc. Chem. Res., 2015, 48, 306–316 CrossRef CAS PubMed
;
(i) S. A. Weicker and D. W. Stephan, Main Group Lewis Acids in Frustrated Lewis Pair Chemistry: Beyond Electrophilic Boranes, Bull. Chem. Soc. Jpn., 2015, 88, 1003–1016 CrossRef CAS
;
(j) D. W. Stephan, The Broadening
Reach of Frustrated Lewis Pair Chemistry, Science, 2016, 354, aaf7229 CrossRef PubMed
. -
(a) M. A. Legare, M. A. Courtemanche, E. Rochette and F. G. Fontaine, Metal-Free Catalytic C-H Bond Activation and Borylation of Heteroarenes, Science, 2015, 349, 513–516 CrossRef CAS PubMed
;
(b) M. A. Legare, E. Rochette, L. J. Legare, N. Bouchard and F. G. Fontaine, Bench-Stable Frustrated Lewis Pair Chemistry: Fluoroborate Salts as Precatalysts for The C-H Borylation of Heteroarenes, Chem. Commun., 2016, 52, 5387–5390 RSC
;
(c) M. A. Courtemanche, M. A. Legare, L. Maron and F. G. Fontaine, A Highly Active Phosphine-Borane Organocatalyst for the Reduction of CO2 to Methanol Using Hydroboranes, J. Am. Chem. Soc., 2013, 135, 9326–9329 CrossRef CAS PubMed
;
(d) R. Declercq, G. Bouhadir, D. Bourissou, M. A. Légaré, M. A. Courtemanche, K. S. Nahi, N. Bouchard, F. G. Fontaine and L. Maron, Hydroboration of Carbon Dioxide Using Ambiphilic Phosphine-Borane Catalysts: on the Role of the Formaldehyde Adduct, ACS Catal., 2015, 5, 2513–2520 CrossRef CAS
. -
(a) K. Chernichenko, B. Kotai, I. Papai, V. Zhivonitko, M. Nieger, M. Leskela and T. Repo, Intramolecular Frustrated Lewis Pair with the Smallest Boryl Site: Reversible H2 Addition and Kinetic Analysis, Angew. Chem., Int. Ed., 2015, 54, 1749–1753 CrossRef CAS PubMed
;
(b) K. Chernichenko, M. Lindqvist, B. Kotai, M. Nieger, K. Sorochkina, I. Papai and T. Repo, Intramolecular Frustrated Lewis Pair with the Smallest Boryl Site: Reversible H2 Addition and Kinetic Analysis, J. Am. Chem. Soc., 2016, 138, 4860–4868 CrossRef CAS PubMed
. - C. M. Möming, E. Otten, G. Kehr, R. Frölich, S. Grimme, D. W. Stephan and G. Erker, Reversible Metal-Free Carbon Dioxide Binding by Frustrated Lewis Pairs, Angew. Chem., Int. Ed., 2009, 48, 6643–6646 CrossRef PubMed
. - A. J. P. Cardenas, B. J. Culotta, T. H. Warren, S. Grimme, A. Stute, R. Frölich, G. Kehr and G. Erker, Capture of NO by a Frustrated Lewis Pair: A New Type of Persistent N-Oxyl Radical, Angew. Chem., Int. Ed., 2011, 50, 7567–7571 CrossRef CAS PubMed
. - R. C. Neu, E. Otten, A. Lough and D. W. Stephan, The synthesis and exchange chemistry of frustrated Lewis pair-nitrous oxide complexes, Chem. Sci., 2011, 2, 170–176 RSC
. - M. Sajid, A. Klose, B. Birkmann, L. Liang, B. Schirmer, T. Wiegand, H. Eckert, A. J. Lough, R. Fröhlich, C. G. Daniliuc, S. Grimme, D. W. Stephan, G. Kehr and G. Erker, Reactions of phosphorus/boron frustrated Lewis pairs with SO2, Chem. Sci., 2013, 4, 213–219 RSC
. - C. M. Möming, S. Fröel, G. Kehr, R. Frölich, S. Grimme and G. Erker, Reactions of an Intramolecular Frustrated Lewis Pair with Unsaturated Substrates: Evidence for a Concerted Olefin Addition Reaction, J. Am. Chem. Soc., 2009, 131, 12280–12289 CrossRef PubMed
. - M. A. Dureen and D. W. Stephan, Terminal Alkyne Activation by Frustrated and Classical Lewis Acid/Phosphine Pairs, J. Am. Chem. Soc., 2009, 131, 8396–8397 CrossRef CAS PubMed
. - H. H. San, S.-J. Wang, M. Jiang and X.-Y. Tang, Boron-Catalyzed O-H Bond Insertion of α-Aryl α-Diazoesters in Water, Org. Lett., 2018, 20, 4672–4676 CrossRef CAS PubMed
. - Y. Dong, H. Zhang, J. Yang, S. He, Z.-C. Shi, X.-M. Zhang and J.-Y. Wang, B(C6F5)3-Catalyzed C-C Coupling of 1,4-Naphthoquinones with the C-3 Position of Indole Derivatives in Water, ACS Omega, 2019, 4, 21567–21577 CrossRef CAS PubMed
. -
(a) S. Sayin and M. Yilmaz, Synthesis and investigation of catalytic affinities of water-soluble amphiphilic calix[n]arene surfactants in the coupling reaction of some heteroaromatic compounds, Tetrahedron, 2016, 72, 6528–6535 CrossRef CAS
;
(b) S. Sayin and M. Yilmaz, Brønsted acidic magnetic nano-Fe3O4-adorned calix[n]arene sulfonic acids: synthesis and application in the nucleophilic substitution of alcohols, Tetrahedron, 2014, 70, 6669–6676 CrossRef CAS
;
(c) G.-P. Fan, Z. Liu and G.-W. Wang, Efficient ZnBr2-catalyzed reactions of allylic alcohols with indoles, sulfamides and anilines under high-speed vibration milling conditions, Green Chem., 2013, 15, 1659–1664 RSC
;
(d) D. Das and S. Roy, Palladium(II)-Catalyzed Efficient C-3 Functionalization of Indoles with Benzylic and Allylic Alcohols under Co-Catalyst, Acid, Base, Additive and External Ligand-Free Conditions, Adv. Synth. Catal., 2013, 355, 1308–1314 CrossRef CAS
;
(e) M. Bandini, A. Melloni and A. Umani-Ronchi, New Versatile Pd-Catalyzed Alkylation of Indoles via Nucleophilic Allylic Substitution: Controlling the Regioselectivity, Org. Lett., 2004, 6(18), 3199–3202 CrossRef CAS PubMed
. - CCDC 1970839 (3q) and CCDC 1970838 (3al) contain supplementary crystallographic data for this paper.
- S. J. Geier and D. W. Stephan, Lutidine/B(C6F5)3: At the Boundary of Classical and Frustrated Lewis Pair Reactivity, J. Am. Chem. Soc., 2009, 131, 3476–3477 CrossRef CAS PubMed
. - J.-H. Zheng, X.-T. Fan, B.-Y. Zhou, Z.-H. Li and H.-D. Wang, Tautomerization of 2,6-lutidines in the presence of B(C6F5)3 using catecholborane as a precatalyst, Chem. Commun., 2016, 52, 4655–4658 RSC
.
Footnote |
† Electronic supplementary information (ESI) available: Experimental procedures, spectroscopic data for all compounds. CCDC 1970838 and 1970839. For ESI and crystallographic data in CIF or other electronic format see DOI: 10.1039/d0ra02912b |
|
This journal is © The Royal Society of Chemistry 2020 |