DOI:
10.1039/D0RA01165G
(Paper)
RSC Adv., 2020,
10, 16254-16265
Effects of germination time and kilning temperature on the malting characteristics, biochemical and structural properties of HomChaiya rice
Received
6th February 2020
, Accepted 16th April 2020
First published on 23rd April 2020
Abstract
Effects of germination time (3, 5 and 7 days) and kilning temperature (40, 50 and 60 °C) on the malting characteristics, biochemical properties and structural properties of HomChaiya rice were examined. Malting potential in terms of germination rate and germination capacity increased as the germination period of rice was prolonged. Diastatic potential, hot water extract and malting loss of rice gradually increased with germination time and with kilning temperature; in contrast, malting yield and viscosity of the samples decreased. Germination time significantly increased the α-amylase activity, but β-amylase activities increased when kilned at different temperatures. Total starch decreased and reducing sugar increased in rice with prolonged germination, and furthermore, the kilning temperature significantly influenced these changes. Higher kilning temperature and prolonged germination period increased the protease activity in rice, and consequently, soluble protein and free amino acids also increased. Among the twelve identified amino acids in the HomChaiya rice, aspartic acid, glutamic acid, asparagine, serine, arginine, isoleucine, tyrosine, and phenylalanine increased with germination time and kilning temperature. FTIR results showed that increased germination time and kilning temperature unfolded the carbohydrates, which is consistent with the enzymatic (α- and β-amylase) activities. XRD results also found higher peak intensities for rice when germinated longer and kilned at a higher temperature. The crystallinity of malted rice decreased with germination time. Ultrastructural changes showed that starch granules are more vulnerable to enzymatic attack upon extended germination time and at higher kilning temperatures.
Introduction
Thailand is one of the largest producers of rice around the world and has the greatest biodiversity of rice. Oryza sativa L., also known as Asian rice, is the main species produced in Thailand and includes 89 varieties of rice (16 from central, 12 from northern, 23 from northeastern and 38 from southern regions). Most of these varieties are regional and known only to the local population. This is due to the planting of government recommended rice varieties, with local differences in the ecosystem, environment, consumer choice, and economic values.1,2 HomChaiya rice (also called Kao HomChaiya) is an indigenous rice variety that is specifically produced in the Chaiya district, Surat Thani province, Thailand. It is unique and well known for its distinct flavor and color (pale brown color). It is normally sold in unpolished form. HomChaiya rice is normally consumed as a staple food, and because of limited availability, this rice is not widely known or widely marketed. HomChaiya rice contains various nutrients, including vitamins (A, B1 and B2 and E), minerals (Fe, Zn and Ca), polyphenols, gamma oryzanol and gamma-aminobutyric acid.3 Several studies have reported that germination and malting could enhance the nutritional content many-fold in cereal grains.4 Recently, rice has been studied as a subject of the malting process as it is gluten-free, so it could be used in gluten-free beer, beverages, bakery products, and confectionaries.5
Malting is an important process that could help create industrial food products and/or raw materials, especially sugar and alcoholic beverages. Malting is a simple technique, which involves a sequence of steeping, germination, and kilning. Grains undergo biochemical changes during steeping and germination and consequently secrete and mobilize various hydrolytic enzymes (amylase and protease) involved in breaking down starch and proteins into sugar and various amino acids.6,7 Malting not only alters contents in the cereals, but it could also improve the organoleptic characteristics such as flavor, taste, color, and tenderness of food products.5,8 Germination consists of three phases, and the first phase starts after the rice has undergone steeping, followed by swelling. This stage induces respiration, energy metabolism, and protein synthesis in rice. In the second phase, rice swelling stagnates and reserve mobilization in the rice embryonic tissue starts. In the third phase, rice embryo cells expand, and major storage reserves mobilize causing rapid hydrolyzation and accumulation of osmotic compounds.9 Several studies have reported that prolonged germination of cereals extends the third phase of germination and leads to various active compounds.10,11 Farzaneh et al.12 reported that cereals germinated for seven days had twofold increased enzymatic activities and consequently, the diastatic activity of cereal malt was increased. Skendi and Papageorgiou13 reported that barley malt kilned at various temperatures showed significant changes in its chemical composition. Nie et al.14 observed that the optimum kilning temperature could increase numerous health-associated and flavor inducing amino acids.
HomChaiya rice has not been studied regarding its malting capabilities or its germination endurance over a prolonged duration or regarding the effects of kilning temperature. Therefore, this present study experimentally assessed the effects of extended germination time and different kilning temperatures on the malting characteristics, biochemical response, and structural integrity of HomChaiya rice.
Results and discussion
Malting characteristics
Malting characteristics of malted HomChaiya rice at the tested germination times and kilning temperatures are shown in Fig. 1A–F. Malting potentials such as germination rate (GR), germination capacity (GC), and dormancy rate (DR) of HomChaiya rice were affected by germination time (Fig. 1A). GR gradually increased with germination time. Prolonged germination significantly increased GR of malted HomChaiya rice. At initial germination time, the GC was very low; as germination progressed, the GC was reached to a high level. Conversely, the DR decreased gradually with germination time. DR represents the inhibitory influence of husk that covers the embryo to protect the grains from moisture.15 During germination, the husk is weakened by water that penetrates the embryo and induces enzymatic reactions in the grain. A decreased DR in germinated rice indicates high-quality malt and is associated with increased GC of the malted rice. Olugbile et al.11 suggested that rice with GR above 90% during germination is an indicator of good quality malt. Diastatic potency (DP) identifies the efficiency of the saccharifying power of the malted rice. It indicates the conversion of starch in malted rice by the diastase enzymes, particularly α- and β-amylase.7,12 The DP of malted HomChaiya rice was significantly affected by both germination time and kilning temperature (Fig. 1B). Prolonged germination increased the diastatic potential of HomChaiya rice. In addition, kilning temperature could also significantly increase the diastatic potential of rice that germinated for 7 days. Aniche and Palmer16 found that increased DP in malted rice was mainly influenced by the accumulation of gibberellic acid (GA) during germination, as GA increased the β-amylolytic activity in malted rice. This is in accordance with the present study, where the β-amylase activity significantly increased with kilning temperature. This study also found that α-amylase activity increased more than β-amylase activity with malting time, and possibly α-amylase could also be contributed to the increment of DP in the malted rice. Malting yield of germinated HomChaiya rice is shown in Fig. 1C. Increasing germination time and kilning temperature adversely affected by the malting yield of rice. The malting yield of rice germinated from 5 to 7 days was nearly constant but differed significantly from the 3rd day of germination. Similarly, the increasing kilning temperature increased the malting yield of rice. On the other hand, malting loss continuously increased with germination time and kilning temperature (Fig. 1D). Malting yield and malting loss were in direct proportion to each other. Malting loss refers to the material loss that occurred by the conversion of rice into malt, specifically from starch degraded into sugar. Suhasini and Malleshi17 reported that the cause of malting loss in cereals is metabolic activity and onset of vegetative growth in the grains. Vinje et al.10 found that increased malting loss in the grain was predominantly caused by increased activity of diastase. Olugbile et al.11 observed that prolonged germination induced up to 54.55% malting loss in germinated rice and recommended that malting loss of germinated rice should be below 20% for economic viability. In the present study, the malting loss in rice was less than 18% at the end of the germination period. Hot water extract (HWE) significantly increased with germination time and kilning temperature (Fig. 2E). HomChaiya rice germinated for 7 days and kilned at 60 °C had increased HME, but the differences were not much. Moneke et al.18 mentioned that HWE is directly related to the diastatic power of the malt. The study of Farzaneh et al.12 had also observed increased HWE from prolonged germination of rice, and it was because of increased β-glucanase and α-amylase activities. The viscosity, which shows the pasting properties of rice, decreased with germination time and kilning temperature. Surprisingly, the kilning temperature, particularly at 40 °C, did not significantly affect the viscosity (Fig. 2F). Germinated HomChaiya rice kilned at 50 °C showed no effect on viscosity on the 3rd day of germination, but the 5th and 7th days of germination showed significant effects. On the other hand, germinated rice kilned at 60 °C had significantly decreased viscosity. Gopaldas et al.19 reported that any amylase rich food could have effectively reduced viscosity because of the hydrolyzation of starch. Fox et al.20 also observed the lower viscosity of malted rice and attributed it to the increased activities of proteases and amylase, which influence the gelatinization temperature and reduce pasting properties.
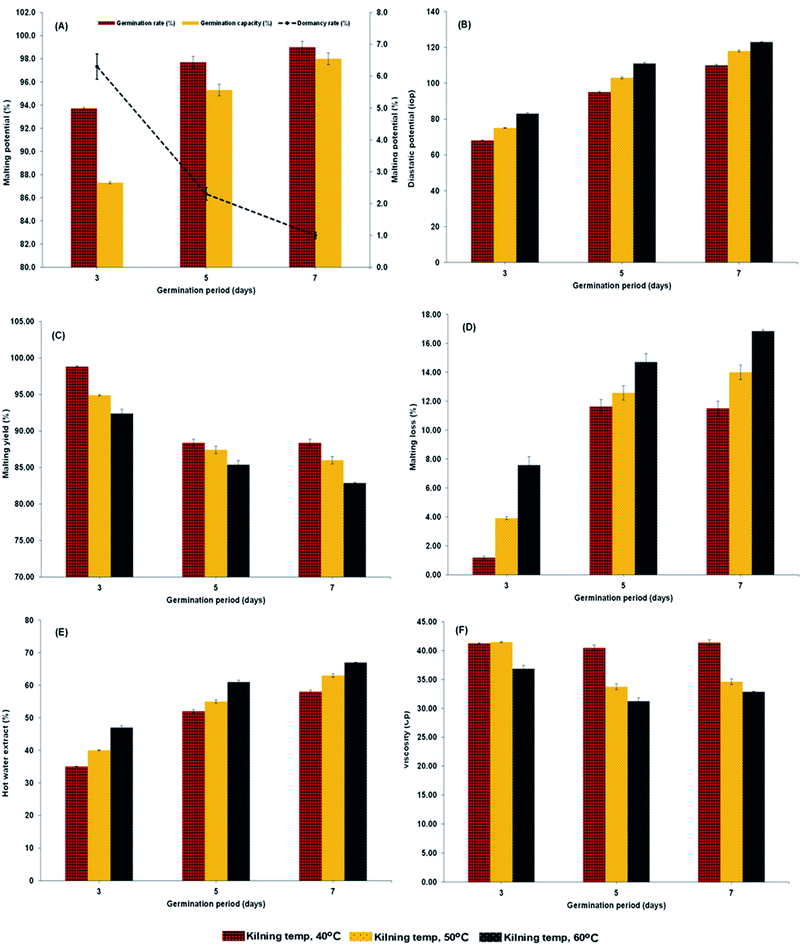 |
| Fig. 1 Diastatic potential (A), malting potential (B), malting yield (C), malting loss (D), hot water extract (E) and viscosity (F) of malted HomChaiya rice granules at different germination times when kilned at different temperatures. | |
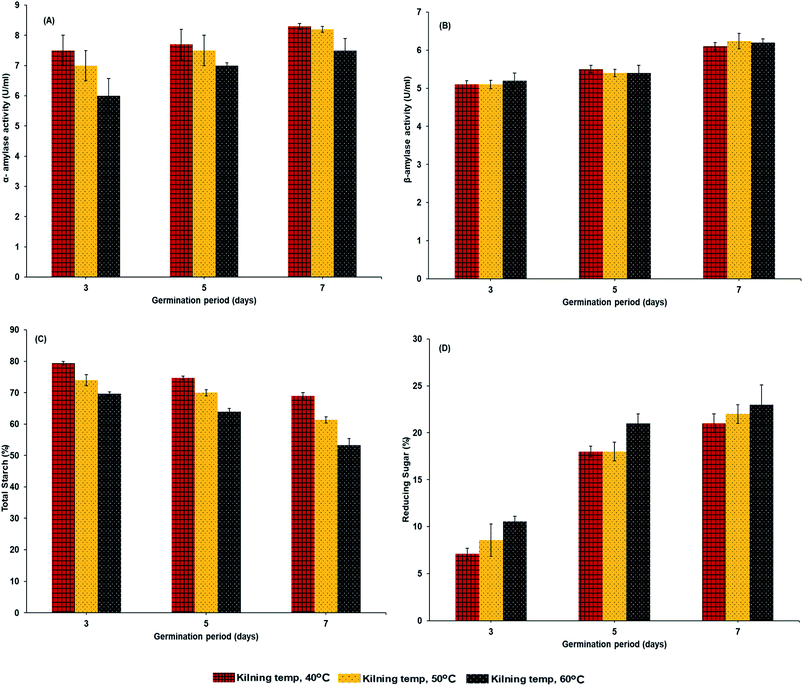 |
| Fig. 2 α-Amylase activities (A), β-amylase activities (B), total starch (C) and reducing sugar (D) of malted HomChaiya rice granules at different times and kilned at different temperatures. | |
α- and β-Amylase activities, total starch and reducing sugar
Enzymatic activities of α-amylase and β-amylase are shown in Fig. 2A and B. The germination time gradually increased amylase activities. α-Amylase activities were higher than those of β-amylase in the HomChaiya germinated rice. α-Amylase is a crucial enzyme, whose primary role is in the hydrolysis of the stored reserves in rice, to provide energy to the developing embryo. Damaris et al.21 reported that the activity of α-amylase is usually high during germination. Among the three observed germination times, the HomChaiya rice germinated for 7 days showed the highest α- and β-amylase activities. In addition, the α-amylase slightly dominated over β-amylase in germinated rice. The results showed that kilning temperature could influence the enzymatic activities in germinated rice, although it did not significantly affect the β-amylase activity as it did to α-amylase (Fig. 2A). Phiarais et al.22 found that α-amylase activity was higher when kilned at low temperatures as compared to higher temperatures, and this indicates that this enzyme is heat sensitive so that prolonged kilning at high temperatures could heat-denature the enzyme. On the other hand, the β-amylase had no significant differences among the kilning temperatures, and probably β-amylase is more heat stable than α-amylase. Mensah et al.15 revealed that β-amylase activities were very stable when processed at 65 °C. In accordance with the enzyme activities, the total starch and reducing sugar were also significantly influenced by germination time and kilning temperature (Fig. 2C and D). However, the starch and sugar contents in germinated HomChaiya rice are directly impacted by the enzyme activities, as shown in Fig. 3A and B. Total starch in the germinated rice gradually decreased as the amylase enzyme activities increased. Starch content in the rice decreased from conversion into reducing sugars (Fig. 2D). An increase of kilning temperature slightly decreased the total starch content in the rice but increased reducing sugar content of HomChaiya rice. Germination time did not significantly affect the total starch content in the rice samples kilned at 40 °C, though with other kilning temperatures, it had significant influence. Park et al.23 reported that β-amylase could hydrolyze rice starch more effectively than α-amylase. The reducing sugar content gradually increased with germination time. At the initial stage of germination, it was below 10% and could be able to reach 25% by the end of the germination period. Velupillai et al.24 reported an increase in reducing sugar during the germination period that depended on the type of cereal. Ayernor and Ocloo7 also found an increasing trend in reducing sugar with prolonged germination of paddy rice. The differences in reducing sugar between the 3rd and 5th days of germination were very high. It could be due to the consumption of reducing the sugar by the rice itself in various biochemical reactions, which can exceed the production rate during germination. This is in an agreement with the study of Magneschi and Perata.25
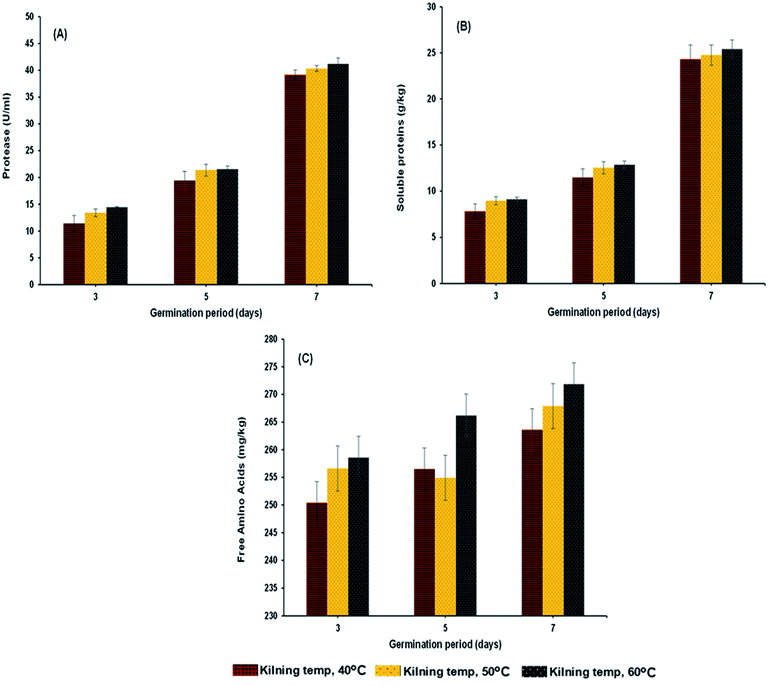 |
| Fig. 3 Protease (A), soluble proteins (B) and reducing sugar (C) of malted HomChaiya rice granules at different germination times when kilned at different temperatures. | |
Protease activity, soluble protein, free amino acids, and amino acid profile
Protease activity of the samples increased steadily with germination time and kilning temperature (Fig. 3A). The level of peptidase activity was at a high level when the germination time increased. Gibberellins in the embryo of rice are responsible for the production of various enzymes, especially protease, and increased protease activity helps break down stored proteins in the rice grain to various proteins and amino acids, which are then used as the substrate to help secrete more gibberellins.26–28 On the other hand, the kilning temperature only slightly affected the protease activity in germinated rice, and an increased kilning temperature did not adversely affect the protease activity. Rizvi et al.29 studied thermostable protease activities in malted barley kilned at different temperatures and found that the germination period significantly influenced protease activity and increased thermostable proteases. Therefore, the insoluble protein content in the malted grains is hydrolyzed to soluble proteins and peptides and subsequently to amino acids. This is in accordance with the present study, where most of the soluble protein (Fig. 3B) and amino acid contents (Fig. 4A–L) in the malted rice increased with germination time and kilning temperature. Similar to the protease activities, the soluble protein in the malted rice increased threefold during germination. The kilning temperature slightly increased the protein content in malted rice, but the differences by temperature were minimal compared to the effects of germination time. Generally, soluble proteins are the main source of nitrogen to a germinated grain. Several studies have reported that soluble proteins are dependent on protease activities and also on the type of grains involved. Furthermore, the free amino acids in HomChaiya rice steadily increased during germination and with kilning temperature (Fig. 3C). However, there was a lower level of amino acids observed in the malted rice on the 5th day of germination and kilned at 50 °C. Amino acids could be consumed in metabolic processes. On the other hand, this study found a total of 12 individual amino acids in the malted HomChaiya rice, and among them, asparagine, arginine, lysine, leucine, isoleucine, alanine and tyrosine were found at higher levels in the malted HomChaiya rice; whereas aspartic acid, glutamic acid, serine, valine, and phenylalanine were found at lower levels (Fig. 4A–L). Among the various amino acids, prolonged germination increased almost every identified amino acid in this study, except for valine, leucine and alanine that decreased with germination time. On the other hand, surprisingly, the kilning temperature plays a crucial role in the amino acid contents in malted HomChaiya rice. An increase of kilning temperature gradually increased the levels of amino acids in malted rice, along with germination time. However, lysine, valine, and alanine decreased with the kilning temperature. Furthermore, tyrosine showed no significant effect from kilning temperature. Normally, amino acids play a crucial role in nutritional value as well as in the regulation of metabolic functions of humans. Arendt and Zannini30 reported that amino acid compositions of cereal grains are quite similar to each other across cereal types. Nie et al.14 observed changes in the amino acid composition of barley malt from prolonged germination as well as from kilning temperature, and it was due to the consumption of amino acids as the substrate to produce various metabolic products.
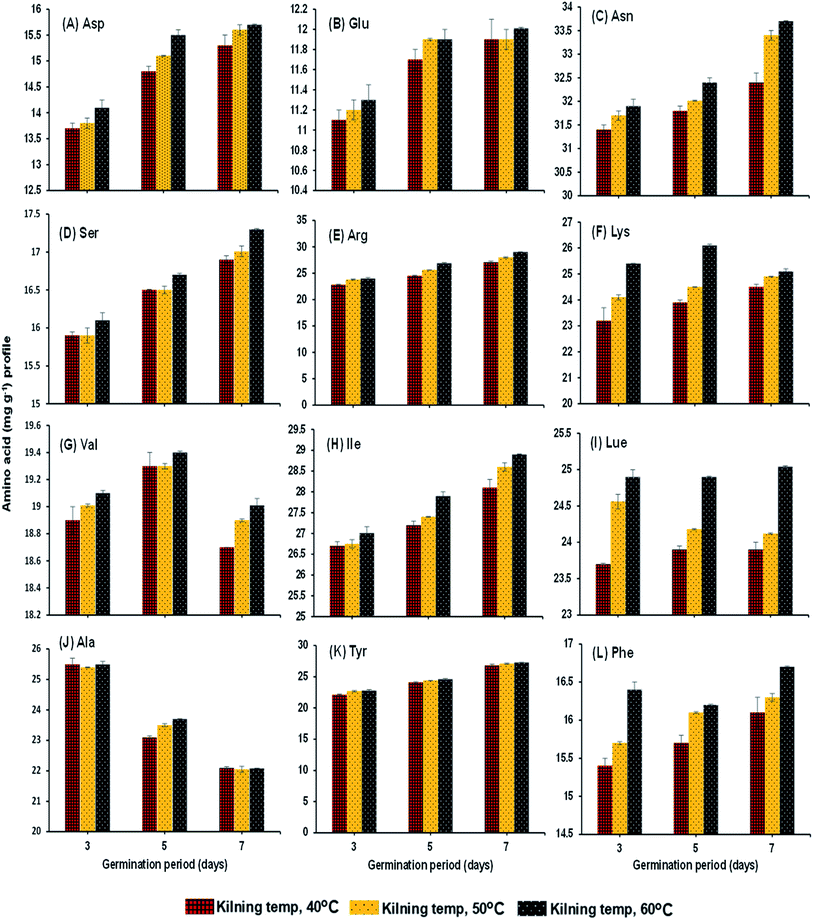 |
| Fig. 4 Amino acid profile of malted HomChaiya rice granules at different germination times when kilned at different temperatures. | |
FT-IR absorbance spectrum (FTIR), X-ray diffractograms (XRD), and crystallinity
The changes in the FTIR of malted HomChaiya rice are shown in Fig. 5A. The differences in the absorbance spectra show that malting time and kilning temperature significantly influenced the structure of the germinated rice. In the spectra from 800 to 1600 cm−1 extensive peaks are noted from 850 to 1164 cm−1, from stretching vibrations of the C–H group that represents carbohydrates and specifically starch. Fang et al.31 reported that FTIR peaks observed between 1020 and 1156 cm−1 could be for anhydrous glucose ring C–O stretching. The band intensities fluctuated because of the enzymatic hydrolysis of starch in the germinated rice. Sun et al.32 observed that FTIR band intensity changes could be from specific conformational changes of the structure, particularly long-range ordering and crystallinity. A similar observation was also noted by Mu et al.33 FTIR results revealed that most of the changes in germinated HomChaiya rice occurred by the 5th day of germination, and the changes peaked when the rice germination was reached the 7th day. Xu et al.34 also observed a high-intensity band for adlay seeds germinated for various times, and their results showed a longer germination time gave higher intensity, similar to this study. Higher kilning temperatures, particularly 50 and 60 °C, stimulated these changes more than 40 °C. Temperature plays a key role in the activation of enzymes. The large spectral changes found by temperature might reflect enzymatic activities in the starch (see Fig. 2A and B) during kilning. The semi-crystalline nature of starch is vastly diverse by plant type and by processing conditions.35 XRD is a well-known method for detecting and characterizing starch granules.36 X-ray diffractograms of malted HomChaiya germinated rice are shown in Fig. 5B. Germination time and kilning time influenced the diffractograms of the rice samples. Khunae et al.37 reported that native starch exhibits three types of crystallinity (A, B, and C) and normally cereal starch is of A-type,5 which exhibits close packing of amylopectin double helixes. In this study, the main diffraction peaks of germinated rice were observed at 2Θ 15.05, 17.38, 18.03 and 23.10, but the intensity of diffraction varied widely. Rice samples germinated for a longer time and kilned at a higher temperature showed a significantly higher intensity (P < 0.05). This is because the germinated rice starch was disrupted by gelatinization in the kilning process.38,39 Xu et al.40 reported that germinated rice starch had lowered gelatinization temperature (<60 °C). Because of the kilning temperature, the germinated rice kilned at the higher temperatures had decreased crystallinity (Fig. 5C). The crystallinity decreased with germination time and kilning temperature. Among the various samples, the germinated rice kilned at 60 °C had lower crystallinity than others. This is in accordance with Chungcharoen et al.39 Also, Contreras-Jimenez et al.41 observed that prolonged germination gradually decreased the crystallinity of barley. Furthermore, the crystallinity degree of germinated starch strongly depends on the ratio of amylose and amylopectin, chain length, molecular weight, and lipid and protein contents.5,27
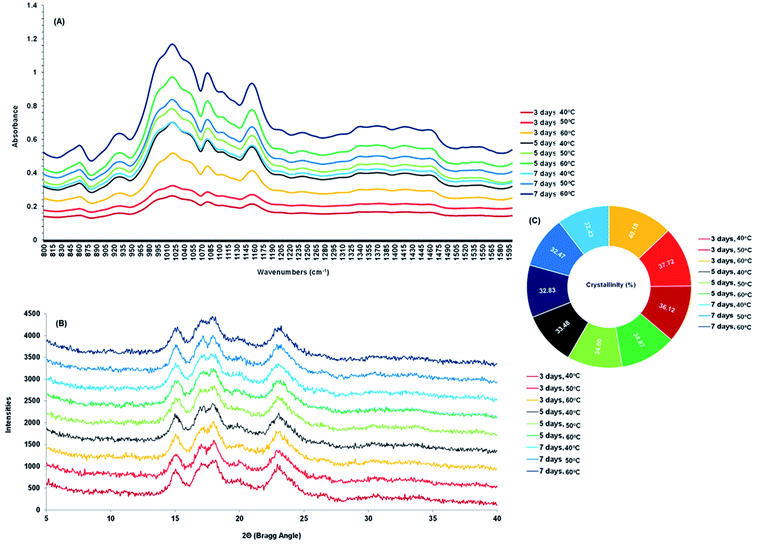 |
| Fig. 5 FT-IR absorbance spectrum (A), X-ray diffractograms (B) and crystallinity (C) of malted HomChaiya rice granules at different germination times when kilned at different temperatures. | |
Ultrastructural changes
The trends in HomChaiya rice ultrastructure by germination time and kilning temperatures are shown in Fig. 6A–I. Germination time and kilning temperature affected the surface of the malted HomChaiya rice granules. The changes in the germinated rice samples include particularly more void space and fragmented surfaces of the rice granules from prolonged germination and kilning. Fragmented rice surfaces increased with germination time (see Fig. 6D–I). The kilning temperature slightly affected the rice granule surfaces, less than the germination time, which induced significant changes. However, the rice kilned at 60 °C showed a more fragmented surface than the other cases. Several studies have reported morphological changes in cereals from enzymatic hydrolysis. Usansa42 reported that the rice embryo is located at the ventral side, and prolonged germination could induce the synthesis of gibberellic acid in the embryo, which then penetrated through the scutellar epithelium and diffused into the aleurone layers and started synthesis of α- and β-amylases through a de novo pathway. Contreras-Jimenez et al.41 also suggested that changes in the rice granule surface area were caused by partial enzymatic hydrolysis of starch. As can be seen in Fig. 2B, increasing the kilning temperature was gradually increased the β-amylase activity in malted rice, along with the germination period. However, kilning did not much affect the α-amylase activity. Wijngaard et al.43 reported that enzymes, such as β-amylase and amyloglucosidase, are the precursors causing pitting and superficial erosion on the malted grains. These changes occur on the malted rice grain surfaces because the enzymes degrade starch at random places. Usansa42 found significant changes in the rice starch close to the embryo and aleurone layers, where most of the amylase enzymes that facilitate hydrolyzation are found. Yu et al.44 reported that during and after malting, there were modifications to starch and protein in the rice granules, which resulted in the rough surfaces of malted rice granules.
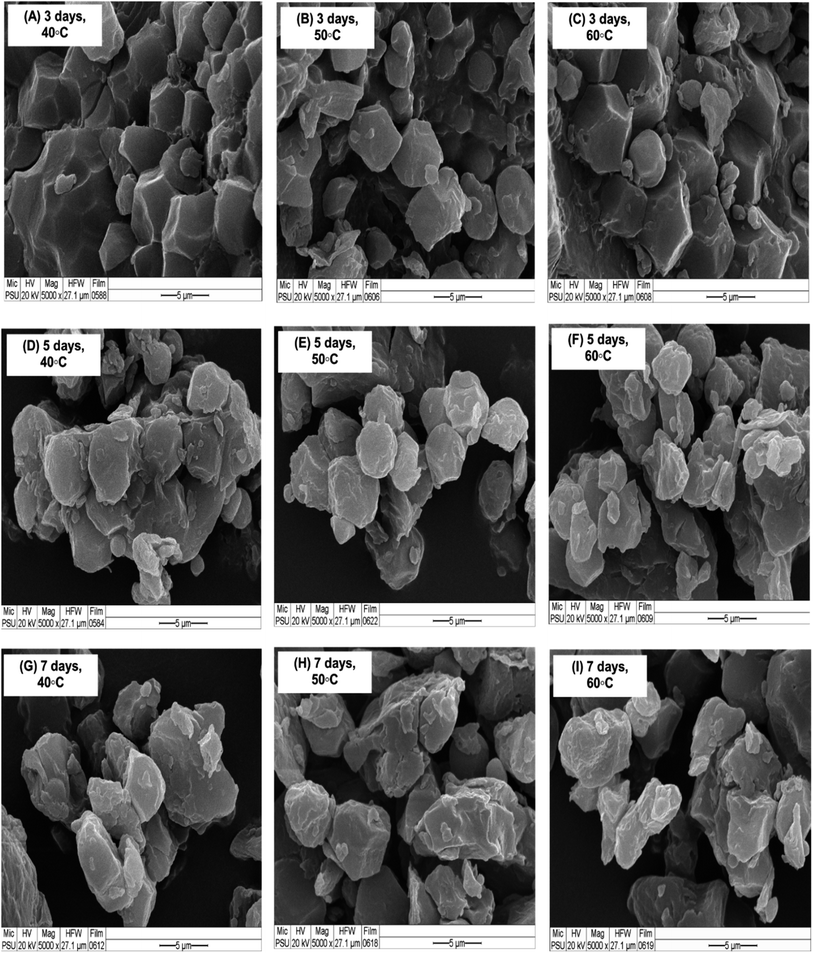 |
| Fig. 6 Surface ultrastructural changes of malted HomChaiya rice granules germinated for different times when kilned at different temperatures. | |
Experimental
Raw material, malting, and kilning process
HomChaiya rice (Oryza sativa L. HomChaiya) was purchased from a local producer in Chaiya district, Surat Thani Province, Thailand. The rice was thoroughly cleaned to remove any foreign materials and prepared for malting. The cleaned HomChaiya rice was malted and kilned as schematically shown in Fig. 7. It was malted for three different germination times (3, 5, and 7 days) followed by kilning at three alternative kilning temperatures (40, 50, and 60 °C). After processing, the malted rice samples were cleaned of rootlets and husks, ground, and stored under refrigerated conditions until further use. The malted HomChaiya rice was subjected to various determinations described next.
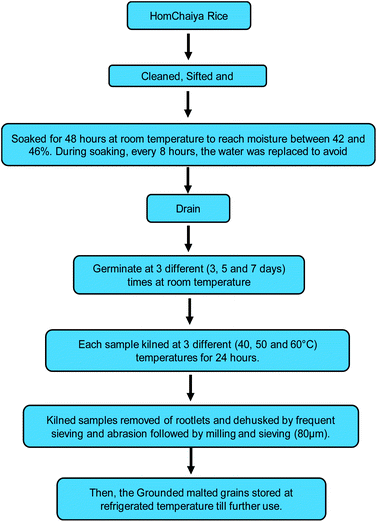 |
| Fig. 7 Experimental scheme for malting and kilning of HomChaiya rice. | |
Analysis
Malting potential. HomChaiya rice was tested for the malting potential, which includes germination rate (GR), dormancy rate (DR) and germination capacity (GC), following the method of Olugbile et al.11 HomChaiya rice (100 no. each) was soaked in water for 48 h at room temperature and after that the soaked rice grains were placed in muslin cloth bags for the various germination times, as shown in Fig. 7. After germination, the grains were examined by counting the germinated and ungerminated grains. The percentages of GR, DR, and GC in the germinated rice were calculated using the following formulas.
Diastatic potential. The diastatic potential of malted HomChaiya rice was measured using Fehling's reagent method, as briefly described by Farzaneh et al.12 The diastatic potential was calculated as follows.
Diastatic potential = (2000 − 200)/(XY − XS) |
Here X represents malt extract amount (mL), Y represents converted starch solution (mL) to 5 mL of the Fehling's solution, and S represents the titer for the starch blank.
Malting yield and malting loss. Malting yield and malting loss of HomChaiya rice were measured in accordance with the method of Kalita et al.5 Rice kernel (100 no.) were weighed before and after malting (the rice after malting was cleaned by hand removing plumules and radicles). Malting yield and malting loss of rice were calculated as follows.
Hot water extract (HWE). HWE of malted HomChaiya rice was measured in accordance with the method of Swanston.45 After HWE of malted rice by temperature timing methods and the specific weight to the extract was determined by the pycnometer and then the extract brix value was identified with the plateau table. The efficiency of the extract was determined as follows.
Here E represents the percentage of hot water extract, M represents the moisture percentage in malted rice, and P represents the total soluble solids in 100 g of extract as found from the plateau table.
Viscosity. The viscosity of HomChaiya rice malt paste was determined using the method of Ayernor and Ocloo7, and the Brookfield Viscometer was used to analyze the viscosity. 10 grams of sample was dissolved in 500 mL of distilled water to obtain a 2% slurry and then the suspension was heated in water for 30 min at 90 °C. After that, the viscosity of the gelatinized rice malt was measured at 75 °C and 50 rpm using a spindle. The results are expressed in cP.
Amylase activities. A germinated rice sample (1 g) was mixed in 5 mL of Tris/HCl buffer (1 M, pH 8.0) containing 20 mM disodium EDTA and 100 mM cysteine HCl. The amylase enzymes were extracted at room temperature for 60 min with occasional vortexing. After that, the extract was centrifuged for 10 min at 4000 rpm. Then the supernatant was collected and diluted with buffer. For α-amylase, 1 mL of supernatant was diluted with 2 mL of a buffer containing sodium malate (1 M, pH 5.4) with 1 M NaCl, 40 mM CaCl2, and 0.1% NaN3. For β-amylase, 1 mL of supernatant was diluted with 50 mM MES buffer, pH 6.2. After that, these diluted amylase extracts were measured for activities. For α-amylase activity, 0.1 mL of enzyme extract was pre-incubated at 60 °C for 5 min, followed by adding 0.1 mL of block p-nitrophenyl maltoheptaoside, and incubation continued for 10 min at 60 °C. For β-amylase activity, 0.1 mL of enzyme extract was pre-incubated at 50 °C for 5 min, followed by adding 0.1 mL of p-nitrophenyl β-maltotrioside, and then incubation continued at 50 °C for 10 min. After incubation, the enzyme reactions were terminated by adding 1.5 mL of 1% Trizma base with pH 11.0 and mixing thoroughly. Then, the absorbances were measured at 400 nm, from which amylase enzyme activities in HomChaiya rice were calculated.15 The results are expressed in units per gram.
Total starch. Total starch content in the rice was measured in accordance with the method of Farzaneh et al.12 using a commercial kit related to the AACC method 11–76. The results expressed as percentages were calculated as follows.
Here, ΔA = absorbance at 510 nm against the blank, F = sugar concentration, FV = final volume, and W = weight of flour (in mg).
Reducing sugar. Reducing sugar in the malted HomChaiya rice was measured following Miller.46 1 g sample of rice was ground into a fine powder, and then it was mixed with 10 mL distilled water and incubated for 30 min at 20 °C. After that, the mixture was centrifuged at 3000 g for 10 min to separate the solubilized reducing sugar. Then, supernatant (0.5 mL) was collected in a test tube and mixed with a 3,5-dinitrosalicylic acid solution (0.5 mL) followed by thorough mixing and boiled for 5 min to develop the color. Standard maltose sugar was used to identify the level of reducing sugar in the malted rice. The results are expressed as percentages.
Protease activity, soluble protein, and free amino acids. Extraction and assaying of protease activity in the malted HomChaiya rice were done following the method of Li et al.47 Malted rice (2 g) was homogenized in 8 mL of citric acid–phosphate buffer (0.1 mol L−1, pH 6.0) containing β-mercaptoethanol (5 mmol L−1) in an ice bath. Then, the homogenate was centrifuged at 16
000 × g for 30 min at 4 °C. The supernatant was used for protease activity determination. The reaction mixture contained 1 mL of casein (20 g L−1 in 0.1 mol citric acid–phosphate buffer, pH 3.5) and 1 mL of supernatant, which were thoroughly mixed and incubated at 40 °C for 10 min. After incubation, the reaction was stopped by incubated at 90 °C for 5 min. After that, 2 mL of TCA (0.4 mol L−1) was added and the reaction mixture containing tubes were cooled for 30 min at 4 °C, followed by centrifugation for 20 min at 12
000 × g. Then the absorbance at 275 nm was measured. The protease activity of malted rice is expressed in units per gram.The soluble protein content in the malted rice samples was extracted using 0.02 mol L−1 Tris–HCl buffer (pH 8). Then, the extract was centrifuged at 2000 × g for 5 min, and after that, the supernatant was used to measure the soluble protein in accordance with the method of Bradford.48
The free amino acid content in the malted rice samples was analyzed using the ninhydrin method with L-leucine as standard.49 The results are expressed in mg per kg.
Amino acid profile. Amino acid composition of malted HomChaiya rice was determined based on the method of Ahmed et al.50 Rice samples were mixed with 6 N HCl in a heat probe special tube for hydrolysis and incubated for 24 h at 110 °C under vacuum conditions and after that, the extraction volume was adjusted to 50 mL. After hydrolysis, 5 mL of supernatant was dried by rotary evaporation at 65 °C in a water bath, and after that 5 mL of 0.02 mol L−1 HCl was added. The samples were washed thoroughly and filtered through a 0.22 μM aqueous phase filter. After that, the amino acids in the malted rice were quantified using an automatic amino acid analyzer (L-8900, Hitachi, Tokyo, Japan).
FT-IR absorbance spectrum (FTIR). FTIR spectrum of the HomChaiya rice was analyzed using an FTIR Spectrometer (Thermo Electron Corp., Madison, WI, USA). Malted rice flour was mixed with potassium bromide in a mass ratio of 1
:
100, the mixture was ground and pressed into a pellet. After that, the samples were measured for FTIR in the wavelength range 800–1600 cm−1 with a resolution of 2 cm−1.
X-ray diffractograms (XRD) and crystallinity. The XRD pattern and crystalline properties of the malted HomChaiya rice powder (sieved to obtained 40 mm size) were obtained from the XRD analyzer (Rigaku, Mini Flex, Japan, Tokyo) with a K value of 1.54040 operating at 40 kV acceleration potential and 40 mA current with a copper target. The angular range from 10 to 30° (2Θ) was covered at a fixed scanning speed (8° min−1). The diffractogram was plotted and smooth cure created and then the smooth curve was taken to use as the crystalline portion and the lower area in between the smooth curve and the linear baseline that connected the 2 points of the intensity 2Θ of 27 and 14 in the malted samples that were taken as an amorphous section. The crystallinity of the malted rice samples was estimated as follows.5
Ultrastructural changes. The ultrastructural changes of malted rice were studied using a scanning electron microscope (JXA-840A, JEOL, Tokyo, Japan), and micrographs were captured at 5000× magnification. The accelerating potential was 20 kV for imaging.
Statistical analysis. All experiments were conducted in triplicates, and the data are presented as mean ± standard deviation. The significant differences between the cases were statistically analyzed using an analysis of variance (ANOVA) by SPSS for windows. Means were compared for significant differences using Duncan's post hoc test at a 5% threshold level. The data were plotted using Microsoft Excel.
Conclusions
This study found that HomChaiya rice was significantly influenced by germination and by kilning temperature. Significant changes in the malting yield, malting loss, and viscosity were observed with the germination time of malted rice. The germination time positively influenced the rice sample by increasing malting potentiality, reducing sugar, proteins and amino acids. Furthermore, the kilning temperature had interaction effects with the germination time of malted rice. It was noted that the comparatively high kilning temperatures (50 and 60 °C) of malted rice influenced the protease enzymes, so that soluble protein and amino acids were markedly increased. While β-amylase activities improved under higher kilning temperatures, the α-amylase activity was inhibited. The structural properties of HomChaiya rice indicate that prolonged germination and also increased kilning temperature degraded the starch and reduced its crystallinity while reducing sugar increased. Though the malting yield and malting loss were adversely affected by extending germination and elevating the kilning temperature, the other important parameters in this study benefited markedly. Therefore, we recommend that HomChaiya rice could be germinated for 7 days and kilned at 60 °C for producing various food products, particularly rice beer or other alcoholic beverages.
Conflicts of interest
This work has no conflict of interest to declare.
Acknowledgements
This research was financially supported by Prince of Songkla University, Surat Thani Campus Collaborative Research Fund (Grant no. SIT6203022S). The authors would also like to acknowledge the Food Innovation and Product Development Laboratory for the provided research space and equipment support.
References
- P. Promsomboon and S. Promsomboon, J. Life Sci., 2016, 10, 371–374 Search PubMed.
- A. Salih, T. Sreewongchai, P. Sripichitt and N. Parinthawong, Kasetsart J., 2013, 47, 1–7 Search PubMed.
- N. Muntana and S. Prasong, Pak. J. Biol. Sci., 2010, 13(4), 170–174 CrossRef CAS PubMed.
- J. Aguilar, A. G. Miano, J. Obregon, J. Soriano-Colchado and G. Barraza-Jauregui, J. Cereal Sci., 2019, 90, 102858 CrossRef CAS.
- D. Kalita, B. Sarma and B. Srivatsava, Food Chem., 2017, 220, 67–75 CrossRef CAS PubMed.
- A. Bekele, G. Bultosa and K. Belete, J. Inst. Brew., 2012, 118, 76–81 CrossRef.
- G. S. Ayernor and F. C. K. Ocloo, Afr. J. Food Sci., 2007, 1, 037–041 Search PubMed.
- F. Wu, H. Chen, N. Yang, J. Wang, X. Duan and Z. Jin, J. Cereal Sci., 2013, 58, 263–271 CrossRef CAS.
- O. E. Makinen and E. K. Arendt, J. Am. Soc. Brew. Chem., 2016, 73(3), 223–227 CrossRef.
- M. A. Vinje, S. H. Duke and C. A. Henson, J. Am. Soc. Brew. Chem., 2015, 73(2), 195–205 CrossRef CAS.
- A. O. Olugbile, A. O. Obadina, A. O. Atanda, O. B. Omemu and S. O. A. Olatope, J. Food Process. Preserv., 2015, 39, 116–122 CrossRef CAS.
- V. Farzaneh, A. Ghodsvali, H. Bakhshabadi, Z. Zare and I. S. Carvalho, Int. J. Biol. Macromol., 2017, 94, 663–668 CrossRef CAS PubMed.
- A. Skendi and M. Papageorgiou, Millenium, 2018, 2(7), 49–58 CrossRef.
- C. Nie, C. Wang, G. Zhou, F. Dou and M. Huang, Afr. J. Biotechnol., 2010, 9(53), 9018–9025 CAS.
- E. O. Mensah, O. Ibok, W. O. Ellis and E. E. Carey, J. Nutr. Food Sci., 2016, 6(4), 1000515 Search PubMed.
- G. N. Aniche and G. H. Palmer, Ferment., 1998, 3, 378–380 Search PubMed.
- A. W. Suhasini and N. G. Malleshi, J. Food Sci. Technol., 1995, 32, 98–103 CAS.
- A. N. Moneke, B. N. Okolo, N. O. Orji and F. S. Ire, Bio-Res., 2009, 7(2), 514–518 Search PubMed.
- T. Gopaldas, S. Deshpande and C. John, Food Nutr. Bullet., 1888, 10(3), 1–10 Search PubMed.
- G. Fox, W. Yu, R. Nischwitz and S. Harasymow, J. Inst. Brew., 2019, 125, 18–27 CrossRef CAS.
- R. N. Damaris, Z. Lin, P. Yang and D. He, Int. J. Mol. Sci., 2019, 20, 1–17 Search PubMed.
- B. P. N. Phiarais, H. H. Wijngaard and E. K. Arendt, J. Inst. Brew., 2005, 111(3), 290–298 CrossRef CAS.
- S. H. Park, Y. Na, J. Kim, S. D. Kang and K. H. Park, Food Sci. Biotechnol., 2018, 27(2), 299–312 CAS.
- S. Velupillai, K. Nithyanantharajah, S. Vasantharuba, S. Balakumar and V. Arasaratnam, Rice Sci., 2009, 16(3), 240–242 CrossRef.
- L. Magneschi and P. Perata, Ann.
Bot., 2009, 103, 181–196 CrossRef CAS PubMed.
- A. K. Grennan, Plant Physiol., 2006, 141, 524–526 CrossRef CAS PubMed.
- M. Diza-Mendoza, I. Diza and M. Martinez, Int. J. Mol. Sci., 2019, 20(2087), 1–11 Search PubMed.
- K. Washio, Plant Physiol., 2003, 133, 850–863 CrossRef CAS PubMed.
- S. M. H. Rizvi, A. D. Beattie, B. Rossnagel and G. Scoles, Cereal Chem., 2011, 88(6), 609–613 CrossRef CAS.
- E. K. Arendt and E. Zannini, Barely, Woodhead Publish Ltd., 2013, pp. 155–201 Search PubMed.
- F. M. Fang, P. A. Fowler, J. Tomkinson and C. A. S. Hill, Carbohyd. Polym., 2002, 47(3), 245–252 CrossRef.
- Q. Sun, M. Gong, Y. Li and L. Xiong, Carbohyd. Polym., 2014, 110, 128–134 CrossRef CAS PubMed.
- T. Mu, M. Zhang, L. Raad, H. Sun and C. Wand, PLoS One, 2015, 10(12), e0143620 CrossRef PubMed.
- L. Xu, L. Chen, B. Ali, N. Yang, Y. Chen, F. Wu, Z. Jin and X. Xu, Food Chem., 2017, 229, 312–318 CrossRef CAS PubMed.
- J. A. Abediyi, A. O. Obadina, A. F. Mulaba-Bafubiandi, O. A. Abedo and E. Kayitesi, J. Cereal Sci., 2016, 70, 132–139 CrossRef.
- A. S. N. Musa, I. M. Umar and M. Ismail, African J. Biotech., 2011, 10(33), 6281–6291 Search PubMed.
- P. Khunae, T. Tran and P. Sirivongpaisal, Starch/Staerke, 2007, 59, 593–599 CrossRef CAS.
- T. Shiotsubo, Agric. Biol. Chem., 1983, 47(11), 2421–2425 CAS.
- T. Chunchareon, S. Prachayawarakorn, S. Soponronnarit and P. Tungtrakul, Drying Technol., 2012, 30, 1844–1853 CrossRef.
- J. Xu, H. Zhang, X. Guo and H. Qian, J. Sci. Food Agric., 2012, 92, 380–387 CrossRef CAS PubMed.
- B. Contreras-Jimenez, A. D. Real, B. M. Millan-Malo, M. Gayatan-Matrinez, E. Morales-Sanchez and M. E. Rodriguez-Garcia, J. Inst. Brew., 2019, 125, 10–17 CrossRef CAS.
- U. Usana, PhD thesis, Suranaree University, Thailand, 2008.
- H. H. Wijngaard and K. E. Arendt, J. Inst. Brew., 2006, 112(1), 57–65 CrossRef CAS.
- W. Yu, K. Tao, M. J. Gidley, G. P. Fox and R. G. Gilbert, Carbohyd. Polym., 2019, 206, 583–592 CrossRef PubMed.
- J. S. Swanstron, Cereal Chem., 1997, 74(4), 452–455 CrossRef.
- M. C. Miller, Anal. Chem., 1959, 31, 426–428 CrossRef.
- C. Li, X. Cao, Z. Gu and H. Wen, J. Sci. Food Agric., 2011, 91, 915–920 CrossRef CAS.
- M. M. Bradford, Anal. Biochem., 1976, 72, 248–257 CrossRef CAS.
- R. C. Agu and G. H. Palmer, J. Inst. Brew., 2003, 109, 110–113 CrossRef.
- I. M. Ahmed, A. F. Cao, Y. Han, U. A. Nadira, G. Zhang and F. Wu, Food Chem., 2013, 141, 2743–2750 CrossRef CAS.
|
This journal is © The Royal Society of Chemistry 2020 |